- 1National Neuroscience Institute, Department of Neurosurgery, Singhealth, Singapore, Singapore
- 2Center for Qualitative Medicine, Duke-NUS Medical School, National University of Singapore, Singapore, Singapore
Background: Decompressive craniectomy (DC) improves the survival and functional outcomes in patients with malignant cerebral infarction. Currently, there are no objective intraoperative markers that indicates adequate decompression. We hypothesise that closure intracranial pressure (ICP) correlates with postoperative outcomes.
Methods: This is a multicentre retrospective review of all 75 DCs performed for malignant cerebral infarction. The patients were divided into inadequate ICP (iICP) and good ICP (gICP) groups based on a suitable ICP threshold determined with tiered receiver operating characteristic and association analysis. Multivariable logistic regression was performed for various postoperative outcomes.
Results: An ICP threshold of 7 mmHg was determined, with 36 patients (48.0%) and 39 patients (52.0%) in the iICP and gICP group, respectively. After adjustment, postoperative osmotherapy usage was more likely in the iICP group (OR 6.32, p = 0.003), and when given, was given for a longer median duration (iICP, 4 days; gICP, 1 day, p = 0.003). There was no difference in complications amongst both groups. When an ICP threshold of 11 mmHg was applied, there was significant difference in the duration on ventilator (ICP ≥11 mmHg, 3–9 days, ICP <11 mmHg, 3–5 days, p = 0.023).
Conclusion: Surgical decompression works complementarily with postoperative medical therapy to manage progressive cerebral edema in malignant cerebral infarctions. This is a retrospective study which showed that closure ICP, a novel objective intraoperative biomarker, is able to guide the adequacy of DC in this condition. Various surgical manoeuvres can be performed to ensure that this surgical aim is accomplished.
Introduction
Malignant cerebral infarction, occurring in up to 10% of all strokes (1), is defined as a large territory stroke associated with significant progressive cerebral edema, leading to raised intracranial pressure (ICP), cerebral herniation, and subsequently death. Treated medically alone (2–4), mortality is up to 80% (5–7). Multiple randomised controlled trials have shown clear evidence of significant reduction in mortality and some improvement in functional outcomes (8–14) when timely surgical decompression is performed.
The primary surgical goal of decompressive craniectomy (DC) is the control of ICP either prophylactically or therapeutically (2, 15–17). The 2014 scientific statement released by the American Heart Association and American Stroke Association (2) suggested that a bone flap of ≥12 cm is performed in DC. In an attempt to further optimise the procedure, numerous other studies have been conducted including the investigation of different bone flap sizes (18–24), retroauricular surgical incision (25), resection of the temporalis muscle (26), temporal lobectomy (20, 21, 27–29), and various forms of duroplasty (30, 31). These adjunctive manoeuvres, although effective, have significant disadvantages such as the risk of intraoperative or postoperative haemorrhage in lobectomies (20, 21, 27–29) and cosmetic and masticatory defects for temporalis resection (26). Hence, they should only be employed in situations where the standard DC is unable to sufficiently alleviate the raised ICP. In addition, none of these recommendations, account for the varying severity of the underlying pathology (32–35) and differences in an individual’s cranial anatomy.
Judgement of the adequacy of decompression is, however, highly subjective. Although objective methods that compared the size of DC and severity of brain shifts have been employed, this was performed using postoperative computed tomography (CT) imaging (36), which renders immediate intraoperative remedies impossible. Furthermore, raised ICP postoperatively in malignant cerebral infarctions has been shown to correlate with poorer outcomes, both in the short (37) and the long term (38), and has been used as a therapeutic target (39, 40).
We hypothesise that the ICP values on closure is firstly, correlated with postoperative outcomes; secondly, a variable that can be actively corrected; and hence, can be used as an objective intraoperative biomarker to guide surgeons in the determination of the adequacy of the surgical decompression.
Methods
Study Design
This multicentre retrospective review was performed in Tan Tock Seng Hospital and Singapore General Hospital, two of the major comprehensive stroke and neurosurgical centres in Singapore, from February 2016 to August 2020. All patients who underwent surgical decompression for cerebral infarctions were recruited. Institutional review board approval was obtained.
Inclusion and Exclusion Criteria
All adult patients from 18 to 80 years of age who underwent DC for large territory supratentorial cerebral infarction were included. These patients either underwent prophylactic or therapeutic decompression, after developing malignant cerebral edema and deteriorating neurologically. Patients fulfilling the selection criteria of the institutional protocol were considered for surgery. The inclusion criteria were: (1) large territory infarctions defined as an acute cerebral infarction involving more than 50% of the middle cerebral artery territory on neuroimaging, (2) occurring within 96 h from onset of stroke, and (3) a good premorbid functional status of modified Rankin scale (MRS) <3. The exclusion criteria were: (1) having a poor premorbid status of MRS ≥3, (2) poor preoperative neurological status of Glasgow coma scale (GCS) <6 or bilateral mydriasis, (3) severe haemorrhagic transformation involving >30% of infarction zone, and (4) medical comorbidities precluding surgical treatment such as life expectancy <3 years, uncorrected coagulopathy, and severe medical comorbidities. Patients with infratentorial infarctions and infarctions secondary to postoperative complications or trauma were excluded from the analysis.
