- 1Department of Neuroscience “Rita Levi Montalcini”—Unit of Neurosurgery, University of Turin, Turin, Italy
- 2Humanitas Gradenigo, Turin, Italy
- 3Dipartimento di Medicina e Chirurgia - Neurochirurgia, Università degli Studi di Milano Bicocca, Milan, Italy
- 4Fondazione Istituto di Ricovero e Cura a Carattere Scientifico (IRCCS) Policlinico San Matteo, Pavia, Italy
- 5UpSurgeOn S.r.l., Assago, Italy
Background: In the recent years, growing interest in simulation-based surgical education has led to various practical alternatives for medical training. More recently, courses based on virtual reality (VR) and three-dimensional (3D)-printed models are available. In this paper, a hybrid (virtual and physical) neurosurgical simulator has been validated, equipped with augmented reality (AR) capabilities that can be used repeatedly to increase familiarity and improve the technical skills in human brain anatomy and neurosurgical approaches.
Methods: The neurosurgical simulator used in this study (UpSurgeOn Box, UpSurgeOn Srl, Assago, Milan) combines a virtual component and a physical component with an intermediate step to provide a hybrid solution. A first reported and evaluated practical experience on the anatomical 3D-printed model has been conducted with a total of 30 residents in neurosurgery. The residents had the possibility to choose a specific approach, focus on the correct patient positioning, and go over the chosen approach step-by-step, interacting with the model through AR application. Next, each practical surgical step on the 3D model was timed and qualitatively evaluated by 3 senior neurosurgeons. Quality and usability-grade surveys were filled out by participants.
Results: More than 89% of the residents assessed that the application and the AR simulator were very helpful in improving the orientation skills during neurosurgical approaches. Indeed, 89.3% of participants found brain and skull anatomy highly realistic during their tasks. Moreover, workshop exercises were considered useful in increasing the competency and technical skills required in the operating room by 85.8 and 84.7% of residents, respectively. Data collected confirmed that the anatomical model and its application were intuitive, well-integrated, and easy to use.
Conclusion: The hybrid AR and 3D-printed neurosurgical simulator could be a valid tool for neurosurgical training, capable of enhancing personal technical skills and competence. In addition, it could be easy to imagine how patient safety would increase and healthcare costs would be reduced, even if more studies are needed to investigate these aspects. The integration of simulators for training in neurosurgery as preparatory steps for the operating room should be recommended and further investigated given their huge potential.
Introduction
In recent years, growing interest in simulation-based surgical education has led to various practical alternatives for medical training (1). Factors such as the cost-effectiveness of the operating room, medico-legal and ethics implications, the actual restrictions for residents in terms of hours spent on work-related activities, and reduced time availability for surgical instruction during surgical activities are the elements most likely connected with a reduction in operative case volume during residency (2). After recent changes in disease management and technological advances, especially for surgical subspecializations, a growing attention has been paid to patient safety during surgical care, keeping back residents from the operating rooms while redirecting them into more administrative mansions (3).
On the other hand, up to 50,000 additional neurosurgeons are estimated worldwide to face the critically growing needs in surgical care (4). A net discrepancy between the need for new experienced neurosurgeons and their actual surgical experience is coming to light over time.
Traditionally, practical solutions for surgical training implementation include cadaveric and animal models and abroad fellowships (2, 3). However, some drawbacks and limitations must be taken into account. Neurosurgical anatomy is highly specific for human brain and does not compare well with animal specimens. Moreover, the management of cadaveric models entails ethical concerns and high maintenance costs, as well as specific and highly equipped structures for their preservation. In all these cases, practical training on these models allows only a partial anatomical practice, excluding the pathologic aspects of neurosurgical experience.
Nowadays, international training for surgical residents is considered desirable for a complete neurosurgical education in this particular medical field (5). Unfortunately, after the COVID-19 pandemic outbreak, surgical activities and international exchange programs have been dramatically reduced (6–8).
