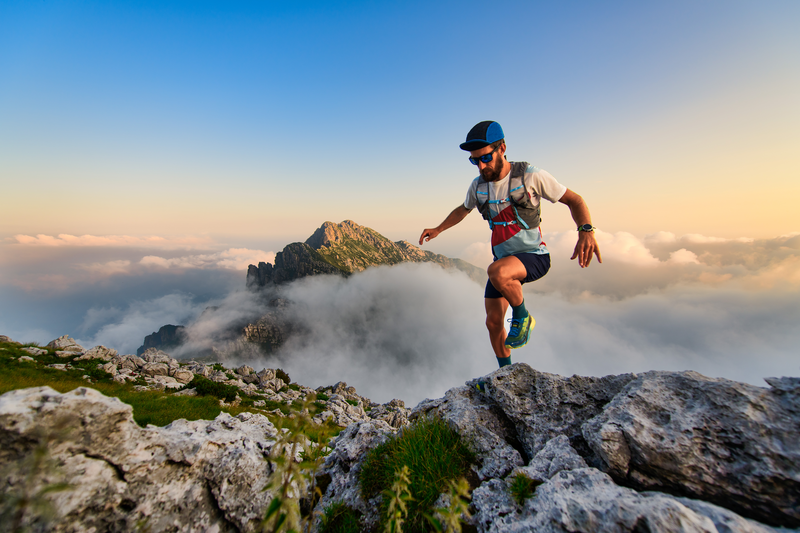
94% of researchers rate our articles as excellent or good
Learn more about the work of our research integrity team to safeguard the quality of each article we publish.
Find out more
PERSPECTIVE article
Front. Surg. , 25 March 2021
Sec. Genitourinary Surgery and Interventions
Volume 8 - 2021 | https://doi.org/10.3389/fsurg.2021.649418
This article is part of the Research Topic Current Perspectives in Robotic Kidney Transplantation View all 7 articles
Kidney transplantation is universally recognized as the gold standard treatment in patients with End-stage Kidney Disease (ESKD, or according to the latest nomenclature, CKD stage 5). Robot-assisted kidney transplantation (RAKT) is gradually becoming preferred technique in adults, even if applied in very few centra, with potentially improved clinical outcomes compared with open kidney transplantation. To date, only very few RAKT procedures in children have been described. Kidney transplant recipient patients, being immunocompromised, might be at increased risk for perioperative surgical complications, which creates additional challenges in management. Applying techniques of minimally invasive surgery may contribute to the improvement of clinical outcomes for the pediatric transplant patients population and help mitigate the morbidity of KT. However, many challenges remain ahead. Minimally invasive surgery has been consistently shown to produce improved clinical outcomes as compared to open surgery equivalents. Robot-assisted laparoscopic surgery (RALS) has been able to overcome many restrictions of classical laparoscopy, particularly in complex and demanding surgical procedures. Despite the presence of these improvements, many challenges lie ahead in the surgical and technical–material realms, in addition to anesthetic and economic considerations. RALS in children poses additional challenges to both the surgical and anesthesiology team, due to specific characteristics such as a small abdominal cavity and a reduced circulating blood volume. Cost-effectiveness, esthetic and functional wound outcomes, minimal age and weight to undergo RALS and effect of RAKT on graft function are discussed. Although data on RAKT in children is scarce, it is a safe and feasible procedure and results in excellent graft function. It should only be performed by a RAKT team experienced in both RALS and transplantation surgery, fully supported by a pediatric nephrology and anesthesiology team. Further research is necessary to better determine the value of the robotic approach as compared to the laparoscopic and open approach. Cost-effectiveness will remain an important subject of debate and is in need of further evaluation as well.
Kidney transplantation (KT) is universally recognized as the gold standard treatment in the adult and pediatric populations with patients with End-Stage Kidney Disease (ESKD).
In contrast to the adult population, KT has taken longer to become an established and preferred treatment for pediatric patients with ESKD (1). KT offers major advantages to the pediatric population such as catch-up growth (2) and longer survival (3). Immunosuppresive treatment increases the risk for perioperative surgical complications which creates additional challenges in management of kidney transplant recipients. Specifically, wound-related complications have been shown to contribute significantly to morbidity post-transplant (4). Applying techniques of minimally invasive surgery may contribute to the improvement of clinical outcomes for the pediatric ESKD population and help mitigate the morbidity of KT.