Operative Steps and Perioperative Management
The DC was performed with a large reverse question mark incision with a convexity craniectomy of at least 12 cm diameter and subtemporal decompression. A temporal lobectomy may be performed at the surgeon’s discretion, if there was severe intraoperative brain swelling or if the surgical decompression was deemed insufficient. A strain gauge intraparenchymal ICP monitor (Codman® Microsensor® ICP transducer) was then inserted into the ipsilateral middle frontal gyrus and dural substitute was overlaid before closure in layers. The ICP was recorded at the end of closure.
Postoperatively the patients were managed in the neuroscience intensive care unit (NICU) using a tiered protocol with the aim of keeping ICP less than 20 (41, 42). The basic tier includes positional measures (head up and neck neutral), and prevention of physiological and metabolic derangements (fever, seizures, etc). Subsequent tiers include osmotherapy (10% mannitol and/or hypertonic saline), then paralysis, and then barbiturate coma. Once the patient was determined to have adequate postoperative ICP control and was over the period of malignant swelling, the ICP treatment was sequentially weaned off. The patient gets extubated or tracheostomised based on their neurological status and ability to maintain airway.
Data Collection
Patient characteristics, including age, sex, premorbid clinical status defined with ambulation and the ability to perform activities of daily living (ADL), were recorded. This is in addition to the modified frailty index (MFI-11) (43), an aggregate score that measures patient’s state of frailty based on medical history. Preoperative GCS and pupillary dilatation were noted. Radiological features of the infarction were recorded, including laterality of stroke, presence of haemorrhagic conversion, midline, and brainstem shift, uncal herniation, and of an internal carotid artery infarction. Internal carotid artery infarction was defined as a complete middle cerebral artery infarction with either anterior and/or posterior cerebral artery territory involvement. The surgical intent, prophylactic, or therapeutic, and additional intraoperative manoeuvres such as lobectomy and temporalis resection along with the ICP reading on closure were also detailed.
Inpatient outcomes measured included the length of stay in NICU, usage of osmotherapy (mannitol and/or hypertonic saline) and barbiturates for ICP control, and duration on ventilator. Complications including repeat surgery and syndrome of trephine (44) were noted also. Long term outcomes included mortality at 30 days and 6 months, and MRS at 3 and 6 months. MRS of 0–2 was defined as favourable in our study.
Statistical Analysis
Patients were grouped into inadequate ICP (iICP) or good ICP groups (gICP) based on an ICP threshold derived using tiered receiver operating characteristic (ROC) and association analysis. These processes were described in detail within the supplementary notes. Model performance indices including the beta coefficient, the area under the ROC curve (AUC), sensitivity and specificity, along with their 95% CI were reported.
Categorical variables were described using frequency (%); and continuous variables were reported as mean ± standard deviation or median (IQR). Baseline characteristics were compared using Chi square test (or Fisher exact test, where appropriate) and two-sample t-test (or Mann-Whitney U test, depending on the normality assumption) for categorical and continuous variables, respectively. Multivariable logistic regression was performed to investigate outcomes after adjusting for age, preoperative GCS, and surgical intent of DC. Un-adjusted and adjusted odds ratios (OR) and 95% confidence intervals (CI) were reported. Firth’s penalized likelihood approach was applied for rare events in logistic regression analysis. Data analysis was performed using SAS software version 9.4 for Windows (Cary, NC: SAS Institute Inc.) and statistical significance was set at p < 0.05.
Results
Patient Characteristics
A total of 81 DCs were performed during the study period. After application of exclusion criteria, 75 DCs were included in the final analysis. An ICP threshold of 7 mmHg (<7 vs ≥7 mmHg) was identified when examining ventilator days, and postoperative osmotherapy for ICP. There were 36 patients (48.0%) assigned into the iICP and 39 patients (52.0%) into the gICP group.
Both groups were comparable in terms of mean age (iICP, 54.6 ± 11.9 years; gICP, 58.3 ± 11.4 years), sex (iICP, 75.0% males; gICP, 61.5% males), premorbid ADL status (iICP, 100% independent; gICP, 100%), preoperative median GCS (iICP, 9; gICP, 10), and the presence of anisocoria (iICP, 36.1%; gICP, 33.3%). Radiological features, surgical intent and intraoperative performance of lobectomy were likewise similar. Of note, the median MRS was significantly better in the iICP group (p = 0.018). No patients in our cohort had temporalis resection performed during DC. These details were summarised in Table 1. Figure 1 demonstrates the number of patients included and remaining at each timepoint.
