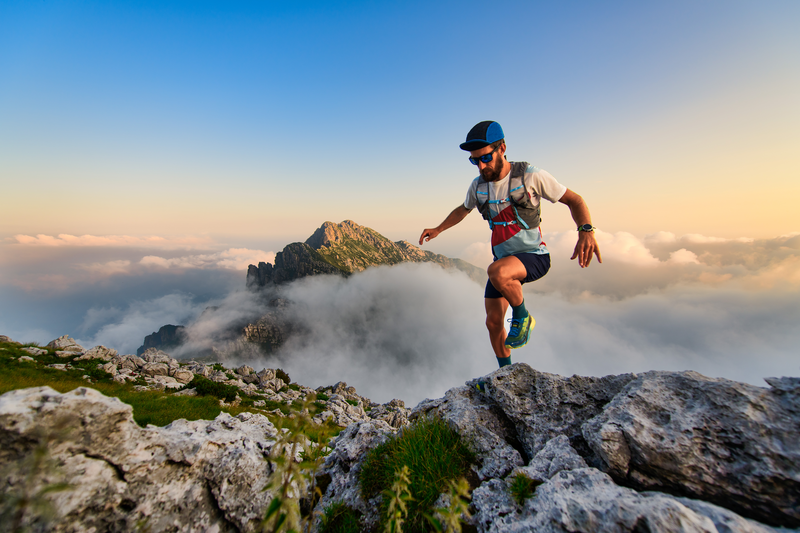
95% of researchers rate our articles as excellent or good
Learn more about the work of our research integrity team to safeguard the quality of each article we publish.
Find out more
ORIGINAL RESEARCH article
Front. Surg. , 12 October 2022
Sec. Visceral Surgery
Volume 9 - 2022 | https://doi.org/10.3389/fsurg.2022.1026586
This article is part of the Research Topic Acute Mesenteric Ischemia: Novel Diagnostic Modalities and Treatment Strategies to Improve Patient Outcome View all 5 articles
Background: Early allograft dysfunction (EAD) is a common postliver transplant complication that has been associated with graft failure and risk for poor prognosis. There are many risk factors for the incidence of EAD after liver transplantation (LT). This study investigated whether elevated postoperative myoglobin (Mb) increases the incidence of EAD in liver transplanted recipients.
Methods: A total of 150 adult recipients who measured Mb within 3 days after liver transplantation between June 2019 and June 2021 were evaluated. Then, all patients were divided into two groups: the EAD group and the non-EAD group. Univariate and multivariate logistic regression analyses were performed, and receiver operating characteristic curves (ROCs) were constructed.
Results: The incidence of EAD was 53 out of 150 patients (35.3%) in our study. Based on the multivariate logistic analysis, the risk of EAD increased with elevated postoperative Mb (OR = 1.001, 95% CI 1.000–1.001, P = 0.002). The Mb AUC was 0.657, and it was 0.695 when combined with PCT. When the subgroup analysis was conducted, the AUC of serum Mb prediction was better in patients whose preoperative model for end-stage liver disease score ≤ 15 or operative time ≥ 10 h (AUC = 0.751, 0.758, respectively, or 0.760, 0.800 when combined with PCT).
Conclusion: Elevated Mb significantly increased the risk of postoperative EAD, suggesting that postoperative Mb may be a novel predictor of EAD after liver transplantation.
The study was registered in the Chinese Clinical Trial Registry (Registration number: ChiCTR2100044257, URL: http://www.chictr.org.cn).
The most effective current treatment for end-stage liver disease (ESLD) is liver transplantation (LT) (1). However, due to the shortage of liver allografts, more extended standard allografts have been used in the clinic (2). There are still many complications associated with LT, such as early allograft dysfunction (EAD), which represents higher mortality and graft failure (3). A few patients with EAD could gradually develop into primary graft nonfunction, which is the most serious complication after orthotropic liver transplantation and often requires surgery again, with high clinical mortality (4, 5). There are many mechanisms of EAD, among which ischemia-reperfusion injury (IRI) remains the most likely (6). There are also many risk factors for the incidence of EAD, such as donor body mass index (BMI), uric acid, postoperative hepatic artery resistance index, and the C-reactive protein-to-albumin ratio, which have been reported in previous studies (5, 7–9).