The past decade has produced greatly improved KT outcomes largely due to enhanced immunosuppressive therapy, expanded living kidney donor transplantation, advances in perioperative care, better pre-transplant preparation of the recipient and improved surgical techniques in kidney extraction from donors. At present, survival rates at 1-, 5- and 10-years post-transplant are 98, 95, and 91%, respectively (5). Survival rates are higher in recipients of living donor kidneys as compared to recipients of deceased donor kidneys (5). It should be noted that patient comfort is higher the procedure is pre-emptive, which is usually thanks to living donor, even if cadaveric donor is possible. Pre-emptive grafting means the patient does not have to undergo dialysis, the kidney graft is achieved before the patient is in need for dialysis.
Progression in robot-assisted laparoscopic surgery (RALS) has revolutionized minimally invasive surgery. Although it is routinely utilized in urology, RALS has remained controversial since it is frequently challenging to demonstrate clear superiority in outcomes when compared to open or classical laparoscopic surgery, whilst also being quite expensive (6). Adaptation of RALS in children is even more hazardous than in adults as the medical equipment for the procedure is produced for use in adult-sized bodies (7).
Usage of RALS in the pediatric urology population was first described by Craig Peters's team in 2002 after they performed a pyeloplasty for an uretero-pelvic junction obstruction (Personal communication, Craig Peters, Children's Hospital in Boston, 2002). Since then, surgical indications for robotics in pediatric urology have expanded substantially. The principal robot-assisted (RA) procedures in children are RA pyeloplasty, RA hemi-nephrectomy, RA nephroureterectomy, RA ureteral reimplantation, RA bladder augmentation and RA Mitrafanoff appendicovesicostomy (8). Because of the insufficient evidence-base related to the lack of randomized-controlled trials, pyeloplasty surgery is the only procedure acknowledged by the European Association of Urology Guidelines on Pediatric Urology as equally successful whether performed using an open, laparoscopic or robotic approach (provided that the surgeon is experienced in the chosen technique) (9). Better cosmesis, less pain and less hospital stay are the main three advantages of robotic surgery stated in the guidelines.
Minimally invasive surgery such as laparoscopic surgery or RALS has consistently shown to produce improved clinical outcomes as compared to open surgery equivalents. These outcomes include fewer complications, reduced length of hospital stay, reduced need for blood transfusion, less postoperative pain, shorter convalescence period, fewer surgical site infections, and better cosmetic results (10–12).
RALS has been able to overcome many restrictions of classical laparoscopy, particularly in complex and demanding surgical procedures (e.g., kidney transplantation). Some major advantages include a superb three-dimensional vision, image magnification, elimination of hand tremor and movement scaling, control of the camera by the surgeon, good surgeon ergonomics to lessen fatigue, and articulated instruments with seven degrees of freedom of movement (13).
Despite the presence of these improvements, many challenges lie ahead in the surgical and technical–material realms, in addition to anesthetic and economic considerations (7, 8).
The first adult robot-assisted kidney transplantation (RAKT) was performed by Giulianotti et al. (14) in 2010. In 2014, Menon et al. (15) standardized the transperitoneal approach with regional hypothermia maintenance during the re-warming time. In the years since, RAKT has become an increasingly common procedure in selected high-volume centers. This can be attributed to promising results which have indicated RAKT to be a safe, feasible and reproducible procedure with a potentially improved morbidity as compared to open kidney transplantation (OKT) (16, 17).
However, this evidence is largely limited to adults (16, 18, 19). Children are not just ‘small adults' and must be assessed within their own separate population, established results in adults cannot be easily extrapolated to the pediatric population. To date, RAKT experience remains limited in the pediatric population.
The first full case report on pediatric (8 years old child) RAKT was published in 2019 by Decaestecker and team at Ghent University Hospital, Belgium. It demonstrated that RAKT in children is technically feasible and safe, and resulted in excellent graft function. Concomitant nephrectomy was done laparoscopically through the single-port GelPOINT® (AFS), while another team retrieved the living donor kidney using robotic assistance (KDK). In order to minimize ischemia in the donated kidney, the harvesting in the living donor and the transplant preparation of the child were simultaneously performed in two operating rooms by two different transplant teams (AFS—KDK). It should be noted that, given these demanding and complex aspects of the procedure, pediatric RAKT should only be attempted by an experienced RAKT team fully supported by a pediatric nephrology and anesthesiology team (20).