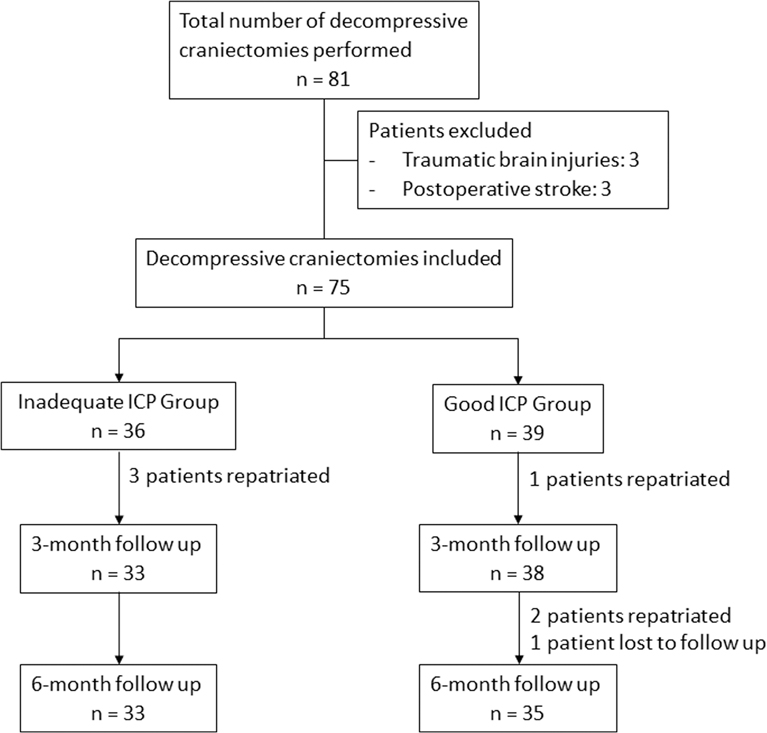
Figure 1. Flowchart of patients at various timepoints. Outcome evaluation of decompression at 3 and 6-month follow up.
Inpatient and Long-Term Outcomes
In terms of postoperative management, the iICP group were more likely to require osmotherapy (iICP, 83.3%; gICP, 51.4%, p = 0.003) and when given, required it for a longer median duration (iICP, 4 days, gICP, 1 day, p = 0.003). Otherwise, barbiturate use and duration, days on ventilator and length of stay in NICU were statistically similar amongst both groups.
Significantly, there were no differences in the rates of repeat surgery (iICP, 2.8%; gICP, 0%), syndrome of the trephine (iICP, 2.8%; gICP, 2.6%), median discharge GCS (iICP, 14; gICP, 13.5) and inpatient mortality (iICP, 22.2%; gICP 17.9%) amongst both groups.
When considering outcomes after discharge, the proportion of patients with 30-day mortality (iICP, 22.9%; gICP, 17.9%), 6-month mortality (iICP, 26.5%; gICP, 22.9%), 3-month favourable MRS (iICP, 6.1%; gICP, 0%) and 6-month favourable MRS (iICP, 15.2%; gICP 8.6%) were alike. The above details are described in Table 2.
For the duration of ventilator usage and length of stay in NICU, an ICP threshold of 11 mmHg differentiated our cohort. The group of patients with ICP of ≥11 mmHg had a significantly longer median duration on mechanical ventilation (ICP ≥11 mmHg, 4 [3–9] days; ICP <11 mmHg, 4 [3–5] days, p = 0.023) and a trend towards a longer stay in the NICU (ICP ≥11 mmHg, 6 [5–10] days; ICP <11 mmHg, 5 [4–8] days, p = 0.065). This information was documented in Table 3.
Discussion
DC has been shown to reduce mortality effectively in malignant cerebral infarctions (8–14). However, there is no known objective gauge that indicates if the surgical aim of sufficient ICP control were met. Our study demonstrated that the novel usage of the closure ICP was correlated with the extent of postoperative ICP medical management and hence can be used as an objective biomarker to indicate adequate decompression.
Measurement of Intraoperative and Postoperative ICP
The central hypothesis behind this study hinges upon the immediate and accurate measurement of ICP on closure. Due to this requirement, a strain gauge ICP monitor is used. Various studies have demonstrated that the Codman ICP monitor is consistent and precise even when compared against the ICP measured from an external ventricular drain (EVD) (45–48). In the authors’ opinion, intraoperative ICP measurements using other methods such as fibre-optic or a fluid-filled catheter, may not be able to achieve accurate measurements promptly and hence are not suitable for the intraoperative determination of decompression adequacy.
