- 1Virginia Tech Carilion School of Medicine, Roanoke, VA, United States
- 2Section of Neurosurgery, Carilion Clinic, Roanoke, VA, United States
- 3School of Neuroscience, Virginia Polytechnic Institute and State University, Blacksburg, VA, United States
- 4Neurointerventional Surgery, Department of Radiology, Carilion Clinic, Roanoke, VA, United States
The authors sought to evaluate whether immunologic counts on admission were associated with shunt-dependent hydrocephalus following aneurysmal subarachnoid hemorrhage. A retrospective analysis of 143 consecutive patients with aneurysmal subarachnoid hemorrhage over a 9-year period was performed. A stepwise algorithm was followed for external ventricular drain weaning and determining the necessity of shunt placement. Data were compared between patients with and without shunt-dependent hydrocephalus. Overall, 11.19% of the cohort developed shunt-dependent hydrocephalus. On multivariate logistic regression analysis, acute hydrocephalus (OR: 61.027, 95% CI: 3.890–957.327; p = 0.003) and monocyte count on admission (OR: 3.362, 95% CI: 1.024–11.037; p = 0.046) were found to be independent predictors for shunt dependence. Receiver operating characteristic curve analysis for the prediction of shunt-dependent hydrocephalus confirmed that monocyte count exhibited an acceptable area under the curve (AUC = 0.737, 95% CI: 0.601–0.872; p < 0.001). The best predictive cutoff value to discriminate between successful external ventricular drain weaning and shunt-dependent hydrocephalus was identified as a monocyte count ≥0.80 × 103/uL at initial presentation. These preliminary data demonstrate that a monocyte count ≥0.80 × 103/uL at admission predicts shunt-dependent hydrocephalus in patients with aneurysmal subarachnoid hemorrhage; however, further large-scale prospective trials and validation are necessary to confirm these findings.
Introduction
Aneurysmal subarachnoid hemorrhage (aSAH) remains a life-threatening disease with an annual worldwide incidence of 7.9 cases per 100,000 individuals (1, 2). Indeed, aSAH is associated with a multitude of secondary neurologic sequelae, such as seizure, vasospasm, delayed cerebral ischemia, hydrocephalus, and, importantly, shunt-dependence. Recent research has begun to reveal the association between neuroinflammation and hydrocephalus after injury (i.e., hemorrhage or infection). Various molecular mediators [e.g., tumor necrosis factor-alpha (TNF-α) (3), transforming growth factor-beta 1 (4, 5), thrombin-induced transforming growth factor-beta (6), interleukin-6 (IL-6) (7), nuclear factor kappa B (8)] in the peripheral blood or cerebrospinal fluid have been correlated with a high likelihood of developing post-hemorrhagic or post-infectious hydrocephalus as well as the clinical severity of symptomatology. In the context of aSAH, there is growing evidence to suggest that an immune-mediated inflammatory response in the central nervous system is a key mechanism of early brain injury following rupture and associated clinical sequelae (9, 10). Data from pre-clinical and clinical studies have demonstrated the activation and proliferation of various leukocyte subsets (e.g., neutrophils, monocytes) following aSAH in murine models and humans (9–14). Previously, our group found that a neutrophil count ≥9.80 × 103/uL on admission was predictive of acute symptomatic hydrocephalus after aneurysmal subarachnoid hemorrhage in an adjusted multivariate logistic regression model (14). Secondary analysis demonstrated that 81.25% of patients who developed shunt dependence exhibited a neutrophil count ≥9.80 × 103/uL on admission (p = 0.003) (14). Here, we sought to further investigate this preliminary finding in an independent analysis to evaluate for potential associations between immunologic counts on admission and shunt-dependent hydrocephalus following aSAH.
Methods
Patient Selection
The present study was conducted with the approval of the Carilion Clinic Institutional Review Board. Informed consent was waived due to the retrospective nature of the research. We performed a retrospective study and analysis of all consecutive patients with aSAH over a 9-year period (2012–2020) admitted to the neurosurgery service at Carilion Roanoke Memorial Hospital. Patients with cisternal subarachnoid hemorrhage diagnosed on computed tomography (CT) of the head with a confirmed cerebral aneurysm on CT angiography or digital subtraction angiography were eligible for inclusion. In order to avoid confounding, we excluded patients with documented infections prior to admission, those who developed in-hospital infectious complications as well as those with known autoimmune diseases or prescribed immunosuppressants. Additionally, we excluded patients who transitioned to palliative care or died during their hospitalization. Patients who met inclusion criteria found to have incomplete data upon chart review were also excluded (Figure 1).
Management of Aneurysmal Subarachnoid Hemorrhage
Patients were managed according to the most recent Guidelines for the Management of Aneurysmal Subarachnoid Hemorrhage, as per the American Heart Association (15). Prompt treatment of the aneurysm was emphasized with either endovascular coil embolization or craniotomy for microsurgical clipping. Our multidisciplinary cerebrovascular team determined the appropriate treatment strategy for aneurysm obliteration on a case-by-case basis. Secondary sequelae of aSAH were managed aggressively in the intensive care unit including treatment of seizures, hydrocephalus, and vasospasm.
Indications for External Ventricular Drain Placement
Both clinical and radiographic features were considered for the decision to place an external ventricular drain (EVD) at the time of admission, including clinical manifestations (e.g., altered mental status, lethargy, somnolence, or pupillary changes) and radiographic evidence indicative of acute hydrocephalus (e.g., ventriculomegaly, increase in temporal horn size, cisternal effacement, sulcal effacement). Lumbar drains were not utilized for cerebrospinal fluid diversion.