To counter the lack of surgical exposure, more recently, courses based on virtual reality (VR) and three-dimensional (3D)-printed models are available (2, 3). In particular, we validated a hybrid (virtual and physical) neurosurgical simulator equipped with augmented reality (AR) capabilities that can be used repeatedly to increase familiarity and improve technical skills in human brain anatomy and neurosurgical approaches.
Materials and Methods
The neurosurgical simulator used in this study (UpSurgeOn Box, UpSurgeOn Srl, Assago, Milan) combines a virtual and a physical component with an intermediate step to provide a hybrid solution. The virtual part is based on an application which allows for the interactive exploration of 3D anatomical models and animations, both in a purely virtual environment and in an AR projection of the physical simulator (hybrid). These tools are designed to be integrated into a 3-step training sequence: mental training (based on a virtual environment), hybrid training (based on virtual models projected onto the physical simulator through a mobile device), and manual training (based on the physical simulator).
Virtual Reality
Through the “Neurosurgery” mobile application, it is possible to explore the different surgical approaches using a smartphone or tablet.
A total of 9 surgical approaches (pterional, mini pterional, frontal monolateral, supraorbital, temporal, mini temporal, retrosigmoid, mini retrosigmoid, interhemispheric, and suboccipital) and 2 pathological modules for brain aneurysms and pituitary adenomas are available. Each virtual exploration uses 3D anatomical models and animations to support a Mental Training system aimed at understanding a patient's positioning according to a specific craniotomy and a specific intradural target.
The study of each approach in 3D mode is divided into 6 phases (Figures 1A–D) as follows:
1. Craniotomy selection: One can select among pterional, mini pterional, frontal monolateral, supraorbital, temporal, mini temporal, retrosigmoid, mini retrosigmoid, interhemispheric, and suboccipital approach.