Postoperative ICP Control
ICP control is an interplay between surgical decompression and postoperative medical therapy. A closure ICP ≥7 mmHg was shown to be associated with difficult postoperative ICP control, and the closure ICP ≥11 mmHg was related to an increased duration of mechanical ventilation. Although osmotherapy usage was significantly different amongst both groups, barbiturate usage was likely not significant due to the small numbers that required it (9.3% of cohort).
Malignant cerebral infarction is known to have progressive swelling (49, 50). This delayed phenomenon occurs when the previously at-risk penumbral tissue progresses to infarction followed by delayed swelling, and in some cases haemorrhagic transformations (29). Hence, despite both thresholds of 7 and 11 mmHg are traditionally considered “low-normal”, if not attained, the likelihood of the progressive swelling leading to difficulties in controlling ICP at its peak, around day two to five after stroke (49, 50), is substantial. Furthermore, there is some evidence that the postoperative target for malignant cerebral infarctions should be lower than the 20 mmHg commonly used in traumatic brain injuries (37, 51, 52), further lending credence to the notion that a lower ICP target should be the goal. In addition, the similar rates of re-operation and syndrome of the trephine amongst both groups demonstrates that further decompression to “low-normal” ICP targets is safe and well tolerated by patients.
One unexpected outcome was the similar duration of mechanical ventilation and length of NICU stay despite a reduced need for medical treatment for ICP treatment in the gICP group. Mechanical ventilation, and hence NICU stay, is necessary when patients are placed on deep sedation on top of osmotherapy. One reason for this could be related to undocumented non-ICP reasons that require prolonged intubation such as pneumonia (53), delayed recovery in GCS, or a small sample size that is not able to detect a difference.
Significantly, despite having a lower closure ICP, iICP and gICP patients had similar inpatient and long-term outcome. This was likely due to the postoperative medical treatment making up for the varying degrees of adequacy of surgical decompression. This was corroborated by the similarly low rates of re-operation for iICP group.
Intraoperative Decision Making During Surgical Decompression
After the completion of a standard DC, the closure ICP should be estimated by bringing the skin flap to the opposite skin edge. Should the ICP value at this point be ≥7 mmHg, the authors recommend the sequential performance of the following manoeuvres: DC extension, temporal lobectomy, temporalis resection, and EVD insertion. The order of performance was suggested based on the surgical difficulty and risk involved. The first option, DC extension, is safe and easily executed in the author’s experience. After dissecting the dura off the bone edge under direct vision, a 1–2 cm rim of bone is then removed. This mitigates the risk of breaching the dural venous sinus. At this point, the closure ICP should be reassessed and should it remain inadequate, the performance of further manoeuvres should be considered. Temporal lobectomy and EVD insertion have an elevated risk of haemorrhage, especially since there will often be antithrombotics given at some stage after presentation of the stroke, while temporalis resection, although effective, leads to cosmetic deficiencies and masticatory dysfunction (26).
Surgical Decompression as Part of Overall Stroke Management
From prehospital management by emergency medical services (54), establishment of stroke centres (55) and mobile stroke units (56), to advances in stroke imaging (57, 58) and extension of the thrombolysis (59) and thrombectomy window (60, 61), stroke management has advanced significantly in the past decades. In light of these developments, surgical decompression, retains its role as the last resort in “end stage” cerebral infarction. When performed well, with clear and objective intraoperative surgical targets to achieve, DC has the potential to influence ICP control postoperatively and potentially reduce the need for ventilation and NICU. This may contribute to further improvements in overall stroke outcomes along with significant cost reductions during intensive care. Economic analysis of the effect of an adequate surgical decompression were beyond the scope of our study and hence was not further explored. We recommend that further large-scale prospective studies and economic analysis can be conducted to further validate this claim.
Limitations
This study suffers from the inherent limitations of a retrospective review. It was, however, mitigated with the use of multivariable logistic regression analysis. Another limitation of our study was the number of patients lost to follow up (7 patients [9.3%]). This effect was minimised as both groups had a similar proportion of such patients.
Conclusion
We established that patients with a closure ICP of <7 mmHg had a lesser need for prolonged postoperative medical management and closure ICP <11 mmHg was associated with a reduced duration on mechanical ventilation and reduced length of NICU stay. This is a retrospective study which showed that closure ICP, a novel objective intraoperative biomarker, is able to guide the adequacy of DC in malignant cerebral infarctions. Various surgical manoeuvres can be performed to ensure that this surgical aim is accomplished.
Data Availability Statement
The raw data is available at reasonable request to the corresponding author.
Ethics Statement
The studies involving human participants were reviewed and approved by Singhealth CIRB 2015/2143. Written informed consent for participation was not required for this study in accordance with the national legislation and the institutional requirements.
Author Contributions
JXL: Conceptualization, methodology, validation, formal analysis, investigation, curation, writing - original draft, writing - review & editing. SJL: methodology, software, validation, formal analysis, writing - review & editing. TMC: methodology, software, validation, writing - review & editing. SES: validation, formal analysis, writing - review & editing. JXH: methodology, resources, writing - review & editing. MWC: conceptualization, validation, project administration, writing - review & editing.
