- 1Department of Business and Sociology, University of Extremadura, Avda de la Universidad s/n, Cáceres, Spain
- 2Department of Engineering, Universidad Popular Autónoma del Estado de Puebla, Puebla, Mexico
- 3Deanship of Graduate Programs in Engineering and Business, Universidad Popular Autónoma del Estado de Puebla, Puebla, Mexico
Introduction: Achieving an energy transition in the power industry in Mexico is a complex task. Despite being one of the most promising countries in Latin America and the world for developing wind and solar photovoltaic energy, energy and climate change efforts are insufficient; therefore, changes are generated slowly and leisurely. This article attempts to make a proposal based on the Quintuple Helix Model as an analytical and decision-making framework to encourage the production and consumption of clean/renewable electric energy and reduce GHG emissions. It proposes the sum of strategic interactions to promote a cooperation system and knowledge transfer, know-how, and innovation through the active and committed collaboration of government, academia, industry, civil society, and the environment to achieve the sustainable development of the electricity industry in Mexico.
Methods: These hypotheses are the result of the development of a singular methodology based on Partial Least Squares (PLS), according to Structural Equation Modeling (SEM). The results point out that the five-helix approach is valid to solve the energy transition problem in the electricity industry in Mexico.
Discussion: Although it is not fully developed and consolidated, it can be replicated in scenarios with similar socioeconomic characteristics. Furthermore, the government is the most opportune intermediary driving agent for the development of the energy transition in the electricity industry, since it is the one that can lead and drive the energy transition process by modifying the electricity sector through structural change in the energy market.
1. Introduction
Global social and economic development dependent on carbon-fossil fuel consumption generates excessive greenhouse gas (GHG) emissions, causing climate change and global warming (Chapman et al., 2018; UNEP, 2021). It negatively impacts lives, ecosystems, livelihoods, health, the economy, services, and the infrastructure of society (IPCC, 2014). CO2 emissions from electricity generation due to the burning of coal, oil, and gas make up a large share of overall GHG emissions driving the increase in global average temperature (Knaut et al., 2016). According to United in Science (2019), the scientific community estimates the global average temperature is 1.1°C above pre-industrial times (1,850–1,900) and 0.2°C higher than the period between 2011 and 2015 as a consequence of climate change and global warming. It is considered that the most important challenge facing humanity in the 21st century is to solve the problem of global warming through energy transition mechanisms (Miller et al., 2013).
The energy transition in the electric power industry requires a transformation of energy sources, technologies, production and consumption methods, actors, values, and governance (Huh et al., 2019). The electricity sector needs to change from a centralized to a decentralized system, where large companies are replaced by multiple small-scale generation and electricity networks (Defeuilley, 2019). Accordingly, the energy transition in the electric power sector is a change process that must simultaneously achieve multiple goals, such as decreasing GHG emissions from electric power generation; increasing the amount of electric power production from renewable sources; decreasing the price of electricity; achieving relatively simple, stable, and sustainable universal access to electricity; and providing a regular and permanent supply of electric power (Araújo, 2014; Singh et al., 2019; IRENA, 2020; World Economic Forum, 2021).
Mexico has the fourth largest share of fossil fuels in the energy matrix of the G20 countries, despite ranking third for the lowest electricity demand among them (Fulghum, 2021). It is one of the Latin American countries with the highest GHG emissions (Sánchez et al., 2018) as 75% of its electricity comes from fossil fuels (Fulghum, 2021). For Mexico, reducing GHG emissions and encouraging clean/renewable energy production and consumption is a priority. It is essential to reduce the vulnerability of its population, ecosystems, and infrastructure to climate change. Its geographic location in the tropics and between two oceans, as well as the poverty of its population make it highly sensitive to hydro-meteorological phenomena such as hurricanes, droughts, and landslides derived from the increase in temperature (INECC SEMARNAT, 2018). On the other hand, its geographical position and meteorological, topographical, and hydrological conditions make it one of the most promising countries in Latin America and the world for the development of wind and solar photovoltaic energy (SENER, 2016; IRENA, 2019b).
Likewise, Mexico is part of the international community that seeks to combat climate change, so it has signed international agreements such as the Sustainable Development Goals (Goal 13) and the Paris Agreement. In the latter, it ratified international commitments, therefore it is obliged to reduce 22% of GHG emissions by 2030 unconditionally and 36% conditionally. At the national level, given the relevance of the electricity sector in mitigating climate change and the fact that Mexico has a large number of renewable resources, in 2015 the Energy Transition Law (LTE) was published. It established as a goal a minimum participation of clean energy in the generation of electricity of 25% by 2018, 30% by 2021, and 35% by 2024 (Honorable Congreso de la Unión, 2015). It also generated a new regulatory framework to regulate the sustainable use of energy and allow all participants in the sector to coordinate long-term efforts to transition to the use of clean energy and reduce emissions at a lower cost.