Myoglobin (Mb) has been widely studied in previous studies. It is a small pigment protein produced by combining globin and heme-iron (10). Mb, as an O2 storage depot, is present in smooth muscle cells and cardiac myocytes (11). Due to the prolonged immobilization or certain surgical positions such as dorsal lithotomy and knee-chest position, another possibility of IRI, large amounts of myoglobin are released into the blood, which may result in organ failure (12). Myoglobin is a biomarker for myocardial infarction known to us (13). Hypermyoglobin may also result in acute kidney injury (AKI), as described in our previous study (14). In addition, among patients with rhabdomyolysis, the level of myoglobin was a strong predictor of AKI (15). Additionally, some studies found that postoperative serum myoglobin after cardiac surgery was associated with poor outcomes (16). In our center, we interestingly found that the level of serum myoglobin was significantly elevated in patients with EAD after LT. However, no previous studies have shown the correlation between myoglobin and the occurrence of EAD after LT.
Our study aims to provide the first description of the level of serum myoglobin on postoperative day 0 (POD0) in patients after LT. We further describe the tendency of serum Mb within 3 days after LT and the difference in Mb between patients with EAD and those without EAD.
All patients who underwent orthotropic liver transplantation (OLT) were prospectively collected from June 2019 to June 2021 in the surgical intensive care unit (ICU) of Beijing Chao-yang Hospital. The informed consent had been obtained. The research program was approved by the Institutional Review Board (Beijing Chao-yang Hospital Affiliated to Capital Medical University, approval number: 2021-55) and was performed in compliance with the declaration of Helsinki. Also, neither piggyback nor venovenous bypass was used in all patients. The grafts were all from donation after brain death (DBD). The included criteria are as follows: (1) age ≥18 years and (2) admission to ICU after LT. Patients were excluded when they met any of the following criteria: (1) secondary liver transplantation; (2) age less than 18 years old; and (3) refused the informed consent. According to the incidence of EAD after LT which was reported in previous study 46.4%, a sample of 159 patients was required at a significance level of a = 0.05, with a power of 80% and allowing for 10% missing data. Finally, a total of 150 patients were included for analysis in our study, as shown in Figure 1.
Figure 1. Study flow chart. Three patients with secondary transplantation were excluded. A total of 150 patients were included for analysis. ICU, intensive care unit; LT, liver transplantation; POD, postoperative day; Mb, myoglobin.
Demographic data including age, sex, BMI, the model for end-stage liver disease (MELD) score, Child–Pugh classification, and comorbidities (history of Ascites, encephalopathy, diabetes mellitus, etc.) were recorded as baseline data. Preoperative data such as white blood cell count (WBC), hemoglobin, platelet count, albumin, aspartate aminotransferase (AST) and alanine aminotransferase (ALT), total bilirubin (TB), direct bilirubin (DB), ammonia, international normalized ratio (INR), prothrombin time (PT), prothrombin activity (PA), and serum creatinine were collected from the latest data before surgery. These data were measured again upon admission to ICU immediately. In addition, laboratory data including AST, ALT, TB, and INR were measured within postoperative 7 days. Also, for all patients, serum myoglobin was measured at the time of admission to ICU and on POD1, POD2, and POD3. However, due to the purpose of predictive utility for EAD, we used the level of Mb on POD0 to explore the value of Mb to predict EAD. Furthermore, we obtained the Sequential Organ Failure Assessment (SOFA) score, the Acute Physiology Chronic Health Disease Classification System II (APACH II) score, and the use of vasopressor on POD0. We also evaluated whether sepsis was diagnosed upon admission to ICU. Intraoperative data including duration of surgery, blood loss, fluid balance, duration of inferior vena cava interruption, intraoperative urine output, cold ischemia time, and warm ischemia time were also obtained from the electronic medical record system.