Recently, Bansal et al. released a comparative analysis of outcomes and long-term follow-up of pediatric RAKT with an open kidney transplantation (OKT) counterpart (21). Twenty-five patients were included in the study, 21 of whom underwent OKT, and four of whom underwent RAKT. A significantly higher re-warming ischemia time was noted in the RAKT group (21), but that may be attributable to the initial phase of the learning curve for the procedure, a phenomenon which was similarly observed in the initial phases of adult RAKT (16, 18). Peri-operative analgesic requirements were significantly higher among the OKT group as compared to the RAKT group. RAKT complications included transplant renal artery stenosis and subcapsular hematoma, albeit not exclusive to RAKT. Despite the limitations of this study, it demonstrated that pediatric RAKT is a feasible and safe procedure that results in excellent graft function (21).
In addition to these two articles, our literature search revealed two abstracts discussing pediatric RAKT. Modi et al. (22) described a group of 5 children undergoing RAKT in 2013. All but one kidney were issued from living donors. In two children, concomitant seminal vesicle cyst excision and nephrectomy were carried out. One child experienced spontaneous graft rupture on the 3rd postoperative day requiring emergency exploration (22). A prospective non-randomized open label trial by Patel et al. (23) compared outcomes of open vs. robot-assisted pediatric KT at a single center, involving 60 and 22 patients undergoing RAKT and OKT, respectively. Endpoints of their trial were feasibility of RAKT and creatinine value at 30 days post-transplantation. Both ureteric reimplantation time and intraoperative blood loss were significantly decreased in the RAKT group. In the RAKT group (n = 60), five patients had slow graft function, and seven patients lost graft during follow-up. Two patients had acute antibody-mediated rejection, four had chronic rejection, and one had de novo collapsing glomerulopathy with BKV nephropathy. Five patients from the RAKT group deceased during follow-up. In the OKT group (n = 20), two patients had slow graft function, two lost graft, and two deceased during follow-up. There was no significant observed difference in graft survival or overall survival (23). Both Modi et al. and Patel et al. (22, 23) concluded that RAKT is feasible and safe, however, a more thorough and comprehensive analysis of the specifics of their experiences could help increase the external validity of their findings.
Based on our extensive experience in RALS in pediatric urology (24–26) and robotic kidney transplantation surgery (16, 27, 28), we decided to initiate a pediatric robot-assisted kidney transplantation program. For the time being, we have limited the program to cases of grafting performed with living donor transplants. Since the first pediatric RAKT case in 2018 (20), we have operated on an additional two children with good results, and our program has continued to advance. We continue to monitor our data and hope to further develop our experience in this unique field and improve patient outcomes.
End-stage Kidney disease (ESKD) occurs in about 5–10 children per million each year (29). Although early pediatric KT was complicated by technical, immunologic, and logistic problems, advances in these areas have led to dramatic improvements and KT has become the preferred treatment for pediatric ESKD patients (1). Innovations in minimally invasive surgical techniques that have been successful in the adult population should be offered to children as well if the benefits are comparable. Nonetheless, several considerations must first be addressed regarding different aspects of a RAKT.
Anesthetists should consider the following challenges in robotic surgical case: increased abdominal pressure, hypothermia, increased CO2 absorption, and the physiologic effects of Trendelenburg position. These may impair cardiovascular function and ventilation, induce cerebral vasodilatation, and negatively affect urine output (30). Anesthetists should take note that intra-abdominal pressures lower than 10 mmHg do not appear to induce any significant clinical hemodynamic effects, and pressures up to 12 mmHg seem to be well-tolerated (31).
The relatively small size of the abdominal cavity of a child presents multiple operative challenges. This decreased workspace in pediatric abdominal surgeries makes trocar placement a critical procedural component as it can impact desufflation risk, bleeding risk, and organ damage. Poorly positioned trocars may generate a gas leak, provoking rapid desufflation and further decreasing the small workspace (32). The use of the AirSeal® system can help to maintain a stable working space with a very low pneumoperitoneum pressure. At our center, it was used in all our pediatric RAKT procedures. Bleeding can be particularly dangerous in children, as their already limited circulating volume causes any unrecognized or uncontrolled bleeding to be hazardous to cardiovascular function (32). Pediatric abdominal cavities typically contain adjacent organs in tight proximity to each other, increasing the risk for damage to surrounding organs during surgical procedures. Therefore, surgeons should be aware that open placement of the first trocar under direct vision (Hasson technique) is the preferred method for the pediatric population (33, 34).