Despite the existence of a favorable framework to incentivize the use of clean energy in Mexico, the current government (2018–2024) has proposed a constitutional counter-reform that reverses progress toward the implementation of climate change and energy transition policies. It curbs private investments in renewable electricity generation and the use of solar and wind plants to give dispatch priority to thermal power plants (Cámara de Diputados LXV Legislatura, 2021). This amendment to the Electricity Industry Law aims to return control of the electricity sector to the state-owned company, Federal Electricity Commission (CFE, for its acronym in Spanish) granting it at least 54% of the electricity market, prioritizing its power generation over private companies (Cámara de Diputados LXV Legislatura, 2021). This means that CFE electricity would be dispatched first despite being in most cases of fossil origin and more expensive than renewable energy generated by the private sector. A study published by the National Renewable Energy Laboratory (NREL) of the U.S. Department of Energy indicates that if this constitutional amendment is approved, carbon emissions will increase between 26 and 65%, and electricity generation costs between 32 and 54%, in addition to an increase in power outages (blackouts) of between 8 and 35% (Bracho et al., 2022); hindering the possibility of electricity generation at more competitive prices and less pollution.
Unfortunately, no route has been defined in Mexico to achieve energy and climate change objectives, therefore efforts are insufficient and changes in the energy industry are being generated slowly and leisurely. In this sense, the importance of this work lies in establishing a framework that will contribute to the strategic planning of investments, infrastructure, human capital, programs, and forms of interaction between the public, private, academic, and social sectors, and the natural environment. The aim is to generate a more efficient energy transition process in the electric power industry in Mexico through helix modeling that establishes a strategy that considers the adoption of new forms of energy production and consumption, as well as the attraction of private capital and the transfer of technology and knowledge to meet Mexico's energy needs.
The article sets out to offer a comprehensive approach based on Quintuple Helix as an analytical framework and decision-making for the sustainable development of the electric power industry in Mexico (Carayannis and Campbell, 2010; Barth, 2011; Carayannis et al., 2012, 2017; Taratori et al., 2021). An exploratory model is developed from the relationship of several constructs drawn from the literature to verify the validity of such a model applicable to similar transition processes.
This article is organized as follows. Section 2 briefly discusses the theoretical background of N-Helix Models and the Quintuple Helix modeling as an analytical framework to achieve the energy transition in the electricity sector in Mexico and the application of the methodology based on Partial Least Squares (PLS) according to structural equation modeling (SEM). Section 3 presents the results of the analysis. Section 4 critically discusses the findings. Finally, conclusions and future research lines will be summarized and drawn in Section 5.
2. Materials and methodology
2.1. N-Helix models
Particularly, given the relevance of the electric power industry in the mitigation of climate change and the fact that Mexico has a large number of renewable resources, it is necessary to design energy transition strategies (GREENPEACE, 2020). These should not only encourage environmental care and compliance with international agreements but also promote development, social sustainability, and the wellbeing of its population. In this sense, a helix analysis is highly effective as it fosters economic and social development (Taratori et al., 2021). It integrates industry, academia, government, society, and/or the natural environment as a system to produce science, technology, and innovation through the collaborative work of all agents, where the N-Helix model is a nexus (Guillén, 2018).
The Triple Helix Model identifies three helixes or subsystems: the state (political system), academia (educational system), and industry (economic system), focusing on the knowledge transfer and the interaction between the helixes (Etzkowitz and Leydesdorff, 2000). This model recognizes the importance of academia for innovation, emphasizing the production of knowledge and innovation in the economy, making it compatible with the knowledge economy (Carayannis and Campbell, 2010). The disadvantage it presents is the isolation and marginalization of the social sector (Etzkowitz and Leydesdorff, 2000).
The Quadruple Helix Model emerged when the Triple Helix Model was expanded by adding to the academia-industry-government relations the fourth helix, “media-based and culture-based public” (Carayannis and Campbell, 2009). This helix disseminates knowledge in a nation-state as well as culture with its values, traditions, lifestyles, experience, and visions promoting knowledge for the knowledge society. Civil society is not limited to using and applying knowledge and demanding innovation in goods and services, it is an active part of the innovation system (Barbosa, 2019). Therefore, this model of innovation and social cooperation is an important tool to provide solutions to problems, needs, and society's proposals (Ramirez and Palos-Sanchez, 2018; Robina-Ramirez et al., 2019).
The Quintuple Helix is a five-helix model of innovation that integrates and contextualizes the Triple Helix and the Quadruple Helix by adding the helix of the “environment” (natural environments) (Carayannis and Campbell, 2010). It offers an analytical framework in which knowledge and innovation are linked to the environment, addressing the relationships among government, academia, industry, civil society, and the environment (Carayannis and Campbell, 2010; Carayannis et al., 2012). This model aims to include the “natural environment” as a new subsystem in knowledge and innovation models (Barth, 2011). Environmental or ecological challenges such as global warming and issues related to the survival of humanity, tare drivers of new knowledge and innovations and have the potential to make society, economy, and democracy thrive (Carayannis et al., 2022). “Nature” is established as a central component of knowledge production and innovation, emphasizing the necessary socio-ecological transition of society, economy, and democracy in the 21st century (Carayannis et al., 2012).