Indications for Ventriculoperitoneal Shunt Placement
Our institution follows a stepwise algorithm to determine the necessity of shunt placement as described by Little et al. (16). External ventricular drains were initially set to 15 cm H2O after placement until the aneurysm was secured. Once secured, the EVD was opened between 0 and 10 cm H2O dependent upon clinical symptomatology attributable to hydrocephalus. Weaning of the EVD typically commenced within 1 week from the date of placement. For patients who developed symptomatic vasospasm requiring intervention, EVD weaning was postponed until vasospastic symptomatology had resolved. EVD weaning consisted of raising the height of the external collection reservoir over the course of several days by 5 cm H2O or less each day and to at least 25 cm H2O prior to clamping. The EVD was clamped for 24–48 h with continuous monitoring of intracranial pressure, frequent neurochecks, and a repeat CT scan to assess for recurrent hydrocephalus prior to removal. The EVD was reopened for the development of a new neurologic deficit or if intracranial pressure sustained above 22 mm Hg for > 10 min without stimulation. Weaning of the EVD was not attempted more than twice prior to considering shunt placement. Patients who tolerated weaning and removal of the EVD but subsequently developed recurrent clinical symptomatology consistent with hydrocephalus underwent a repeat CT scan and a lumbar puncture to evaluate for elevated pressure (> 22 cm H2O). Patients who failed EVD weaning, exhibited progressive enlargement of the ventricular system, or had elevated opening pressures via lumbar puncture were deemed candidates for placement of a ventriculoperitoneal shunt.
Clinical and Laboratory Parameters
We analyzed data on demographic parameters (age, sex, ethnicity), clinical status (Hunt and Hess grade), radiographic imaging (modified Fisher score, presence of intraventricular hemorrhage, aneurysm morphology), and laboratory data (neutrophil, lymphocyte, monocyte, hemoglobin A1C) on admission. Treatment modality (endovascular coil embolization or open craniotomy for microsurgical clipping) and incidence and severity of vasospasm during hospitalization were assessed as well as patients requiring ventriculoperitoneal shunt placement prior to discharge. Laboratory data utilized for analysis was obtained immediately upon admission. Monocyte count was recorded from the first venous blood test on admission expressed as 103 per microliter (103/uL). Angiographic vasospasm was graded based upon digital subtraction angiography images and according to severity as follows: mild (0–33%), moderate (34–66%), or severe (67–100%) vessel narrowing of any vessel within the cerebrovasculature irrespective of the patient's corresponding neurologic deficit. The primary outcome of this study was to evaluate for potential associations between immunologic counts on admission and shunt-dependent hydrocephalus in aSAH patients.
Statistical Analysis
XL STAT (Addinsoft) software was used to perform the statistical analyses. Chi-square test, Fisher's exact test, or Student's t-test were utilized to analyze clinical and laboratory data as appropriate. All variables with p-values < 0.05 in univariate analysis were included in the final multivariate logistic regression model. Vasospasm was dichotomized for multivariate analysis as none or mild vasospasm vs. moderate or severe vasospasm. We evaluated for interactions among all of the independent variables in the final model. Receiver operating characteristic (ROC) curve analysis was utilized to determine associations of monocyte count on admission with the primary outcome and evaluate the optimal cutoff value for outcome prediction. A p-value < 0.05 was considered statistically significant.
Results
Demographics and Clinical Features
Over the 9-year study period, 1,751 patients were diagnosed with subarachnoid hemorrhage of which 164 patients had aneurysmal subarachnoid hemorrhage. After applying inclusion and exclusion criteria, 143 patients met the eligibility criteria. The average documented time to presentation was 11.75 h. The majority of patients were female (81.82%) with a mean age of 54.99 ± 14.04 years. Of this cohort, Caucasians and African Americans represented 72.02% and 23.08% of patients, respectively. The vast majority of aneurysms (90.91%) were localized to the anterior circulation. Specifically, the anterior cerebral arteries (i.e., pre-communicating segment, communicating segment, post-communicating segment, pericallosal branch) represented the most common location of rupture (49.65%). Endovascular coil embolization was utilized in 81.12% of cases with the remaining undergoing open craniotomy for microsurgical clipping. A Hunt and Hess grade of two was most common representing 46.85% of patients while 57.34% exhibited a modified Fisher score of three. Intraventricular hemorrhage was observed in 39.86% of cases.
Shunt-Dependent Hydrocephalus
Acute symptomatic hydrocephalus was diagnosed in 56 patients (39.16%): all of which required emergent EVD placement for cerebrospinal fluid diversion. Of this cohort, 28.57% of patients failed EVD weaning and requirement shunt placement. Overall, 16 patients (11.19%) developed shunt-dependent hydrocephalus. Univariate analysis indicated multiple factors associated with shunt dependence following aSAH, including: intraventricular hemorrhage [46/127 (36.22%) vs. 11/16 (68.75%); p = 0.012], acute hydrocephalus [40/127 (31.50%) vs. 16/16 (100%); p < 0.001], incidence of vasospasm [9/127 (7.08%) vs. 7/16 (43.75%); p < 0.001], neutrophil count on admission [9.69 vs. 13.03; p = 0.002], monocyte count on admission (0.67 vs. 1.21; p < 0.001), prior ischemic stroke [10/127 (7.87%) vs. 6/16 (37.50%); p < 0.001], and a history of diabetes mellitus [4/127 (3.15%) vs. 4/16 (25.00%); p = 0.006] (Table 1).
