- 1Department of Physiology, Medical College of Georgia at Augusta University, Augusta, GA, United States
- 2Department of Physiology and Pharmacology, University of Toledo College of Medicine and Life Sciences, Toledo, OH, United States
- 3Department of Surgery, Medical College of Georgia at Augusta University, Augusta, GA, United States
Diabetic bladder dysfunction (DBD) is a well-recognized and common symptom affecting up to 50% of all diabetic patients. DBD has a broad range of clinical presentations ranging from overactive to underactive bladder symptoms that develops in middle-aged to elderly patients with long standing and poorly controlled diabetes. Low efficacy of current therapeutics and lifestyle interventions combined with high national healthcare costs highlight the need for more research into bladder dysfunction pathophysiology and novel treatment options. Cellular senescence is an age-related physiologic process in which cells undergo irreversible growth arrest induced by replicative exhaustion and damaging insults. While controlled senescence negatively regulates cell proliferation and promotes tissue regeneration, uncontrolled senescence is known to result in tissue dysfunction through enhanced secretion of inflammatory factors. This review presents previous scientific findings and current hypotheses that characterize diabetic bladder dysfunction. Further, we propose the novel hypothesis that cellular senescence within the urothelial layer of the bladder contributes to the pro-inflammatory/pro-oxidant environment and symptoms of diabetic bladder dysfunction. Our results show increased cellular senescence in the urothelial layer of the bladder; however, whether this phenomenon is the cause or effect of DBD is unknown. The urothelial layer of the bladder is made up of transitional epithelia specialized to contract and expand with demand and plays an active role in transmission by modulating afferent activity. Transition from normal functioning urothelial cells to secretory senescence cells would not only disrupt the barrier function of this layer but may result in altered signaling and sensation of bladder fullness; dysfunction of this layer is known to result in symptoms of frequency and urgency. Future DBD therapeutics may benefit from targeting and preventing early transition of urothelial cells to senescent cells.
Introduction
The bladder functions to store and excrete urine, which is required to remove waste from the body. Normal urine storage occurs over a broad range of volumes that empties in a controlled and efficient manner at an appropriate time and location. This not only requires accurate sensation of bladder fullness, but also coordinated actions of the parasympathetic and sympathetic nervous systems to contract and relax the urinary bladder wall and urethral sphincters (1). Dysregulation of the urinary bladder results in lower urinary tract symptoms (LUTS) such as nocturia, urgency, frequency, incontinence, etc. (2), which if left untreated, can lead to systemic inflammatory response syndrome and sepsis, and even possibly death (3, 4). LUTS have a significant negative impact on quality of life and are strongly associated with depression (5–7). These symptoms also impact United States health care costs; overactive bladder alone is estimated to cost $82.6 billion by 2020 (8). LUTS are frequent in the general population, affecting 62.5% of men and 66.6% of women (9). The incidence of LUTS also increases with age (10) and with an aging population (11), we expect the number of patients experiencing LUTS to be on the rise.
LUTS are not only associated with age, they are also comorbid with stroke, brain tumors, cerebral palsy, dementia, sexual dysfunction, spinal cord injury, multiple sclerosis, and diabetes (12–20). Diabetes is characterized by defects in the secretion or signaling of insulin that results in the impairment of glucose uptake and ensuing high plasma glucose levels (21). Initially, hyperglycemia results in an elevated concentration of glucose in the urine, exceeding the amount reabsorbed by the kidney, leading to osmotic diuresis and polyuria. During this early compensated state, patients may present with symptoms of overactive bladder, including urgency, frequency, and nocturia. Over time, the early compensated state progresses into a late decompensated state that research suggests is due to chronically high levels of glucose and oxidative stress (Figure 1; 22; 13). Patients within the late decompensated state present with symptoms of underactive bladder, including decreased bladder contraction, increased post-void residual volume, difficulty initiating and maintaining voiding, and enhanced capacity.
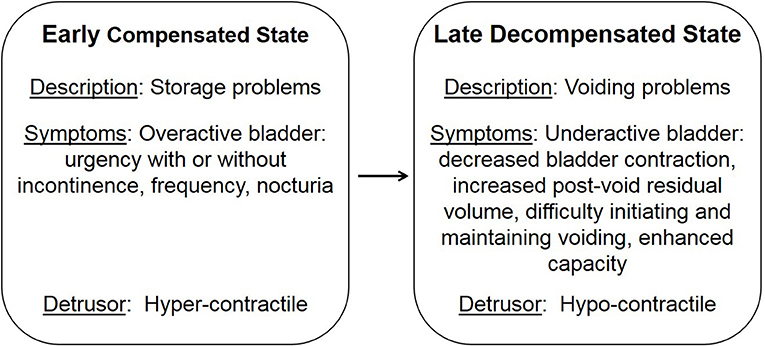
Figure 1. Temporal progression of diabetic bladder dysfunction. Adapted from Daneshgari et al. (13).
LUTS affect up to 50% of all diabetic patients and are referred to as diabetic bladder dysfunction (DBD) (13, 22). Despite recent advances in understanding DBD, underlying mechanisms are unclear and to complicate things further, there are few studies that parcel out pure age-dependent changes from dysfunction due to underlying pathology or disease (10, 23). Here, we discuss the current theories underlying DBD and a novel hypothesis that increased cellular senescence within the bladder is the initiating factor in tissue dysfunction, which culminates in symptoms of DBD.
Theories and Hypotheses on the Etiology of Diabetic Bladder Dysfunction
There are multiple suggested mechanisms underlying DBD symptoms that are generalized to the whole bladder although the majority are focused on the smooth muscle layer. Literature reports alterations within the components of muscle contraction (calcium handling, receptors, channels, protein isoforms, thin, and thick filaments) (24–29), oxidative stress (30, 31), inflammation (32–34), neuropathy (35–37), and age-related changes (38). Possibly the most consistently reported change is an increase in whole bladder weight (Figure 2; original data). Interestingly, diabetic animals and control aged animals have similar increases in bladder weight compared to young controls; these changes parallel the increased presence of LUTS with diabetes and age in human populations.
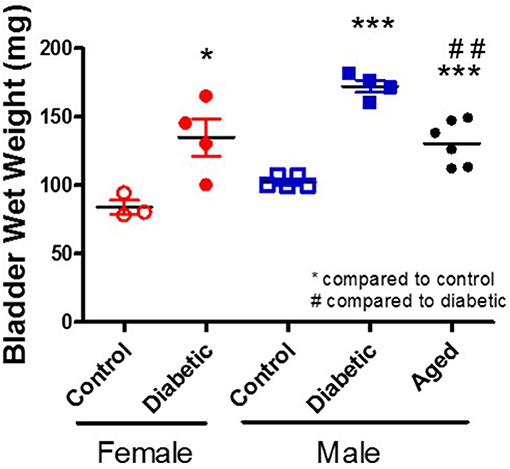
Figure 2. Increased bladder weight in diabetic and aged animals. Bladder wet weight was measured in female and male control and diabetic animals (18–20 w of age; 1 month post-STZ injections) and in aged male animals (53 w of age). Diabetes was induced using the combination of a high fat diet and one streptozotocin injection (30 mg/kg) in Wistar animals.
DBD encompasses both storage and voiding symptoms and has been hypothesized, by Daneshgari's group in 2009, to progress temporally from an early compensated state, where the bladder adapts to increased polyuria, to a late decompensated state due to chronically high levels of glucose and oxidative stress (13, 39, 40). This temporal hypothesis is the most current hypothesis detailing disease progression. In 2014, Chancellor further hypothesized that the early compensated state leads to the late decompensated state through tissue fatigue and changes in the structure and function of the bladder leading to ischemia, inflammation, oxidative stress, and this induces impaired contractility in the decompensated state (38).
This section highlights reported changes within the different layers of the urinary bladder. Scientific studies mentioned within this section rarely parcel out voiding behavior as an output measurement when investigating mechanisms of bladder dysfunction; therefore, classification into overactive or underactive bladder pathophysiology is unclear.
Urinary Bladder
Urothelium
The urothelial layer is the inner most layer of the bladder that functions as a permeability barrier to urine, protecting the underlying tissue. It has sensory functions that respond to chemical, mechanical, and thermal stimuli by releasing factors such as ATP, acetylcholine, and nitric oxide to modulate afferent transmission, relaying the extent of bladder fullness (41–44). The urothelium is made up of three layers of cells: basal, intermediate, and apical or superficial cells (45). The superficial cells are the main component in barrier function and exhibit specialized tight junctions, which facilitate the prevention of unregulated chemical diffusion (45). Barrier dysfunction results in symptoms such as urgency and frequency and this layer has been shown to be altered by diabetes (46). For example, Hanna-Mitchell et al. in a streptozotocin (STZ; 65 mg/kg)—induced model of diabetes, through preferential toxicity to the insulin producing β-cells of the pancreas, reported that exposure to chronic hyperglycemia induces desquamation of the superficial cells, subsequently contributing to breaches in the barrier function of the urothelium (47). At a later time point, the urothelium exhibited barrier repair and vast changes both in cell morphology and gene expression (glucose metabolism, cell survival and proliferation, parasympathetic receptors, and cell stress/death), which are also factors that are altered in senescence (47). Further, Wang et al. reported increased urothelial inflammation in overactive bladder, which they noted did not differ between overactive patients with or without diabetes; however, overactive bladder was diagnosed solely based on urgency and urgency incontinence (48). Initial diabetes-induced damage to the urothelial layer could contribute to symptoms of overactive bladder, whereas repair accompanied with cellular changes may indicate the transition between the compensated and decompensated states of DBD.
