- 1Orthopedics Department, Second Hospital of Shanxi Medical University, Taiyuan, China
- 2Department of Computer & Information Technology, Shanxi University, Taiyuan, China
Purpose: To find out the most appropriate management scheme through the analysis and comparison of different inactivation methods and filling materials.
Method: A systematic literature search was performed using the terms, anhydrous ethanol, phenol, hypertonic saline, cryotherapy, thermal therapy, bone reconstruction, GCTB, and etc., Selected articles were studied and summarized. The mechanism, clinical effects, and influence on bone repair of various methods are presented. Recent developments and perspectives are also demonstrated.
Recent Findings: Compared to curettage alone, management of the residual cavity can effectively reduce the recurrence of giant cell tumours of bone. It is a complex and multidisciplinary process that includes three steps: local control, cavity filling, and osteogenic induction. In terms of local control, High-speed burring can enlarge the area of curettage but may cause the spread and planting of tumour tissues. Among the inactivation methods, Anhydrous ethanol, and hyperthermia therapy are relatively safe and efficient. The combination of the two may achieve a better inactivation effect. When inactivating the cavity, we need to adjust the approach according to the invasion of the tumour. Filling materials and bone repair should also be considered in management.
Introduction
Giant cell tumour of bone (GCTB) is one of the most common primary bone tumours, and its incidence in China is as high as 14.9%. Treatment of this tumour has been controversial in recent years because GCTB is more aggressive than normal benign tumours. However, extensive intralesional curettage is still the most commonly accepted procedure. This surgical method can not only ensure postoperative function but also reduce the recurrence and metastasis rate to a certain extent. This method is also widely used for other benign lesions that are prone to relapse and malignant transformation, such as enchondroma, aneurysmal bone cyst, and chondromyxoid fibroma. Operationally, the surgeon should first open a bone window sufficiently large to manage the tumour and residual cavity under direct vision and then perform curettage, inactivation, and reconstruction (Figure 1). During these steps, management of the residual cavity is as important as complete curettage to reduce postoperative recurrence. Studies have shown that the recurrence rate after simple curettage is relatively high, ranging from 25 to 50% (1–6). Curettage combined with residual cavity management can effectively reduce the recurrence rate (1, 7, 8). Currently, there are three vital pillars for management of the tumour cavity (Figure 2):
1. Local control. Local tumour control and extensive clearance are crucial for decreasing the existing microlesions and avoiding further invasion and recurrence.
2. Cavity filling. Cavity filling and hard material implants are effective in promoting early functional recovery and mechanical stability.
3. Osteogenic induction. Inhibiting fibrous proliferation and promoting osteogenic differentiation are major steps in tumour cavity management to accelerate self-reconstruction and incorporation of filling materials.
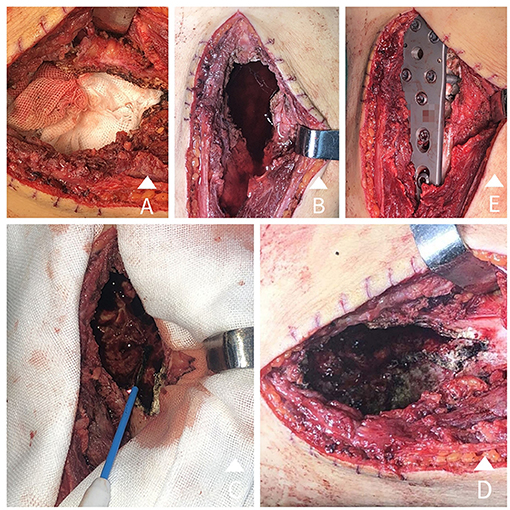
Figure 1. (A) A large incision, a large bone window opening, and tumour curettage are conducted. (B) Anhydrous ethanol is poured into the tumour residual cavity and set for 15 minutes. (C) The residual cavity wall is heated with electrotome thermocoagulation inch-by-inch under proper protection. (D) The residual cavity wall is obviously carbonized after heating. (E) Internal fixation is carried out using nails and steel plates after closing the bone window.
Almost all studies on the treatment of bone defects in GCTB are based on the three theories mentioned above. To date, many methods have been tested. These methods are reviewed in this article. Trends and updates regarding GCTB management are also introduced.
Methods
We searched Medline, EMBASE, and PubMed with the terms GCTB, intralesional curettage, adjuvant therapy, high-speed burring, denosumab, juxta-articular giant cell tumour of bone, bone defect, bone repair, thermoablation, cryotherapy, phenol, ethanol, and bone graft. After a cursory review of more than 200 studies, we read 100 of them thoroughly. The inclusion criteria were as follows: (1) literature published mainly between 2005 and 2020; (2) all clinical retrospective studies of curettage combined with adjuvant therapy for the treatment of GCTB; (3) all reviews of methods on residual cavity inactivation; (4) literature on the repair of residual bone defects after adjuvant therapy; and (5) basic studies on tolerance of bone repair-related factors in a special environment. The following articles were excluded: (1) articles on segmental resection and drug therapy in GCTB; (2) case reports and (3) articles on adjuvant therapy combined with radiation and chemotherapy. Majority of my information was derived mainly from articles in the last 10 years. The key focus of this review is to understand various methods of residual cavity management from various aspects. Different methods are compared, and their advantages and disadvantages are summarized. Finally, recommendations should be made for clinicians to treat GCTB more effectively. Majority of my information was dervied mainly from articles in the last 10 years.
Review and Findings
Tumour Cell Elimination
Sufficient curettage and a second elimination using adjuvant agents or physiotherapy are needed in GCTB. There are data indicating that when local adjuvants are not utilized, the mean recurrence rate is ~42% (21–65%) (9–16). In contrast, recurrence rates of less than 20% have been cited in the literature following the addition of adjuvant therapies to curettage (13, 17–19). In the following section, we describe and compare various inactivation methods from the perspectives of the principle, application, range of influence, clinical effect and influence on bone repair.
Chemical Reagents
Phenol
Phenol is an important reagent that has been used for a long time. Until approximately 2010, one of the most established standard treatments with acceptable recurrence rates was curettage with local adjuvant application of phenol and polymethyl methacrylate (PMMA) (recurrence rate, 3–33%) (12, 13, 15, 16, 20–26). Phenol is a chemical agent that induces tumour necrosis and coagulation of proteins (27, 28). The concentration of phenol for local inactivation ranges from 5 to 95%. The effects achieved by using 5% phenol are safer for patients (29, 30). Increasing the temperature does not significantly increase the therapeutic effect but does increase the absorption of phenol and lead to poisoning (31). Usage includes a direct infusion or smearing the wall of the residual cavity using a tampon or gauze. However, we think the effect of soaking is much better than that of surface coating. To achieve the ideal therapeutic effect, phenol should be applied at least 3 times. Phenol application times ranging from 1 to 6 min have been reported in different studies. Studies have shown that it takes 6 min for phenol to effectively kill GCT cells (32). The cytotoxic effect of phenol has been studied in vitro on monolayer cultures of cells from GCTB, and the infiltration depth has been estimated at 0.2 mm (33). The in vivo effect was studied in fresh animal cadavers (34), and in 90% of cases, phenol caused a mean 566-μm-wide zone of devitalization of bone marrow cells. To date, there have been no direct in vivo experiments in humans. Therefore, the real infiltration depth and specific reaction mechanism are still unknown, especially for different locations. We hypothesize that tissues adjacent to the articular surface, bone cortex, and bone cancellous may respond differently to certain chemical or physical stimuli. However, further research is needed.
There are some articles introducing the beneficial and adverse effects of phenol. Low recurrence rates have been recorded (12, 14, 15, 21), but complications have been less frequently mentioned. Only two articles mentioned complications such as chemical burns and damage to the neurovascular structures and soft tissues nearby (8, 35). It has been suggested that phenol is potentially carcinogenic to other organs after absorption. Thus, after inactivation, the cavity should be thoroughly washed with normal saline to avoid heart, liver, or kidney failure caused by excessive phenol absorption (30, 36). Although, the common use of phenol in practice has guaranteed safety to a certain extent, the other effects on normal tissues with long-term follow-up still require exploration.
Anhydrous Ethanol
Anhydrous ethanol (AE) is widely used in microvascular embolization of tumours, tissue fixation, and bacterial killing. The inactivation effect is caused mainly by destruction of the osmotic balance on the cell membrane, which results in dehydration and denaturation of intracellular proteins, lysing of lipids, and finally pyknosis of the nucleus and cytoplasm (37). In the last decade, AE has been widely used for inactivation of the cavity after the removal of GCTB. Compared with phenol, it has superior safety. The usage is similar to that of phenol (Figure 1B). It is generally believed that a better inactivation effect can be achieved by ethanol soaking in the scraped cavity for more than 15 min. However, researchers also found that there were slight differences between different tumour specimens. Considering the particularity of bone tissues (the strong wrapping effect of bone minerals), the inactivation effect is expected to be worse than that of other tumours. Therefore, AE needs to remain in the residual cavity longer to ensure the inactivation effect. This condition can be more easily achieved by soaking.