Trocar positioning is also important for maximization of robot dexterity within the already restricted pediatric abdominal cavity. The recommended 8 cm gap for collision avoidance between the robot arms in adult RALS cases is often impossible in children due to workspace space limitations. Contrary to some adult procedures, there is no standardized trocar placement in robotic pediatric procedures due to broad variations in weight and height which are dependent on patient age. Trocar positioning should therefore be individually adjusted to allow optimal workspace (8). It is also worth to mention that the later generation robots (such as Da Vinci Xi and X) facilitate the docking procedure related to easy connection of instruments and the increased movement ability of the patient cart.
Additionally, while most robotic adult procedures involve four robot arms, the limited workspace in pediatric patients commonly only allows usage of three out of four robot arms (camera port and two robotic ports) without any additional 5-mm assisting port (8). In our initial phase of RAKT in children, our team opted for four robot arms with two assisting ports (the standardized adult port position) to limit operative time and more specifically to limit the (re)warm(ing) ischemia time, as these factors are potentially associated with adverse long-term patient and graft survival outcomes (35).
Current studies have not found RALS to be cost-effective (6). It has been suggested that RALS may prove cost-effective in high-volume centers with at least 349 annual interventions (36). Therefore, cooperation between pediatric and adult urology units might be necessary as the volume for pediatric urology RALS is not as high as it is in adults (8). RALS may become more cost-effective as more experience is gained, leading to improved outcomes and reduced length of hospital stay. This issue remains to be further evaluated.
Figure 1, also published in a previous paper of our group (8), provides a diagrammatic overview of the main challenges in adapting the robotic platform to children.
The differences in both the esthetic and functional outcomes of RAKT as compared to OKT must not be underestimated (21, 37, 38). RAKT can deliver superior esthetic outcomes, which are especially desired for young children (21). Barbosa et al. (38) demonstrated that parents consistently perceive smaller scars resulting from RALS as esthetically superior to open surgical scars following pediatric urological procedures. Regardless of scarring, most parents ultimately base their choice of surgical technique on superiority of clinical outcomes (38). Surgeons should therefore discuss differences in clinical outcomes with their patient's parents, in addition to the resultant scarring of different approach modalities.
To date, an eight kilograms cut-off value is the lowest weight allowed for consideration of robot-assisted minimally invasive surgery in children. For RAKT specifically, 8 years of age is considered to be the minimum threshold, as most transplanted kidneys are adult-sized kidneys which are then transplanted into a smaller child. At our center, we were able to adopt the standard trocar configuration from adult RAKT to pediatric RAKT up to a lower age limit of 8 years old. Extending to younger children would necessitate further adjustment of the trocar configuration, but would as well be very challenging or impossible due to the size of an adult-sized kidney in the particularly small pneumoperitoneum space of a small child. Therefore, 8 years of age is currently considered to be the minimum threshold for RAKT.
As the length of the incision in open surgery in smaller children is limited, there is discussion whether this population would benefit from this approach. While RA laparoscopic approach can provide better visualization with decreased inflammatory reaction and wound tension, opponents argue that incisions for open surgery are already small in size and that the sum of laparoscopic incisions equals the total length of an open incision (8, 39). However, this argument assumes that surgical morbidity is proportional to the sum of several incisions. However, morbidity has already proven to be a linear function of the tension present across the incision. As calculated by Blinman (37), an open incision is consistently subject to more total closing tension than any combination of trocar incisions of equal total length. By decreasing wound tension, there is an important reduction in pain and morbidity (risk of dehiscence, hernia or infection) (37).
In order to start a pediatric RAKT program, a team of surgeons experienced both in RALS and kidney transplantation surgery, along with the full support of a pediatric nephrology and anesthesiology team, is recommended. Even during the training phase of learning how to perform RAKT, the safety of the procedure and optimal graft survival should be guaranteed.
According to Decaestecker et al. (27), it cannot be emphasized enough that a high level of robotic experience is recommended before initiating a RAKT program. Training the technique on both dry and wet lab models is mandatory (27). The only structured RAKT course, provided at ORSI Academy (Melle, Belgium) (40), is recommended. And additional structured RA pediatric course is available at the same place, and is highly recommended. In conjunction with an experienced RAKT proctor supporting first cases, a safe introduction of this new technique is possible (16, 27, 41, 42).