2. Target selection: One can select specific anatomical (non-pathological) structures visible from the craniotomy previously selected.
3. Patient positioning: An artificial intelligence (AI)-based system calculates in real time a range of correct patient's body/head positionings according to the craniotomy and the target selected.
4. Surgical approach: This step involves an interactive 3D animation of the surgical steps of the approach.
5. Microsurgical exploration: This step simulates the microscope view using the gyroscopic technology of the hardware. Furthermore, tissues can be deformed by tapping the screen to expose the deep anatomy.
6. Closure: An interactive step that explains, through an animation, the reconstruction technique.
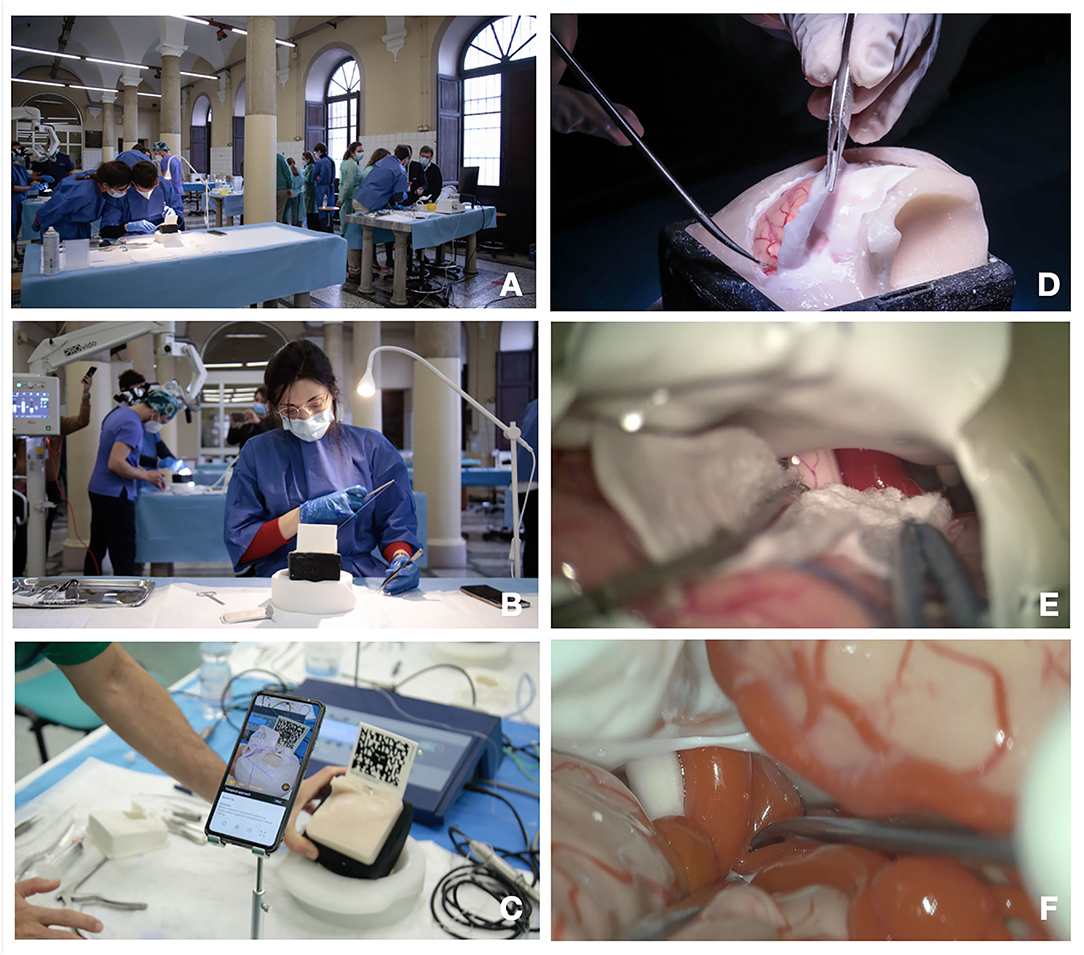
Figure 1. (A–E) Training steps. (A) Training laboratory set up; (B) suturing exercise; (C) augmented reality (AR) for craniotomy and approach planning; (D) dura opening on the pterional approach simulator box; (C) microscopic intradural phase through pterional approach with gentle brain retraction; (E,F) microscopic exploration and dissection through pterional approach of the carotid artery, optic nerve, and sylvian vessels.
Augmented Reality
The application software presents a module dedicated to the use of AR able to project 3D anatomical models and animations on the physical simulator, acting as a guide to plan the hands-on surgical approach (Figure 2).
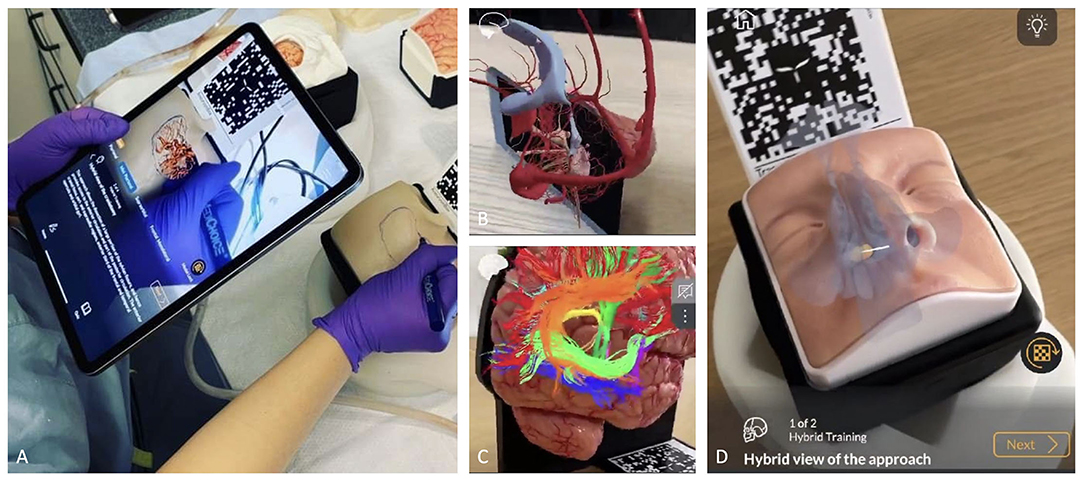
Figure 2. (A-D) The role of AR and the hybrid simulation. (A) AR of a pterional approach. Before performing the craniotomy, it is possible to see the vessels and the brain under the skull by framing the specific QR code through tablet and/or smartphone app; (B) brain parenchyma has been hidden to allow better evaluation of dural venous system and ventricular system; (C) AR used to better evaluate white matter fiber anatomy; (D) application of AR in endoscopic endonasal approach allows to identify anatomical landmarks before entering the nose.