The primary outcome of this study was the incidence of EAD within 7 days after surgery. Early allograft dysfunction was defined according to Olthoff et al., which met one or more of the following items: (1) serum levels of ALT or AST > 2000 U/L within the first week; (2) TB ≥ 10 mg/dl on postoperative day 7; and (3) INR ≥ 1.6 on postoperative day 7 (17). Patients who met one or more of the above criteria were included in the EAD group, and others were included in the non-EAD group. Secondary outcomes included AKI, the length of ICU and hospital stay, and 28-day overall mortality. AKI was defined as per the following criteria, according to Kidney Disease: Improving Global Outcome (KDIGO) criteria: an increase in serum creatinine by ≥26.5 μmol/L (≥3 mg/dl) within 48 h or an increase in serum creatinine to ≥1.5 times baseline, which is known or presumed to have occurred within the first 7 postoperative days (Supplementary Table S1) (18).
All data were analyzed by SPSS statistical software version 26.0 (IBM, Chicago, IL, USA) and GraphPad Prism 8.3.0 (Graphpad Software Inc., San Diego, CA, USA). Our data were presented as the mean ± standard deviation (SD) and the median (interquartile range, IQR) for continuous variables or frequency (percentages) for categorical variables. The baseline characteristics, perioperative parameters, and outcomes were compared using the Student t-test, Mann–Whitney U test, chi-square test, or Fisher exact test as appropriate. In addition, univariate and multivariate logistic regression analyses were also performed to examine the effect of the level of Mb on POD0 on primary and secondary outcomes. We used multiple imputations to account for missing data. The receiver operating characteristic (ROC) curve analysis was performed to evaluate the predictive value of serum Mb on POD0 for EAD. Associations were expressed as odds ratio (OR) and 95% confidence interval (95% CI). Two-sided P values less than 0.05 were considered statistically significant.
Excluding 3 patients who underwent the second LT, a total of 150 patients were initially recruited for the current study, including 127 (84.7%) men and 23 (15.3%) women (Figure 1). The mean ± SD of age and BMI were 52.6 ± 9.4 years and 23.7 ± 3.6 kg/m2. There were 53 (35.3%) patients occurring with EAD after LT. Overall, the etiology of LT is as follows: 44.0% liver tumor, 25.3% viral diseases, 10.7% alcohol-related, 7.3% biliary cirrhosis, and 12.7% other liver diseases. All patients received liver grafts from donation after brain death (DBD). There was no statistical difference in demographics between the two groups (P > 0.05), as shown in Table 1.
The comparison of baseline characteristics between the two groups is listed in Table 2. Patients in the EAD group had higher WBC counts (3.72 [2.79, 6.60] vs. 3.34 [2.18, 5.00], P = 0.021) than those in the non-EAD group. In terms of intraoperative variables, we could find that patients with EAD experienced a longer duration of surgery, even though there was no difference between the two groups (P = 0.256). Significantly, the mean intraoperative urine output in the EAD group patients was lower than that in the non-EAD group patients (168.8 [103.8, 225.3] vs. 187.3 [124.9, 268.9], P = 0.050). Postoperative data including SOFA score, APACH II score, lactate, WBC, PLT, PA, Mb, and PCT were obtained upon admission to ICU immediately. There was also a statistical significance in the level of postoperative lactate (2.1 [1.5, 4.1] vs. 1.6 [1.1, 2.6], P = 0.011), APACH II score (15.9 ± 8.0 vs. 14.2 ± 6.0, P = 0.023), PA (33.1 [23.6, 42.1] vs. 39.9 [31.4, 47.6], P = 0.002), and PCT (30.9 [8.5, 82.5] vs. 14.2 [4.7, 28.6], P = 0.007) between the two groups. Patients with EAD were more likely to have a prolonged ICU stay (5.6 [3.3, 10.4] vs. 4.2 [2.7, 5.7], P = 0.003). Moreover, of the 150 patients, 11 (7.4%) patients died, 7 (13.2%) in the EAD group and 4 (4.1%) in the non-EAD group (P = 0.041). No significant difference was observed in other variables between the two groups.