Lamina Propria
The lamina propria is a loose areolar connective tissue that is located deep to the urothelial layer. The lamina propria connects the urothelial layer to the underlying smooth muscle layer and is both blood perfused and innervated, supporting the urothelial layer with nutrients and neuromediators. This layer contains collagen as well as several cell types, including interstitial cells (IC), fibroblasts, and adipocytes (41). Studies have reported changes in collagen composition (both increased and decreased) possibly contributing to altered strength and stiffness of the tissue (40). Increased collagen levels within the lamina propria could lead to increased tissue stiffness and strength as well as decreased nutrient diffusion to the urothelial layer and initially contribute to symptoms of overactive bladder. Decreased collagen levels would result in decreased tissue and contribute to symptoms of underactive bladder. The function of resident cells of the lamina propria could also be affected by diabetes. Recent identification of IC seem to serve similar roles as IC of Cajal (ICC) in the gastrointestinal (GI) tract by regulating smooth muscle excitability and mediating responses to neurotransmitters (49–51). ICC have spontaneous pacemaker activity which is conducted to the smooth muscle layer through gap junctions resulting in electrical slow waves and phasic contractions. Neural inputs, from motor neurons in close proximity to ICC, can also induce contraction by electrically coupling with smooth muscle cells. This complex operates below the level of neural and hormonal smooth muscle regulation and a loss of any component or connectivity of the complex has been associated with GI motor disorders (52). For example, a decrease in the number of GI ICC is believed to contribute to disturbed GI motility in human patients with type 2 diabetes (53) and diabetic db/db mice (54). IC within the urinary bladder has gained much interest due to evidence of its association with bladder pathophysiology where the number of IC have been shown to be both increased (55, 56) and decreased in overactive bladder and bladder outlet obstruction (57). Further, alterations or disruptions of these IC have been proposed to disrupt homeostatic tissue function within the urinary bladder (55, 58, 59). Increases in the number of IC could in theory increase the amount of spontaneous smooth muscle activity; therefore contribute to symptoms of urgency within overactive bladder. In contrast, a decrease in the number of IC could lead to decreased contractility and symptoms present within underactive bladder.
Smooth Muscle
The smooth muscle layer of the urinary bladder functions to contract (parasympathetic nervous system) and relax (sympathetic nervous system) the bladder during micturition and urine storage, respectively. There are a variety of DBD studies focused at the layer of the smooth muscle that depict modifications within contractility, neurotransmitter release, receptor and channel expression, thick and thin filament and contractile protein expression, and protein phosphorylation; however, results are varied showing both increased and decreased expression of components mentioned (13, 25, 27–29, 31, 60).
Urethra
The urethra is a duct that transfers urine from the urinary bladder to the exterior of the body. The urethra is held closed by two sphincters, the internal urethral sphincter, and the external urethral sphincter, which are made up of smooth and skeletal muscle, respectively. Alterations with urethral function have been reported, showing both smooth and skeletal muscle dysfunction of urethral relaxation during the voiding reflex (61, 62). This could contribute to increased bladder pressure, increased void volume, and trouble initiating and maintaining voiding, altering normal bladder function and causing further damage to the urinary bladder. Consequences of these alterations include increased intravesicle pressure, as well as increased detrusor muscle contraction as a compensatory mechanism, contributing to underactive bladder symptoms.
Diabetes Is Associated With Increased Cellular Senescence
Cellular senescence is a physiologic process in which replication-competent cells undergo irreversible cell cycle arrest induced essentially by any insult that produces DNA damage (replicative exhaustion and telomere shortening, excessive/ prolonged cellular stress) that can be grouped into three different subtypes: replicative senescence, stress-induced premature senescence, and oncogene-induced senescence (63, 64). This DNA damage results in the downstream activation of p53 and convergence on the inhibition of cyclin-dependent kinases (CDK), a family of protein kinases that are involved with cell cycle regulation (63). The inhibition of CDK–cyclin complexes results in proliferative arrest and the crucial component responsible for the implementation of senescence is the hypo-phosphorylated form of retinoblastoma protein (64). Senescence is generally considered a physiologic process and a protective mechanism to prevent morbidity by reducing the transmission of genomic defects to the next generation (63). In fact, studies suggest that controlled senescence promotes tissue regeneration via the recruitment of phagocytic immune cells to sites of injury after which senescent cells are then cleared (65, 66). Recruitment of immune cells depend on senescent secretory activity known as the senescence-associated secretory phenotype (SASP), allowing the secretion of pro-inflammatory cytokines and chemokines, reactive oxygen species (ROS), growth factors, and proteases into the local tissue microenvironment through autocrine and paracrine signaling (64, 67). An accumulation of senescent cells occurs with aging and is associated with tissue dysfunction, as well as numerous pathologies. Interestingly, we have evidence that urothelial senescence also increases with age (Figure 3; original data). Mechanistically, senescence-induced tissue dysfunction and pathology is thought to occur through the chronic release of SASP mediators and a heightened inflammatory state (64, 68–72).
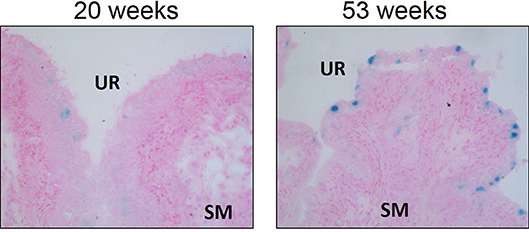
Figure 3. Senescence associated-β-galactosidase staining is increased in aged Wistar urothelium compared to young control counterparts. Senescence associated-β-galactosidase staining (blue) and nuclear fast red counterstain in young (20 w of age) animals and old (53 w of age) animals (left and right panels, respectively). Representative images of bladder SA-β-galactosidase at 200X. SM, smooth muscle. UR, urothelium.
Recent evidence suggests that cellular senescence is an important developmental contributor and/or consequence of type 2 diabetes (70). For example, several studies describe augmented inflammation and increased number of senescent cells within pancreatic β-cells of type 2 diabetic patients (73, 74). Monocytes from type 2 diabetic patients exhibit telomere shortening and increased oxidative DNA damage compared to healthy individuals (75). Fibroblasts isolated from diabetic wounds exhibit disturbed proliferation (76). Senescent cells are increased in adipose tissue of both type 2 diabetic animal models and patients (77, 78). Further, high glucose induces cellular senescence in multiple cell types in vitro including endothelial cells, mesothelial cells, vascular smooth muscle cells, mesenchymal stem cells, and skin fibroblasts (79–83). We also have evidence that high glucose increases cellular senescence in primary bladder smooth muscle cells (Figure 4; original data). Overall, evidence suggests an association between type 2 diabetes and increased cellular senescence, possibly via heightened (mitochondrial) oxidative stress (77, 78). In fact, targeting senescent cells in type 2 diabetes has been suggested as a therapeutic option (70). Low-grade systemic inflammation is a common manifestation of diabetes; however, the exact mechanisms that initiate this pathophysiological response, thereby contributing to diabetic complications, including DBD and LUTS is not known.
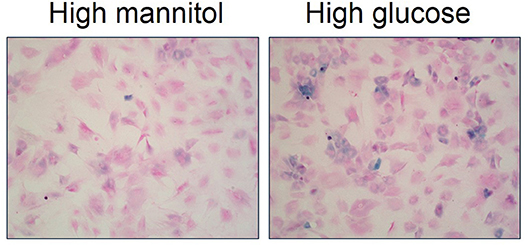
Figure 4. High glucose increases senescence associated-β-galactosidase staining in primary bladder smooth muscle cells. Senescence associated-β-galactosidase (blue) and nuclear fast red counterstain in high mannitol osmotic control (22 mM; left panel) and high glucose (22 mM; right panel) after a 4 day incubation. Representative images of primary bladder smooth muscle cell senescence associated-β-galactosidase at 100X.
The Immune Response and Inflammation in Diabetic Bladders
The urinary tract has several lines of defense that protect against infection. The first line of defense are superficial urothelial cells that not only act as a physical barrier, but also secrete pro-inflammatory mediators and antibacterial agents. Urothelial cells are connected by tight junctions, made up of 4–6 intracellular protein strands containing claudin, occludin, junction adhesion molecules, and zonula occludens that link these strands to the intracellular cytoskeleton (84, 85). This robust architecture is essential, and it contributes to the sterility of the bladder by preventing passive movement of particles between the blood and the urine. Further, these cells contain thick scallop-shaped plaques along the apical membrane that are covered with a sulfated polysaccharide glycosaminoglycan layer to further maintain the physical barrier of the bladder by deterring microbial colonization (84, 86, 87). In addition, urothelial cells also secrete factors that inhibit bacterial growth by interfering with the structure of the bacterial cell membrane or preventing protein synthesis within the cell (88–92).
Urothelial cells possess an abundance of pattern recognition receptors (PRRs) of the innate immune system including Toll-like receptors (TLRs), which recognize early indications of infection and release pro-inflammatory mediators (93). TLR4 is perhaps the best understood and most prevalent TLR in the urothelium; stimulation of which has been shown to result in expulsion of bacteria containing vacuoles from urothelial cells (94). Activation of TLR4 leads to interaction of its intracellular binding domain with an adaptor protein complex that includes MyD88 ultimately promoting the NF-κB pathway and transcription of cytokines such as interleukin 6 (IL-6) and interleukin 8 (IL-8) (94). TLR4 and its downstream mediators have been shown to be increased in DBD suggesting increased inflammation may play a role in DBD pathogenesis (34).
Bacterial infection can also activate caspase induced apoptosis of infected urothelial cells, effectively releasing contents into the lumen of the bladder. This not only removes bacteria, but it also attenuates excessive release of pro-inflammatory molecules. However, excessive removal of urothelial cells can expose underlying cell layers to toxins that are present within urine. As a protective measure, the urothelium secretes sonic hedgehog (SHH) to activate the WNT pathway, initiating urothelial cell proliferation to restore the epithelial barrier (95). This mechanism is reinforced by studies that show higher levels of apoptotic cells, antiproliferative factor, ATP, and vascular endothelial growth factor in the urine or tissue of patients with bladder infection (96–100). While the WNT pathway has not been studied in DBD, bladder thickness of the urothelium are increased in DBD and could be the result of this signaling process (32, 101).
Neutrophils are the first immune cells recruited to the urothelium from the blood vessels to the lumen of the bladder where they can act upon bacteria (102). The number of neutrophils recruited is congruent with the relative amount of bacteria present, and peaks approximately 6 h after activation (103). While neutrophils are highly effective at eliminating bacteria, they are also toxic to bladder tissue because they release ROS, increase expression of cyclooxygenase 2 (COX2), and induce expression of other cytotoxic products that cause inflammatory damage to the bladder. Neutrophil byproducts have been shown to cause hyperplasia and also predispose the bladder to persistent infections (104).