This phase, defined by the term “Hybrid Training,” includes:
1. Patient positioning in AR: using AR it is possible to view the patient's entire skull on the simulator and modify the position of the head on the three axis. The AR system also allows to view the position of the target on the screen, before visualizing it on the physical simulator.
2. Craniotomy planning in AR: the Application projects on the physical skull several possible craniotomies.
3. Surgical steps in AR: the software reproduces the surgical approach step by step to understand which tasks will be carried out on the physical model.
Physical Simulator
The simulator is designed to reproduce different surgical approaches (pterional, temporal, retrosigmoid, interhemispheric, suboccipital, and transsphenoidal) and different patient positions via a semi-spheric support. Through interchangeable skulls, one can perform multiple craniotomies and dural openings using the same deep microanatomical scenario, which can be explored under the microscope/exoscope/endoscope once the skull and dura are opened (Figures 1E,F, 3).
Once the craniotomy has been performed, it is then possible to replace the skull and start again.
Data Collection
The first reported and evaluated practical experience on the anatomical 3D-printed model was conducted at the Department of Neuroscience “Rita Levi Montalcini” in Turin, Italy.
A total of 30 neurosurgery residents were involved. The first phase of the workshop consisted in using the AR application. The residents had the possibility to choose a specific approach (pterional, subtemporal, retrosigmoid), to focus on the correct patient positioning, and to go over the chosen approach step-by-step by interacting with the model through AR application.
Next, each practical surgical step on the 3D model was timed and qualitatively evaluated by 3 senior neurosurgeons. The training comprehended 4 standardized surgical moments: craniotomy, opening of the dura mater, reaching the pre-assigned target, and closing of the dura mater. Surgical loupes and a surgical microscope were available. For the pterional approach, optic nerve, middle cerebral artery (MCA), and III cranial nerve were identified as the principal targets of interest. Similarly, the IV cranial nerve and posterior cerebral artery (PCA) were chosen for the subtemporal approach, while VII-VIII, V cranial nerves, and superior cerebral artery (SCA) were identified for the retrosigmoid approach.
At the end of the workshop, quality and usability-grade surveys were filled out by participants. The second questionnaire was a modified version of the System Usability Scale (9). Each answer was graded by a 5-point scale (Likert score) (10). Incomplete questionnaires were excluded, and a total of 28 forms were considered for the assessment.
Results
Descriptive results regarding stratifications by year of neurosurgery residents who participated in the surveys are reported in Figure 4A. In addition, the number of craniotomies and the number of previous cadaveric labs performed were assessed to define the experience level of the audience (Figures 4B,C). The results of the survey about teaching effectiveness and quality model are summarized in Table 1. More than 89% (agree and strongly agree) of the residents assessed that the application and the AR simulator were very helpful in improving the orientation skills during neurosurgical approaches. Indeed, 89.3% of the participants found brain and skull anatomy highly realistic during their tasks. Moreover, workshop exercises were considered useful in increasing the competency and technical skills required in operating room, by 85.8 and 84.7% of residents, respectively.