In univariate logistic analysis, preoperative WBC, duration of IVC interruption, cold ischemic time, serum Mb on POD0, serum PCT, and PA may be related to the increased risk of EAD (P < 0.1). Based on multivariate logistic analysis, serum Mb on POD 0 [1.001 (1.000, 1.001), P = 0.02] and PCT on POD0 [1.008 (1.001, 1.015) P = 0.022] were independent risk factors for the incidence of EAD (Table 3).
Furthermore, we constructed different models to predict EAD based on independent risk factors. I predictive utility of Mb, PCT, and combined model for post-LT EAD is presented in Table 4. The AUC of serum Mb prediction was 0.657 (95% CI 0.561–0.754, sensitivity = 47.2%, specificity = 85.6%). Although Mb combined PCT to predict, the prediction was not good (AUC = 0.695, 95% CI 0.602–0.788, sensitivity = 62.4%, specificity = 72.2%). The ROC curve analysis is shown in Figure 2.
Figure 2. ROC curves of Mb, PCT, and Mb + PCT for EAD. The AUC values of Mb, PCT, and Mb + PCT were 0.657, 0.635, and 0.695, respectively. In total, 1327 ng/ml was the best cutoff value of myoglobin for predicting EAD (sensitivity = 47.2%, specificity = 85.6%). AUC, area under the curve.
We examined changes in Mb and found that the level of Mb decreased gradually after LT, as shown in Figure 3. A significant difference could be discovered between the two groups for different periods within 3 days after LT (P < 0.05).
Figure 3. Distribution characteristics of myoglobin within 3 days between the EAD group and the non-EAD group after LT. The level of Mb decreased gradually after LT. Mb, myoglobin; EAD, early allograft dysfunction; POD, postoperative day; LT, liver transplantation. **P < 0.01 compared with the non-EAD group.
A previous study has identified MELD score, recipient age, and duration of surgery as risk factors for EAD. So, we performed a subgroup analysis based on these factors. In patients with preoperative MELD ≤ 15, the model of Mb, PCT, and Mb + PCT showed good prediction (AUC = 0.751, 0.656, 0.760, respectively), which was better in patients with duration of surgery ≥ 10 h (AUC = 0.758, 0.760, 0.800, respectively) (Figure 4).
Figure 4. Subgroup analysis for ROC curves. (A) ROC curves of Mb, PCT, and Mb + PCT for EAD in patients whose preoperative MELD ≤ 15; (B) ROC curves of Mb, PCT, and Mb + PCT for EAD in patients whose operative time ≥ 10 h. The predictive value was better in patients whose preoperative MELD ≤ 15 or operative time ≥ 10 h (AUC = 0.751, 0.758, respectively, for Mb).
As shown in Supplementary Figure S1, we found that an increased duration of surgery (≥10 h) significantly increased the serum level of Mb (P = 0.003). However, no linear relationship existed between operative time and Mb. Also, comparing perioperative variables, operative time was not associated with EAD (P = 0.265).
Liver transplantation is the most effective treatment for patients with liver tumors or liver failure. With improved surgical techniques, the complications after LT have decreased significantly over the past decade. In our center, orthotopic liver transplantation (OLT) is mostly used, and the surgical technology is relatively mature. However, EAD is still a common complication after LT (2). Development of EAD after LT can impact short- and long-term prognosis. Thus, a better understanding of risk factors associated with EAD was critical for improving mortality.
Our current article demonstrated an independent association of the level of Mb on PDO0 with the incidence of EAD after LT. In our multivariate logistic analysis, we found that the risk of postoperative EAD was higher in patients with high Mb levels. However, its predictive value was limited. Accordingly, we performed subgroup analysis. For patients with preoperative MELD ≤ 15 or operative time ≥ 10 h, Mb could attain higher prediction. In addition, we observed a declined tendency of the Mb level from POD0 to POD3, and a statistical difference was also discovered between patients with EAD and those without EAD within 3 days after surgery. To our knowledge, the present study is the first to describe the predictive utility of postoperative Mb for EAD after LT.