Supplementary Material
The Supplementary Material for this article can be found online at: https://www.frontiersin.org/articles/10.3389/fsurg.2022.823899/full#supplementary-material.
Conflict of Interest
The authors declare that the research was conducted in the absence of any commercial or financial relationships that could be construed as a potential conflict of interest.
Publisher's Note
All claims expressed in this article are solely those of the authors and do not necessarily represent those of their affiliated organizations, or those of the publisher, the editors and the reviewers. Any product that may be evaluated in this article, or claim that may be made by its manufacturer, is not guaranteed or endorsed by the publisher.
References
1. Minnerup J, Wersching H, Ringelstein EB, Heindel W, Niederstadt T, Schilling M, et al. Prediction of malignant middle cerebral artery infarction using computed tomography-based intracranial volume reserve measurements. Stroke. (2011) 42:3403–9. doi: 10.1161/STROKEAHA.111.619734
2. Wijdicks EFM, Sheth KN, Carter BS, Greer DM, Kasner SE, Kimberly WT, et al. Recommendations for the management of cerebral and cerebellar infarction with swelling: a statement for healthcare professionals from the American Heart Association/American Stroke Association. Stroke. (2014) 45:1222–38. doi: 10.1161/01.str.0000441965.15164.d6
3. Tan TK, Cheng MH, Sim EY. Options for managing raised intracranial pressure. Proc Sing Healthcare. (2015) 24:156–64. doi: 10.1177/2010105815598444
4. Rangel-Castilla L, Gopinath S, Robertson CS. Management of intracranial hypertension. Neurol Clin. (2008) 26:521–41. doi: 10.1016/j.ncl.2008.02.003
5. Doerfler A, Forsting M, Reith W, Staff C, Heiland S, Schabitz WR, et al. Decompressive craniectomy in a rat model of “malignant”cerebral hemispheric stroke: experimental support for an aggressive therapeutic approach. J Neurosurg. (1996) 85:853–9. doi: 10.3171/jns.1996.85.5.0853
6. Kalia KK, Yonas H. An aggressive approach to massive middle cerebral artery infarction. Arch Neurol. (1993) 50:1293–7. doi: 10.1001/archneur.1993.00540120010005
7. Schwab S, Steiner T, Aschoff A, Schwarz S, Steiner HH, Jansen O, et al. Early hemicraniectomy in patients with complete middle cerebral artery infarction. Stroke. (1998) 29:1888–93. doi: 10.1161/01.STR.29.9.1888
8. Hofmeijer J, Kappelle LJ, Algra A, Amelink GJ, van Gijn J, van der Worp HB, et al. HAMLET investigators. Surgical decompression for space-occupying cerebral infarction (the Hemicraniectomy After Middle Cerebral Artery infarction with Life-threatening Edema Trial [HAMLET]): a multicentre, open, randomised trial. Lancet Neurol. (2009) 8:326–33. doi: 10.1016/S1474-4422(09)70047-X
9. Juttler E, Schwab S, Schmiedek P, Unterberg A, Hennerici M, Woitzik K, et al. DESTINY Study Group.Decompressive surgery for the treatment of malignant infarction of the middle cerebral artery (DESTINY): a randomised, controlled trial. Stroke. (2007) 38:2518–25. doi: 10.1161/STROKEAHA.107.485649
10. Vahedi K, Vicaut E, Mateo J, Kurtz A, Orabi M, Guichard JP, et al. Sequential-design, multicenter, randomized, controlled trial of early decompressive craniectomy in malignant middle cerebral artery infarction (DECIMAL trial). Stroke. (2007) 38:2506–17. doi: 10.1161/STROKEAHA.107.485235
11. Vahedi K, Hofmeijer J, Juettler E, Vicaut E, Geroge B, Algra A, et al. Early decompressive surgery in malignant infarction of the middle cerebral artery: a pooled analysis of three randomised controlled trials. Lancet Neurol. (2007) 6:215–22. doi: 10.1016/S1474-4422(07)70036-4