Warm ischemia time has already been shown to be a negative predictive factor of long-term patient and graft survival outcomes (35). In the adult RAKT series, a wide range of ischemia times has been described, but no correlation has been found between rewarming time and graft function (16, 17). To reduce potential damage after prolonged warm ischemia times, Menon et al. have reported a new technique for regional hypothermia with ice slush during vascular anastomosis (15).
Contrary to the adult RAKT procedure, in pediatric RAKT the graft renal vessels are anastomosed to the common iliac vessels instead of the external iliac vessels, in order to match the vessel size of the donor (adult-sized renal vessels) and acceptor (pediatric-sized iliac vessels) (20, 21).
It should be noted that according to the literature, renal blood flow and function are reduced in the presence of pneumoperitoneum, potentially leading to graft impairment (43). The potential damage to the graft as a result of pneumoperitoneum is not yet fully known, and a correlation between operative time (and thus exposure to pneumoperitoneum) and graft function in adult RAKT has not yet been identified (16). Aside from the anesthetic concerns regarding intra-abdominal pressure, this might be another reason to keep intra-abdominal pressure around 10 mmHg or lower. We prefer a stable low pneumoperitoneum pressure of 8 mmHg for the (RA) laparoscopic living donor nephrectomy and for RAKT as of the moment of reperfusion to mitigate any potential adverse influence of higher pneumoperitoneum pressures in donor and/or recipient (44, 45).
Differences regarding complications and length of hospital stay after RAKT as compared to OKT are still subject to debate. To date, RAKT in children remains in the initiation phase. A larger patient database could lead to more valuable conclusions. A randomized controlled trial comparing outcomes of RAKT and OKT should provide the best level of evidence.
Although data on RAKT in children is scarce, it is a safe and feasible procedure and results in excellent graft function. It should only be performed by a RAKT team experienced in both robot-assisted laparoscopic surgery and transplantation surgery, fully supported by a team of pediatric nephrologists and anesthesiologists. Further research is necessary to better determine the value of the robotic approach as compared to the laparoscopic and open approach. Cost-effectiveness will remain an important subject of debate and is in need of further evaluation as well.
The original contributions generated for this study are included in the article/supplementary material, further inquiries can be directed to the corresponding author/s.
JG was the main author and carried out literature search. KD and A-FS performed the initial surgery reported in Spinoit et al. (20). A-FS initiated the scientific project and develops the pediatric robotic program of minimally invasive surgery. KD initiated the scientific and develops the surgical robotic kidney transplantation program in Ghent. AB and MS are promotors of the international robotic programs, respectively for Kidney transplant and for pediatric urology. They critically revised the manuscript and approved the submitted version. All authors from Ghent are part of the pediatric uro-nephrology team making the development of the program possible and critically revised the manuscript and approved the submitted version.
MS was fully supported by the Gianni Eggermont Fund for advancement of research in pediatric Urology.
KD is a consultant for Intuitive Surgical, Sunnyvale, CA, United States of America.
The remaining authors declare that the research was conducted in the absence of any commercial or financial relationships that could be construed as a potential conflict of interest.
1. Dharnidharka VR, Fiorina P, Harmon WE. Kidney transplantation in children. N Engl J Med. (2014) 371:549–58. doi: 10.1056/NEJMra1314376
2. Nissel R, Brázda I, Feneberg R, Wigger M, Greiner C, Querfeld U, et al. Effect of renal transplantation in childhood on longitudinal growth and adult height. Kidney Int. (2004) 66:792–800. doi: 10.1111/j.1523-1755.2004.00805.x
3. McDonald SP, Craig JC. Long-term survival of children with end-stage renal disease. N Engl J Med. (2004) 350:2654–62. doi: 10.1056/NEJMoa031643
4. Humar A, Matas AJ. Surgical Complications After Kidney Transplantation. Vol. 18, Seminars in Dialysis. London: John Wiley & Sons, Ltd (2005). p. 505–10. doi: 10.1111/j.1525-139X.2005.00097.x
5. North American Pediatric Renal Trials and Collaborative Studies (NAPRTCS). 2014 Annual Transplant Report. New york, NY (2014).