The estimated incidence of EAD after LT varies in different reports (2, 19, 20). A study demonstrated that the incidence of EAD was 46.4% (21). However, in the present study, 35.3% of patients experienced EAD after LT. The major reason may be the drafts from DBD in our study. In addition, the occurrence of EAD can significantly result in poor outcomes and high mortality. In this study, patients with EAD experienced prolonged ICU stays and higher motility within 28 days. So, it is of significance to identify innovative clinical markers for early recognition of EAD. However, the etiology of EAD is still unclear and complex. IRI was mostly believed to lead to EAD after LT (6). IRI is associated with hypoxia of liver cells, sinusoidal endothelial cell damage, or the release of inflammatory factors (4, 20, 22).
A number of risk factors for the incidence of EAD have been identified by previous studies including recipient-related, donor-related, and surgical factors (7, 9, 23). Golse et al. reported the potentially predictive utility of lactate for EAD. They indicated that arterial lactate concentration at the end of LT, as a reflection of tissue perfusion, was a strong predictor of EAD (24). A similar result was observed in our study, but serum lactate was not a predictor of EAD. The possible reason is that lactate was acquired immediately after admission to ICU. Kuse et al. demonstrated that PCT, which is mainly produced by the liver, allowed differentiation between infection and rejection after LT (25). Our study found that PCT was associated with a higher risk of EAD. Also, previous studies have identified that PCT could reflect the severity of liver injury (26). In addition to these risk factors, some other variables, to our knowledge, were also the risk factors for the incidence of EAD. Quite a few studies have confirmed that donor age over 60 years, donor BMI, graft steatosis, predictive serum sodium, cold ischemia time, and warm ischemia time were important risk factors for EAD after LT (5, 27–29).
Myoglobin is found in skeletal muscle and heart muscle cells and will be released into the blood when a muscle is damaged. During LT, muscle damage occurs by prolonged postural retention and intraoperative muscle pulling, and when rhabdomyolysis occurs, Mb is released into the blood (30). During liver transplantation, the prolonged operative time means longer immobilization or certain surgical positions, which will lead to varying degrees of muscle damage. In addition, due to the presence of anhepatic phase and IRI during LT in all patients, muscle cell injury also results from insufficient blood supply and microcirculatory disturbance (31). In our current study, we demonstrated that prolonged operative time leads to higher postoperative serum Mb. The reason is that this phenomenon may result from more serious muscle damage in prolonged surgery.
In the previous study performed by Fricke et al., they indicated that the level of Mb could predict the extent of impairment after revascularization (32). When Mb was more than 20,000 µg/L, there was an obvious correlation with organ failure. Our results also showed that an increased serum Mb level was closely related to the incidence of EAD after LT. Moreover, the elevated Mb may be related to an inflammatory reaction, and a previous study indicated that elevated Mb is associated with sepsis (33). Before our study, there was no study on the relation between Mb and the occurrence of EAD. Thus, the pathophysiological of Mb predicting utility for EAD after LT was unclear. A previous study discovered that the level of serum Mb peaked a few hours after reperfusion during surgery (32), and quick reperfusion may help reduce Mb release into the bloodstream. Clinically, serum myoglobin can be partially removed by continuous renal replacement therapy and hemoperfusion. Our findings may provide a new direction for treating EAD in patients who underwent LT. In addition, we believed that the level of Mb might reflect the severity of inflammatory responses, which play an important role in the occurrence of EAD after LT. Further study of animal experiments is still needed to explore the pathophysiological mechanism.
Our innovative study is the first to discover the association between postoperative Mb and the risk of EAD. Despite being novel, there are a few limitations to this study. First, it was limited due to a single center and department with a small sample size. Second, although one person finished data collection, there was still bias. Finally, as the study was performed in a single department, which was the surgical ICU, the variables related to donors that may influence the occurrence of EAD were difficult to obtain.
Among patients after LT, postoperative Mb gradually decreased within 3 days after surgery. Mb might be a novel predictor of EAD. In addition, the predictive value of Mb combined PCT was better, especially in patients with preoperative MELD ≤ 15 or operative time ≥ 10 h.