12. Zhao J, Su YY, Zhang Y, Zhang YZ, Zhao R, Wang L, et al. Decompressive hemicraniectomy in malignant middle cerebral artery infarct: a randomized controlled trial enrolling patients up to 80 years old. Neurocrit Care. (2012) 17:161–71. doi: 10.1007/s12028-012-9703-3
13. Juttler E, Unterberg A, Woitzik J, Bosel J, Amiri H, Sakowitz OW, et al. Hemicraniectomy in older patients with extensive middle-cerebral-artery stroke. N Engl J Med. (2014) 370:1091–100. doi: 10.1056/NEJMoa1311367
14. Frank JI, Schumm LP, Wroblewski K, Chyatte D, Rosengart AJ, Kordeck D, et al. HeADDFIRST Trialists. Hemicraniectomy and durotomy upon deterioration from infarction-related swelling trial: randomized pilot clinical trial. Stroke. (2014) 45:781–7. doi: 10.1161/STROKEAHA.113.003200
15. Pallesen LP, Berlin K, Puetz V. Role of decompressive craniectomy in ischemic stroke. Front Neurol. (2019) 9:1119. doi: 10.3389/fneur.2018.01119
16. Beez T, Munoz-Bendix C, Steiger HJ, Beseoglu K. Decompressive craniectomy for acute ischemic stroke. Crit Care. (2019) 23:209. doi: 10.1186/s13054-019-2490-x
17. Zweckberger K, Juettler E, Bosel J, Unterberg WA. Surgical aspects of decompression craniectomy in malignant stroke: review. Cerebrovasc Dis. (2014) 38:313–23. doi: 10.1159/000365864
18. Wagner S, Schnippering H, Aschoff A, Koziol JA, Schwab S, Steiner T. Suboptimum hemicraniectomy as a cause of additional cerebral lesions in patients with malignant infarction of the middle cerebral artery. J Neurosurg. (2001) 94:693–6. doi: 10.3171/jns.2001.94.5.0693
19. Wang KW, Chang WN, Ho JT, Chang HW, Lui CC, Cheng MH, et al. Factors predictive of fatality in massive middle cerebral artery territory infarction and clinical experience of decompressive hemicraniectomy. Eur J Neurol. (2006) 13:765–71. doi: 10.1111/j.1468-1331.2006.01365.x
20. Walz B, Zimmerman C, Bottger S, Haberl RL. Prognosis of patients after hemicraniectomy in malignant middle cerebral artery infarction. J Neurol. (2002) 249:1183–90. doi: 10.1007/s00415-002-0798-x
21. Curry WT Jr, Sethi MK, Ogilvy CS, Carter BS. Factors associated with outcome after hemicraniectomy for large middle cerebral artery territory infarction. Neurosurg. (2005) 56:681–92. doi: 10.1227/01.NEU.0000156604.41886.62
22. Chung J, Bang OY, Lim YC, Park SK, Shin YS. Newly suggested surgical method of decompressive craniectomy for patients with middle cerebral artery infarction. Neurologist. (2011) 17:11–5. doi: 10.1097/NRL.0b013e3181f4ec88
23. Neugebauer H, Fiss I, Pinczolits A, Hecht N, Witsch J, Dengler NF, et al. Large size hemicraniectomy reduces early herniation in malignant middle cerebral artery infarction. Cerebrovasc Dis. (2016) 41:283–90. doi: 10.1159/000443935
24. Tanrikulu L, Oez-Tanrikulu A, Weiss C, Scholz T, Schiefer J, Clusmann H, et al. The bigger, the better? About the size of decompressive hemicraniectomies. Clin Neurol Neurosurg. (2015) 135:15–21. doi: 10.1016/j.clineuro.2015.04.019
25. Dowlati E, Mortazavi A, Keating G, Jha RT, Felbaum DR, Chang JJ, et al. The retroauricular incision as an effective and safe alternative incision for decompressive hemicraniectomy. Open Neurosurg (Hagerstown). (2021) 20:549–58. doi: 10.1093/ons/opab021.
26. Park J, Kim E, Kim GY, Hur YK, Guthikonda M. External decompressive craniectomy including resection of temporal muscle and fascia in malignant hemispheric infarction. J Neurosurg. (2009) 110:101–5. doi: 10.3171/2008.4.17540
27. Greenwood J Jr. Acute brain infarctions with high intracranial pressure: surgical indications. Johns Hopkins Med J. (1968) 122:254–60.