6. Schroeck FR, Jacobs BL, Bhayani SB, Nguyen PL, Penson D, Hu J. Cost of new technologies in prostate cancer treatment: systematic review of costs and cost effectiveness of robotic-assisted laparoscopic prostatectomy, intensity-modulated radiotherapy, and proton beam therapy. Eur Urol. (2017) 72:712–35. doi: 10.1016/j.eururo.2017.03.028
7. Spinoit AF, Subramaniam R. Update on the minimally invasive approach in paediatric urology: remote help for human hands? Eur Urol. (2015) 14:20–4. doi: 10.1016/j.eursup.2015.01.006
8. Spinoit A-F, Nguyen H, Subramaniam R. Role of robotics in children: a brave new world! Eur Urol Focus. (2017) 3:172–80. doi: 10.1016/j.euf.2017.08.011
9. Radmayr C, Bogaert G, Dogan HS, Nijman JN, Silay MS, Stein R, et al. European Association of Urology Guidelines. 2020 Edition. In Arnhem: European Association of Urology Guidelines Office (2020).
10. Elsamra SE, Theckumparampil N, Garden B, Alom M, Waingankar N, Leavitt DA, et al. Open, laparoscopic, and robotic ureteroneocystotomy for benign and malignant ureteral lesions: a comparison of over 100 minimally invasive cases. J Endourol. (2014) 28:1455–9. doi: 10.1089/end.2014.0243
11. Traxel EJ, Minevich EA, Noh PH. A Review: the application of minimally invasive surgery to pediatric urology: upper urinary tract procedures. Urology. (2010) 76:122–33. doi: 10.1016/j.urology.2009.11.072
12. Tejwani R, Young BJ, Wang HHS, Wolf S, Purves JT, Wiener JS, et al. Open versus minimally invasive surgical approaches in pediatric urology: trends in utilization and complications. J Pediatr Urol. (2017) 13:283.e1–9. doi: 10.1016/j.jpurol.2017.01.013
13. Territo A, Mottrie A, Abaza R, Rogers C, Menon M, Bhandari M, et al. Robotic kidney transplantation: Current status and future perspectives. Minerva Urol Nefrol. (2017) 69:5–13. doi: 10.23736/S0393-2249.16.02856-3
14. Giulianotti P, Gorodner V, Sbrana F, Tzvetanov I, Jeon H, Bianco F, et al. Robotic transabdominal kidney transplantation in a morbidly obese patient. Am J Transplant. (2010) 10:1478–82. doi: 10.1111/j.1600-6143.2010.03116.x
15. Menon M, Sood A, Bhandari M, Kher V, Ghosh P, Abaza R, et al. Robotic kidney transplantation with regional hypothermia: a step-by-step description of the vattikuti urology institute-medanta technique (IDEAL phase 2a). Eur Urol. (2014) 65:991–1000. doi: 10.1016/j.eururo.2013.12.006
16. Breda A, Territo A, Gausa L, Tugcu V, Alcaraz A, Musquera M, et al. Robot-assisted kidney transplantation: the European experience [Figure presented]. Eur Urol. (2018) 73:273–81. doi: 10.1016/j.eururo.2017.08.028
17. Musquera M, Peri L, Ajami T, Campi R, Tugcu V, Decaestecker K, et al. Robot-assisted kidney transplantation: update from the European Robotic Urology Section (ERUS) series. BJU Int. (2021) 127:222–8. doi: 10.1111/bju.15199
18. Pein U, Girndt M, Markau S, Fritz A, Breda A, Stöckle M, et al. Minimally invasive robotic versus conventional open living donor kidney transplantation. World J Urol. (2020) 38:795–802. doi: 10.1007/s00345-019-02814-7
19. Territo A, Gausa L, Alcaraz A, Musquera M, Doumerc N, Decaestecker K, et al. European experience of robot-assisted kidney transplantation: minimum of 1-year follow-up. BJU Int. (2018) 122:255–62. doi: 10.1111/bju.14247
20. Spinoit AF, Moreels N, Raes A, Prytula A, De Groote R, Ploumidis A, et al. Single-setting robot-assisted kidney transplantation consecutive to single-port laparoscopic nephrectomy in a child and robot-assisted living-related donor nephrectomy: initial Ghent experience. J Pediatr Urol. (2019) 15:578–9. doi: 10.1016/j.jpurol.2019.08.005
21. Bansal A, Maheshwari R, Chaturvedi S, Bansal D, Kumar A. Comparative analysis of outcomes and long-term follow-up of robot-assisted pediatric kidney transplantation, with open counterpart. Pediatr Transplant. (2020) 1:e13917. doi: 10.1111/petr.13917