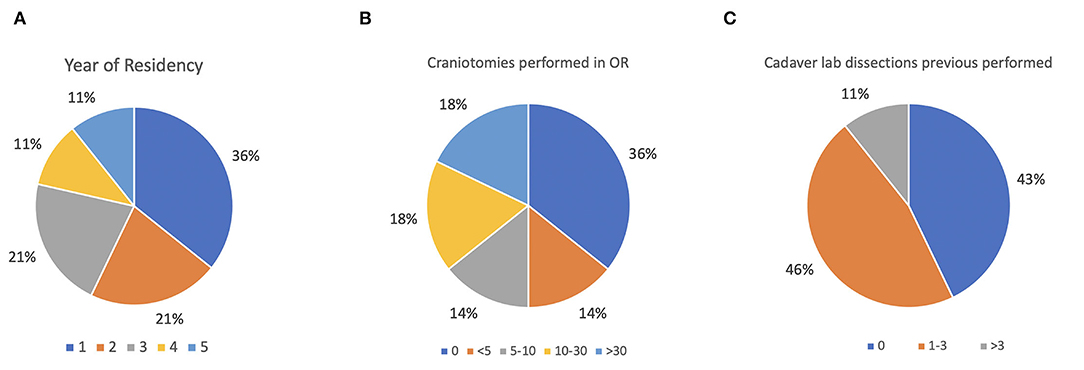
Figure 4. (A–C) Baseline assessment of the audience. (A) Stratification by year of neurosurgery residents; (B) assessment of performed craniotomies; (C) assessment of attended cadaver labs.
On the other side, tactile feedback of the brain tissue and dura mater consistency were found as relative weaknesses of anatomical models with a mean score of 3 points (neutral). In particular, the most critical issue found was the lower elasticity and higher hardness and tension of the anatomical model compared to normal parenchyma.
Interestingly, when the residents were asked, 85.7% agreed with the possibility to include this kind of training into a standard surgical training for neurosurgeons (4 and 5 scores).
Data collected with the second survey confirmed that the anatomical model and its application were intuitive, well integrated, and easy to use. The results of grade of usability questionnaire about anatomy models are summarized in Table 2.
Moreover, although the aim of the study was not a quantitative analysis, data regarding length of exercises and performance qualitative evaluation are reported in Tables 3, 4. Results showed that residents attending the last 2 years of residency performed the various skills quicker and with higher quality than younger residents. However, no significant comparisons or analysis could be made on these data because of the heterogeneity of surgical approach among different groups of residents.
Discussion
Neurosurgery residency is characterized by high levels of competence and an intense hands-on experience. Due to monetary restrictions, infrastructure conditions, and recent work time restrictions (11), it is hard for a resident to reach an adequate operative case volume over the education program (3). To counter these drawbacks in neurosurgical training, cadaveric specimen (12) and animal model courses (13) are some of the common alternatives for the improvement of surgical skills in neurosurgery, even if their high maintenance costs and ethical issues represent some of the principal limitations to date (14). Some abroad experiences or post-graduate surgical courses are still valid options, but recently, after the COVID-19 pandemic, international exchange programs have been dramatically reduced (6, 7). Also, learning new surgical skills is different from perfecting them, which implies that they have to be repeated with constancy over time (15). The neurosurgical learning curve is still too long and dominated by conventional mentor–apprentice relationships (16).
In this scenario, neurosurgical simulators are becoming increasingly important. Among the modern surgical training solutions, interest in VR or AR and 3D models has been growing (16, 17). Our experience with hybrid AR and 3D-printed neurosurgical simulator showed that the combination of such learning methods could lead to interesting results. Specifically, hybrid AR represents a helpful tool to guide young neurosurgeons from notional knowledge to practical experience. Indeed, after an anatomical revise, residents can focus on the correct patient positioning and go over the chosen approach step-by-step by interacting with the model through AR application. In the second phase of the simulation, high detailed 3D-printed neurosurgical models allow trainees to obtain immediate feedback of the previous theoretical topics.