28. Ivamoto HS, Numoto M, Donaghy RM. Surgical decompression for cerebral and cerebellar infarcts. Stroke. (1974) 5:365–70. doi: 10.1161/01.STR.5.3.365
29. Robertson SC, Lennarson P, Hasan DM, Traynelis VC. Clinical course and surgical management of massive cerebral infarction. Neurosurg. (2004) 55:55–61. doi: 10.1227/01.NEU.0000126875.02630.36
30. Lee CH, Cho DS, Jin SC, Kim SH, Park DB. Usefulness of silicone elastomer sheet as another option of adhesion preventive material during craniectomies. Clin Neurol Neurosurg. (2007) 109:667–71. doi: 10.1016/j.clineuro.2007.05.015
31. Butlers D, Belli A. Placement of silicone sheeting at decompressive craniectomy to prevent adhesions at cranioplasty. Br J Neurosurg. (2010) 24:75–6. doi: 10.3109/02688690903506135
32. Roper AH, Shafran B. Brain edema after stroke. Clinical syndrome and intracranial pressure. Arch Neurol. (1984) 41:26–9. doi: 10.1001/archneur.1984.04050130032017
33. Schwab S, Aschoff A, Spranger M, Albert F, Hacke W. The value of intracranial pressure monitoring in acute hemispheric stroke. Neurol. (1996) 47:393–8. doi: 10.1212/WNL.47.2.393
34. Hacke W, Schwab S, Horn M, et al. ‘Malignant’ idle cerebral artery territory infarction:clinical course and prognostic signs. Arch Neurol. (1996) 53:309–15. doi: 10.1001/archneur.1996.00550040037012
35. Wijdicks EF, Diringer MN. Middle cerebral artery territory infarction and early brain swelling: progression and effect of age on outcome. Mayo Clin Proc. (1998) 73:829–36. doi: 10.4065/73.9.829
36. Bruno A, Zahran A, Paletta N, Maali L, Nichols FT, Figueroa R. A standardised method to measure brain shifts with decompressive hemicraniectomy. J Neurosci Methods. (2017) 280:11–5. doi: 10.1016/j.jneumeth.2017.01.021
37. Sauvigny T, Gottsche J, Czorlich P, Vettorazzi E, Westphal M, Regelsberger J. Intracranial pressure in patients undergoing decompressive craniectomy: new perspective on thresholds. J Neurosurg. (2018) 128:819–27. doi: 10.3171/2016.11.JNS162263
38. Jeon SB, Park JC, Kwon SU, Kim YJ, Lee S, Kang DW, et al. Intracranial pressure soon after hemicraniectomy in malignant cerebral artery infarction. J Intensive Care Med. (2018) 33:310–6. doi: 10.1177/0885066616675598
39. Paldor I, Rosenthal G, Cohen JE, Leker R, Harnof S, Shoshan Y, et al. Intracranial pressure monitoring following decompressive hemicraniectomy for malignant cerebral infarction. J Clin Neurosci. (2015) 22:79–82. doi: 10.1016/j.jocn.2014.07.006
40. Funchal BF, Alves MM, Suriano IC, Chaddad-Neto FE, Ferraz MEMR, Silva GS. Intracranial pressure following decompressive hemicraniectomy for malignant cerebral infarction: clinical and treatment correlations. Arq Neuropsiquiatr. (2018) 76:812–5. doi: 10.1590/0004-282x20180132
41. Tan TK, Cheng MH, Sim EY. Options for managing raised intracranial pressure. Proc Sing Healthcare. (2015) 24:156–64. doi: 10.1177/2010105815598444
42. Tripathy S, Ahmad SR. Raised intracranial pressure syndrome: a stepwise approach. Indian J Crit Care Med. (2019) 23:S129–35. doi: 10.5005/jp-journals-10071-23190
43. Velanovich V, Antione H, Swartz A, Peters D, Rubinfeld I. Accumulating deficits model of frailty and postoperative mortality and morbidity: its application to a national database. J Surg Res. (2013) 183:104–10. doi: 10.1016/j.jss.2013.01.021
44. Ashayeri K, Jackson EM, Huang J, Brem H, Gordon CR. Syndrome of the trephined: a systematic review. Neurosurgery. (2016) 79:525–34. doi: 10.1227/NEU.0000000000001366
45. Koskinen LP, Olivecrona M. Clinical experience with the intraparenchymal intracranial pressure monitoring CODMAN MICROSENSOR system. Neurosurgery. (2005) 56:693–8. doi: 10.1227/01.NEU.0000156609.95596.24
46. Gopinath SP, Robertson CS, Contant CF, Narayan RK, Grossman RG. Clinical evaluation of a miniature strain-gauge transducer for monitoring intracranial pressure. Neurosurgery. (1995) 36:1137–40. doi: 10.1227/00006123-199506000-00011
47. Exo J, Kochanek PM, Adelson PD, Greene S, Clark RSB, Bayir H, et al. Intracranial pressure-monitoring systems in children with traumatic brain injury: combining therapeutic and diagnostic tools. Pediatr Crit Care Med. (2011) 12:560–55. doi: 10.1097/PCC.0b013e3181e8b3ee
48. Vender J, Waller J, Dhandapani K, McDonnell D. An evaluation and comparison of intraventricular, intraparenchymal, and fluid-coupled techniques for intracranial pressure monitoring in patients with severe traumatic brain injury. J Clin Monit Comput. (2011) 25:231–6. doi: 10.1007/s10877-011-9300-6