Oh (38) reported that four (9.5%) patients who had received anhydrous alcohol treatment developed local recurrence, whereas, 15 (48.4%) out of 31 patients who had not received any adjuvant treatment developed recurrence. Lin et al. (35) et al. conducted a prospective study on the inactivation effect of phenol and AE. The results showed that the recurrence rates of the two groups were 12 and 11% within an average follow-up period of 58 months. Short-term complications after the use of AE have not been reported. However, the team of Farah Sharieh, in recent years, indicated that AE inhibits mesenchymal stem cell (MSC) osteochondral lineage differentiation through the activation of forkhead box protein O-specific signalling (39). Thus, we hypothesized that, in the long term, this inactivation method may affect bone repair and osteocompatibility by inhibiting the osteogenetic differentiation or other unknown mechanisms in osteoblast cells. This area of research requires further in-depth mechanistic and long-term clinical prospective studies, which may influence clinicians' choice.
Hypertonic Saline
HS has been used to inactivate the residual cavity of GCTB (31). The main mechanism is the formation of osmotic pressure differences inside and outside the cells, which leads to their dehydration. However, due to the particularity of bone tissues, the inactivation range, and cell death rate associated with this method remains to be evaluated. Studies have shown that 20% HS has a positive effect on cell inactivation. Moreover, increasing the temperature or time could significantly increase the inactivation rate. The best inactivation effect could be achieved by increasing the time to more than 30 min. However, the most commonly used treatment in practice is 10% HS for 20 min. Whether the difference in concentration has an effect on the inactivation rate is unknown.
Because of the small number of cases, the exact inactivation effect of HS has not been confirmed. The complications of HS and the effect on bone repair have not been reported. Karjalainen et al. (40) concluded that chondrocytes could tolerate rather high differences in osmolarity, especially in the presence of 20 mM taurine in the cell culture medium, which may partly explain why joint complications were rarely reported and suggest a minor effect on bone repair.
Physical Inactivation
Compared with chemical reagents, physical inactivation has a greater impact on cells and can theoretically achieve a thorough tumour-killing effect. As a result, it is more commonly used in practice. At present, the most commonly used inactivation methods are high-speed burring, thermal therapy and cryotherapy.
High-Speed Burring
In 2002, Ghert et al. (22) first proposed the surgical procedure of high-speed burring (HSB) combined with other treatments after curettage. This idea has been supported by the majority of clinicians. When we remove tumour tissue intraoperatively, the wall of the residual cavity is not actually smooth and is filled with bumpy bone ridges. Therefore, it is difficult to remove tissue between the bone ridges by curettage alone. HSB has a strong cutting effect on bone tissue, which can easily erase the bone ridges to achieve complete removal of tumour tissue and even expand the surgical area. Depths for HSB use have been defined [~1 mm in the normal cortex and 5 mm in the normal cancellous or subchondral bone (41)]. However, it is almost impossible to accurately measure the depth of burring in a surgical procedure. Surgeons can only decide when to end burring according to their own subjective feelings, which may be the reason for the mixed results. Some researchers have argued that the role of HSB in reducing postoperative recurrence is critical (42), while others argue that HSB use after thorough curettage has no effect (2). In contrast, sputtering of fine particles during the use of HSB may lead to the dissemination and implantation of tumour cells (43). However, such a conclusion remains to be confirmed by a large number of studies. Based on a large number of cases, no clinicians have observed that HSB use will increase the possibility of recurrence and invasion, while the convenience to surgeons is obvious. Most researchers contend that HSB alone cannot play a definite role in the reduction of tumour cells and needs to be combined with other inactivation methods (44). Although, there is no literature regarding the effect of HSB on local bone repair, we argue that the mere removal of tissues has very little effect.
Thermal Therapy
Thermal cauterization refers to a technique that kills tumour cells by direct or indirect heat shock applied to the wall of the tumour cavity. In recent years, many methods have emerged, including electrotome thermocoagulation (ET) (Figures 1C,D), argon beam coagulation (ABC), high-temperature microwave treatment (HMT), and radiofrequency ablation (RA). Ofluoglu (45) burned the residual cavity with a 120 W ABC combined with 90% phenol inactivation treatment. One case (4%) showed recurrence postoperatively. Lewis et al. (46) burned the residual cavity of 37 cases with an ABC until obvious charring appeared. The power was set at 100 W, and the recurrence rate was 11%. Benevenia et al. (47) reported 93 cases of stage II and III lesions from 1992 to 2007 in which the residual cavity of 33 cases was inactivated with ABC, and the recurrence rate was 15%. Complications, such as postoperative fracture, physeal arrests, synovitis, bursitis, and joint instability, were observed.
Different technologies rely on different heat production mechanisms. ET produces heat mainly by high-frequency and high-voltage current at the electrode tip. ABC can continuously transmit the coagulation current to the surroundings of the electric tip, producing a better thermal effect. RA produces a resistance electrothermal effect in tissues through electrode catheters. Microwave energy is generated by the rotation and continuously accelerated collision of dipoles in the microwave field. RA is easily affected by the concentration of free ions and the electrical conductivity. The expansion of the solidification range relies mainly on heat conduction and dissipation. Therefore, its application is limited due to the low water content and poor thermal conductivity of bone tissue. It is often applied to liver, kidney, and other soft tissue tumours. For microwave energy, the dipoles in the magnetic field are all heat sources. The concentration of free ions and conductivity have little influence. Thus, the surrounding tissues can acquire higher thermal effects. RA and HMT have scope effects rather than point effects (Figure 3). If we cannot control the high temperature range, damage to surrounding tissues and other serious complications may occur. As a result, these two methods are less commonly used. Regardless of the mode of heat production, the fundamental purpose of heat burning is to cause proteins inside and outside the cell to coagulate and denature, leading to rapid tissue necrosis. In that way, what degree of heat can cause necrosis of bone tumour tissue?
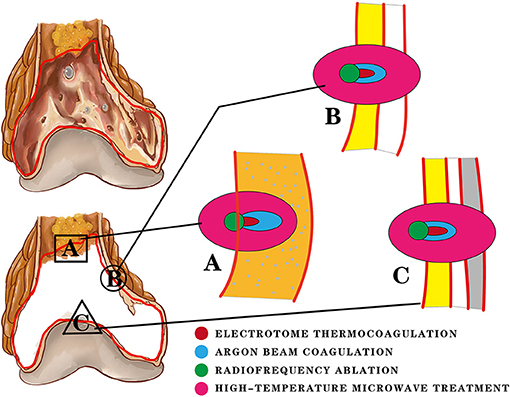
Figure 3. Three locations of the residual cavity after curettage are treated with four thermal therapy methods. The difference in the inactivation range is shown in the figure. (A) The orange area represents cancellous bone and bone marrow. Electrotome thermocoagulation (ET) and argon beam coagulation (ABC) are used to burn the tissues at and below their contact point, producing a certain depth of inactivation (red). ABC provides a continuous current and has a deeper distance (blue) of inactivation than ET. Radiofrequency ablation (RA) and high-temperature microwave treatment (HMT) both have scope effects. RA depends on heat conduction and moisture, which results in a small inactivation range (green). Most of the heat is concentrated in the residual cavity. HMT is not limited by moisture and heat conduction, so it has a large inactivation range. (B) The yellow area represents the residual cancellous bone; the white area represents the bone cortex. Due to the poor thermal conductivity and low water content of the bone cortex, the inactivation distance of ET is usually limited within the cancellous bone and cannot break through the bone cortex. RA produces only a small inactivation range. The inactivation distance of ABC can break through the cortex slightly. HMT is not limited by the cortex and can cause a wide range of inactivation, causing damage to extra-skeletal tissues. (C) The gray area represents the cartilaginous surface of the joint. ET and RA have no effect on the bone cortex and cartilage surface based on the above description. The thermal effect of ABC is limited to the cortex. However, the unrestricted inactivation range of HMT is likely to cause irreversible articular surface damage and some joint complications.
Moritz and Henriques proposed the principle that high temperatures should be used for a short period and low temperatures for a long period. However, no specific temperature was proposed. In 1986, Nelson et al. (48) reported that the temperature and time of osteonecrosis were 50°C for 6 min. Subsequently, studies on the correlation between heat conduction and heat apoptosis were conducted. Heck et al. (49) used ABC at 50, 100, and 150 to cauterize the cancellous bone of pigs and achieved necrosis depths of 1.0 ± 0.5 mm, 2.9 ± 1.0 mm, and 4.2 ± 0.7 mm, respectively, indicating that the necrosis depth would increase correspondingly with increased power. However, there was a study in China proposing that although, the heat and radiation distance were proportional to the time and power, the inactivation distance tended to be stable. Thus, we hypothesized that inactivated tissues that have undergone rapid coagulation under ultrahigh temperature may form a strong “protective shield,” thus preventing further damage to other cells. Phimolsarnti et al. (50) conducted an in vitro study on the temperature and apoptosis rate in 2012. The results were consistent with the findings of Li Leibo. The apoptosis rate of cells was proportional to the temperature and time. However, when the temperature was set to 50°C, the apoptosis rate did not increase with increasing time but tended to be stable or even decreased. Moreover, Rapin also found that tumour cells could maintain their growth and survival better than normal cells. However, after thermoablation between 47 and 50°C for periods of 20 and 30 min, chondrocytes and osteoblasts had lower apoptosis rates than giant cell tumours, which is also the current concern of surgeons regarding which temperature can ensure effective tumour killing and activity of normal tissues simultaneously. Rapin proposed that 47°C for 20 to 30 min is the most suitable choice for GCTB. However, many questions remain concerning this temperature because the temperature used in the clinic is much higher. The heat used in practice may cause serious damage to normal tissues during tumour killing.