Winkler-Schwartz et al. (18) have already described 17 students' and residents' training experience with a VR simulator. Their results suggest the possibility to categorize participants' technical abilities and use this tool to develop and maintain psychomotor skills. Licci et al. (2) developed a synthetic simulator based on patient-specific computed tomography (CT) data set, and different realistic skull models were produced by a 3D printer, including vascular structures and some soft tissue portions mimicking ventricle tumors. Neurosurgical trainees were invited to a neuroendoscopic workshop and qualitatively assessed afterwards. They found that this training empowered the development of specific surgical skills.
Joseph et al. (19) developed and used a physical simulator that was able to reproduce the experience of clipping intracranial aneurysms based on 3D-printed models of skull, brain, and arteries. They judged this simulator as a reliable and useful tool for neurosurgical training.
Chawla et al. recently reported a systematic review addressing 4 major neurosurgical skills using various modalities of training, assessed by face, content, and construct validity. An increased use of simulation models in neurosurgical training was found. Currently, synthetic models have been found to be the most convenient and practical, especially during the pandemic breakdown, but VR models are found promising due to the visual realism and improved haptic feedback technology (20).
The topic of burnout among neurosurgery residents is widely covered in scientific literature (21, 22). Training outside the operating room provides the possibility of practical education based on constructive criticism in a stress-free field. Surgical simulation allows the residents to perform a constant self-assessment of their growth from a technical and cognitive point of view. Personal growth and intellectual reward could be a real solution for the burnout issue. Furthermore, the repetitive use of a 3D model associated with AR would allow to standardize the surgical act in the different years of residency. More than 85% of residents agree or strongly agree (Table 1) to make this kind of simulation a part of the standard curriculum in neurosurgery. Our next target will be to create a surgical portfolio based on hybrid simulation to complete before joining the procedure in the operating room.
The 3D anatomic model allows to recreate even complex pathological conditions such as tumors and cerebral aneurysm. Indeed, senior residents were asked to perform an extra trial: clipping exercise on few 3D models enhanced with saccular aneurysm of middle cerebral artery (MCA), anterior communicating artery (ACoA) and posterior communicating artery (PCoA). This is certainly an important strength of the simulator that human or animal cadaveric specimens cannot provide. It is conceivable that it will be possible to perform the same procedure several times on the same patient in a simulated way before arriving at the day of the planned surgery.
On the other side, the texture and tactile feedback of the brain tissue and dura mater can still be improved. These characteristics were assessed with a mediocre scores in about 40% of the responses (3 out of 5 points). Furthermore, the arachnoid and the cisterns, which constitute some of the anatomical references for the surgery of the skull base, are not represented. These are the current limits of the tool.
The hybrid simulation with AR and 3D model represents a constant, modular, and repeatable training tool, while the surgeon will be the only variable. This new way of learning could change the old rules of knowledge transmission in neurosurgery centered on the mentor–apprentice relationship.
Limitations
The main limitation of this study is its purely qualitative nature and lack of quantitative analysis. However, as reported in the method section, this study was a preliminary experience with this new hybrid simulation, and the purpose of the study was primarily to assess the usability and liking of the tool. Therefore, despite its qualitative nature, the encouraging results that emerged from the survey could be considered a driving force for further quantitative studies aimed at analyzing the actual possibility of improving the learning curve of residents outside the operating room, using a reproducible and less expensive tool. To this end, the authors are developing a standardized 1-year surgical training program, tailored to the needs of different residency years, in which the residents' skills are assessed with quantitative scales in order to evaluate their growth curve.
Conclusion
The hybrid AR and 3D-printed neurosurgical simulator could be a valid tool for neurosurgical training, which is capable of enhancing the technical skills and competence of the residents. In addition, it could be easy to imagine how patient safety would increase and healthcare costs would be reduced, even if more studies are needed to investigate these aspects. The integration of simulators for training in neurosurgery as preparatory steps for the operating room should be recommended and further investigated given their huge potential.
Data Availability Statement
The raw data supporting the conclusions of this article will be made available by the authors, without undue reservation.
Ethics Statement
Written informed consent was obtained from the individual(s) for the publication of any potentially identifiable images or data included in this article.