The raw data supporting the conclusions of this article will be made available by the authors, without undue reservation.
The studies involving human participants were reviewed and approved by Beijing Chao-yang Hospital Affiliated with Capital Medical University (approval number: 2021-55). The patients/participants provided their written informed consent to participate in this study.
JZ, YZH, SHK, WXL and LFH designed research, performed study, collected data, analyzed data, drafted paper. XCS, RYG, SZ and PY collected data and revised the manuscript. JZ, HMJ and QD analyzed data, revised the manuscript. YZ assisted with the compilation, collected data and revised the manuscript. All authors were approval of the submitted and final versions.
This study was supported by the Multidisciplinary Clinical Research Innovation Team Project of Beijing Chaoyang Hospital Affiliated to Capital Medical University (no. CYDXK202212), the Beijing Natural Science Foundation (no. 7222065), the Beijing Key Clinical Specialty Excellence Project.
The authors declare that the research was conducted in the absence of any commercial or financial relationships that could be construed as a potential conflict of interest.
All claims expressed in this article are solely those of the authors and do not necessarily represent those of their affiliated organizations, or those of the publisher, the editors and the reviewers. Any product that may be evaluated in this article, or claim that may be made by its manufacturer, is not guaranteed or endorsed by the publisher.
The Supplementary Material for this article can be found online at: https://www.frontiersin.org/articles/10.3389/fsurg.2022.1026586/full#supplementary-material.
1. Briceño J, Ciria R. Early graft dysfunction after liver transplantation. Transplant Proc. (2010) 42:631–3. doi: 10.1016/j.transproceed.2010.02.004
2. Deschenes M. Early allograft dysfunction: causes, recognition, and management. Liver Transpl. (2013) Suppl 2:S6–8. doi: 10.1002/lt.23746
3. Brea-Gómez E, Villar-Quintana R, Plata-lllescas C, Zambudio-Carroll N, Lopez-Garrido MA, Nogueras-Lopez F, et al. Analysis of the predictive ability for graft loss and mortality of two criteria for early allograft dysfunction after liver transplantation. Transplant Proc. (2018) 50:605–9. doi: 10.1016/j.transproceed.2017.11.057
4. Zhou J, Chen J, Wei Q, Saeb-Parsy K, Xu X. The role of ischemia/reperfusion injury in early hepatic allograft dysfunction. Liver Transpl. (2020) 26:1034–48. doi: 10.1002/lt.25779
5. Bastos-Neves D, Salvalaggio P, Almeida M. Risk factors, surgical complications and graft survival in liver transplant recipients with early allograft dysfunction. Hepatob Pancreat Dis Int. (2019) 18:423–9. doi: 10.1002/lt.25779
6. Zhai Y, Petrowsky H, Hong JC, Busuttil RW, KupiecWeglinski JW. Ischaemia-reperfusion injury in liver transplantation – from bench to bedside. Nat Rev Gastro Hepat. (2013) 10:79–89. doi: 10.1038/nrgastro.2012.225
7. Park J, Lim SJ, Choi HJ, Hong SH, Park CS, Choi JH, et al. Predictive utility of the C-reactive protein to albumin ratio in early allograft dysfunction in living donor liver transplantation: a retrospective observational cohort study. PLoS One. (2019) 14:e0226369. doi: 10.1371/journal.pone.0226369
8. Lv T, Kong L, Yang J, Wu H, Wen T, Jiang L, et al. The postoperative hepatic artery resistance index after living donor liver transplantation can predict early allograft dysfunction. Medicine. (2020) 99:e18677. doi: 10.1097/MD.0000000000018677
9. Hu LM, Tsai HI, Lee CW, Chen HM, Lee WC, Yu HP. Uric acid as a predictor for early allograft dysfunction after living donor liver transplantation: a prospective observational study. J Clin Med. (2021) 10:2729. doi: 10.3390/jcm10122729