Subsequently, local bone repair becomes important after injury. Bone morphogenetic protein (BMP), vascular endothelial growth factor (VEGF), transforming growth factor B (TGF2B), and other factors participate in repair. What about the heat tolerance of these factors? Most attention has been paid to BMP, which has strong heat resistance. Within a certain temperature range, its activity gradually increases with increasing temperature. After heat treatment at 70°C for 30 min, its activity reaches a maximum. Then, as the temperature increases, the activity gradually decreases. Bone induction activity still occurs after heating at 170°C for 10 min and 140°C for 30 min (51). Lucas et al. (52) studied a chemokine with a molecular weight between 50,000 and 90,000. The role of this factor is to guide the movement of MSCs, which eventually differentiate into osteoblasts and osteoclasts. The results showed that the heat resistance of this factor was strong. It could be inactivated by heating at 100°C for 10 min. It was suggested that 50°C could be used as a dividing line in terms of the activity change of biologically active factors. When the temperature reached 50°C, the antigenicity began to weaken and then gradually disappeared above 70°C (such as alkaline phosphatase). These studies suggest that bone-induced activity still exists under acute thermal stress, but it depends on temperature and time to maintain good activity (53). In summary, 50–65°C for 30 min can achieve an inactivation effect and confirm bone repair activity. The temperature in practice is often too high (>100°C), and local bone repair is likely to be inhibited to varying degrees. Some researchers have mentioned this complication after inactivation with ABC. Bone repair usually begins immediately when bone defects form. However, reconstruction of the local blood supply often requires a longer time. Only by one year did the inactivated segments recover close to the normal lever. Inhibition of VEGF and its products by hyperthermia (54) may explain the long vascular remodelling period. These observations also indicate that the initial bone repair of the residual cavity often involves reactive hyperplasia and crawling replacement of surrounding bone tissues. Reconstruction of the local osteogenic microenvironment often takes more than one year. It is advised that patients undergo longer periods of internal fixation and proper immobilization to match the lengthy process. There have been many studies on the effects of hyperthermia on the peripheral blood supply. The results showed that a prolonged heating time and increased temperature can lead to the stasis of vessels (55–59). However, in practice, the bone cortex blocks most of the heat due to its poor thermal conductivity. With proper protection, hyperthermia is less likely to cause complications of peripheral vessels.
At present, the application of heat inactivation in the field of bone tumours is becoming increasingly frequent. This method can not only reliably reduce the recurrence rate but also ensure fewer clinical complications. However, many questions remain regarding the choice of temperature, time and power. More attention and in vitro and in vivo experiments are needed.
Cryotherapy
After hyperthermia was found to be useful for inactivation, the destructive effect of hypothermia on cells was considered. The earliest hypothermia therapy can be traced back to the 19th century. With the development and utilization of resources, the temperature that could be achieved gradually decreased until the appearance of liquid nitrogen, which marks the beginning of modern cryotherapy and offers hypothermia at −196°C (60, 61). The mechanism of cell disintegration caused by hypothermia is complex, including direct, and indirect damage. Among them, direct damage includes two main damage processes: quick freezing and slow melting (Figure 4). Indirect damage involves circulation failure, immune clearance, and apoptosis (62–65) (Figure 5). Compared with healthy cells, tumour cells have greater and variable resistance to cold (66). Therefore, the freezing speed, application time, and frequency of freeze-thaw cycles usually increase to achieve a better tumour-killing effect (67). At present, −50 to 70°C is considered an effective temperature (68). The use of at least two freeze-thaw cycles with fast freezing and slow thawing is particularly effective (69).
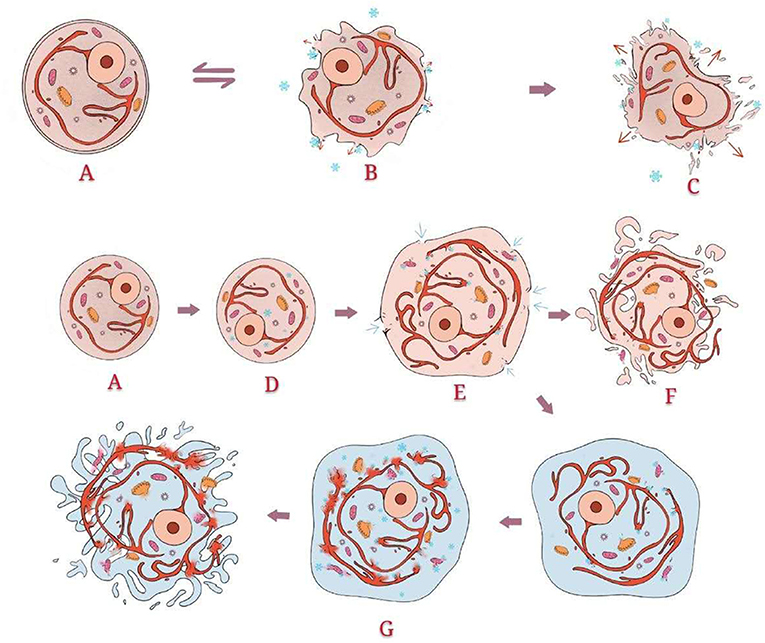
Figure 4. Direct damage: Normal structure of the cell. When the temperature drops slowly and does not reach −40°C, the effect of hypothermia occurs mainly outside the cells. Ice crystals form outside the cells, which directly destroys the cell membrane and changes the osmotic pressure. Intracellular fluid flows to the extracellular area along the osmotic pressure gradient, leading to shrinkage and even death of the cells. However, this process is partially reversible. When the temperature drops sharply and reaches below −40°C, ice crystals form inside the cells, directly destroying the internal organelles. Simultaneously, extracellular fluid flows to the intracellular area, resulting in cell swelling. Through thawing, the swelling of cells becomes severe. Some cells split into fragments. The swelling cells without splitting enter the second freeze-thaw cycle. As the present intracellular fluid is more abundant than that in the first cycle, more ice crystals form. The cells finally split due to the greater osmotic pressure difference.
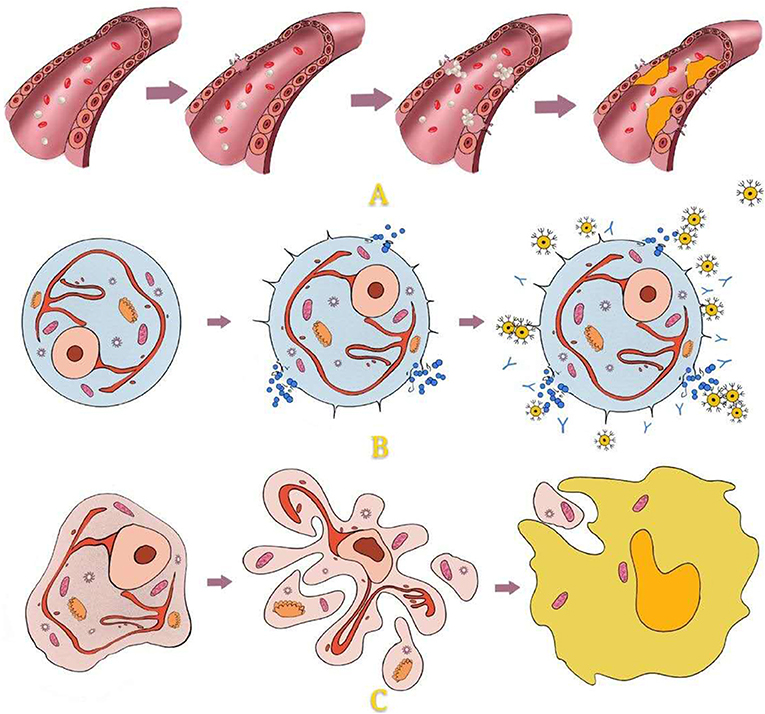
Figure 5. Indirect damage: For peripheral areas that are not in direct contact with hypothermia, temperature cannot cause direct cell disruption. This kind of cell damage is caused mainly by injury to vascular endothelial cells, immune responses, and apoptosis. Damage to vascular endothelial cells leads to the aggregation of platelets, thrombus formation, stasis of blood, and local ischaemia, finally causing cell damage. Hypothermia causes membrane damage and then antigen exposure, which causes the production and accumulation of antibodies and cytotoxic T cells. Hypothermia activates the expression of apoptosis genes (proteocleavage-activated caspases 3, 8, and 9, Bax, Bcl2, etc.), which leads to the release of enzymes and the formation of apoptotic bodies. Apoptotic bodies are internalized by phagocytes.