22. Modi P, Pal B, Modi J, Sarmah A, Kumar S, Kute V, et al. Robotic assisted laparoscopic kidney transplantation in children-an initial experience.: Abstract# A356. Transplantation. (2014) 98:501. doi: 10.1097/00007890-201407151-01673
23. Patel D, Singla SK, Mishra A, Chauhan R, Patel H, Kute V, et al. Prospective non-randomized open label trial comparing outcome of open versus robotic pediatric kidney transplant at single Centre. Transplantation. (2020) 104:S23. doi: 10.1097/01.tp.0000698360.42101.4d
24. Ferong K, Waterschoot M, Sinatti C, Van Laecke E, Cools M, Hoebeke P, et al. Rare and special robotic surgery indications in the pediatric population: ectopic organs and differences of sexual development. World J Urol. (2020) 38:1865–8. doi: 10.1007/s00345-019-02913-5
25. Waterloos M, Ploumidis A, Pappas A, De Bleser E, De Groote R, Weyers S, et al. Robot-assisted resection of ectopic kidney in children: an anatomical illustration. J Pediatr Urol. (2019) 15:87–8. doi: 10.1016/j.jpurol.2018.10.018
26. Silay MS, Spinoit AF, Undre S, Fiala V, Tandogdu Z, Garmanova T, et al. Global minimally invasive pyeloplasty study in children: results from the Pediatric Urology Expert Group of the European Association of Urology Young Academic Urologists working party. J Pediatr Urol. (2016) 12:229.e1–7. doi: 10.1016/j.jpurol.2016.04.007
27. Decaestecker K, Territo A, Campi R, Van Parys B, Bevilacqua G, Desender L, et al. Robot-assisted kidney transplantation. In: Küçük S, Canda AE, editors. Medical Robots - New Achievements. London, UK: IntechOpen (2020). doi: 10.5772/intechopen.90276
28. Decaestecker K, Van Parys B, Van Besien J, Doumerc N, Desender L, Randon C, et al. Robot-assisted kidney autotransplantation: a minimally invasive way to salvage kidneys. Eur Urol Focus. (2018) 4:198–205. doi: 10.1016/j.euf.2018.07.019
29. Disney APS, Russ GR, Walker R, Collins J, Herberrt K, Kerr P. Twenty-second annual report of the Australian and New Zealand dialysis and transplant registry. Adelaide Aust New Zeal Dial Transpl Regist. (1999) 3:21–3.
30. Tobias JD. Anaesthesia for minimally invasive surgery in children. Best Pract Res Clin Anaesthesiol. (2002) 16:115–30. doi: 10.1053/bean.2001.0211
31. Baroncini S, Gentili A, Pigna A, Fae M, Tonini C, Tognù A. Anaesthesia for laparoscopic surgery in paediatrics. Minerva Anestesiol. (2002) 68:406–13.
32. Peters CA. Robotically assisted surgery in pediatric urology. Urol Clin North Am. (2004) 31:743–52. doi: 10.1016/j.ucl.2004.06.007
33. Peters CA. Complications in pediatric urological laparoscopy: results of a survey. J Urol. (1996) 155:1070–3. doi: 10.1016/S0022-5347(01)66394-8
34. Passerotti CC, Nguyen HT, Retik AB, Peters CA. Patterns and predictors of laparoscopic complications in pediatric urology: the role of ongoing surgical volume and access techniques. J Urol. (2008) 180:681–5. doi: 10.1016/j.juro.2008.04.042
35. Tennankore KK, Kim SJ, Alwayn IPJ, Kiberd BA. Prolonged warm ischemia time is associated with graft failure and mortality after kidney transplantation. Kidney Int. (2016) 89:648–58. doi: 10.1016/j.kint.2015.09.002
36. Tedesco G, Faggiano FC, Leo E, Derrico P, Ritrovato M. A comparative cost analysis of robotic-assisted surgery versus laparoscopic surgery and open surgery: the necessity of investing knowledgeably. Surg Endosc. (2016) 30:5044–51. doi: 10.1007/s00464-016-4852-7
37. Blinman T. Incisions do not simply sum. Surg Endosc. (2010) 24:1746–51. doi: 10.1007/s00464-009-0854-z
38. Barbosa JABA, Barayan G, Gridley CM, Sanchez DCJ, Passerotti CC, Houck CS, et al. Parent and patient perceptions of robotic vs open urological surgery scars in children. J Urol. (2013) 190:244–50. doi: 10.1016/j.juro.2012.12.060
39. Casale P. Laparoscopic and robotic approach to genitourinary anomalies in children. Urol Clin North Am. (2010) 37:279–86. doi: 10.1016/j.ucl.2010.03.005
40. Breda A, Territo A, Decaestecker K. Robotic Kidney Transplantation Course. ORSI Academy, Belgium. Available online at: https://www.orsi-online.com/en/training/rkt-w-dr-breda (accessed March 11, 2021).