49. Hacke W, Schwab S, Horn M. ‘Malignant’ middle cerebral artery territory infarction. Clinical course and prognostic signs. Arch Neurol. (1996) 53:309–15. doi: 10.1001/archneur.1996.00550040037012
50. Hofmeijer J, Algra A, Kappelle LJ, van der Worp HB. Predictors of life-threatening brain edema in middle cerebral artery infarction. Cerebrovasc Dis. (2008) 26:176–84. doi: 10.1159/000113736
51. Poca MA, Benejam B, Sahuquillo J, Riveiro M, Frascheri L, Merino MA, et al. Monitoring intracranial pressure in patients with malignant middle cerebral artery infarction: is it useful? J Neurosurg. (2010) 112:648–57. doi: 10.3171/2009.7.JNS081677
52. Hernandez-Duran S, Meinen L, Rohde V, von der Brelie C. Invasive monitoring of intracranial pressure after decompressive craniectomy in malignant stroke. Stroke. (2021) 52:707–11. doi: 10.1161/STROKEAHA.120.032390
53. Li J, Zhang P, Wu S, Wang Y, Zhou J, Yi X, et al. Stroke-related complications in large hemisphere infarction: incidence and influence on unfavourable outcome. Ther Adv Neurol Disord. (2019) 12:1756286419873264. doi: 10.1177/1756286419873264
54. Powers WJ, Rabinstein AA, Ackerson T, Adeoye OM, Bambakidis NC, Becker K, et al. Guidelines for the early management of patients with acute ischaemic stroke: 2019 update to the 2018 guidelines for the early management of acute ischemic stroke: a guideline for healthcare professionals from the American heart association/American stroke association. Stroke. (2019) 50:e344–e418. doi: 10.1161/STROKEAHA.118.022606
55. Pierot L, Jayaramanm MV, Szikora I, Hirsh JA, Baxter B, Miyachi S, et al. Standards of practice in acute ischemic stroke intervention: international recommendations. J Neurointerv Surg. (2018) 10:1121–6. doi: 10.1136/neurintsurg-2018-014287
56. Calderon VJ, Kasturiarachi BM, Lin E, Bansal V, Zaidat OO. Review of the mobile stroke unit experience worldwide. Interv Neurol. (2018) 7:347–58. doi: 10.1159/000487334
57. Vagal A, Wintermark M, Nael K, Bivard A, Parsons M, Grossman AW, et al. Automated CT perfusion imaging for acute ischemic stroke: pearls and pitfalls for real-world use. Neurology. (2019) 893:888–98. doi: 10.1212/WNL.0000000000008481
58. Demeestere J, Wouters A, Christensen S, Lemmens R, Lansberg MG. Review of perfusion imaging in acute ischemic stroke: from time to tissue. Stroke. (2020) 51:1017–24. doi: 10.1161/STROKEAHA.119.028337
59. Campbell BCV, Ma H, Ringleb PA, Parsons MW, Churilov L, Bendszus M, et al. EXTEND, ECASS-4, and EPITHET Investigators. Extending thrombolysis to 4.5 – 6 h and wake-up stroke using perfusion imaging: a systemic review and meta-analysis of individual patient data. Lancet. (2019) 394:139–47. doi: 10.1016/S0140-6736(19)31053-0
60. Nogueira RG, Jadhav AP, Haussen DC, Bonafe A, Budzik RF, Bhuva R, et al. DAWN Trial Investigators. Thrombectomy 6 to 24 hours after stroke with a mismatch between deficit and infarct. N Eng J Med. (2018) 378:11–21. doi: 10.1056/NEJMoa1706442
Keywords: large territory infarctions, decompressive craniectomy, intracranial pressure, modified Rankin scale, outcomes, middle cerebral artery infarction, malignant infarction, hyperosmolar therapy
Citation: Lim JX, Liu SJ, Cheong TM, Saffari SE, Han JX and Chen MW (2022) Intracranial Pressure as an Objective Biomarker of Decompression Adequacy in Large Territory Infarction: A Multicenter Observational Study. Front. Surg. 9:823899. doi: 10.3389/fsurg.2022.823899
Received: 28 November 2021; Accepted: 6 April 2022;
Published: 6 May 2022.
Edited by:
Ciaran Scott Hill, National Hospital for Neurology and Neurosurgery (NHNN), United KingdomReviewed by:
Daniel Holsgrove, Manchester Centre of Clinical Neurosciences, Salford Royal NHS Foundation Trust, Salford, United KingdomOliver Mueller, Neurochirurgie KlinikumDO, Germany
Luis Rafael Moscote-Salazar, Latinamerican Council of Neurocritical Care (CLaNi), Colombia
Copyright © 2022 Lim, Liu, Cheong, Saffari, Han and Chen. This is an open-access article distributed under the terms of the Creative Commons Attribution License (CC BY). The use, distribution or reproduction in other forums is permitted, provided the original author(s) and the copyright owner(s) are credited and that the original publication in this journal is cited, in accordance with accepted academic practice. No use, distribution or reproduction is permitted which does not comply with these terms.
*Correspondence: Jia Xu Lim amlheHUubGltQG1vaGguY29tLnNn
Speciality section: This article was submitted to Neurosurgery, a section of the journal Frontiers in Surgery