Marcove et al. (70–72), Marcove and Miller (73) first applied cryotherapy to bone lesions to relieve pain. Cryotherapy is widely recognized as having a significant effect on pain relief (74–76). In 1978, Marcove (77) treated 52 patients with GCTs by large incision, full reveal, thorough curettage, and repeated freezing to −20°C. The overall recurrence rate was 23%, which is lower than that of simple curettage [40–55% (78, 79)]. This process provided an effective tumour clearance method for surgeons. Subsequent reports have shown that curettage combined with cryotherapy can significantly reduce the recurrence rate (68, 80–82). Concurrently, many complications have also been reported, including postoperative fracture, skin necrosis, transient nerve palsy, infection, traumatic arthritis, tumour malignancy, and bone graft non-union. The incidence has ranged from 12 to 50% (83, 84). Moreover, individual cases showed that cryotherapy resulted in systemic cytokine-mediated cryo-shock syndrome with hypotension, dyspnoea and disseminated intravasal coagulation (85). Various studies have also shown that the safety of cryotherapy is not guaranteed.
Rapid freezing and slow thawing for 3 cycles has been reported to increase the surgical boundary to 2 cm (9, 77). The necrotic distance increased correspondingly with the decrease in temperature (86). Unlike the extensive damage caused by hyperthermia, the inactivation rate of cryotherapy is relatively low. Cells in direct contact often suffer acute damage, while others depend on delayed immune responses and apoptotic mechanisms. The necrosis of the microarterial system after cryotherapy is often difficult to reverse. However, the large vessels and nerves are generally not damaged. Even if they are damaged, recovery commonly occurs within 24 h (86–88), which ensures the basic safety and wide application of cryotherapy. In the initial stage of cryotherapy, direct infusion using liquid nitrogen was the most commonly used technique. However, it greatly increased the incidence of complications. Later, with the continuous improvement of technology, the plug-in type, pressor spray type, and a modern argon-helium knife were applied. An argon-helium knife uses argon for freezing and helium for thawing. Although, the tumour-killing effect is excellent, it is rarely used in practice due to its high price (7). In cryotherapy, temperature control and detection systems are also important. Researchers have conducted numerous studies in this area (89–93), which are not further discussed herein.
For osseous lesions, cryotherapy offers some unique advantages over other adjuvant treatments since it will kill cells but leave the inorganic osseous framework intact, which can remain as a matrix to achieve better bone healing (82). Generally, bone healing is achieved 3 months after cryotherapy, which marks the beginning of weight bearing for patients (94). At present, there is a lack of research examining the effects of 68 temperatures (below −50°C) on the activity of osteogenesis-related cytokines (BMP, VEGF, etc.,) and the expression of osteogenic genes (Runx2, OSX, etc.,). Therefore, information about bone repair of the residual cavity after cryotherapy remains sparse.
The combined application of cryotherapy has recently become a trend. Because liquid nitrogen is difficult to control, researchers created a semisolid cryogen, a freezing nitrogen ethanol composite, which achieved good security, and a low recurrence rate. The freshly prepared freezing nitrogen ethanol composite was frozen to −136°C. When it was applied in the cavity, an isotherm of −122°C across a piece of 10 ± 0.50-mm-thick bone was achieved after several minutes (95). The combination of cryotherapy and immunoregulatory drugs has also been reported. In a mouse model of melanomas, it was shown that the combination of cryotherapy and CpG-B oligodeoxynucleotides resulted in significantly more effective tumour control than monotherapy (96), providing us with new ideas for the treatment of GCTB. The combination of cryotherapy with immunomodulators may also be effective in reducing the recurrence and metastasis of GCTB. However, there are many kinds of immunomodulators with obvious side effects in the clinic. To achieve a reliable conclusion, the pros and cons of many studies must be evaluated.
Cavity Filling and Osteogenic Induction
After completion of tumour cell reduction, cavity filling, and osteogenic induction are also necessary. The filling materials of the residual cavity include autogenous bone, allograft bone (AB), and synthetic bone substitute materials (SBSMs). Reconstruction using autogenous bone is usually performed with a segment of the fibular or iliac wing. However, due to the irregularity of the residual cavity and the fixed shape of the autogenous bone, it is often difficult to achieve complete filling and good mechanical results using autogenous bone alone. Thus, AB and SBSM are widely used. AB is excellent as an implant material. Its structure and properties are completely consistent with the bone tissues surrounding the cavity. Pogrel et al. (86) assessed the influence of bone grafts on bone healing in the mandible of minipigs and showed that the group with bone grafts achieved a better clinical healing effect while that without bone grafts showed a 50% rate of wound breakdown and sequestrum formation with delayed healing. However, bone grafting does not appear to increase the bone density or rate of bone formation in defects at three months postoperatively, which suggests a minimal effect of bone grafts on bone repair and a long duration of the bone repair procedure. When AB is used as the only implant, it cannot provide sufficient local mechanical strength in the early stage. With the continuous development of materials, an increasing number of SBSMs have been applied, including apatite-wollastonite-containing glass ceramics (AWGCs), hydroxyapatite (HA), tricalcium phosphate (TCP), and PMMA. The ideal SBSM should have good biocompatibility and biodegradability. Simultaneously, it should promote the formation of new bone tissues. Excluding PMMA, the other three materials are all biocompatible, bioactive, and osteoconductive (97–100). The unique point of PMMA is its good mechanical strength and deformability. It has also been reported to reduce local recurrence due to its cytotoxic effect and heat product ability (22, 101, 102). However, its disadvantages are also obvious. Due to the difference between PMMA and surrounding tissues in nature, stress-induced loosening (103), peripheral fractures, accelerated loss of articular cartilage, and displacement of the bone cement block are common complications. Therefore, internal fixation is advised (104) when using PMMA as the filling material. TCP is a bone substitute material that is widely studied and applied worldwide. It has ideal biocompatibility and biodegradability because of its similar physicochemical properties to bone tissues. However, TCP is granular and difficult to shape. It is often necessary to combine with other biological excipients (such as cross-linked gelatine) to achieve a better adhesion effect.
At present, the application of composite materials has become a trend. The combination of different inorganic materials, inorganic and organic, inorganic materials, and bone induction factors as well as organic materials and bone induction factors has gradually appeared. Kotrych et al. (105) reported that the application of Highly Injectable Bi-Phasic Bone Substitute (CERAMENT) achieved good clinical effects. Hattori et al. (106) found that HA/TCP was more bioresorbable and osteoconductive than HA or AWGC. Shin et al. (107) presented successful reconstruction with autogenous bone grafts and autologous bone marrow mesenchymal stem cells. Other composites have also been described, including PL GA capsules containing RHBMP-2 (108) and poly(DL-lactic-co-glycolic acid)/calcium-phosphate cement composites (109). In the next phase, the selection and production of bioactive substances will be more systematic and efficient, and the proportion of biomaterials in composites will be more specialized. Accompanied by the progress of the controlled-release system, the promotion of local bone repair and bone fusion by filling materials will become a kind of periodic treatment.
Discussion
We listed the postoperative recurrence rates and complications caused by different management strategies for the residual cavities of GCTB in the last ten years (12, 16, 20, 35, 47, 104, 110–116) (Table 1). The recurrence rates and incidences of complications vary between different treatments. Even when using the same method, they can also be different, possibly because the application processes are different. In general, a definite pattern or rule is lacking in the management of the residual cavity to guide the choice of surgeons and ensure good clinical results. We summarize the characteristics of each method (Table 2) and propose some of our own opinions. For HS and AE, we believe that the inactivation effect of soaking is far superior to that of smearing. For phenol, smearing is relatively safe due to its high biological toxicity. The onset time and frequency should be guaranteed. When inactivating the residual cavity by hyperthermia, the wall should be burnt inch by inch to ensure that every corner is covered. We do not recommend using an exceedingly high power over a long time. The total cauterization time should be less than 15 min. Direct dumping of liquid nitrogen in cryotherapy is an approach with which we strongly disagree. Use of the Plug-in Type and the Pressor Spray Type can better ensure safety.