Bladder mast cells reside beneath the epithelium and are triggered via secretions of damaged or stressed epithelial cells in response to ATP, IL-33, and β-defensin (105–107). Mast cells regulate the immune response by releasing pro-inflammatory molecules such as histamine and TNF-α, which further contributes to the recruitment of neutrophils (108). Diabetic patients have significantly higher levels of mast cells in the bladder than patients without diabetes (48). Interestingly, TNF-α is not only elevated in bladder smooth muscle cells and serum isolated from diabetic mice, it also plays a critically important role in DBD by activating Rho kinase and ultimately causing bladder smooth muscle cell hypercontractility; neutralizing TNF-α improved bladder function (33).
Oxidative Stress in Diabetic Bladders
Oxidative stress due to elevated levels of ROS has also been implicated in the pathogenesis of DBD. Diabetic hyperglycemia is capable of causing oxidative stress through several key mechanisms: upregulation of the polyol pathway, increased production of advanced glycation end-products, increased activity of protein kinase C, increased activation of the hexosamine pathway, and ultimately the overproduction of superoxide by the electron transport chain (109–115). As oxidative stress ensues, cellular functions are impaired due to damaged DNA, mitochondrial enzyme dysfunction, and phospholipid bilayer destruction. These damaging effects contribute to morphological and functional changes associated with DBD.
Multiple studies show the presence of oxidative stress and subsequent structural and functional modifications in diabetic bladders. For example, bladders from type 2 diabetic female Sprague-Dawley rats exhibit significant increases in nitrotyrosine and manganese superoxide dismutase protein expression compared to both age-matched control and diuresis (40). Diabetes and diuresis both resulted in significant increases in bladder weight; however, the increase in oxidative stress was specific only to the diabetic model. In another study, diabetic Sprague Dawley rats exhibited a reduction in catalase-like activity, a measure of antioxidant scavenging capability in aerobic cells compared to age-matched control and diuresis (30). Further, these diabetic bladders presented higher levels of apoptotic cells, an increase in lipid peroxidation, and an increase in inducible nitric oxide synthase expression and concluded that oxidative damage to the smooth muscle layer may contribute to DBD symptoms (30). In addition, diabetic bladders from 2 month old rats exhibited increased expression of genes involved in the production or regulation of ROS, higher levels of lipid peroxidation, reduced glutathione S-transferase activity, an increase in protein oxidation and nitrosylation, and increased apoptosis markers (116). Results from this study suggest that increased oxidative stress and apoptosis are associated with impaired bladder contractile capacity. Likewise, bladders from diabetic New Zealand rabbits, exhibited significantly increased lipid peroxides, aldose reductase, and sorbitol compared to both age-matched control and a diuresis model (31).
ROS can also lead to the breakdown of proteins via autophagy, which is a compensatory mechanism for an organism under oxidative stress to preserve cell organelles and proteins. ROS can induce autophagy by oxidizing a cysteine residue in Atg4, which acts to convert microtubule-associated protein light chain 3 to its active form, leading to autophagy (117, 118). A study involving both diabetic human and animal studies showed increased levels of autophagy in β-cells, and further proposed that autophagy serves a protective role against apoptosis by preserving cells and preventing further oxidative damage (119–121). However, persistent autophagy is detrimental as it leads to autophagic cell death (120, 122–124). Consequently, ROS-induced autophagy caused by diabetes can lead to further oxidative stress that damages or destroys smooth muscle cells, contributing to hypocontractility and bladder dysfunction (116).
We have observed increased senescence in DBD from type 2 diabetic female Wistar rats (high fat diet and 30 mg/kg i.p. STZ) at 1 month post-STZ injections (Figure 5; original data). At this time point, senescence was localized mainly to the urothelium layer of the bladder, which corresponds with Hanna-Mitchell's findings that the urothelial layer changes both in cell morphology and gene expression (47). Further, gene expression alterations in the diabetic urothelium reported by Hanna-Mitchell et al. are also factors that are altered in senescence (125–127). While inappropriate immune system activation has been well established in diabetes and DBD, it is not known whether the presence of senescent cells can exacerbate the pro-inflammatory/pro-oxidative milieu, thereby worsening LUTS.
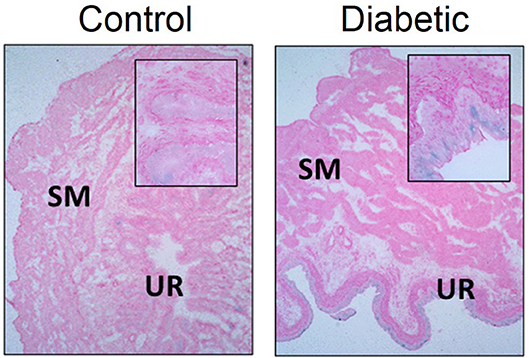
Figure 5. Senescence associated-β-galactosidase staining is increased in diabetic urothelium compared to control counterparts. Senescence associated-β-galactosidase (blue) staining and nuclear fast red counterstain in control (Left) and diabetic (Right) bladders. Diabetes was induced using the combination of a high fat diet and one streptozotocin injection (30 mg/kg) in Wistar animals. Representative images of bladder senescence associated-β-galactosidase staining at 100X, inserts at 400X magnification. SM, smooth muscle. UR, urothelium.
Hypothesis
We hypothesize that uncontrolled urothelial senescence contributes to increased inflammation and oxidative stress within the bladder wall and contributes to symptoms of DBD (Figure 6). The SASP of senescent bladder cells, through uncontrolled and excessive pro-inflammatory mediators (e.g., cytokines, chemokines) and ROS generation, could further impair the function of healthy bladder cells, as well as mediate additional transition to the senescent state.
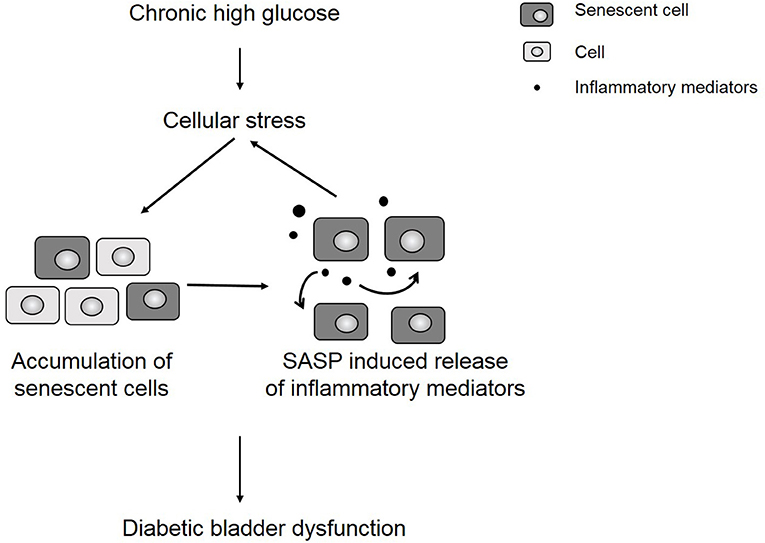
Figure 6. Chronic hyperglycemia contributes to cellular stress and an accumulation of senescent cells. The presence of senescent cells further contributes to increased inflammation in the bladder wall, which leads to symptoms of diabetic bladder dysfunction.
Mechanisms driving initial senescence induction on the apical side of urothelial cells may include chronic increases in urine volume and therefore increased workload and bladder stretch, and exposure to an altered urine content (increased protein, glucose, damaged tubule cell contents). Diabetic kidney disease is a prominent complication of diabetes that is characterized by glomerular hyperfiltration (128). The ultra-filtrate (urine), containing proteins, growth factors, and cytokines, causes apical tubular cell injury and interstitial fibrogenesis within the kidney (128). This ultra-filtrate is transferred from the kidney to the urinary bladder via ureters (128). Therefore, these same factors, which mediated kidney damage, are now exposed to the urothelial layer of the bladder. Increased exposure to chronic high blood glucose concentrations may also affect urothelial cells from the basal side. High and chronic levels of glucose is known to cause mitochondrial dysfunction and increases the expression of advanced glycation end products and ROS, both of which have been shown to induce senescence (129–134).
As mentioned previously, senescent cells release multiple factors (cytokines, ROS, etc.), which may induce DBD symptoms and further drive the accumulation of senescent cells. Oxidative stress, which has been shown in numerous in vitro and in vivo studies to be remarkably high in diabetic patients, is also a major factor in the activation of cellular senescence (80). The major source of senescence-inducing ROS appears to occur through mitochondrial dysfunction (132), although other enzymatic sources of ROS are also known to contribute (134). Furthermore, increased advanced glycation end products and chronic inflammation, both linked to diabetes, have also been shown to induce senescence (130, 131, 133). Whether accumulating senescent cells could be responsible for or the result of increased inflammation and ROS reported in diabetic bladders is not explicitly known; however, this process could be complementary in that high glucose results in a heightened inflammatory state that increases the accumulation of senescent cells. The SASP could enhance development and accumulation of senescence leading to a positive feedback loop (Figure 3).