41. Wagenaar S, Nederhoed JH, Hoksbergen AWJ, Bonjer HJ, Wisselink W, van Ramshorst GH. Minimally invasive, laparoscopic, and robotic-assisted techniques versus open techniques for kidney transplant recipients: a systematic review. Eur Urol. (2017) 72:205–17. doi: 10.1016/j.eururo.2017.02.020
42. Gallioli A, Territo A, Boissier R, Campi R, Vignolini G, Musquera M, et al. Learning curve in robot-assisted kidney transplantation: results from the European robotic urological society working group. Eur Urol. (2020) 78:239–47. doi: 10.1016/j.eururo.2019.12.008
43. Demyttenaere S, Feldman LS, Fried GM. Effect of pneumoperitoneum on renal perfusion and function: A systematic review. Surg Endosc Other Interventional Tech. (2007) 21:152–60. doi: 10.1007/s00464-006-0250-x
44. Warlé MC, Berkers AW, Langenhuijsen JF, van der Jagt MF, Dooper PM, Kloke HJ, et al. Low-pressure pneumoperitoneum during laparoscopic donor nephrectomy to optimize live donors' comfort. Clin Transplant. (2013) 27:E478–83. doi: 10.1111/ctr.12143
45. Aditianingsih D, Mochtar CA, Lydia A, Siregar NC, Margyaningsih NI, Madjid AS, et al. Effects of low versus standard pressure pneumoperitoneum on renal syndecan-1 shedding and VEGF receptor-2 expression in living-donor nephrectomy: a randomized controlled study. BMC Anesthesiol. (2020) 20:37. doi: 10.1186/s12871-020-0956-7
Keywords: pediatric kidney transplantation, kidney transplantation, pediatric robot-assisted kidney transplantation, robot-assisted kidney transplantation, robotics, robotic surgery, minimal-invasive surgery
Citation: Grammens J, Schechter MY, Desender L, Claeys T, Sinatti C, VandeWalle J, Vermassen F, Raes A, Vanpeteghem C, Prytula A, Silay MS, Breda A, Decaestecker K and Spinoit A-F (2021) Pediatric Challenges in Robot-Assisted Kidney Transplantation. Front. Surg. 8:649418. doi: 10.3389/fsurg.2021.649418
Received: 04 January 2021; Accepted: 01 March 2021;
Published: 25 March 2021.
Edited by:
Felix K. H. Chun, University Hospital Frankfurt, GermanyReviewed by:
Luis Alex Kluth, University Hospital Frankfurt, GermanyCopyright © 2021 Grammens, Schechter, Desender, Claeys, Sinatti, VandeWalle, Vermassen, Raes, Vanpeteghem, Prytula, Silay, Breda, Decaestecker and Spinoit. This is an open-access article distributed under the terms of the Creative Commons Attribution License (CC BY). The use, distribution or reproduction in other forums is permitted, provided the original author(s) and the copyright owner(s) are credited and that the original publication in this journal is cited, in accordance with accepted academic practice. No use, distribution or reproduction is permitted which does not comply with these terms.
*Correspondence: Anne-Françoise Spinoit, YWZzcGlub2l0QGhvdG1haWwuY29t
Disclaimer: All claims expressed in this article are solely those of the authors and do not necessarily represent those of their affiliated organizations, or those of the publisher, the editors and the reviewers. Any product that may be evaluated in this article or claim that may be made by its manufacturer is not guaranteed or endorsed by the publisher.
Research integrity at Frontiers
Learn more about the work of our research integrity team to safeguard the quality of each article we publish.