In comparisons of phenol and ethanol, phenol, and ABC, and phenol and liquid nitrogen (Table 1), several researchers concluded that there was no difference in postoperative recurrence. Suggestions for surgeons were based on the incidence of complications. Accordingly, phenol and liquid nitrogen are not recommended. The inactivation effect of HS is weak, and it requires a long time to have an effect. Although, its safety is extremely high compared with phenol and ethanol, we do not generally recommend the use of HS for residual cavity treatment. Ethanol is similar to phenol in all aspects but is much safer. Thus, we recommend the use of ethanol for inactivation. Hyperthermia is a safe and efficient inactivation method. Among approaches, ET and ABC are recommended for use. However, as we stated previously, excessive temperatures are not recommended. Complications following high-power ABC inactivation have been reported. Therefore, we recommend the use of ET or low-power ABC for treatment. We also propose a scenario in which the combination of ethanol and hyperthermia can achieve better results. We believe that after ethanol immersion and washing, a low concentration of ethanol will remain in the tissues. At this point, when we conduct hyperthermia, deeper depths of inactivation may be achieved without increasing the temperature. This idea has not been confirmed, but it could open up a new avenue for surgeons. Of course, complete removal of the tumour is of the utmost importance before any inactivation. When this cannot be ensured with curettes alone, use of HSB is also particularly important. PMMA is recommended by most researchers as the filling material. Re-inactivation of PMMA is beneficial for management of the residual cavity, but complications are also possible. We believe that allograft implantation is also a good option. Patients with bone grafts have less discomfort and fewer complications after surgery. With adequate internal fixation, mechanical strength during the recovery period can also be partially assured. Moreover, after combination with other osteo-inductive materials, composite bone grafts are the best choice.
The main foothold of this review is the implementation of extended curettage through various inactivation methods, which were described and compared in detail. However, most of the conclusions we have mentioned above are not feasible for GCTB that has penetrated the articular surface and surrounding soft tissues. For extra-articular and cortical GCTB, we should first evaluate the possibility of curettage. When articular surface destruction exceeds 50% or large areas of soft tissues are affected, intralesional curettage is not recommended (117). At this point, we should choose segmental resection to ensure low postoperative recurrence. For GCTB with a small range of invasion to the articular surface and soft tissues or with pathological fracture, we should pay attention to the following points when we choose to perform curettage. The use of chemical reagents can only involve smearing rather than soaking. Soaking may lead to leakage of fluid into the joint cavity and surrounding tissues, which will increase the incidence of complications. Use of liquid nitrogen is not permitted. Hyperthermia is still an alternative method. However, it should be noted that the power and time when used at juxta-articular points should be appropriately reduced to avoid large-scale destruction of articular chondrocytes and synovial tissues. In general, the effect of tumour reduction under these conditions was greatly reduced, and the possibility of recurrence increased. Moreover, filling materials may also have a certain effect on joint function. Therefore, for these special GCTBs, the choice of surgery should be carefully decided after communication with patients.
In 2013, a humanized monoclonal antibody against the receptor activator of nuclear factor-κB-ligand (RANKL), denosumab, was approved for the treatment of advanced GCTB. Because this review does not focus on drug management, we do not elaborate on the mechanism of denosumab. At present, various disadvantages of denosumab have been reported by researchers (118, 119). Therefore, we do not support the use of denosumab in combination with various residual cavity management strategies. Advanced technology has rarely been applied in this field, including nanocryotherapy (120), controlled and sustained release systems, microsphere carriers, and some new osteo-induction and reconstruction materials. The addition of these new technologies will achieve accurate tumour-killing effects with reduced loss of normal tissues. Moreover, the promotion of bone repair will also become an important part of adjuvant therapy.
Conclusion
Compared to curettage alone, management of the residual cavity can effectively reduce the recurrence of giant cell tumours of bone. It is a complex and multidisciplinary process that includes three steps: local control, cavity filling, and osteogenic induction. In terms of local control, HSB can enlarge the area of curettage but may cause the spread and planting of tumour tissues. Among the inactivation methods, AE and hyperthermia therapy are relatively safe and efficient. The combination of the two may achieve a better inactivation effect. When inactivating the cavity, we need to adjust the approach according to the invasion of the tumour. Filling materials and bone repair should also be considered in management.
Author Contributions
YW conceived and wrote most of the manuscript. CW made the figures and tables. QT and JL collected the related data. HL provided partial financial support. YF thoroughly revised and amended the manuscript. All authors contributed to the article and approved the submitted version.
Funding
This work was supported by Applied Basic Research of Shanxi Province (201901D111373).
Conflict of Interest
The authors declare that the research was conducted in the absence of any commercial or financial relationships that could be construed as a potential conflict of interest.
Publisher's Note
All claims expressed in this article are solely those of the authors and do not necessarily represent those of their affiliated organizations, or those of the publisher, the editors and the reviewers. Any product that may be evaluated in this article, or claim that may be made by its manufacturer, is not guaranteed or endorsed by the publisher.
References
1. Szendröi M. Giant-cell tumour of bone. J Bone Joint Surg Br. (2004) 86:5–12. doi: 10.1302/0301-620X.86B1.14053
2. Algawahmed H, Robert T, Farrokhyar F, Ghert M. High-speed burring with and without the use of surgical adjuvants in the intralesional management of giant cell tumor of bone: a systematic review and meta-analysis. Sarcoma. (2010) 2010:586090. doi: 10.1155/2010/586090
3. Shih HN, Hsu RW, Sim FH. Excision curettage and allografting of giant cell tumor. World J Surg. (1998) 22:432–7. doi: 10.1007/s002689900411
4. Trieb K, Bitzan P, Dominkus M, Kotz R. Giant-cell tumors of long bones. J Bone Joint Surg Am. (2000) 82:1360–1. doi: 10.2106/00004623-200009000-00026
5. Karpik M. Giant cell tumor (tumor gigantocellularis, osteoclastoma) - epidemiology, diagnosis, treatment. Ortop Traumatol Rehabil. (2010) 12:207–15.
6. Yu Xc, Ming X, Song Rx, Fu Zh, Liu Xp. Long-term outcome of giant cell tumors of bone around the knee treated by en bloc resection of tumor and reconstruction with prosthesis. Orthop Surg. (2010) 2:211–7. doi: 10.1111/j.1757-7861.2010.00089.x
7. Turcotte Robert E. Giant cell tumor of bone. Orthop Clin North Am. (2006) 37:35–51. doi: 10.1016/j.ocl.2005.08.005
8. Blackley HR, Wunder JS, Davis AM, White LM, Kandel R, Bell RS. Treatment of giant-cell tumors of long bones with curettage and bone-grafting. JBJS. (1999) 81:811–20. doi: 10.2106/00004623-199906000-00008
9. Balke M, Schremper L, Gebert C, Ahrens H, Streitbuerger A, Koehler G, et al. Giant cell tumor of bone: treatment and outcome of 214 cases. J Cancer Res Clin Oncol. (2008) 134:969–78. doi: 10.1007/s00432-008-0370-x
10. Knochentumoren A. Local recurrence of giant cell tumor of bone after intralesional treatment with and without adjuvant therapy. JBJS. (2008) 90:1060–7. doi: 10.2106/JBJS.D.02771
11. Kivioja AH, Blomqvist C, Hietaniemi K, Trovik C, Walloe A, Bauer HC, et al. Cement is recommended in intralesional surgery of giant cell tumors: a Scandinavian Sarcoma Group study of 294 patients followed for a median time of 5 years. Acta Orthopaed. (2008) 79:86–93. doi: 10.1080/17453670710014815
12. Klenke FM, Wenger DE, Inwards CY, Rose PS, Sim FH. Giant cell tumor of bone: risk factors for recurrence. Clin Orthop Relat Res. (2011) 469:591–9. doi: 10.1007/s11999-010-1501-7
13. Capanna R, Fabbri N, Bettelli G. Curettage of giant cell tumor of bone. The effect of surgical technique and adjuvants on local recurrence rate. Chir Organi Mov. (1990) 75(Suppl. 1):206.
14. Dürr HR, Maier M, Jansson V, Baur A, Refior HJ. Phenol as an adjuvant for local control in the treatment ofgiant cell tumour of the bone. Eur J Surg Oncol. (1999) 25:610–8. doi: 10.1053/ejso.1999.0716
15. Trieb K, Bitzan P, Lang S, Dominkus M, Kotz R. Recurrence of curetted and bone-grafted giant-cell tumours with and without adjuvant phenol therapy. Eur J Surg Oncol. (2001) 27:200–2. doi: 10.1053/ejso.2000.1086
16. Gaston CL, Bhumbra R, Watanuki M, Abudu AT, Carter SR, Jeys LM, et al. Does the addition of cement improve the rate of local recurrence after curettage of giant cell tumours in bone? J Bone Joint Surg Br. (2011) 93:1665–9. doi: 10.1302/0301-620X.93B12.27663
17. Athanasian EA, Wold LE, Amadio PC. Giant cell tumors of the bones of the hand. J Hand Surg. (1997) 22:91–8. doi: 10.1016/S0363-5023(05)80187-X
18. Campanacci M, Baldini N, Boriani S, Sudanese A. Giant-cell tumor of bone. J Bone Joint Surg Am. (1987) 69:106–14. doi: 10.2106/00004623-198769010-00018
19. Rock M. Curettage of giant cell tumor of bone. Factors influencing local recurrences and metastasis. Chir Organi Mov. (1990) 75:204–5.