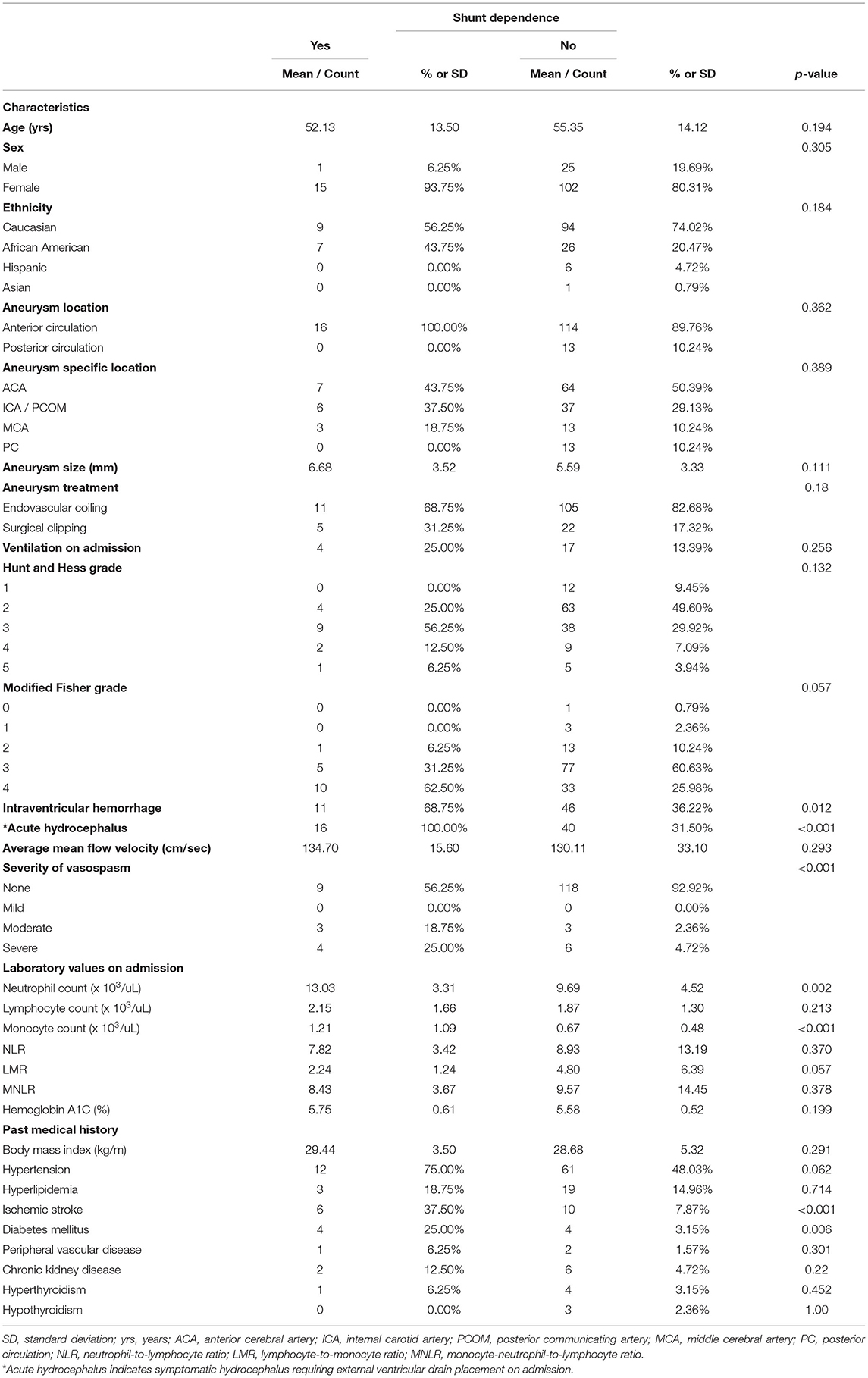
Table 1. Univariate analysis of predictors of shunt dependence after aneurysmal subarachnoid hemorrhage.
Regression analysis demonstrated that monocyte count on admission was positively associated with shunt-dependent hydrocephalus (OR: 2.838, 95% CI: 1.242–6.483; p = 0.013). After adjusting the model for intraventricular hemorrhage, acute hydrocephalus, moderate to severe vasospasm, neutrophil count on admission, prior ischemic stroke, and a history of diabetes mellitus, monocyte count on admission remained an independent predictor for shunt-dependent hydrocephalus following aSAH [OR: 3.362, 95% CI: 1.024–11.037; p = 0.046). Moreover, acute hydrocephalus (OR: 61.027, 95% CI: 3.890–957.327; p = 0.003) was independently associated with shunt dependence. All other variables failed to retain statistical significance in the multivariate model (Table 2).
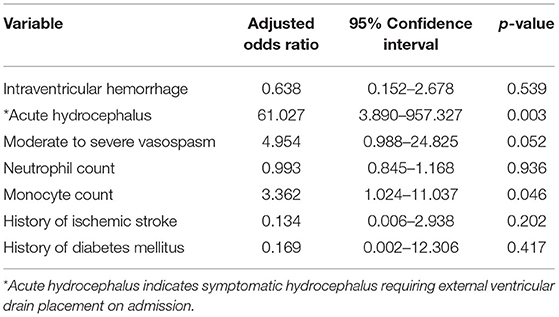
Table 2. Multivariate logistic regression analysis with adjusted odds ratios of shunt dependence after aneurysmal subarachnoid hemorrhage.
ROC analysis for the prediction of shunt-dependent hydrocephalus demonstrated that monocyte count exhibited an acceptable area under the curve (AUC = 0.737, 95% CI: 0.601–0.872; p < 0.001) (Figure 2). The best predictive cutoff value to discriminate between successful EVD weaning and shunt-dependent hydrocephalus was identified as a monocyte count ≥0.80 × 103/uL at initial presentation with a sensitivity and specificity of 81.25and 66.93%, respectively.
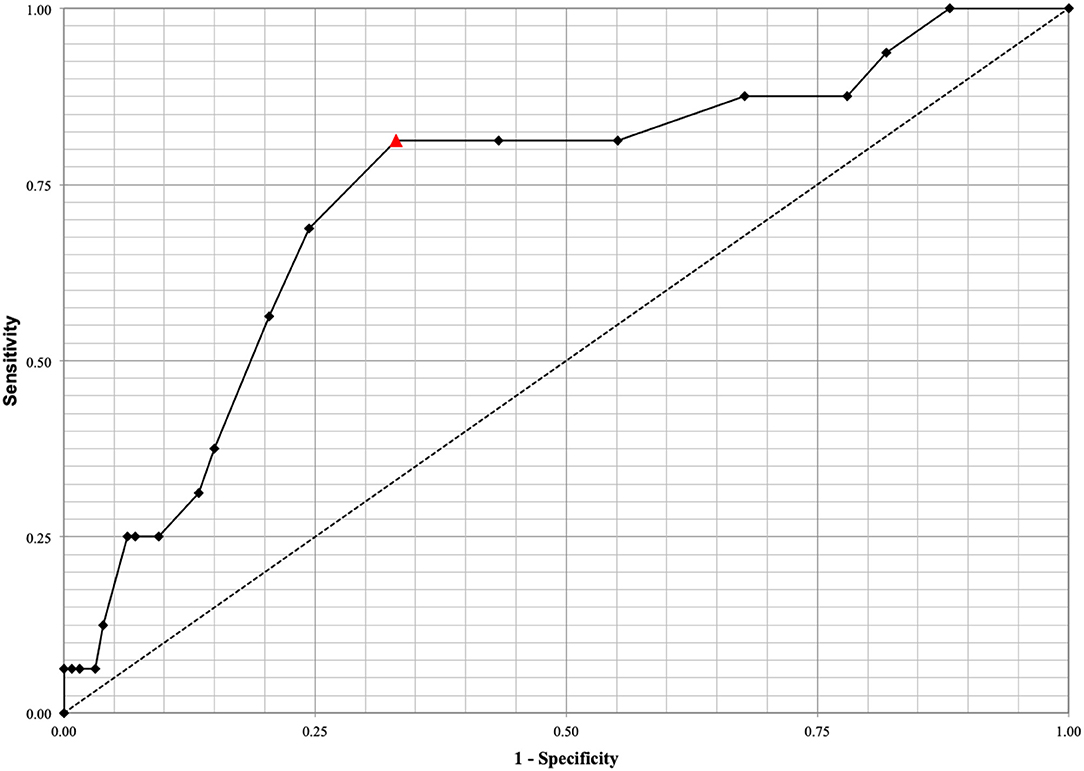
Figure 2. ROC analysis for the prediction of shunt-dependent hydrocephalus demonstrated that monocyte count exhibits an acceptable area under the curve (AUC = 0.737, 95% CI: 0.601–0.872, p < 0.001). The best predictive cutoff value to discriminate between successful EVD weaning and shunt-dependent hydrocephalus was identified at a monocyte count ≥0.80 × 103/uL with a sensitivity and specificity of 81.25% and 66.93%, respectively. The optimal cutoff value of monocyte count on admission is depicted by the red triangle.