Author Contributions
SP: writing draft. FC: editing and writing. FN: conceptualization and reviewing. GS: conceptualization and supervision. MM: conceptualization and data collection. GD: editing and data collection. AL: data collection. ML and DG: supervision and reviewing. All authors contributed to the article and approved the submitted version.
Conflict of Interest
FN is Founder and CEO of UpSurgeOn. GS is Co-founder of UpSurgeOn. MM is Lead Bioengineer at UpSurgeOn.
The remaining authors declare that the research was conducted in the absence of any commercial or financial relationships that could be construed as a potential conflict of interest.
The handling editor declared a past co-authorship with several of the authors FC and DG.
Publisher's Note
All claims expressed in this article are solely those of the authors and do not necessarily represent those of their affiliated organizations, or those of the publisher, the editors and the reviewers. Any product that may be evaluated in this article, or claim that may be made by its manufacturer, is not guaranteed or endorsed by the publisher.
References
1. Haji FA, Dubrowski A, Drake J, De Ribaupierre S. Needs assessment for simulation training in neuroendoscopy: a Canadian national survey; clinical article. J Neurosurg. (2013) 118:250–7. doi: 10.3171/2012.10.JNS12767
2. Licci M, Thieringer FM, Guzman R, Soleman J. Development and validation of a synthetic 3D-printed simulator for training in neuroendoscopic ventricular lesion removal. Neurosurg Focus. (2020) 48:E18. doi: 10.3171/2019.12.FOCUS19841
3. Stienen MN, Freyschlag CF, Schaller K, Meling T, EANS Young Neurosurgeons and EANS Training Committee. Procedures performed during neurosurgery residency in Europe. Acta Neurochir. (2020) 162:2303–11. doi: 10.1007/s00701-020-04513-4
4. Mukhopadhyay S, Punchak M, Rattani A, Hung YC, Dahm J, Faruque S, et al. The global neurosurgical workforce: a mixed-methods assessment of density and growth. J Neurosurg. (2019) 4:1–7. doi: 10.3171/2018.10.JNS171723
5. Lundy P, Miller C, Woodrow S. Current US neurosurgical resident involvement, interest, and barriers in global neurosurgery. Neurosurg Focus. (2020) 48:E16. doi: 10.3171/2019.12.FOCUS19808
6. Vincitorio F, Cofano F, Colzani G, Titolo P, Lavorato A, Battiston B, et al. Letter to the editor regarding “opinion piece: microsurgery in COVID-19–positive patients.” World Neurosurg. (2020) 139:712–3. doi: 10.1016/j.wneu.2020.05.161
7. Chau KH, Nouri SN, Madhavan MV. Fellowship in the time of coronavirus disease 2019 (COVID-19): a time to adapt. JAMA Cardiol. (2020) 5:749–0. doi: 10.1001/jamacardio.2020.1562
8. Tartara F, Cofano F, Zenga F, Boeris D, Garbossa D, Cenzato M. Are we forgetting non-COVID-19-related diseases during lockdown? Acta Neurochir. (2020) 162:1501. doi: 10.1007/s00701-020-04385-8
9. Bangor A, Kortum PT, Miller JT. An empirical evaluation of the system usability scale. Int J Hum Comput Interact. (2008) 24:574–94. doi: 10.1080/10447310802205776
10. Joshi A, Kale S, Chandel S, Pal D. Likert scale: explored and explained. Br J Appl Sci Technol. (2015) 7:396–403. doi: 10.9734/BJAST/2015/14975
11. Moiraghi A, Perin A, Sicky N, Godjevac J, Carone G, Ayadi R, et al. EANS basic brain course (ABC): combining simulation to cadaver lab for a new concept of neurosurgical training. Acta Neurochir. (2020) 162:453–60. doi: 10.1007/s00701-020-04216-w
12. Aboud E, Al-Mefty O, Yaşargil MG. New laboratory model for neurosurgical training that simulates live surgery. J Neurosurg. (2002) 97:1367–72. doi: 10.3171/jns.2002.97.6.1367