The authors believe that the hypothesis presented is testable within the framework of current knowledge. While we have shown increased senescence associated-β-galactosidase staining within the urothelial layer of diabetic bladders, other markers indicative of cellular senescence at different and longer time points and within different models of diabetes should be measured. Multiple standard measurements have been established to indicate senescence. Senescent cells exhibit increased expression of senescence associated-β-galactosidase and p16INK4A as well as secreted inflammatory factors (IL-1, IL-6, IL-8, VEGFA, MMPs) as part of their SASP, measureable by senescence associated-β-galactosidase assays, western blotting, and ELISA (135). Further, increased DNA damage and telomere shortening are also indicators of cellular senescence. The effect and presence of senescence during each state of DBD needs to be investigated coinciding with voiding behavior and cytometric measurements. For example, senescent cells may increase initially after initial insult of DBD due to increased volume of urine and subside with the recruitment of immune cells to the site of injury. One would expect that senescent cells may accumulate again within the later stages of DBD due to increased ROS after prolonged hyperglycemia and chronic increased voiding volume; this would concur with current literature showing the presence of cellular senescence with age as well as enhanced inflammation. One may further postulate if increased urothelial senescence could then lead to increased cellular senescence within the smooth muscle layer of the bladder. There are multiple models of diabetes and all could be used to test this hypothesis. A limitation to the use of diabetic animal models is that these models develop overt disease in short time frames whereas the human condition may develop over longer periods of time and are then managed with various therapeutics and lifestyle interventions; therefore, timing of disease progression and corresponding mechanistic alterations may not be identical. An important control group to consider would be cases of bladder dysfunction, both overactive and underactive bladder, that are not associated with increased blood glucose levels such as diabetes insipidus and bladder dysfunction associated with old age and other disease states. This would determine whether increased cellular senescence was the direct consequence of oxidative stress due to increased and chronic levels of blood glucose. Once evidence of senescence in animal models is established, it would be imperative to show increased urothelial senescence in human DBD patients within both overactive and underactive bladder symptoms possibly through urothelial biopsies.
Some potential limitations to this hypothesis include understanding whether senescent cells are the cause or the effect of DBD in vivo. Therefore, although therapeutic targeting of senescent cells may offer symptomatic relief to LUTS, it may not reveal the underlying pathogenic mechanism of bladder dysfunction. Furthermore, parsing out the contribution of senescent urothelial cells to the omnipresent oxidative and inflammatory burden in diabetes could prove challenging. This is especially pertinent given that the specific enzymatic and pattern recognition receptor specific sources of ROS and cytokines, respectively, have not been determined in senescent urothelial cells and will require careful experimental design and potentially novel models of DBD.
Clinical Perspectives
Due to the insidious onset of DBD, patients may not notice changes in voiding habits until later stages (86). Additionally, patients may not voice their concerns to their healthcare providers unless questioned specifically on symptoms due to the stigma associated with LUTS, particularly with urinary incontinence (136, 137). In one study of women ages 20–45, only 10.8% with overactive bladder and 12.7% with urge incontinence have seen their physicians for those symptoms (136, 138).
Patients with suspected DBD should be evaluated with detailed history and physical exam. Once more serious causes of LUTS are ruled out, patients may undergo urodynamic testing to assess lower urinary tract function (139, 140). Urodynamic testing may not be necessary if diagnosis is clear (139, 140).
Current treatment for DBD include behavioral changes (i.e., weight loss, timed voids, pelvic floor exercises), pharmacological, and surgical interventions (86, 139). For overactive bladder, medications target bladder specific muscarinic receptors (anticholinergics) or ß3-adrenergic receptor agonists to reduce detrusor contractions (86, 139). Muscarinic antagonism improves some lower urinary symptoms; however, efficacy is questionable and tolerability is poor due to side effects, the major one being “dry mouth.” In a study of patients with overactive bladder, oxybutynin and tolterodine treatment only resulted in one fewer leakage episode or micturition event per 48 h compared to placebo (141). Similarly, in a study where women took anti-muscarinic agents reported an average of three leaks a day after 6 months of treatment compared to an average of five a day at the start of treatment. Not only is efficacy of these drugs in question, there are significant side effects of these medications such as dry mouth, constipation, difficulty in urination, blurred vision, dry eyes, drowsiness, dizziness, and cognitive decline, which makes long term treatment a challenge for patients (141). The most bothersome side effect for patients is dry mouth; 25% of patients will cease taking this medication because of this side effect. Increased duration of dry mouth will cause dental caries and difficulty in speech; in order to alleviate the dry mouth symptoms, patients will drink more water and therefore exacerbate lower urinary tract symptoms. Patients that fail behavioral modification and pharmacological therapy may benefit from more invasive methods such as intradetrusor botulinum toxin A injections or neuromodulation (86, 139). For underactive bladder, behavior modifications are encouraged but pharmacologic options are limited; current treatment options include methods such as asking patients to “double-void,” placement of an indwelling catheter, and intermittent self-catheterization (86). Long-term use of indwelling catheters are associated with high risk of complications such as urinary tract infections and urethral lesions (142, 143). Ultimately, other surgical intervention may be explored for both overactive and underactive bladder if the patient does not respond to less invasive treatment options (86, 139).
Senescence as a Novel Target for Diabetic Bladder Dysfunction Treatment
Aged or diseased tissues are not able to efficiently clear senescent cells, thereby resulting in their accumulation (64). Therefore, it is not surprising that recent studies support the therapeutic approach of targeting senescence for the treatment (144–146). Agents that prevent the activation of specific mechanisms of senescence, such as those involving telomerase, DNA-damage repair machinery, cell-cycle checkpoint kinases, and tumor suppressors, are all known to reduce indices of disease (69, 147). Similarly, the removal of senescent cells was recently shown to delay the onset of several age-related disease processes (72, 144).
As mentioned previously, LUTS is seen in up to half of diabetic patients and is associated with a significant negative impact on quality of life. With the increasing prevalence of diabetes, it is expected that complication of diabetes, such as DBD, is also on the rise. Further investigation into the association between urothelial senescence and DBD may lead to new therapeutic pathways. Senolytic therapy during the early stages of DBD may prevent further oxidative stress and possibly prevent progression of early stages of DBD to a later stage.
Agents that specifically target senescent cells for apoptosis represents a promising field of pharmacology and could be a more selective option for the treatment of DBD. To illustrate the impact of senescent cells on its surrounding tissue, one study showed that transplanting senescent ear chondrocytes into the knee joint region caused osteoarthritis-like condition in mice, while transplanting non-senescent cells did not (148, 149). A recent study reported that administration of two senolytics, dasatinib, and quercetin, reduced physical dysfunction induced by senescent cell transplantation and increased lifespan in old mice (149). Currently, senolytic therapy is used in some cancer treatments. For example, dasatinib is a senolytic used to treat certain forms of acute lymphoblastic leukemia and chronic myeloid leukemia (150). Other senolytics, such as navitoclax and UBX0101, are under clinical trials for the treatment of solid tumors and osteoarthritis, respectively. A recent article highlights the use of FOXO4-p53 interfering peptide, causing p53 nuclear exclusion in senescent cells and apoptosis, and resulting in protection against chemotoxicity and restoring the effects of age (151). Apoptosis of bladder senescent cells could decrease hypertrophy and prevent increased ROS and inflammation, both of which are characteristic of DBD. Interestingly, metformin is being evaluated as a SASP regulator for its effects on inhibiting the NF-κB pathway, ROS production, and inflammation (152, 153). The effects of metformin on urothelial senescence would be hard to elucidate; not only would metformin decrease plasma and urine glucose levels, it also acts as a SASP regulator. Further, treatment with sodium-glucose co-transporter-2 inhibitors would be an interesting positive control if increased urine glucose levels are responsible for increased urothelial senescence (154).
Conclusion
Despite recent advances in understanding DBD, the underlying pathways are poorly understood and therapeutic options are limited and not always effective, highlighting the need for novel interventions (13, 22). While cellular senescence is a normal physiological response to stress, uncontrolled senescence can be detrimental to human health in high accumulating numbers as evidenced in multiple age-related disease states, including diabetes. Numerous studies suggest that the increased presence of senescent cells leads to tissue dysfunction. In addition, urothelial barrier dysfunction is associated with symptoms of urgency and frequency. Therefore, we put forward the hypothesis that increased senescent urothelial cells in diabetic bladders contribute to symptoms of DBD. Because the urothelium plays an important role not only as a barrier to urine, but also as a sensor, identifying molecular mechanisms leading to senescence is essential. Additional work is needed to link the clinical symptoms of each state of DBD with the presence of cellular senescence within urinary bladder layers. Normal aging induced senescence and possible bladder dysfunctions associated with the aging process, as well as sex, hormonal status, diabetes duration, and reversal with treatment/exercise need to be investigated in coordination with bladder cellular senescence and DBD.
Ethics Statement
All animal procedures were performed in accordance with the Guide for the Care and Use of Laboratory Animals from the National Institutes of Health and were reviewed and approved by the Institutional Animal Care and Use Committee of Augusta University.
Author Contributions
NK designed the hypothesis, conducted experiments, and wrote the paper. CM contributed to and edited the paper. JV contributed to the initial research into the inflammation and oxidative stress section. SL researched, edited, and rewrote the section. JM wrote the clinical perspectives section, contributed to the section senescence as a novel target for diabetic bladder dysfunction treatment, and edited the paper. RW designed the hypothesis and edited the paper.
Funding
National Institutes of Health (HL134604) and Bridge-funding program, Augusta University. AHA Post-doctoral fellowship (18POST34060003).
Conflict of Interest Statement
The authors declare that the research was conducted in the absence of any commercial or financial relationships that could be construed as a potential conflict of interest.
Abbreviations
ATP, adenosine triphosphate; DBD, diabetic bladder dysfunction; CDK, cyclin-dependent kinase; COX, cyclooxygenase; GI, gastrointestinal; IC, interstitial cells; ICC, interstitial cells of Cajal; IL, interleukin; LUTS, lower urinary tract symptoms; M, muscarinic receptor; MMP, matrix metalloproteinases; MYD88, myeloid differentiation primary response 88; NF-κB, nuclear factor-kappa B; ROS, reactive oxygen species; SASP, senescence-associated secretory phenotype; SHH, sonic hedgehog; STZ, streptozotocin; TLR, toll-like receptors; TNF, tumor necrosis factor; VEGFA, vascular endothelial growth factor A; WNT, wingless-related integration site.