10. Laurence A. Serum myoglobin and creatine kinase following surgery. Brit J Anaesth. (2000) 84:763–6. doi: 10.1093/oxfordjournals.bja.a013590
11. Chun K, Chung W, Kim AJ, Kim H, Ro H, Chang JH, et al. Association between acute kidney injury and serum procalcitonin levels and their diagnostic usefulness in critically ill patients. Sci Rep. (2019) 9:4777. doi: 10.1038/s41598-019-41291-1
12. Carrier FM, Chasse M, Wang HT, Aslanian P, Iorio S, Bilodeau M, et al. Restrictive fluid management strategies and outcomes in liver transplantation: a systematic review. Can J Anaesth. (2020) 67:109–27. doi: 10.1007/s12630-019-01480-y
13. Hachey BJ, Kontos MC, Newby LK, Christenson RH, Peacock WF, Brewer KC, et al. Trends in use of biomarker protocols for the evaluation of possible myocardial infarction. J Am Heart Assoc. (2017) 6:e005852. doi: 10.1161/JAHA.117.005852
14. Han YZ, Zhang J, Gao RY, Zhao S, Zheng Y, Ding Q, et al. Predictive utility of postoperative serum myoglobin in acute kidney injury after liver transplantation. Ann Palliat Med. (2021) 10:11265–77. doi: 10.21037/apm-21-2340
15. Li X, Yan H, Zhang X, Huang J, Xiang ST, Yao Z, et al. Elevated serum myoglobin levels at hospital admission and the risk of early death among patients with hemophagocytic lymphohistiocytosis: evidence from 155 pediatric patients. Ann Hemato. (2020) 99:963–71. doi: 10.1007/s00277-020-03980-0
16. Hofmann D, Buettner M, Rissner F, Wahl M, Sakka SG. Prognostic value of serum myoglobin in patients after cardiac surgery. J Anesth. (2007) 21:304–10. doi: 10.1007/s00540-007-0507-0
17. Olthoff KM, Kulik L, Samstein B, Kaminski M, Abecassis M, Emond J, et al. Validation of a current definition of early allograft dysfunction in liver transplant recipients and analysis of risk factors. Liver Transpl. (2010) 16:943–9. doi: 10.1002/lt.22091
18. Ostermann M, Joannidis M. Acute kidney injury 2016: diagnosis and diagnostic workup. Crit Care. (2016) 20:299. doi: 10.1186/s13054-016-1478-z
19. Xie H, Zhang L, Guo D, Yang Z, Zhu H, Zhou K, et al. Protein profiles of pretransplant grafts predict early allograft dysfunction after liver transplantation from donation after circulatory death. Transplantation. (2020) 104:79–89. doi: 10.1097/TP.0000000000002787
20. Ito T, Naini BV, Markovic D, Aziz A, Younan S, Lu M, et al. Ischemia-reperfusion injury and its relationship with early allograft dysfunction in liver transplant patients. Am J Transplant. (2021) 21:614–25. doi: 10.1111/ajt.16219
21. Wang K, Lu D, Liu YH, Li W, Zhuang L, Ma Z, et al. Severity of early allograft dysfunction following donation after circulatory death liver transplantation: a multicentre study. Hepatobiliary Surg Nutr. (2021) 10:9–19. doi: 10.21037/hbsn.2019.09.02
22. Pomposelli J, Goodrich NP, Emond JC, Humar A, Baker TB, Grant DR, et al. Patterns of early allograft dysfunction in adult live donor liver transplantation: the A2ALL experience. Transplantation. (2016) 100:1490–9. doi: 10.1097/TP.0000000000001240
23. Yoshino O, Wong BKL, Cox DR, Lee E, Hepworth G, Christophi C, et al. Elevated levels of circulating mitochondrial DNA predict early allograft dysfunction in patients following liver transplantation. J Gastroen Hepatol. (2021) 36:3500–7. doi: 10.1111/jgh.15670
24. Golse N, Guglielmo N, Metni AEI, Frosio F, Cosse C, Naili S, et al. Arterial lactate concentration at the end of liver transplantation is an early predictor of primary graft dysfunction. Ann Surg. (2018) 1:131–8. doi: 10.1097/SLA.0000000000002726