Two supplemental multivariate analyses were performed on the cohort (n = 143) and included all statistically significant variables identified in the original univariate analysis with the exclusion of the acute hydrocephalus variable in the first additional analysis and the intraventricular hemorrhage variable in the second additional analysis. This was performed to investigate the statistical significance of the other variables given the fact that acute hydrocephalus was highly associated with shunt-dependence in the original multivariate analysis. In the first multivariate analysis (acute hydrocephalus variable excluded), moderate to severe vasospasm was significantly associated with shunt-dependence (OR: 6.397, 95% CI: 1.532–26.703; p = 0.011) (Table 3). Monocyte count trended toward significance (OR: 3.659, 95% CI: 0.93–14.4; p = 0.064). Best model selection based upon likelihood ratio associated moderate to severe vasospasm (OR: 6.794, 95% CI: 1.927–23.946; p = 0.003) and monocyte count (OR: 4.224, 95% CI: 1.164–15.33; p = 0.028) with shunt-dependence. In the second multivariate analysis (intraventricular hemorrhage variable excluded), acute hydrocephalus was significantly associated with shunt-dependence (OR: 45.868, 95% CI: 3.461–607.93; p = 0.004) (Table 4). Monocyte count trended toward significance (OR: 5.113, 95% CI: 0.978–26.732; p = 0.053). Best model selection based upon likelihood ratio associated acute hydrocephalus (OR: 53.9, 95% CI: 3.179–913.849; p = 0.006) and moderate to severe vasospasm (OR: 5.114, 95% CI: 1.352–19.35; p = 0.016) with shunt-dependence.
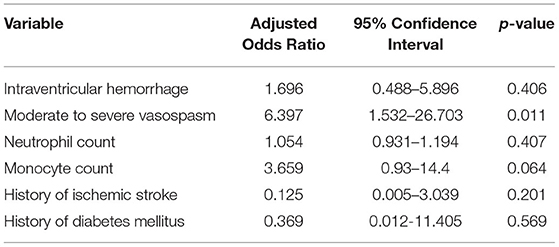
Table 3. Multivariate logistic regression analysis with adjusted odds ratios of shunt dependence after aneurysmal subarachnoid hemorrhage (acute hydrocephalus variable excluded).
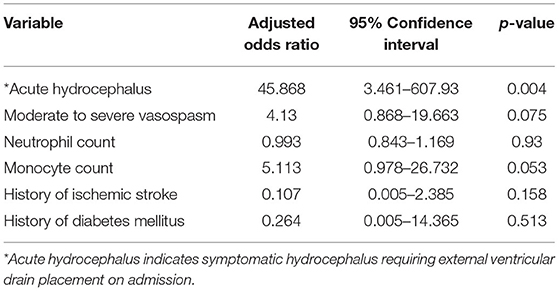
Table 4. Multivariate logistic regression analysis with adjusted odds ratios of shunt dependence after aneurysmal subarachnoid hemorrhage (intraventricular hemorrhage variable excluded).
Additional sub-cohort statistical analyses were performed for all patients exhibiting acute hydrocephalus (n = 56) as well as intraventricular hemorrhage (n = 57). Univariate analysis of the acute hydrocephalus sub-cohort indicated sex (p = 0.043), incidence of vasospasm (p = 0.026), monocyte count on admission (p = 0.013), and history of diabetes mellitus (p = 0.02) to be associated with shunt-dependence (Table 5). Univariate analysis of the intraventricular hemorrhage sub-cohort indicated acute hydrocephalus (p = 0.005), incidence of vasospasm (p = 0.007), neutrophil count on admission (p = 0.018), monocyte count on admission (p = 0.003), and prior ischemic stroke (p = 0.027) to be associated with shunt-dependence (Table 6). Multivariate analyses failed to reveal statistically significant relationships given the small sample size of each sub-cohort.