References
1. Andersson KE, Arner A. Urinary bladder contraction and relaxation: physiology and pathophysiology. Physiol Rev. (2004) 84:935–86. doi: 10.1152/physrev.00038.2003
2. Abrams P. New words for old: lower urinary tract symptoms for “prostatism”. BMJ (1994) 308:929–30. doi: 10.1136/bmj.308.6934.929
3. Foxman B. Epidemiology of urinary tract infections: incidence, morbidity, and economic costs. Am. J. Med. (2002) 113(Suppl. 1A):5–13S. doi: 10.1016/S0002-9343(02)01054-9
4. Andersson KE. Storage and voiding symptoms: pathophysiologic aspects. Urology (2003) 62:3–10. doi: 10.1016/j.urology.2003.09.030
5. Robertson C, Link CL, Onel E, Mazzetta C, Keech M, Hobbs R, et al. The impact of lower urinary tract symptoms and comorbidities on quality of life: the BACH and UREPIK studies. BJU Int. (2007) 99:347–54. doi: 10.1111/j.1464-410X.2007.06609.x
6. Sacco E, Tienforti D, D'addessi A, Pinto F, Racioppi M, Totaro A, et al. Social, economic, and health utility considerations in the treatment of overactive bladder. Open Access J. Urol. (2010) 2:11–24. doi: 10.2147/OAJU.S4166
7. Jeong WS, Choi HY, Nam JW, Kim SA, Choi BY, Moon HS, et al. Men with severe lower urinary tract symptoms are at increased risk of depression. Int Neurourol J. (2015) 19:286–92. doi: 10.5213/inj.2015.19.4.286
8. Ganz ML, Smalarz AM, Krupski TL, Anger JT, Hu JC, Wittrup-Jensen KU, et al. Economic costs of overactive bladder in the United States. Urology (2010) 75:526–32, 532.e1–18. doi: 10.1016/j.urology.2009.06.096
9. Irwin DE, Milsom I, Hunskaar S, Reilly K, Kopp Z, Herschorn S, et al. Population-based survey of urinary incontinence, overactive bladder, and other lower urinary tract symptoms in five countries: results of the EPIC study. Eur. Urol. (2006) 50, 1306–14; discussion: 1314–15. doi: 10.1016/j.eururo.2006.09.019
11. Harper S. Economic and social implications of aging societies. Science (2014) 346:587–91. doi: 10.1126/science.1254405
12. Tibaek S, Gard G, Klarskov P, Iversen HK, Dehlendorff C, Jensen R. Prevalence of lower urinary tract symptoms (LUTS) in stroke patients: a cross-sectional, clinical survey. Neurourol Urodyn. (2008) 27:763–71. doi: 10.1002/nau.20605
13. Daneshgari F, Liu G, Birder L, Hanna-Mitchell AT, Chacko S. Diabetic bladder dysfunction: current translational knowledge. J Urol. (2009) 182:S18–26. doi: 10.1016/j.juro.2009.08.070
14. Seftel AD, De La Rosette J, Birt J, Porter V, Zarotsky V, Viktrup L. Coexisting lower urinary tract symptoms and erectile dysfunction: a systematic review of epidemiological data. Int J Clin Pract. (2013) 67:32–45. doi: 10.1111/ijcp.12044
15. Akhavan-Sigari R, Abili M, Rohde V, Tezval H. The influence of skull base chordoma on lower urinary tract symptoms. Urology (2014) 83:756–61. doi: 10.1016/j.urology.2013.12.018
16. Mito Y, Yabe I, Yaguchi H, Tajima Y. Urinary dysfunction and motor symptoms in untreated Parkinson's disease. J Neurol Sci. (2016) 365:147–50. doi: 10.1016/j.jns.2016.04.031
17. Wyndaele JJ. The management of neurogenic lower urinary tract dysfunction after spinal cord injury. Nat Rev Urol. (2016) 13:705–14. doi: 10.1038/nrurol.2016.206
18. Aharony SM, Lam O, Corcos J. Evaluation of lower urinary tract symptoms in multiple sclerosis patients: review of the literature and current guidelines. Can Urol Assoc J. (2017) 11:61–4. doi: 10.5489/cuaj.4058
19. Samijn B, Van Laecke E, Renson C, Hoebeke P, Plasschaert F, Vande Walle J, et al. Lower urinary tract symptoms and urodynamic findings in children and adults with cerebral palsy: a systematic review. Neurourol Urodyn. (2017) 36:541–9. doi: 10.1002/nau.22982
20. Schumpf LF, Theill N, Scheiner DA, Fink D, Riese F, Betschart C. Urinary incontinence and its association with functional physical and cognitive health among female nursing home residents in Switzerland. BMC Geriatr. (2017) 17:17. doi: 10.1186/s12877-017-0414-7
21. Rave K, Nosek L, Posner J, Heise T, Roggen K, Van Hoogdalem EJ. Renal glucose excretion as a function of blood glucose concentration in subjects with type 2 diabetes–results of a hyperglycaemic glucose clamp study. Nephrol Dial Transplant. (2006) 21:2166–71. doi: 10.1093/ndt/gfl175
22. Brown JS, Wessells H, Chancellor MB, Howards SS, Stamm WE, Stapleton AE, et al. Urologic complications of diabetes. Diabetes Care (2005) 28:177–85. doi: 10.2337/diacare.28.1.177
23. Gibson W, Wagg A. Incontinence in the elderly, ‘normal’ ageing, or unaddressed pathology? Nat Rev Urol. (2017) 14:440–8. doi: 10.1038/nrurol.2017.53
24. Gupta S, Yang S, Cohen RA, Krane RJ, Saenz De Tejada I. Altered contractility of urinary bladder in diabetic rabbits: relationship to reduced Na+ pump activity. Am J Physiol. (1996) 271:C2045–C2052. doi: 10.1152/ajpcell.1996.271.6.C2045
25. Mutoh S, Latifpour J, Saito M, Weiss RM. Evidence for the presence of regional differences in the subtype specificity of muscarinic receptors in rabbit lower urinary tract. J Urol. (1997) 157:717–21. doi: 10.1016/S0022-5347(01)65257-1
26. Waring JV, Wendt IR. Effects of streptozotocin-induced diabetes mellitus on intracellular calcium and contraction of longitudinal smooth muscle from rat urinary bladder. J Urol. (2000) 163:323–30. doi: 10.1016/S0022-5347(05)68046-9
27. Disanto ME, Stein R, Chang S, Hypolite JA, Zheng Y, Zderic S, et al. Alteration in expression of myosin isoforms in detrusor smooth muscle following bladder outlet obstruction. Am J Physiol Cell Physiol. (2003) 285:C1397–C1410. doi: 10.1152/ajpcell.00513.2002
28. Su X, Changolkar A, Chacko S, Moreland RS. Diabetes decreases rabbit bladder smooth muscle contraction while increasing levels of myosin light chain phosphorylation. Am J Physiol Renal Physiol. (2004) 287:F690–F699. doi: 10.1152/ajprenal.00027.2004
29. Mannikarottu AS, Changolkar AK, Disanto ME, Wein AJ, Chacko S. Over expression of smooth muscle thin filament associated proteins in the bladder wall of diabetics. J Urol. (2005) 174:360–4. doi: 10.1097/01.ju.0000161602.18671.c7
30. Beshay E, Carrier S. Oxidative stress plays a role in diabetes-induced bladder dysfunction in a rat model. Urology (2004) 64:1062–7. doi: 10.1016/j.urology.2004.06.021
31. Changolkar AK, Hypolite JA, Disanto M, Oates PJ, Wein AJ, Chacko S. Diabetes induced decrease in detrusor smooth muscle force is associated with oxidative stress and overactivity of aldose reductase. J Urol. (2005) 173:309–13. doi: 10.1097/01.ju.0000141583.31183.7a
32. Pinna C, Zanardo R, Puglisi L. Prostaglandin-release impairment in the bladder epithelium of streptozotocin-induced diabetic rats. Eur J Pharmacol. (2000) 388:267–73. doi: 10.1016/S0014-2999(99)00833-X
33. Wang Z, Cheng Z, Cristofaro V, Li J, Xiao X, Gomez P, et al. Inhibition of TNF-alpha improves the bladder dysfunction that is associated with type 2 diabetes. Diabetes (2012) 61:2134–45. doi: 10.2337/db11-1763
34. Szasz T, Wenceslau CF, Burgess B, Nunes KP, Webb RC. Toll-like receptor 4 activation contributes to diabetic bladder dysfunction in a murine model of type 1 diabetes. Diabetes (2016) 65:3754–64. doi: 10.2337/db16-0480
35. Steers WD, Mackway-Gerardi AM, Ciambotti J, De Groat WC. Alterations in neural pathways to the urinary bladder of the rat in response to streptozotocin-induced diabetes. J Auton Nerv Syst. (1994) 47:83–94. doi: 10.1016/0165-1838(94)90069-8
36. Ueda T, Yoshimura N, Yoshida O. Diabetic cystopathy: relationship to autonomic neuropathy detected by sympathetic skin response. J Urol. (1997) 157:580–4. doi: 10.1016/S0022-5347(01)65209-1
37. Sasaki K, Chancellor MB, Phelan MW, Yokoyama T, Fraser MO, Seki S, et al. Diabetic cystopathy correlates with a long-term decrease in nerve growth factor levels in the bladder and lumbosacral dorsal root Ganglia. J Urol. (2002) 168:1259–64. doi: 10.1016/S0022-5347(05)64636-8
38. Chancellor MB. The overactive bladder progression to underactive bladder hypothesis. Int. Urol. Nephrol. (2014) 46(Suppl. 1), S23–7. doi: 10.1007/s11255-014-0778-y
39. Liu G, Lin YH, Li M, Xiao N, Daneshgari F. Temporal morphological and functional impact of complete urinary diversion on the bladder: a model of bladder disuse in rats. J Urol. (2010) 184:2179–85. doi: 10.1016/j.juro.2010.06.090
40. Xiao N, Wang Z, Huang Y, Daneshgari F, Liu G. Roles of polyuria and hyperglycemia in bladder dysfunction in diabetes. J Urol. (2013) 189:1130–6. doi: 10.1016/j.juro.2012.08.222