20. Errani C, Ruggieri P, Asenzio MAN, Toscano A, Colangeli S, Rimondi E, et al. Giant cell tumor of the extremity: a review of 349 cases from a single institution. Cancer Treat Rev. (2010) 36:1–7. doi: 10.1016/j.ctrv.2009.09.002
21. van der Heijden L, Van de Sande MA, Dijkstra PD. Soft tissue extension increases the risk of local recurrence after curettage with adjuvants for giant-cell tumor of the long bones: a retrospective study of 93 patients. Acta Orthopaed. (2012) 83:401–5. doi: 10.3109/17453674.2012.711193
22. Ghert MA, Rizzo M, Harrelson JM, Scully SP. Giant-cell tumor of the appendicular skeleton. Clin Orthop Relat Res. (2002) 400:201–10. doi: 10.1097/00003086-200207000-00025
23. Turcotte RE, Wunder JS, Isler MH, Bell RS, Schachar N, Masri BA, et al. Giant cell tumor of long bone: a Canadian Sarcoma Group study. Clin Orthopaed Relat Res. (2002) 397:248–58. doi: 10.1097/00003086-200204000-00029
24. Ward WG Sr, Li G III. Customized treatment algorithm for giant cell tumor of bone: report of a series. Clin Orthopaed Relat Res. (2002) 397:259–70. doi: 10.1097/00003086-200204000-00030
25. O'Donnell RJ, Springfield DS, Motwani HK, Ready JE, Gebhardt MC, Mankin HJ. Recurrence of giant-cell tumors of the long bones after curettage and packing with cement. J Bone Joint Surg Am. (1994) 76:1827–33. doi: 10.2106/00004623-199412000-00009
26. Saiz P, Virkus W, Piasecki P, Templeton A, Shott S, Gitelis S. Results of giant cell tumor of bone treated with intralesional excision. Clin Orthopaed Relat Res. (2004) 424:221–6. doi: 10.1097/01.blo.0000128280.59965.e3
27. McDonald DJ, Sim FH, McLeod RA, Dahlin DC. Giant-cell tumor of bone. J Bone Joint Surg Am. (1986) 68:235–42. doi: 10.2106/00004623-198668020-00009
28. Boons HW, Keijser LCM, Bart Schreuder HW, Pruszczynski M, Lemmens JAM, Veth RPH. Oncologic and functional results after treatment of giant cell tumors of bone. Arch Orthop Trauma Surg. (2002) 122:17–23. doi: 10.1007/s004020100317
29. Quint U, Vanhöfer U, Harstrick A, Müller RT. Cytotoxicity of phenol to musculoskeletal tumours. J Bone Joint Surg. (1996) 78:984–5. doi: 10.1302/0301-620X.78B6.0780984
30. Quint U, Müller RT, Müller G. Instillation in intralesional tumor excision of chondroblastoma, osteoclastoma, and enchondroma. Arch Orthopaed Trauma Surg. (1998) 117:43–6. doi: 10.1007/BF00703438
31. Li X, Guo W, Yang Y, Wei R. Surgical treatment for long bone giant cell tumor of extremity with pathologic fracture. Beijing Da Xue Xue Bao Yi Xue Ban. (2013) 45:745–51.
32. Nithyananth M, Priscilla AJ, Boopalan, Titus VTK, Lee VN. Time required for effective action of phenol against giant cell tumour cells. J Orthopaed Surg. (2014) 22:104–7. doi: 10.1177/230949901402200126
33. Mittag F, Leichtle C, Kieckbusch I, Wolburg H, Rudert M, Kluba T, et al. Cytotoxic effect and tissue penetration of phenol for adjuvant treatment of giant cell tumours. Oncol Lett. (2013) 5:1595–8. doi: 10.3892/ol.2013.1244
34. Lang S, Lack W, Brand G, Salzer-Kuntschik M. Devitalizing effects of phenol on bone marrow cells: histologic study on cadaveric animal vertebrae. Acta Orthopaed Scand. (1991) 62:383–5. doi: 10.3109/17453679108994476
35. Lin WH, Lan TY, Chen CY, Wu K, Yang RS. Similar local control between phenol-and ethanol-treated giant cell tumors of bone. Clin Orthopaed Relat Res. (2011) 469:3200–8. doi: 10.1007/s11999-011-1962-3
36. Lackman RD, Hosalkar HS, Ogilvie CM, Torbert JT, Fox EJ. Intralesional curettage for grades II and III giant cell tumors of bone. Clin Orthopaed Relat Res. (2005) 438:123–7. doi: 10.1097/01.blo.0000180051.27961.c3
37. McDowell EM, Trump BF. Histologic fixatives suitable for diagnostic light and electron microscopy. Arch Pathol Lab Med. (1976) 100:405–14.
38. Oh JH, Yoon PW, Lee SH, Cho HS, Kim WS, Kim HS. Surgical treatment of giant cell tumour of long bone with anhydrous alcohol adjuvant. Int Orthop. (2006) 30:490–4. doi: 10.1007/s00264-006-0154-3
39. Sharieh F, Eby JM, Roper PM, Callaci JJ. Ethanol inhibits mesenchymal stem cell osteochondral lineage differentiation due in part to an activation of FoxO-specific signaling. Alcohol Clin Exp Res. (2020) 44:1204–13. doi: 10.1111/acer.14337
40. Karjalainen HM, Qu C, Leskelä SS, Rilla K, Lammi MJ. Chondrocytic cells express the taurine transporter on their plasma membrane and regulate its expression under anisotonic conditions. Amino Acids. (2015) 47:561–70. doi: 10.1007/s00726-014-1888-7
41. Jamshidi K, Shirazi MR, Bagherifard A, Mirzaei A. Curettage, phenolization, and cementation in paediatric Ewing's sarcoma with a complete radiological response to neoadjuvant chemotherapy. Int Orthopaed. (2019) 43:467–73. doi: 10.1007/s00264-018-4094-5
42. Gouin F, Dumaine V. Local recurrence after curettage treatment of giant cell tumors in peripheral bones: retrospective study by the GSF-GETO (French Sarcoma and Bone Tumor Study Groups). Orthopaed Traumatol Surg Res. (2013) 99:S313–8. doi: 10.1016/j.otsr.2013.07.006
43. Wang PH, Wu CL, Chen CM, Wang JY, Wu PK, Chen WM. Adjuvant therapy by high-speed burr may cause intraoperative bone tumor seeding: an animal study. BMC Musculoskelet Disord. (2020) 21:1–7. doi: 10.1186/s12891-020-03544-3
44. Machak GN, Snetkov AI. The impact of curettage technique on local control in giant cell tumour of bone. Int Orthopaed. (2020) 45:779–89. doi: 10.1007/s00264-020-04860-y
45. Ofluoglu O. Aggressive treatment of giant cell tumour with multiple local adjuvants. Acta Orthopaed Belg. (2008) 74:831. doi: 10.1186/1471-2474-9-160
46. Lewis VO, Wei A, Mendoza T, Primus F, Peabody T, Simon MA. Argon beam coagulation as an adjuvant for local control of giant cell tumor. Clin Orthopaed Relat Res. (2007) 454:192–7. doi: 10.1097/01.blo.0000238784.98606.d4
47. Benevenia J, Patterson FR, Beebe KS, Abdelshahed MM, Uglialoro AD. Comparison of phenol and argon beam coagulation as adjuvant therapies in the treatment of stage 2 and 3 benign–aggressive bone tumors. Orthopedics. (2012) 35:e371–8. doi: 10.3928/01477447-20120222-22
48. Nelson CG, Krishnan EC, Neff JR. Consideration of physical parameters to predict thermal necrosis in acrylic cement implants at the site of giant cell tumors of bone. Med Phys. (1986) 13:462–8. doi: 10.1118/1.595852
49. Heck RK, Pope WD, Ahn JI, Smith RA, Webber BL. Histologic evaluation of the depth of necrosis produced by argon beam coagulation: implications for use as adjuvant treatment of bone tumors. J Surg Orthopaed Adv. (2009) 18:69–73.
50. Phimolsarnti R, Charoenlap C, Ariyaboonsiri B, Wongkajornsilpa A, Waikakul S. In vitro effects of thermoablation on apoptosis of giant cell tumor of bone: a preliminary report. J Med Assoc Thai. (2012) 95(Suppl. 9):S138–45.
51. Sato T, Iwata H, Takahashi M, Miura. Heat tolerance of activity toward ectopic bone formation by rabbit bone matrix protein. Ann Chir Gynaecol Suppl. (1993) 207:37–40.