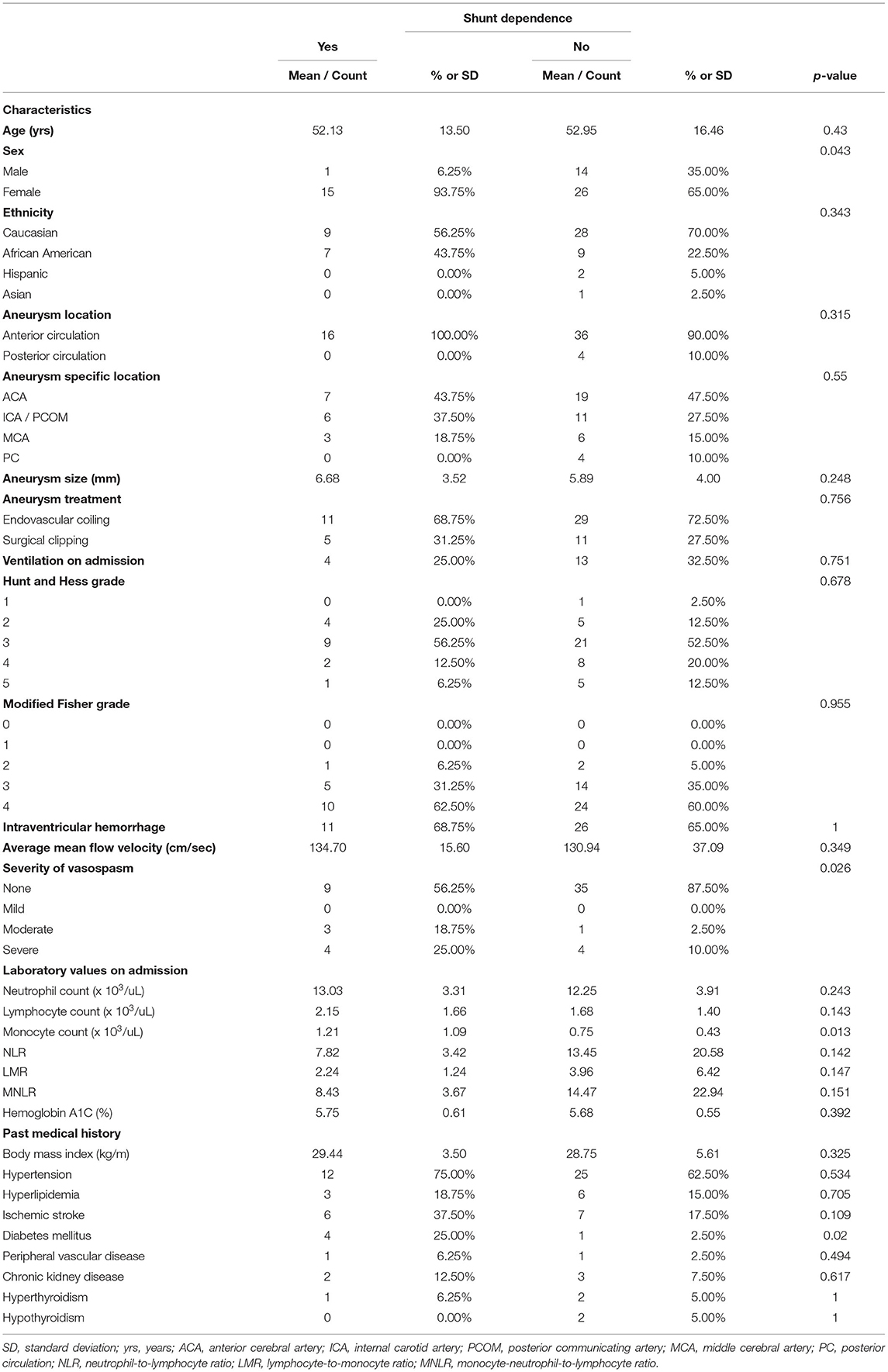
Table 5. Sub-cohort univariate analysis of predictors of shunt dependence after aneurysmal subarachnoid hemorrhage in patients with acute hydrocephalus.
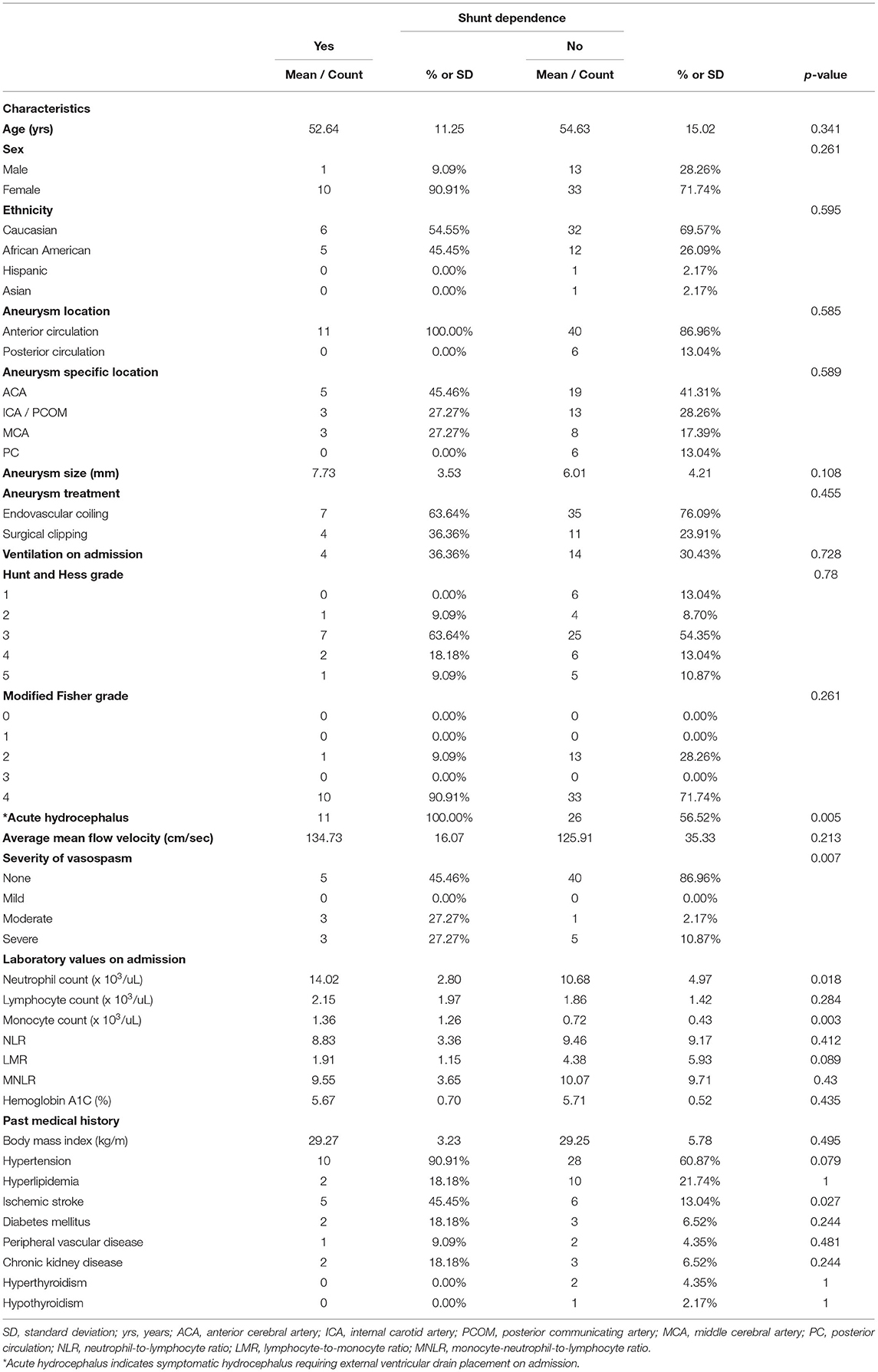
Table 6. Sub-cohort univariate analysis of predictors of shunt dependence after aneurysmal subarachnoid hemorrhage in patients with intraventricular hemorrhage.