41. Birder L, Andersson KE. Urothelial signaling. Physiol Rev. (2013) 93:653–80. doi: 10.1152/physrev.00030.2012
42. Birder LA, De Groat WC. Mechanisms of disease: involvement of the urothelium in bladder dysfunction. Nat Clin Pract Urol. (2007) 4:46–54. doi: 10.1038/ncpuro0672
43. Birder LA. Urothelial signaling. Auton Neurosci. (2010) 153:33–40. doi: 10.1016/j.autneu.2009.07.005
44. Birder LA. Urinary bladder, cystitis and nerve/urothelial interactions. Auton Neurosci. (2014) 182:89–94. doi: 10.1016/j.autneu.2013.12.005
45. Khandelwal P, Abraham SN, Apodaca G. Cell biology and physiology of the uroepithelium. Am J Physiol Renal Physiol. (2009) 297:F1477–F1501. doi: 10.1152/ajprenal.00327.2009
46. Cheng SF, Jiang YH, Kuo HC. Urothelial dysfunction and chronic inflammation are associated with increased bladder sensation in patients with chronic renal insufficiency. Int Neurourol J. (2018) 22:S46–S54. doi: 10.5213/inj.1832814.407
47. Hanna-Mitchell AT, Ruiz GW, Daneshgari F, Liu G, Apodaca G, Birder LA. Impact of diabetes mellitus on bladder uroepithelial cells. Am J Physiol Regul Integr Comp Physiol. (2013) 304:R84–R93. doi: 10.1152/ajpregu.00129.2012
48. Wang CC, Kuo HC. Urothelial dysfunction and chronic inflammation in diabetic patients with overactive bladder. Low Urin Tract Symptoms (2017) 9:151–6. doi: 10.1111/luts.12126
49. Johnston L, Woolsey S, Cunningham RM, O'kane H, Duggan B, Keane P, et al. Morphological expression of KIT positive interstitial cells of Cajal in human bladder. J. Urol. (2010) 184, 370–377. doi: 10.1016/j.juro.2010.03.005
50. Koh BH, Roy R, Hollywood MA, Thornbury KD, Mchale NG, Sergeant GP, et al. Platelet-derived growth factor receptor-alpha cells in mouse urinary bladder: a new class of interstitial cells. J Cell Mol Med. (2012) 16:691–700. doi: 10.1111/j.1582-4934.2011.01506.x
51. Mccloskey KD, Gurney AM. Kit positive cells in the guinea pig bladder. J Urol. (2002) 168:832–6. doi: 10.1016/S0022-5347(05)64752-0
52. Sanders KM, Ward SM, Koh SD. Interstitial cells: regulators of smooth muscle function. Physiol Rev. (2014) 94:859–907. doi: 10.1152/physrev.00037.2013
53. Nakahara M, Isozaki K, Hirota S, Vanderwinden JM, Takakura R, Kinoshita K, et al. Deficiency of KIT-positive cells in the colon of patients with diabetes mellitus. J Gastroenterol Hepatol. (2002) 17:666–70. doi: 10.1046/j.1440-1746.2002.02756.x
54. Yamamoto T, Watabe K, Nakahara M, Ogiyama H, Kiyohara T, Tsutsui S, et al. Disturbed gastrointestinal motility and decreased interstitial cells of Cajal in diabetic db/db mice. J Gastroenterol Hepatol. (2008) 23:660–7. doi: 10.1111/j.1440-1746.2008.05326.x
55. Biers SM, Reynard JM, Doore T, Brading AF. The functional effects of a c-kit tyrosine inhibitor on guinea-pig and human detrusor. BJU Int. (2006) 97:612–6. doi: 10.1111/j.1464-410X.2005.05988.x
56. Johnston L, Cunningham RM, Young JS, Fry CH, Mcmurray G, Eccles R, et al. Altered distribution of interstitial cells and innervation in the rat urinary bladder following spinal cord injury. J Cell Mol Med. (2012) 16:1533–43. doi: 10.1111/j.1582-4934.2011.01410.x
57. Kim SO, Oh BS, Chang IY, Song SH, Ahn K, Hwang EC, et al. Distribution of interstitial cells of Cajal and expression of nitric oxide synthase after experimental bladder outlet obstruction in a rat model of bladder overactivity. Neurourol Urodyn. (2011) 30:1639–45. doi: 10.1002/nau.21144
58. Chen W, Jiang C, Jin X, Shen W, Song B, Li L. Roles of stem cell factor on loss of interstitial cells of Cajal in bladder of diabetic rats. Urology (2011) 78:1443.e1–6. doi: 10.1016/j.urology.2011.08.019
59. Vahabi B, Mckay NG, Lawson K, Sellers DJ. The role of c-kit-positive interstitial cells in mediating phasic contractions of bladder strips from streptozotocin-induced diabetic rats. BJU Int. (2011) 107:1480–7. doi: 10.1111/j.1464-410X.2010.09507.x
60. Kendig DM, Ets HK, Moreland RS. Effect of type II diabetes on male rat bladder contractility. Am J Physiol Renal Physiol. (2016) 310:F909–F922. doi: 10.1152/ajprenal.00511.2015
61. Liu G, Lin YH, Yamada Y, Daneshgari F. External urethral sphincter activity in diabetic rats. Neurourol Urodyn. (2008) 27:429–34. doi: 10.1002/nau.20543
62. Torimoto K, Fraser MO, Hirao Y, De Groat WC, Chancellor MB, Yoshimura N. Urethral dysfunction in diabetic rats. J Urol. (2004) 171:1959–64. doi: 10.1097/01.ju.0000121283.92963.05
63. Campisi J, D'adda Di Fagagna F. Cellular senescence: when bad things happen to good cells. Nat. Rev. Mol. Cell Biol. (2007) 8, 729–740. doi: 10.1038/nrm2233
64. Munoz-Espin D, Serrano M. Cellular senescence: from physiology to pathology. Nat Rev Mol Cell Biol. (2014) 15:482–96. doi: 10.1038/nrm3823
65. Munoz-Espin D, Canamero M, Maraver A, Gomez-Lopez G, Contreras J, Murillo-Cuesta S, et al. Programmed cell senescence during mammalian embryonic development. Cell (2013) 155:1104–18. doi: 10.1016/j.cell.2013.10.019
66. Storer M, Mas A, Robert-Moreno A, Pecoraro M, Ortells MC, Di Giacomo V, et al. Senescence is a developmental mechanism that contributes to embryonic growth and patterning. Cell (2013) 155:1119–30. doi: 10.1016/j.cell.2013.10.041
67. Coppe JP, Patil CK, Rodier F, Sun Y, Munoz DP, Goldstein J, et al. Senescence-associated secretory phenotypes reveal cell-nonautonomous functions of oncogenic RAS and the p53 tumor suppressor. PLoS Biol. (2008) 6:2853–68. doi: 10.1371/journal.pbio.0060301
68. Hayflick L, Moorhead PS. The serial cultivation of human diploid cell strains. Exp Cell Res. (1961) 25:585–621. doi: 10.1016/0014-4827(61)90192-6
69. Childs BG, Durik M, Baker DJ, Van Deursen JM. Cellular senescence in aging and age-related disease: from mechanisms to therapy. Nat Med. (2015) 21:1424–35. doi: 10.1038/nm.4000
70. Palmer AK, Tchkonia T, Lebrasseur NK, Chini EN, Xu M, Kirkland JL. Cellular senescence in type 2 diabetes: a therapeutic opportunity. Diabetes (2015) 64:2289–98. doi: 10.2337/db14-1820
71. Tchkonia T, Morbeck DE, Von Zglinicki T, Van Deursen J, Lustgarten J, Scrable H, et al. Fat tissue, aging, and cellular senescence. Aging Cell (2010) 9:667–84. doi: 10.1111/j.1474-9726.2010.00608.x
72. Baker DJ, Childs BG, Durik M, Wijers ME, Sieben CJ, Zhong J, et al. Naturally occurring p16(Ink4a)-positive cells shorten healthy lifespan. Nature (2016) 530:184–9. doi: 10.1038/nature16932
73. Donath MY. Targeting inflammation in the treatment of type 2 diabetes: time to start. Nat Rev Drug Discov. (2014) 13:465–76. doi: 10.1038/nrd4275
74. Sone H, Kagawa Y. Pancreatic beta cell senescence contributes to the pathogenesis of type 2 diabetes in high-fat diet-induced diabetic mice. Diabetologia (2005) 48:58–67. doi: 10.1007/s00125-004-1605-2
75. Sampson MJ, Winterbone MS, Hughes JC, Dozio N, Hughes DA. Monocyte telomere shortening and oxidative DNA damage in type 2 diabetes. Diabetes Care (2006) 29:283–9. doi: 10.2337/diacare.29.02.06.dc05-1715
76. Loots MA, Lamme EN, Mekkes JR, Bos JD, Middelkoop E. Cultured fibroblasts from chronic diabetic wounds on the lower extremity (non-insulin-dependent diabetes mellitus) show disturbed proliferation. Arch Dermatol Res. (1999) 291:93–9. doi: 10.1007/s004030050389
77. Minamino T, Orimo M, Shimizu I, Kunieda T, Yokoyama M, Ito T, et al. A crucial role for adipose tissue p53 in the regulation of insulin resistance. Nat Med. (2009) 15:1082–7. doi: 10.1038/nm.2014
78. Markowski DN, Thies HW, Gottlieb A, Wenk H, Wischnewsky M, Bullerdiek J. HMGA2 expression in white adipose tissue linking cellular senescence with diabetes. Genes Nutr. (2013) 8:449–56. doi: 10.1007/s12263-013-0354-6
79. Ksiazek K, Korybalska K, Jorres A, Witowski J. Accelerated senescence of human peritoneal mesothelial cells exposed to high glucose: the role of TGF-beta1. Lab Invest. (2007) 87:345–56. doi: 10.1038/labinvest.3700519
80. Yokoi T, Fukuo K, Yasuda O, Hotta M, Miyazaki J, Takemura Y, et al. Apoptosis signal-regulating kinase 1 mediates cellular senescence induced by high glucose in endothelial cells. Diabetes (2006) 55:1660–5. doi: 10.2337/db05-1607
81. Blazer S, Khankin E, Segev Y, Ofir R, Yalon-Hacohen M, Kra-Oz Z, et al. High glucose-induced replicative senescence: point of no return and effect of telomerase. Biochem Biophys Res Commun. (2002) 296:93–101. doi: 10.1016/S0006-291X(02)00818-5