25. Kuse ER, Langefeld I, Jaeger K, Kulpmann WR. Procalcitonin-a new diagnostic tool in complications following liver transplantation. Intensive Care Med. (2000) Suppl 2:187–92. doi: 10.1007/BF02900736
26. Qu J, Feng P, Luo Y, Lv XJ. Impact of hepatic function on serum procalcitonin for the diagnosis of bacterial infections in patients with chronic liver disease: a retrospective analysis of 324 cases. Medicine. (2016) 95:e4270. doi: 10.1097/MD.0000000000004270
27. Lee DD, Croome KP, Shalev JA, Musto KR, Sharma M, Keaveny AP, et al. Early allograft dysfunction after liver transplantation: an intermediate outcome measure for targeted improvements. Ann Hepatol. (2016) 15:53–60. doi: 10.5604/16652681.1184212
28. Park JB, Kwon CH, Choi GS, Chun JM, Jung GO, Kim SJ, et al. Prolonged cold ischemic time is a risk factor for biliary strictures in duct-to-duct biliary reconstruction in living donor liver transplantation. Transplantation. (2008) 86:1536–42. doi: 10.1097/TP.0b013e31818b2316
29. Feng S, Goodrich NP, Bragg-Gresham JL, Dykstra DM, Punch JD, Debroy MA, et al. Characteristics associated with liver graft failure: the concept of a donor risk index. Am J Transplant. (2006) 6:783–90. doi: 10.1111/j.1600-6143.2006.01242.x
30. Huerta-Alardín AL, Varon J, Marik PE. Bench-to-bedside review: rhabdomyolysis – an overview for clinicians. Crit Care. (2005) 9:158–69. doi: 10.1186/cc2978
31. Hendgen-Cotta UB, Kelm M, Rassaf T. Myoglobin functions in the heart. Free Radical Bio Med. (2014) 73:252–9. doi: 10.1016/j.freeradbiomed.2014.05.005
32. Fricke P, Wei G, Lippert H. Ischmie-/reperfusions-syndrom – das serummyoglobin im verlauf und seine wertigkeit als prognoseparameter eine retrospektive analyse klinischer daten. Med Klin-Intensivmed. (2002) 39:38–46. doi: 10.1007/s003900200004
Keywords: myoglobin, early allograft dysfunction, liver transplantation, predictor, complication
Citation: Zhang J, Han YZ, Ke SH, Gao RY, Shi XC, Zhao S, You P, Jia HM, Ding Q, Zheng Y, Li WX and Huang LF (2022) Postoperative serum myoglobin as a predictor of early allograft dysfunction after liver transplantation. Front. Surg. 9:1026586. doi: 10.3389/fsurg.2022.1026586
Received: 24 August 2022; Accepted: 12 September 2022;
Published: 12 October 2022.
Edited by:
Hans-Jonas Meyer, Leipzig University, GermanyReviewed by:
Birkan Bozkurt, Başakşehir Çam / Sakura City Hospital Organ Transplant Center, Turkey© 2022 Zhang, Han, Ke, Gao, Shi, Zhao, You, Jia, Ding, Zheng, Li and Huang. This is an open-access article distributed under the terms of the Creative Commons Attribution License (CC BY). The use, distribution or reproduction in other forums is permitted, provided the original author(s) and the copyright owner(s) are credited and that the original publication in this journal is cited, in accordance with accepted academic practice. No use, distribution or reproduction is permitted which does not comply with these terms.
*Correspondence: Li-Feng Huang YnVybnNobGZAc2luYS5jb20= Wen-Xiong Li bHd4NzExNUBzaW5hLmNvbQ==
†These authors have contributed equally to this work
Specialty Section: This article was submitted to Visceral Surgery, a section of the journal Frontiers in Surgery
Disclaimer: All claims expressed in this article are solely those of the authors and do not necessarily represent those of their affiliated organizations, or those of the publisher, the editors and the reviewers. Any product that may be evaluated in this article or claim that may be made by its manufacturer is not guaranteed or endorsed by the publisher.
Research integrity at Frontiers
Learn more about the work of our research integrity team to safeguard the quality of each article we publish.