Discussion
In the present study, we identified independent predictors for shunt dependence in a consecutive cohort of patients with aSAH. On multivariate logistic regression analysis, monocyte count on admission and acute hydrocephalus were found to be independent risk factors for shunt dependence after aSAH. These data were irrespective of the clinical or radiographic grades of injury burden.
Thirty-nine percent of patients required placement of an EVD for treatment of acute symptomatic hydrocephalus. Of the cohort requiring cerebrospinal fluid diversion, 28.57% of patients developed shunt dependence. Overall, 11.19% included in the study developed shunt-dependent hydrocephalus. Our rate of shunt dependence is similar to prior reports, which range between 6 and 67% (17–19). Prior studies have found an association between shunt dependence and a multitude of clinical and radiographic variables including age (17), sex (18), Hunt and Hess grade ≥4 (19), Fisher grade 4 (20), bicaudate index of at least 0.20 (20), acute hydrocephalus (17–19), presence of intraventricular hemorrhage (19, 20), posterior circulation aneurysms (17, 19), giant aneurysms (17), vasospasm (18), ventilation on admission (17), sustained systemic inflammatory response syndrome criteria (18) ≥78 cc/day of cerebrospinal fluid drainage (21) as well as ≥1,500 cc of cerebrospinal fluid drainage during the first week after ictus (22), among others. Our univariate analysis corroborated several of these traditional factors associated with shunt dependence including acute hydrocephalus, presence of intraventricular hemorrhage, and incidence of vasospasm. However, after accounting for monocyte count on admission, only acute hydrocephalus retained statistical significance in the adjusted multivariate model. Additional novel findings of the present report are the associations of neutrophil count on admission, prior ischemic stroke, and a history of diabetes mellitus with shunt dependence; however, all of these variables failed to retain statistical significance in the multivariate model.
These preliminary data invoke several questions regarding the association between monocyte count and shunt dependence in aSAH patients including: (i) what are the clinical implications of increased monocyte count on admission, (ii) can monocyte count on admission be used as a biomarker for shunt-dependent hydrocephalus, and (iii) do monocytes contribute to the inflammatory changes associated with impaired cerebrospinal fluid dynamics following aSAH? Regarding clinical implications, our preliminary data have shown that monocyte count on admission predicts shunt dependence after aSAH. Moreover, prior studies have shown an association between monocyte counts on admission or monocyte-based inflammatory indices with unfavorable functional outcomes and cerebral infarction (23, 24). Feghali et al. (23) showed that the monocyte-neutrophil-to-lymphocyte ratio and lymphocyte-to-monocyte ratio, but not the neutrophil-to-lymphocyte ratio, to be independently associated with unfavorable functional outcomes (mRS of 3–6) at 12–18 months following discharge. Moreover, the authors reported the lymphocyte-to-monocyte ratio to be significantly associated with vasospasm (23). Unda et al. (24) found a relationship between monocyte count on admission with cerebral infarction and unfavorable functional outcomes (mRS of 3–6) at 12 months after discharge. Indeed, the present report along with the aforementioned studies has begun to demonstrate a preliminary association between monocytes and clinical sequelae following aSAH.
Immune characterization in aSAH has found that monocytes exhibit a fundamental role in propagating a pro-inflammatory state after aneurysm rupture (10–12). Data from pre-clinical and clinical studies have demonstrated the activation and proliferation of pro-inflammatory monocytes following aSAH in murine models and humans (10–12). Gris et al. (10) found an early increase in recruitment of pro-inflammatory monocytes within the first 48 h after aSAH in a murine model. Moreover, in a murine model, the authors found an increase of circulating and brain monocytes and brain neutrophils following aSAH (10). Mohme et al. (11) showed that systemic and intrathecal immune activation and secondary immune dissemination of non-classical monocytes (i.e., pro-inflammatory, CD14+, CD16++) exhibit a critical role in aSAH-induced neuroinflammation and delayed cerebral ischemia in humans. Moreover, in the same study, ex-vivo analysis demonstrated that non-classical monocytes were the major monocytic cell population in the early phase (day 1–4) after aSAH (11). Korostynski et al. (12) found that rupture of an intracranial aneurysm significantly increases monocyte activity while depressing the lymphocyte response in both cellular composition and gene expression profiles. These data have implicated monocytes in the pathogenesis of neuroinflammation following aSAH.
The etiopathogenesis of shunt dependence following aSAH is likely a multifactorial process (3–8, 18). In the context of neuroinflammation, it is known that non-classical monocytes are the main source of pro-inflammatory cytokines, such as TNF- α, IL-6, and interleukin 1 beta: all of which are elevated in serum and cerebrospinal fluid following aSAH and contribute to the inflammatory process (18, 25–28). Moreover, elevated cerebrospinal fluid IL-6 is a known independent predictor for shunt dependence after aSAH (7). Might non-classical monocytes be responsible, to some degree, for neuroinflammation and consequential shunt dependence following aSAH? Future prospective studies examining non-classical monocyte levels within the cerebrospinal fluid and the expression of pro-inflammatory cytokines in the context of aSAH may determine the validity of this relationship.