82. Cramer C, Freisinger E, Jones RK, Slakey DP, Dupin CL, Newsome ER, et al. Persistent high glucose concentrations alter the regenerative potential of mesenchymal stem cells. Stem Cells Dev. (2010) 19:1875–84. doi: 10.1089/scd.2010.0009
83. Rolo AP, Palmeira CM. Diabetes and mitochondrial function: role of hyperglycemia and oxidative stress. Toxicol Appl Pharmacol. (2006) 212:167–78. doi: 10.1016/j.taap.2006.01.003
84. Lewis SA. Everything you wanted to know about the bladder epithelium but were afraid to ask. Am J Physiol R Physiol. (2000) 278:F867–F874. doi: 10.1152/ajprenal.2000.278.6.F867
85. Rickard A, Dorokhov N, Ryerse J, Klumpp DJ, Mchowat J. Characterization of tight junction proteins in cultured human urothelial cells. In Vitro Cell Dev Biol Anim. (2008) 44:261–7. doi: 10.1007/s11626-008-9116-y
86. Golbidi S, Laher I. Bladder dysfunction in diabetes mellitus. Front Pharmacol. (2010) 1:136. doi: 10.3389/fphar.2010.00136
87. Smith NJ, Hinley J, Varley CL, Eardley I, Trejdosiewicz LK, Southgate J. The human urothelial tight junction: claudin 3 and the ZO-1alpha(+) switch. Bladder (2015) 2:e9. doi: 10.14440/bladder.2015.33
88. Chromek M, Slamova Z, Bergman P, Kovacs L, Podracka L, Ehren I, et al. The antimicrobial peptide cathelicidin protects the urinary tract against invasive bacterial infection. Nat Med. (2006) 12:636–41. doi: 10.1038/nm1407
89. Nielsen KL, Dynesen P, Larsen P, Jakobsen L, Andersen PS, Frimodt-Moller N. Role of urinary cathelicidin LL-37 and human beta-defensin 1 in uncomplicated Escherichia coli urinary tract infections. Infect Immun. (2014) 82:1572–8. doi: 10.1128/IAI.01393-13
90. Spencer JD, Schwaderer AL, Becknell B, Watson J, Hains DS. The innate immune response during urinary tract infection and pyelonephritis. Pediatr Nephrol. (2014) 29:1139–49. doi: 10.1007/s00467-013-2513-9
91. Spencer JD, Schwaderer AL, Wang H, Bartz J, Kline J, Eichler T, et al. Ribonuclease 7, an antimicrobial peptide upregulated during infection, contributes to microbial defense of the human urinary tract. Kidney Int. (2013) 83:615–25. doi: 10.1038/ki.2012.410
92. Valore EV, Park CH, Quayle AJ, Wiles KR, Mccray PB Jr Ganz T. Human beta-defensin-1: an antimicrobial peptide of urogenital tissues. J Clin Invest. (1998) 101:1633–42. doi: 10.1172/JCI1861
93. Abraham SN, Miao Y. The nature of immune responses to urinary tract infections. Nat Rev Immunol. (2015) 15:655–63. doi: 10.1038/nri3887
94. Song J, Bishop BL, Li G, Grady R, Stapleton A, Abraham SN. TLR4-mediated expulsion of bacteria from infected bladder epithelial cells. Proc Natl Acad Sci USA. (2009) 106:14966–71. doi: 10.1073/pnas.0900527106
95. Shin K, Lee J, Guo N, Kim J, Lim A, Qu L, et al. Hedgehog/Wnt feedback supports regenerative proliferation of epithelial stem cells in bladder. Nature (2011) 472:110–4. doi: 10.1038/nature09851
96. Keay S, Leitzell S, Ochrzcin A, Clements G, Zhan M, Johnson D. A mouse model for interstitial cystitis/painful bladder syndrome based on APF inhibition of bladder epithelial repair: a pilot study. BMC Urol. (2012) 12:17. doi: 10.1186/1471-2490-12-17
97. Kiuchi H, Tsujimura A, Takao T, Yamamoto K, Nakayama J, Miyagawa Y, et al. Increased vascular endothelial growth factor expression in patients with bladder pain syndrome/interstitial cystitis: its association with pain severity and glomerulations. BJU Int. (2009) 104, 826–31; discussion: 831. doi: 10.1111/j.1464-410X.2009.08467.x
98. Shie JH, Kuo HC. Higher levels of cell apoptosis and abnormal E-cadherin expression in the urothelium are associated with inflammation in patients with interstitial cystitis/painful bladder syndrome. BJU Int. (2011) 108:E136–E141. doi: 10.1111/j.1464-410X.2010.09911.x
99. Sun Y, Chai TC. Augmented extracellular ATP signaling in bladder urothelial cells from patients with interstitial cystitis. Am J Physiol Cell Physiol. (2006) 290:C27–C34. doi: 10.1152/ajpcell.00552.2004
100. Zhang CO, Wang JY, Koch KR, Keay S. Regulation of tight junction proteins and bladder epithelial paracellular permeability by an antiproliferative factor from patients with interstitial cystitis. J Urol. (2005) 174:2382–7. doi: 10.1097/01.ju.0000180417.11976.99
101. Pitre DA, Ma T, Wallace LJ, Bauer JA. Time-dependent urinary bladder remodeling in the streptozotocin-induced diabetic rat model. Acta Diabetol. (2002) 39:23–7. doi: 10.1007/s005920200008
102. Godaly G, Bergsten G, Hang L, Fischer H, Frendeus B, Lundstedt AC, et al. Neutrophil recruitment, chemokine receptors, and resistance to mucosal infection. J Leukoc Biol. (2001) 69:899–906.
103. Shahin RD, Engberg I, Hagberg L, Svanborg Eden C. Neutrophil recruitment and bacterial clearance correlated with LPS responsiveness in local gram-negative infection. J Immunol. (1987) 138:3475–80.
104. Hannan TJ, Roberts PL, Riehl TE, Van Der Post S, Binkley JM, Schwartz DJ, et al. Inhibition of cyclooxygenase-2 prevents chronic and recurrent cystitis. EBioMedicine (2014) 1:46–57. doi: 10.1016/j.ebiom.2014.10.011
105. Jang TY, Kim YH. Interleukin-33 and mast cells bridge innate and adaptive immunity: from the allergologist's perspective. Int Neurourol J. (2015) 19:142–50. doi: 10.5213/inj.2015.19.3.142
106. Save S, Persson K. Extracellular ATP and P2Y receptor activation induce a proinflammatory host response in the human urinary tract. Infect Immun. (2010) 78:3609–15. doi: 10.1128/IAI.00074-10
107. Soruri A, Grigat J, Forssmann U, Riggert J, Zwirner J. beta-Defensins chemoattract macrophages and mast cells but not lymphocytes and dendritic cells: CCR6 is not involved. Eur J Immunol. (2007) 37:2474–86. doi: 10.1002/eji.200737292
108. Abraham SN, St John AL. Mast cell-orchestrated immunity to pathogens. Nat Rev Immunol. (2010) 10:440–52. doi: 10.1038/nri2782
109. Bouche C, Serdy S, Kahn CR, Goldfine AB. The cellular fate of glucose and its relevance in type 2 diabetes. Endocr Rev. (2004) 25:807–30. doi: 10.1210/er.2003-0026
110. Du XL, Edelstein D, Rossetti L, Fantus IG, Goldberg H, Ziyadeh F, et al. Hyperglycemia-induced mitochondrial superoxide overproduction activates the hexosamine pathway and induces plasminogen activator inhibitor-1 expression by increasing Sp1 glycosylation. Proc Natl Acad Sci USA. (2000) 97:12222–6. doi: 10.1073/pnas.97.22.12222
111. Geraldes P, King GL. Activation of protein kinase C isoforms and its impact on diabetic complications. Circ Res. (2010) 106:1319–31. doi: 10.1161/CIRCRESAHA.110.217117
112. Kolm-Litty V, Sauer U, Nerlich A, Lehmann R, Schleicher ED. High glucose-induced transforming growth factor beta1 production is mediated by the hexosamine pathway in porcine glomerular mesangial cells. J Clin Invest. (1998) 101:160–9. doi: 10.1172/JCI119875
113. Nishikawa T, Edelstein D, Du XL, Yamagishi S, Matsumura T, Kaneda Y, et al. Normalizing mitochondrial superoxide production blocks three pathways of hyperglycaemic damage. Nature (2000) 404:787–90. doi: 10.1038/35008121
114. Sayeski PP, Kudlow JE. Glucose metabolism to glucosamine is necessary for glucose stimulation of transforming growth factor-alpha gene transcription. J Biol Chem. (1996) 271:15237–43. doi: 10.1074/jbc.271.25.15237
115. Wautier JL, Schmidt AM. Protein glycation: a firm link to endothelial cell dysfunction. Circ Res. (2004) 95:233–8. doi: 10.1161/01.RES.0000137876.28454.64
116. Kanika ND, Chang J, Tong Y, Tiplitsky S, Lin J, Yohannes E, et al. Oxidative stress status accompanying diabetic bladder cystopathy results in the activation of protein degradation pathways. BJU Int. (2011) 107:1676–84. doi: 10.1111/j.1464-410X.2010.09655.x
117. Scherz-Shouval R, Shvets E, Fass E, Shorer H, Gil L, Elazar Z. Reactive oxygen species are essential for autophagy and specifically regulate the activity of Atg4. EMBO J. (2007) 26:1749–60. doi: 10.1038/sj.emboj.7601623
118. Yoshimura K, Shibata M, Koike M, Gotoh K, Fukaya M, Watanabe M, et al. Effects of RNA interference of Atg4B on the limited proteolysis of LC3 in PC12 cells and expression of Atg4B in various rat tissues. Autophagy (2006) 2:200–8. doi: 10.4161/auto.2744
119. Ebato C, Uchida T, Arakawa M, Komatsu M, Ueno T, Komiya K, et al. Autophagy is important in islet homeostasis and compensatory increase of beta cell mass in response to high-fat diet. Cell Metab. (2008) 8:325–32. doi: 10.1016/j.cmet.2008.08.009