13. Lv X, Li C, Jiang W. The intracranial vasculature of canines represents a model for neurovascular ischemia and training residents and fellows in endovascular neurosurgery. Neuroradiol J. (2020) 33:292–6. doi: 10.1177/1971400920920787
14. Gnanakumar S, Kostusiak M, Budohoski KP, Barone D, Pizzuti V, Kirollos R, et al. Effectiveness of cadaveric simulation in neurosurgical training: a review of the literature. World Neurosurg. (2018) 118:88–96. doi: 10.1016/j.wneu.2018.07.015
15. Higgins M, Madan C, Patel R. Development and decay of procedural skills in surgery: a systematic review of the effectiveness of simulation-based medical education interventions. Surgeon. (2021) 19:e67–77. doi: 10.1016/j.surge.2020.07.013
16. Grosch AS, Schröder T, Schröder T, Onken J, Picht T. Development and initial evaluation of a novel simulation model for comprehensive brain tumor surgery training. Acta Neurochir. (2020) 162:1957–65. doi: 10.1007/s00701-020-04359-w
17. Cofano F, Di Perna G, Bozzaro M, Longo A, Marengo N, Zenga F, et al. Augmented reality in medical practice: from spine surgery to remote assistance. Front Surg. (2021) 8:657901. doi: 10.3389/fsurg.2021.657901
18. Winkler-Schwartz A, Bajunaid K, Mullah MAS, Marwa I, Alotaibi FE, Fares J, et al. Bimanual psychomotor performance in neurosurgical resident applicants assessed using neurotouch, a virtual reality simulator. J Surg Educ. (2016) 73:942–53. doi: 10.1016/j.jsurg.2016.04.013
19. Joseph F, Weber S, Raabe A, Bervini D. Neurosurgical simulator for training aneurysm microsurgery—a user suitability study involving neurosurgeons and residents. Acta Neurochir. (2020) 162:2313–21. doi: 10.1007/s00701-020-04522-3
20. Chawla S, Devi S, Calvachi P, Gormley WB, Rueda-Esteban R. Evaluation of simulation models in neurosurgical training according to face, content, and construct validity: a systematic review. Acta Neurochir. (2022) 164:1–20. doi: 10.1007/s00701-021-05003-x
21. Zaed I, Jaaiddane Y, Chibbaro S, Tinterri B. Burnout among neurosurgeons and residents in neurosurgery: a systematic review and meta-analysis of the literature. World Neurosurg. (2020) 143:e529–34. doi: 10.1016/j.wneu.2020.08.005
Keywords: simulator, training, virtual reality, life-like actuation, brain, neurosurgery, residents
Citation: Petrone S, Cofano F, Nicolosi F, Spena G, Moschino M, Di Perna G, Lavorato A, Lanotte MM and Garbossa D (2022) Virtual-Augmented Reality and Life-Like Neurosurgical Simulator for Training: First Evaluation of a Hands-On Experience for Residents. Front. Surg. 9:862948. doi: 10.3389/fsurg.2022.862948
Received: 26 January 2022; Accepted: 04 April 2022;
Published: 19 May 2022.
Edited by:
Daniele Bongetta, ASST Fatebenefratelli Sacco, ItalyReviewed by:
Sahin Hanalioglu, Hacettepe University, TurkeyRoberto Assietti, ASST Fatebenefratelli Sacco, Italy
Copyright © 2022 Petrone, Cofano, Nicolosi, Spena, Moschino, Di Perna, Lavorato, Lanotte and Garbossa. This is an open-access article distributed under the terms of the Creative Commons Attribution License (CC BY). The use, distribution or reproduction in other forums is permitted, provided the original author(s) and the copyright owner(s) are credited and that the original publication in this journal is cited, in accordance with accepted academic practice. No use, distribution or reproduction is permitted which does not comply with these terms.
*Correspondence: Giuseppe Di Perna, ZHIuZ2l1c2VwcGVkaXBlcm5hJiN4MDAwNDA7Z21haWwuY29t