There are several limitations to our study. This study lacks a prospective design and is limited to a single-center experience. Despite accounting for confounding variables, our findings may still overestimate or underestimate causal relationships. Although our study cohort had a reasonable number of subjects, an increased sample size may have yielded additional statistically significant associations. We limited our investigation to laboratory data on the date of admission. We cannot decipher whether only the acute increase in monocyte count on admission exhibits predictive value or if additional changes in monocyte count in the days or weeks following aneurysmal rupture plays any role. Moreover, we could not definitively determine time to presentation. Although the average documented time to presentation was 11.75 h, this was entirely dependent upon retrospective chart review including physician, resident, nurse, emergency medical technician, and transfer center notes. We could not determine if such information was obtained from the patient, family, or other means. As such, this is a limitation of the retrospective design of our study, which could be addressed with a prospective design in the future. Lastly, several studies in the literature have identified intraventricular hemorrhage as a risk factor for the development of shunt-dependent hydrocephalus (19, 20). While our univariate analysis confirmed this relationship, intraventricular hemorrhage failed to retain statistical significance in our multivariate analysis. We initially attributed this to the fact that intraventricular hemorrhage and acute hydrocephalus were entered together in the multivariate analysis with acute hydrocephalus becoming more statistically significant and intraventricular hemorrhage losing significance consequentially. However, a secondary multivariate analysis with the exclusion of the acute hydrocephalus variable failed to reestablish intraventricular hemorrhage as a statistically significant factor. An additional multivariate analysis with the exclusion of three variables (i.e., acute hydrocephalus, vasospasm, monocyte count on admission) reestablished intraventricular hemorrhage (p = 0.05) as a statistically significant predictor of shunt-dependence.
Conclusions
In our retrospective study, a monocyte count ≥0.80 × 103/uL at admission was found to be predictive of shunt-dependent hydrocephalus in patients with aSAH. Knowledge of monocyte count on admission may help predict the occurrence of shunt-dependent hydrocephalus; however, these data should be viewed as preliminary results as the validity of this association will be dependent on future larger prospective studies.
Data Availability Statement
The datasets presented in this article are not readily available because these data are protected by the Institutional Review Board of Carilion Clinic (IRB-20-1059). Requests to access the datasets should be directed to JC, jacuoco@carilionclinic.org.
Ethics Statement
The studies involving human participants were reviewed and approved by Institutional Review Board of Carilion Clinic (IRB 20-1059; Approved on 10/13/2020). Written informed consent for participation was not required for this study in accordance with the national legislation and the institutional requirements.
Author Contributions
JC, EG, BK, MW, EM, BP, and JE: conceptualization, methodology, validation, formal analysis, investigation, resources, writing–review and editing, and visualization. JC and EG: data curation. JC: writing–original draft preparation. MW, EM, BP, and JE: supervision. JE: project administration. All authors contributed to the article and approved the submitted version.
Conflict of Interest
The authors declare that the research was conducted in the absence of any commercial or financial relationships that could be construed as a potential conflict of interest.
Publisher's Note
All claims expressed in this article are solely those of the authors and do not necessarily represent those of their affiliated organizations, or those of the publisher, the editors and the reviewers. Any product that may be evaluated in this article, or claim that may be made by its manufacturer, is not guaranteed or endorsed by the publisher.
References
1. Etminan N, Chang HS, Hackenberg K, de Rooij NK, Vergouwen MDI, Rinkel GJE, et al. Worldwide incidence of aneurysmal subarachnoid hemorrhage according to region, time period, blood pressure, and smoking prevalence in the population: a systematic review and meta-analysis. JAMA Neurol. (2019) 76:588–97. doi: 10.1001/jamaneurol.2019.0006
2. Oppong MD, Buffen K, Pierscianek D, Herten A, Ahmadipour Y, Dammann P, et al. Secondary hemorrhagic complications in aneurysmal subarachnoid hemorrhage: when the impact hits hard. J Neurosurg. (2020) 132:79–86. doi: 10.3171/2018.9.JNS182105
3. Sharma S, Goyal MK, Sharma K, Modi M, Sharma M, Khandelwal N, et al. Cytokines do play a role in pathogenesis of tuberculous meningitis: a prospective study from a tertiary care center in India. J Neurol Sci. (2017) 379:131–6. doi: 10.1016/j.jns.2017.06.001
4. Kitazawa K, Tada T. Elevation of transforming growth factor-beta 1 level in cerebrospinal fluid of patients with communicating hydrocephalus after subarachnoid hemorrhage. Stroke. (1994) 25:1400–4. doi: 10.1161/01.STR.25.7.1400
5. Whitelaw A, Christie S, Pople I. Transforming growth factor-beta1: a possible signal molecular for posthemorrhagic hydrocephalus? Pediatr Res. (1999) 46:576–80. doi: 10.1203/00006450-199911000-00014
6. Li T, Zhang P, Yuan B, Zhao D, Chen Y, Zhang X. Thrombin-induced TGF-ß1 pathway: a cause of communicating hydrocephalus post subarachnoid hemorrhage. Int J Mol Med. (2013) 31:660–6. doi: 10.3892/ijmm.2013.1253
7. Wostrack M, Reeb T, Martin J, Kehl V, Shiban E, Preuss A, et al. Shunt-dependent hydrocephalus after aneurysmal subarachnoid hemorrhage: the role of intrathecal interleukin-6. Neurocrit Care. (2014) 21:78–84. doi: 10.1007/s12028-014-9991-x