52. Lucas PA, Syftestad GT, Caplan AI. Partial isolation and characterization of a chemotactic factor from adult bovine bone for mesenchymal cells. Bone. (1986) 7:365–71. doi: 10.1016/8756-3282(86)90257-7
53. Ito T, Sakano S, Sato K, Sugiura H, Iwata H, Murata Y, et al. Sensitivity of osteoinductive activity of demineralized and defatted rat femur to temperature and duration of heating. Clin Orthopaed Relat Res. (1995) 316:267–75. doi: 10.1097/00003086-199507000-00036
54. Sawaji Y, Sato T, Takeuchi A, Hirata M, Ito A. Anti-angiogenic action of hyperthermia by suppressing gene expression and production of tumour-derived vascular endothelial growth factor in vivo and in vitro. Br J Cancer. (2002) 86:1597–603. doi: 10.1038/sj.bjc.6600268
55. Bacharach SL, Libutti SK, Carrasquillo JA. Measuring tumor blood flow with H215O: practical considerations. Nucl Med Biol. (2000) 27:671–6. doi: 10.1016/S0969-8051(00)00136-0
56. Stewart FA, Denekamp J. The therapeutic advantage of combined heat and X rays on a mouse fibrosarcoma. Br J Radiol. (1978) 51:307–16. doi: 10.1259/0007-1285-51-604-307
57. Cheng HLM, Plewes DB. Tissue thermal conductivity by magnetic resonance thermometry and focused ultrasound heating. J Magn Reson Imaging. (2002) 16:598–609. doi: 10.1002/jmri.10199
58. Akyürekli D, Gerig LH, Raaphorst GP. Changes in muscle blood flow distribution during hyperthermia. Int J Hyperthermia. (1997) 13:481–96. doi: 10.3109/02656739709023547
59. Milligan AJ, Conran PB, Ropar MA, McCulloch HA, Ahuja RK, Dobelbower RR Jr. Predictions of blood flow from thermal clearance during regional hyperthermia. Int J Radiat Oncol Biol Phys. (1983) 9:1335–43. doi: 10.1016/0360-3016(83)90265-1
60. Arnott J. Practical illustrations of the remedial efficacy of a very low or anoesthetic temperature.–I. in cancer. West J Med Surg. (1851) 154:1840–55.
61. Pusey WA. The use of carbon dioxid snow in the treatment of nevi and other lesions of the skin. A preliminary report. J Am Med Assoc. (1907) 49:1354–6. doi: 10.1001/jama.1907.25320160032001h
62. Gage AA, Baust J. Mechanisms of tissue injury in cryosurgery. Cryobiology. (1998) 37:171–86. doi: 10.1006/cryo.1998.2115
63. Hoffmann NE, Bischof JC. The cryobiology of cryosurgical injury. Urology. (2002) 60:40–9. doi: 10.1016/S0090-4295(02)01683-7
64. Wen J, Duan Y, Zou Y, Nie Z, Feng H, Lugnani F, et al. Cryoablation induces necrosis and apoptosis in lung adenocarcinoma in mice. Technol Cancer Res Treat. (2007) 6:635–40. doi: 10.1177/153303460700600607
65. Han B, Bischof JC. Direct cell injury associated with eutectic crystallization during freezing. Cryobiology. (2004) 48:8–21. doi: 10.1016/j.cryobiol.2003.11.002
66. Gage AA, Baust JM, Baust JG. Experimental cryosurgery investigations in vivo. Cryobiology. (2009) 59:229–43. doi: 10.1016/j.cryobiol.2009.10.001
67. Mahnken AH, König AM, Figiel JH. Current technique application of percutaneous cryotherapy. Röfo. (2018) 190:836–46. doi: 10.1055/a-0598-5134
68. Abdelrahman M, Bassiony AA, Shalaby H, Assal MK. Cryosurgery and impaction subchondral bone graft for the treatment of giant cell tumor around the knee. HSS J. (2009) 5:123–8. doi: 10.1007/s11420-009-9125-8
69. Gage AA, Guest K, Montes M, Caruana JA, Whalen DA Jr. Effect of varying freezing and thawing rates in experimental cryosurgery. Cryobiology. (1985) 22:175–82. doi: 10.1016/0011-2240(85)90172-5
70. Marcove RC, Miller TR, Cahan WC. The treatment of primary and metastaticbone tumors by repetitive freezing. Bull N Y Acad Med. (1968) 44:532.
71. Marcove RC, Stovell PB, Huvos AG, Bullough PG. The use of cryosurgery in the treatment of low and medium grade chondrosarcoma. A preliminary report. Clin Orthopaed Relat Res. (1977) 122:147–56. doi: 10.1097/00003086-197701000-00020
72. Marcove RC, Lyden JP, Huvos AG, Bullough PB. Giant-cell tumors treated by cryosurgery: a report of twenty-five cases. JBJS. (1973) 55:1633–44. doi: 10.2106/00004623-197355080-00007
73. Marcove RC, Miller TR. Treatment of primary and metastatic bone tumors by cryosurgery. JAMA. (1969) 207:1890–4. doi: 10.1001/jama.207.10.1890
74. Tomasian A, Wallace A, Northrup B, Hillen TJ, Jennings JW. Spine cryoablation: pain palliation and local tumor control for vertebral metastases. Am J Neuroradiol. (2016) 37:189–95. doi: 10.3174/ajnr.A4521
75. Callstrom MR, Dupuy DE, Solomon SB, Beres RA, Littrup PJ, Davis KW, et al. Percutaneous image-guided cryoablation of painful metastases involving bone: multicenter trial. Cancer. (2013) 119:1033–41. doi: 10.1002/cncr.27793
76. Fan WZ, Niu LZ, Wang Y, Yao XH, Zhang YQ, Tan GS, et al. Initial experience: alleviation of pain with percutaneous CT–guided cryoablation for recurrent retroperitoneal soft-tissue sarcoma. J Vasc Intervent Radiol. (2016) 27:1798–805. doi: 10.1016/j.jvir.2016.06.034
77. Marcove RC, Weis LD, Vaghaiwalla MR, Pearson R, Huvos AG. Cryosurgery in the treatment of giant cell tumors of bone. A report of 52 consecutive cases. Cancer. (1978) 41:957–69. doi: 10.1002/1097-0142(197803)41:3<957::AID-CNCR2820410325>3.0.CO;2-Y
78. Goldenberg RR, Campbell CJ, Bonfiglio M. Giant-cell tumor of bone: an analysis of two hundred and eighteen cases. JBJS. (1970) 52:619–64. doi: 10.2106/00004623-197052040-00001
79. Johnson EW, Dahlin DC. Treatment of giant-cell tumor of bone. J Bone Joint Surg Am. (1959) 41-A:895–904.
80. Malawer MM, Bickels J, Meller I, Buch RG, Henshaw RM, Kollender Y. Cryosurgery in the treatment of giant cell tumor. A long-term followup study. Clin Orthop Relat Res. (1999) 359:176–88. doi: 10.1097/00003086-199902000-00019
81. Veth R, Schreuder B, van Beem H, Pruszczynski M, de Rooy J. Cryosurgery in aggressive, benign, and low-grade malignant bone tumors. Lancet Oncol. (2005) 6:25–34. doi: 10.1016/S1470-2045(05)70023-1
82. Rahman MA, El Masry AM, Azmy SI. Review of 16 cases of aneurysmal bone cyst in the proximal femur treated by extended curettage and cryosurgery with reconstruction using autogenous non-vascularized fibula graft. J Orthopaed Surg. (2018) 26:1–6. doi: 10.1177/2309499018783905
83. Mazur P. Cryobiology: the freezing of biological systems. Science. (1970) 168:939–949. doi: 10.1126/science.168.3934.939
84. Jacobs PA, Clemency RE Jr. The closed cryosurgical treatment of giant cell tumor. Clin Orthop Relat Res. (1985) 192:149–58. doi: 10.1097/00003086-198501000-00021
85. Seifert JK, Morris DL. World survey on the complications of hepatic and prostate cryotherapy. World J Surg. (1999) 23:109–14. doi: 10.1007/PL00013173
86. Pogrel M A, Regezi J A, Fong B, Hakim-Faal Z, Rohrer M, Tran C, et al. Effects of liquid nitrogen cryotherapy and bone grafting on artificial bone defects in minipigs: a preliminary study. Int J Oral Maxillofac Surg. (2002) 31:296–322. doi: 10.1054/ijom.2001.0210