120. Masini M, Lupi R, Bugliani M, Boggi U, Filipponi F, Masiello P, et al. A role for autophagy in beta-cell life and death. Islets (2009) 1:157–9. doi: 10.4161/isl.1.2.9372
121. Wang S, Sun QQ, Xiang B, Li XJ. Pancreatic islet cell autophagy during aging in rats. Clin Invest Med. (2013) 36:E72–E80. doi: 10.25011/cim.v36i2.19569
122. Bartolome A, Guillen C, Benito M. Autophagy plays a protective role in endoplasmic reticulum stress-mediated pancreatic beta cell death. Autophagy (2012) 8:1757–68. doi: 10.4161/auto.21994
123. Demirtas L, Guclu A, Erdur FM, Akbas EM, Ozcicek A, Onk D, et al. Apoptosis, autophagy & endoplasmic reticulum stress in diabetes mellitus. Indian J Med Res. (2016) 144:515–24. doi: 10.4103/0971-5916.200887
124. Las G., Shirihai O. S. (2010). The role of autophagy in beta-cell lipotoxicity and type 2 diabetes. Diabetes Obes. Metab. 12(Suppl. 2), 15–19. doi: 10.1111/j.1463-1326.2010.01268.x
125. Laberge RM, Sun Y, Orjalo AV, Patil CK, Freund A, Zhou L, et al. MTOR regulates the pro-tumorigenic senescence-associated secretory phenotype by promoting IL1A translation. Nat Cell Biol. (2015) 17:1049–61. doi: 10.1038/ncb3195
126. Suzuki E, Takahashi M, Oba S, Nishimatsu H. Oncogene- and oxidative stress-induced cellular senescence shows distinct expression patterns of proinflammatory cytokines in vascular endothelial cells. Sci World J. (2013) 2013:754735. doi: 10.1155/2013/754735
127. Weichhart T. mTOR as regulator of lifespan, aging, and cellular senescence: a mini-review. Gerontology (2018) 64:127–34. doi: 10.1159/000484629
128. Zeni L, Norden AGW, Cancarini G, Unwin RJ. A more tubulocentric view of diabetic kidney disease. J Nephrol. (2017) 30:701–17. doi: 10.1007/s40620-017-0423-9
129. Sivitz WI, Yorek MA. Mitochondrial dysfunction in diabetes: from molecular mechanisms to functional significance and therapeutic opportunities. Antioxid Redox Signal. (2010) 12:537–77. doi: 10.1089/ars.2009.2531
130. Freund A, Orjalo AV, Desprez PY, Campisi J. Inflammatory networks during cellular senescence: causes and consequences. Trends Mol Med. (2010) 16:238–46. doi: 10.1016/j.molmed.2010.03.003
131. Jurk D, Wilson C, Passos JF, Oakley F, Correia-Melo C, Greaves L, et al. Chronic inflammation induces telomere dysfunction and accelerates ageing in mice. Nat Commun. (2014) 2:4172. doi: 10.1038/ncomms5172
132. Ksiazek K, Passos JF, Olijslagers S, Von Zglinicki T. Mitochondrial dysfunction is a possible cause of accelerated senescence of mesothelial cells exposed to high glucose. Biochem Biophys Res Commun. (2008) 366:793–9. doi: 10.1016/j.bbrc.2007.12.021
133. Liu J, Huang K, Cai GY, Chen XM, Yang JR, Lin LR, et al. Receptor for advanced glycation end-products promotes premature senescence of proximal tubular epithelial cells via activation of endoplasmic reticulum stress-dependent p21 signaling. Cell Signal. (2014) 26:110–21. doi: 10.1016/j.cellsig.2013.10.002
134. Salazar G, Huang J, Feresin RG, Zhao Y, Griendling KK. Zinc regulates Nox1 expression through a NF-kappaB and mitochondrial ROS dependent mechanism to induce senescence of vascular smooth muscle cells. Free Radic Biol Med. (2017) 108:225–35. doi: 10.1016/j.freeradbiomed.2017.03.032
135. Sharpless NE, Sherr CJ. Forging a signature of in vivo senescence. Nat Rev Cancer (2015) 15:397–408. doi: 10.1038/nrc3960
136. Elstad EA, Taubenberger SP, Botelho EM, Tennstedt SL. Beyond incontinence: the stigma of other urinary symptoms. J Adv Nurs. (2010) 66:2460–70. doi: 10.1111/j.1365-2648.2010.05422.x
137. Tubaro A, Palleschi G. Overactive bladder: epidemiology and social impact. Curr Opin Obstet Gynecol. (2005) 17:507–11. doi: 10.1097/01.gco.0000183529.26352.52
138. Van Der Vaart CH, De Leeuw JR, Roovers JP, Heintz AP. The effect of urinary incontinence and overactive bladder symptoms on quality of life in young women. BJU Int. (2002) 90:544–9. doi: 10.1046/j.1464-410X.2002.02963.x
139. Gormley EA, Lightner DJ, Faraday M, Vasavada SP, American Urological A, Society Of Urodynamics FPM. Diagnosis and treatment of overactive bladder (non-neurogenic) in adults: AUA/SUFU guideline amendment. J Urol. (2015) 193:1572–80. doi: 10.1016/j.juro.2015.01.087
140. Kempler P, Amarenco G, Freeman R, Frontoni S, Horowitz M, Stevens M, et al. Management strategies for gastrointestinal, erectile, bladder, and sudomotor dysfunction in patients with diabetes. Diabetes Metab Res Rev. (2011) 27:665–77. doi: 10.1002/dmrr.1223
141. Ford AP, Gever JR, Nunn PA, Zhong Y, Cefalu JS, Dillon MP, et al. Purinoceptors as therapeutic targets for lower urinary tract dysfunction. Br. J. Pharmacol. (2006) 147(Suppl. 2), S132–43. doi: 10.1038/sj.bjp.0706637
142. Taylor JA IIIm Kuchel GA. Detrusor underactivity: Clinical features and pathogenesis of an underdiagnosed geriatric condition. J Am Geriatr Soc. (2006) 54:1920–32. doi: 10.1111/j.1532-5415.2006.00917.x
143. Van Koeveringe GA, Vahabi B, Andersson KE, Kirschner-Herrmans R, Oelke M. Detrusor underactivity: a plea for new approaches to a common bladder dysfunction. Neurourol Urodyn. (2011) 30:723–8. doi: 10.1002/nau.21097
144. De Keizer PL. The fountain of youth by targeting senescent cells? Trends Mol Med. (2017) 23:6–17. doi: 10.1016/j.molmed.2016.11.006
145. Kirkland JL, Tchkonia T. Clinical strategies and animal models for developing senolytic agents. Exp Gerontol. (2015) 68:19–25. doi: 10.1016/j.exger.2014.10.012
146. Tchkonia T, Zhu Y, Van Deursen J, Campisi J, Kirkland JL. Cellular senescence and the senescent secretory phenotype: therapeutic opportunities. J Clin Invest. (2013) 123:966–72. doi: 10.1172/JCI64098
148. Xu M, Bradley EW, Weivoda MM, Hwang SM, Pirtskhalava T, Decklever T, et al. Transplanted senescent cells induce an osteoarthritis-like condition in mice. J Gerontol A Biol Sci Med Sci. (2017) 72:780–5. doi: 10.1093/gerona/glw154
149. Xu M, Pirtskhalava T, Farr JN, Weigand BM, Palmer AK, Weivoda MM, et al. Senolytics improve physical function and increase lifespan in old age. Nat Med. (2018) 24:1246–56. doi: 10.1038/s41591-018-0092-9
150. Zhu Y, Doornebal EJ, Pirtskhalava T, Giorgadze N, Wentworth M, Fuhrmann-Stroissnigg H, et al. New agents that target senescent cells: the flavone, fisetin, and the BCL-XL inhibitors, A1331852 and A1155463. Aging (2017) 9:955–63. doi: 10.18632/aging.101202
151. Baar MP, Brandt RM, Putavet DA, Klein JD, Derks KW, Bourgeois BR, et al. Targeted apoptosis of senescent cells restores tissue homeostasis in response to chemotoxicity and aging. Cell (2017) 169:132.e16–147.e16. doi: 10.1016/j.cell.2017.02.031
152. Holman RR, Paul SK, Bethel MA, Matthews DR, Neil HA. 10-year follow-up of intensive glucose control in type 2 diabetes. N Engl J Med. (2008) 359:1577–89. doi: 10.1056/NEJMoa0806470
153. Martin-Montalvo A, Mercken EM, Mitchell SJ, Palacios HH, Mote PL, Scheibye-Knudsen M, et al. Metformin improves healthspan and lifespan in mice. Nat Commun. (2013) 4:2192. doi: 10.1038/ncomms3192
Keywords: lower urinary tract, senescence, urothelium, diabetic cystopathy, diabetic bladder
Citation: Klee NS, McCarthy CG, Lewis S, McKenzie JL, Vincent JE and Webb RC (2018) Urothelial Senescence in the Pathophysiology of Diabetic Bladder Dysfunction—A Novel Hypothesis. Front. Surg. 5:72. doi: 10.3389/fsurg.2018.00072
Received: 08 August 2018; Accepted: 12 November 2018;
Published: 04 December 2018.
Edited by:
Clemens Mathias Rosenbaum, University Medical Center Hamburg-Eppendorf, GermanyReviewed by:
Riccardo Campi, Università degli Studi di Firenze, ItalyMarco Roscigno, Azienda Socio-Sanitaria Territoriale Papa Giovanni XXIII, Italy
Copyright © 2018 Klee, McCarthy, Lewis, McKenzie, Vincent and Webb. This is an open-access article distributed under the terms of the Creative Commons Attribution License (CC BY). The use, distribution or reproduction in other forums is permitted, provided the original author(s) and the copyright owner(s) are credited and that the original publication in this journal is cited, in accordance with accepted academic practice. No use, distribution or reproduction is permitted which does not comply with these terms.
*Correspondence: Nicole S. Klee, nklee@augusta.edu