8. Simard PF, Tosun C, Melnichenko L, Ivanova S, Gerzanich V, Simard JM. Inflammation of the choroid plexus and ependymal layer of the ventricle following intraventricular hemorrhage. Transl Stroke Res. (2011) 2:227–31. doi: 10.1007/s12975-011-0070-8
9. Zhang Z, Fang Y, Lenahan C, Chen S. The role of immune inflammation in aneurysmal subarachnoid hemorrhage. Exp Neurol. (2021) 336:113535. doi: 10.1016/j.expneurol.2020.113535
10. Gris T, Laplante P, Thebault P, Cayrol R, Najjar A, Joannette-Pilon B, et al. Innate immunity activation in the early brain injury period following subarachnoid hemorrhage. J Neuroinflammation. (2019) 16:253. doi: 10.1186/s12974-019-1629-7
11. Mohme M, Sauvigny T, Mader MMD, Schweingruber N, Maire CL, Runger A, et al. Immune characterization in aneurysmal subarachnoid hemorrhage reveals distinct monocytic activation and chemokine patterns. Transl Stroke Res. (2020) 11:1348–61. doi: 10.1007/s12975-019-00764-1
12. Korostynski M, Piechota M, Morga R, Hoinkis D, Golda S, Zygmunt M, et al. Systemic response to rupture of intracranial aneurysms involves expression of specific gene isoforms. J Transl Med. (2019) 17:141. doi: 10.1186/s12967-019-1891-6
13. Kushamae M, Miyata H, Shirai M, Shimizu K, Oka M, Koseki H, et al. Involvement of neutrophils in machineries underlying the rupture of intracranial aneurysms in rats. Sci Rep. (2020) 10:20004. doi: 10.1038/s41598-020-74594-9
14. Cuoco JA, Guilliams EL, Klein BJ, Benko MJ, Darden JA, Olasunkanmi AL, et al. Neutrophil count on admission predicts acute symptomatic hydrocephalus after aneurysmal subarachnoid hemorrhage. World Neurosurg. (2021) 156:e338–44. doi: 10.1016/j.wneu.2021.09.059
15. Connolly ES Jr, Rabinstein AA, Carhuapoma JR, Derdeyn CP, Dion J, Higashida RT, et al. Guidelines for the management of aneurysmal subarachnoid hemorrhage: a guideline for healthcare professionals from the American Heart Association/American Stroke Association. Stroke. (2012) 43:1711–37. doi: 10.1161/STR.0b013e3182587839
16. Little AS, Zabramski JM, Peterson M, Goslar PW, Wait SD, Albuquerque FC, et al. Ventriculoperitoneal shunting after aneurysmal subarachnoid hemorrhage: analysis of the indications, complications, and outcome with a focus on patients with borderline ventriculomegaly. Neurosurgery. (2008) 62:618–27. doi: 10.1227/01.neu.0000317310.62073.b2
17. O'Kelly CJ, Kulkarni AV, Austin PC, Urbach D, Wallace MC. Shunt-dependent hydrocephalus after aneurysmal subarachnoid hemorrhage: incidence, predictors, and revision rates. Clinical article. J Neurosurg. (2009) 111:1029–35. doi: 10.3171/2008.9.JNS08881
18. Wessell AP, Kole MJ, Cannarsa G, Oliver J, Jindal G, Miller T, et al. A sustained systemic inflammatory response syndrome is associated with shunt-dependent hydrocephalus after aneurysmal subarachnoid hemorrhage. J Neurosurg. (2018) 130:1984–91. doi: 10.3171/2018.1.JNS172925
19. Jabbarli R, Bohrer AM, Pierscianek D, Muller D, Wrede KH, Dammann P, et al. The CHESS score: a simple tool for early prediction of shunt dependency after aneurysmal subarachnoid hemorrhage. Eur J Neurol. (2016) 23:912–8. doi: 10.1111/ene.12962
20. Rincon F, Gordon E, Starke RM, Buitrago MM, Fernandez A, Schmidt JM, et al. Predictors of long-term shunt-dependent hydrocephalus after aneurysmal subarachnoid hemorrhage. Clinical article. J Neurosurg. (2010) 113:774–80. doi: 10.3171/2010.2.JNS09376
21. Tso MK, Ibrahim GM, Macdonald RL. Predictors of shunt-dependent hydrocephalus following aneurysmal subarachnoid hemorrhage. World Neurosurg. (2016) 86:226–32. doi: 10.1016/j.wneu.2015.09.056
22. Erixon HO, Sorteberg A, Sorteberg W, Eide PK. Predictors of shunt dependency after aneurysmal subarachnoid hemorrhage: results of a single-center clinical trial. Acta Neurochir. (2014) 156:2059–69. doi: 10.1007/s00701-014-2200-z
23. Feghali J, Kim J, Gami A, Rapaport S, Caplan JM, McDougall CG, et al. Monocyte-based inflammatory indices predict outcomes following aneurysmal subarachnoid hemorrhage. Neurosurg Rev. (2021). doi: 10.1007/s10143-021-01525-1
24. Unda SR, Birnbaum J, Labagnara K, Wong M, Vaishnav DP, Altschul DJ. Peripheral monocytosis at admission to predict cerebral infarct and poor functional outcomes in subarachnoid hemorrhage patients. World Neurosurg. (2020) 138:e523–9. doi: 10.1016/j.wneu.2020.02.170
25. Wong KL, Yeap WH, Tai JJY, et al. The three human monocyte subsets: implications for health and disease. Immunol Res. (2012) 53:41–57. doi: 10.1007/s12026-012-8297-3
26. Wong KL, Tai JJY, Wong WC, Han H, Sem X, Yeap WH, et al. Gene expression profiling reveals the defining features of the classical, intermediate, and nonclassical human monocyte subsets. Blood. (2011) 118:e16–31. doi: 10.1182/blood-2010-12-326355
27. Yang J, Zhang L, Yu C, Yang XF, Wang H. Monocyte and macrophage differentiation: circulation inflammatory monocyte as biomarker for inflammatory diseases. Biomark Res. (2014) 2:1. doi: 10.1186/2050-7771-2-1
Keywords: aneurysmal subarachnoid hemorrhage, immune system, hydrocephalus, monocyte, shunt-dependence
Citation: Cuoco JA, Guilliams EL, Klein BJ, Witcher MR, Marvin EA, Patel BM and Entwistle JJ (2022) Monocyte Count on Admission Is Predictive of Shunt-Dependent Hydrocephalus After Aneurysmal Subarachnoid Hemorrhage. Front. Surg. 9:879050. doi: 10.3389/fsurg.2022.879050
Received: 18 February 2022; Accepted: 04 April 2022;
Published: 28 April 2022.
Edited by:
Alfio Spina, San Raffaele Hospital (IRCCS), ItalyReviewed by:
Myung-Hoon Han, Hanyang University Guri Hospital, South KoreaTijana Nastasovic, University of Belgrade, Serbia
Copyright © 2022 Cuoco, Guilliams, Klein, Witcher, Marvin, Patel and Entwistle. This is an open-access article distributed under the terms of the Creative Commons Attribution License (CC BY). The use, distribution or reproduction in other forums is permitted, provided the original author(s) and the copyright owner(s) are credited and that the original publication in this journal is cited, in accordance with accepted academic practice. No use, distribution or reproduction is permitted which does not comply with these terms.
*Correspondence: Joshua A. Cuoco, jacuoco@carilionclinic.org