87. Whittaker Dk. Degeneration and regeneration of nerves following cryosurgery. Br J Exp Pathol. (1974) 55:595–600.
88. Beazley RM, Bagley DH, Ketcham AS. The effect of cryosurgery on peripheral nerves. J Surg Res. (1974) 16:231–4. doi: 10.1016/0022-4804(74)90036-5
89. Irimia D, Karlsson JO. Kinetics of intracellular ice formation in one-dimensional arrays of interacting biological cells. Biophys J. (2005) 88:647–60. doi: 10.1529/biophysj.104.048355
90. Weis J, Covaciu L, Rubertsson S, Allers M, Lunderquist A, Ahlström H. Non-invasive monitoring of brain temperature during mild hypothermia. Magn Reson Imaging. (2009) 27:923–32. doi: 10.1016/j.mri.2009.01.011
91. Yan JF, Wang HW, Liu J, Deng ZS, Rao W, Xiang SH. Feasibility study on using an infrared thermometer for evaluation and administration of cryosurgery. Minim Invasive Ther Allied Technol. (2007) 16:173–80. doi: 10.1080/13645700701384074
92. Floery D, Helbich TH. MRI-Guided percutaneous biopsy of breast lesions: materials, techniques, success rates, and management in patients with suspected radiologic-pathologic mismatch. Magn Reson Imaging Clin. (2006) 14:411–25. doi: 10.1016/j.mric.2006.10.002
93. Zhou J, Liu J, Yu A. Numerical study on the thawing process of biological tissue induced by laser irradiation. J Biomech Eng. (2005) 127:416–31. doi: 10.1115/1.1894294
94. Bradley PF, Polayes IM. Cryosurgery of the maxillofacial region. Volumes one and two. Plastic Reconstr Surg. (1989) 84:534–5. doi: 10.1097/00006534-198909000-00029
95. Wu PK, Chen CF, Wang JY, Chen PCH, Chang MC, Hung SC, et al. Freezing nitrogen ethanol composite may be a viable approach for cryotherapy of human giant cell tumor of bone. Clin Orthop Relat Res. (2017) 475:1650–63. doi: 10.1007/s11999-017-5239-3
96. den Brok MH, Sutmuller RP, Nierkens S, Bennink EJ, Toonen LW, Figdor CG, et al. Synergy between in situ cryoablation and TLR9 stimulation results in a highly effective in vivo dendritic cell vaccine. Cancer Res. (2006) 66:7285–92. doi: 10.1158/0008-5472.CAN-06-0206
97. Fujita H, Iida H, Ido K, Matsuda Y, Oka M, Nakamura T. Porous apatite-wollastonite glass-ceramic as an intramedullary plug. J Bone Joint Surg Br. (2000) 82:614–8. doi: 10.1302/0301-620X.82B4.0820614
98. Holmes RE, Bucholz RW, Mooney V. Porous hydroxyapatite as a bone-graft substitute in metaphyseal defects. A histometric study. J Bone Joint Surg Am. (1986) 68:904–11. doi: 10.2106/00004623-198668060-00013
99. Teramoto H, Kawai A, Sugihara S, Yoshida A, Inoue H. Resorption of apatite-wollastonite containing glass-ceramic and beta-tricalcium phosphate in vivo. Acta Med Okayama. (2005) 59:201–7. doi: 10.18926/AMO/31974
100. Walsh WR, Vizesi F, Michael D, Auld J, Langdown A, Oliver R, et al. Beta-TCP bone graft substitutes in a bilateral rabbit tibial defect model. Biomaterials. (2008) 29:266–71. doi: 10.1016/j.biomaterials.2007.09.035
101. Mjöberg B, Pettersson H, Rosenqvist R, Rydholm A. Bone cement, thermal injury and the radiolucent zone. Acta Orthop Scand. (1984) 55:597–600. doi: 10.3109/17453678408992403
102. Rock M. Adjuvant management of benign tumors; basic concepts of phenol and cement use. Chir Organi Mov. (1990) 75(Suppl. 1):195–7.
103. Takeuchi A, Suwanpramote P, Yamamoto N, Shirai T, Hayashi K, Kimura H, et al. Mid- to long-term clinical outcome of giant cell tumor of bone treated with calcium phosphate cement following thorough curettage and phenolization. J Surg Oncol. (2018) 117:1232–8. doi: 10.1002/jso.24971
104. Fraquet N, Faizon G, Rosset P, Phillipeau J, Waast D, Gouin F. Long bones giant cells tumors: treatment by curettage and cavity filling cementation. Orthop Traumatol Surg Res. (2009) 95:402–6. doi: 10.1016/j.otsr.2009.07.004
105. Kotrych D, Korecki S, Zietek P, Kruk B, Kruk A, Wechmann M, et al. Preliminary results of highly injectable bi-phasic bone substitute (CERAMENT) in the treatment of benign bone tumors and tumor-like lesions. Open Med. (2018) 13:487–92. doi: 10.1515/med-2018-0072
106. Hattori H, Matsuoka H, Yamamoto K. Radiological and histological analysis of synthetic bone grafts in recurring giant cell tumor of bone: a retrospective study. J Orthop Surg. (2010) 18:63–7. doi: 10.1177/230949901001800114
107. Shin HS, Kim MS, Kim BH, Lim HJ, Kim BC, Lee J. Reconstruction of mandibular defects with bone marrow-derived stem cells in odontogenic myxoma. J Craniofac Surg. (2020) 31:e236–9. doi: 10.1097/SCS.0000000000006147
108. Isobe M, Yamazaki Y, Mori M, Amagasa T. Bone regeneration produced in rat femur defects by polymer capsules containing recombinant human bone morphogenetic protein-2. J Oral Maxillofac Surg. (1999) 57:695–8; discussion 699. doi: 10.1016/S0278-2391(99)90435-4
109. Ruhe PQ, Hedberg EL, Padron NT, Spauwen PH, Jansen JA, Mikos AG. rhBMP-2 release from injectable poly (DL-lactic-co-glycolic acid)/calcium-phosphate cement composites. JBJS. (2003) 85(Suppl. 3):75–81. doi: 10.2106/00004623-200300003-00013
110. Pietschmann MF, Dietz RA, Utzschneider S, Baur-Melnyk A, Jansson V, Dürr HR. The influence of adjuvants on local recurrence rate in giant cell tumour of the bone. Acta Chir Belg. (2010) 110:584–9. doi: 10.1080/00015458.2010.11680682
111. Moon MS, Kim SS, Moon JL, Kim SS, Moon H. Treating giant cell tumours with curettage, electrocautery, burring, phenol irrigation, and cementation. J Orthopaed Surg. (2013) 21:209–12. doi: 10.1177/230949901302100219
112. Gao ZH, Yin JQ, Xie XB, Zou CY, Huang G, Shen JN. Local control of giant cell tumors of the long bone after aggressive curettage with and without bone cement. BMC Musculoskelet Disord. (2014) 15:330. doi: 10.1186/1471-2474-15-330
113. van der Heijden L, van der Geest IC, Schreuder HW, van de Sande MA, Dijkstra PD. Liquid nitrogen or phenolization for giant cell tumor of bone? A comparative cohort study of various standard treatments at two tertiary referral centers. J Bone Joint Surg Am. (2014) 96:e35. doi: 10.2106/JBJS.M.00516
114. Benevenia J, Rivero SM, Moore J, Ippolito JA, Siegerman DA, Beebe KS, et al. Supplemental bone grafting in giant cell tumor of the extremity reduces non-oncologic complications. Clin Orthop Relat Res. (2017) 475:776–83. doi: 10.1007/s11999-016-4755-x
115. Sirin E, Akgülle AH, Topkar OM, Sofulu Ö, Baykan SE, Erol B. Mid-term results of intralesional extended curettage, cauterization, and polymethylmethacrylate cementation in the treatment of giant cell tumor of bone: a retrospective case series. Acta Orthop Traumatol Turc. (2020) 54:524–9. doi: 10.5152/j.aott.2020.19082
116. Ke J, Cheng S, Yao MY, Chu X, Wang M, Zeng XL, et al. Novel strategy of curettage and adjuvant microwave therapy for the treatment of giant cell tumor of bone in extremities: a preliminary study. Orthop Surg. (2021) 13:185–95. doi: 10.1111/os.12865
117. Pan KS, Boyce AM. Denosumab treatment for giant cell tumors, aneurysmal bone cysts, and fibrous dysplasia-risks and benefits. Curr Osteoporos Rep. (2021) 19:141–50. doi: 10.1007/s11914-021-00657-z
118. Zhao Y, Cai Z, Tang X, Du Z, Yang Y, Guo W. Preoperative denosumab may increase the risk of local recurrence of giant-cell tumor of bone treated with curettage: a systematic review and meta-analysis. J Cancer. (2021) 12:508. doi: 10.7150/jca.50575
119. Perrin DL, Visgauss JD, Wilson DA, Griffin AM, Abdul Razak AR, Ferguson PC, et al. The role of Denosumab in joint preservation for patients with giant cell tumour of bone. Bone Joint J. (2021) 103-B:184–91. doi: 10.1302/0301-620X.103B1.BJJ-2020-0274.R1
Keywords: giant cell tumor of bone, surgical treatment, local control, filling materials, osteogenic induction
Citation: Wang Y, Tian Q, Wu C, Li H, Li J and Feng Y (2021) Management of the Cavity After Removal of Giant Cell Tumor of the Bone. Front. Surg. 8:626272. doi: 10.3389/fsurg.2021.626272
Received: 16 November 2020; Accepted: 24 June 2021;
Published: 29 July 2021.
Edited by:
Jaimo Ahn, University of Michigan, United StatesReviewed by:
Grant Pang, St Vincent's Hospital (Melbourne), AustraliaClaudia Di Bella, The University of Melbourne, Australia
Copyright © 2021 Wang, Tian, Wu, Li, Li and Feng. This is an open-access article distributed under the terms of the Creative Commons Attribution License (CC BY). The use, distribution or reproduction in other forums is permitted, provided the original author(s) and the copyright owner(s) are credited and that the original publication in this journal is cited, in accordance with accepted academic practice. No use, distribution or reproduction is permitted which does not comply with these terms.
*Correspondence: Yi Feng, ZmVuZ3lpMTYwQDE2My5jb20=