This model focuses on the sum of social interactions to promote and visualize a system of cooperation and transfer of knowledge, know-how, and innovation, thus it can be used as an interdisciplinary analytical framework for decision-making and transdisciplinary problem-solving related to sustainable development and social ecology (Carayannis and Campbell, 2010; Barth, 2011). It is a theoretical and practical model offered to society to understand the link between knowledge and innovation to promote living in balance with nature and achieve lasting development, since “the environment should be considered as an active partner of innovation, not as a resource to be exploited” (Carayannis, 2020). It has been used in some projects to boost green employment, growth, and sustainability in the construction industry (Fundación Laboral de la Construcción, 2021), the sustainable development of the energy platform of the Russian Arctic zone (Carayannis et al., 2017), and the creation of remote control and smart metering systems for a water supplying system and social awareness and education on the use of these services (Taratori et al., 2021).
2.2. The challenge of energy transition in the electric power industry in a Quintuple Helix Model
For Mexico, it is crucial to implement a strategy to achieve the energy transition in the electricity industry. This proposal is based on the Quintuple Helix Model to evolve from a state of greenhouse gas (GHG) emissions due to the generation of electricity through the use of fossil fuels to one where electricity generation is carried out through renewable energy sources.
The Quintuple Helix supports the formation of a win-win situation between ecology, knowledge, and innovation, creating synergies between economy, society, and democracy (Carayannis et al., 2012). It can be successfully achieved through the active and committed cooperation of the various stakeholders (subsystems): 1. Political System (Government), 2. Education System (Academia), 3. Economic System (Industry), 4. Media-Based and Culture-Based Public (Civil Society), and 5. Natural Environment. Each of these five helixes (subsystems) has a special and necessary asset at its disposal, with a social and academic (scientific) relevance for its use, as indicated below.
2.2.1. Political system (government)
The political system or government establishes, organizes, and administers the general conditions of the state (nation-state). It formulates the objective of where the state is heading concerning the present and the future through laws, policies, projects, and plans (Barth, 2011; Carayannis et al., 2012; Sánchez-Hernández et al., 2020).
Government support for the energy transition in the electric power industry in Mexico is necessary since it establishes the legal framework, national objectives, and pricing policies, and makes strategic decisions to support the development of renewable energies (Vargas et al., 2016). Consequently, the development of energy policies integrated into economic, industrial, labor, educational, social, and environmental policies in favor of electricity production/consumption from renewable sources is mandatory (Hoekstra et al., 2017; IRENA, 2020). In parallel, financial support is a key factor for the success of renewable energy integration. The government can create the framework and necessary conditions to encourage this type of support and investments from public or private sources for the early stages of technology development, capital, and/or operational costs (Abdmouleh et al., 2015; Liu and Chu, 2019).
Similarly, the government can incentivize renewable energy development by establishing strategic plans and mechanisms such as green subsidies for clean and renewable energy and imposing carbon or energy taxes on conventional energy sources, to modify the levels of fossil energy production and consumption (Abdmouleh et al., 2015; Zhao et al., 2017). This contributes to improving the efficiency of capital allocation in the electricity sector because the externalities (costs imposed on society due to air pollution and climate change) derived from the use of fossil fuels are not fully valued in the electric energy price (Pikk and Viiding, 2014; Taylor, 2020). Likewise, it is of utmost importance to avoid the loss of electricity, which, can be caused by storage, transformation, transportation, and distribution, as well as administrative errors, anomalies in metering, self-connected customers, and electricity theft (Vargas et al., 2016; CEPAL, 2022). In addition, to raise awareness and encourage the participation of the population in the transformation of a low-carbon electricity sector, the government can carry out non-formal education and outreach programs and campaigns on climate change, promote sustainable consumption and the use of renewable energies, at federal, state, and municipal levels (Yanfei and Zhao, 2008; Kuzemko et al., 2016; INECC SEMARNAT, 2018). In accordance with the previous text, H1 is introduced:
H1: G-Government -> ET-Energy Transition
2.2.2. Education system (academia)
The education system refers to academia, universities, research centers, higher education systems, and other institutions focused on academic activities and contains human capital, i.e., students, teachers, scientists/researchers, and intellectual capital, that is, knowledge (Barth, 2011; Carayannis et al., 2012). Knowledge is the basis for economic development and social progress; it is crucial for value creation (Wehn and Montalvo, 2018), and technology is the set of knowledge and techniques, which, applied in a logical and orderly manner, allow human beings to modify their environment to meet their needs (Tabares Quiroz and Correa Vélez, 2014). Because of the growing need to reduce GHG emissions from electric power generation, research, knowledge creation, and technology generation are fundamental for making the shift to a sustainable electricity system (FAES, 2018; Lantz et al., 2021; Sandin and Benner, 2022). Universities and research centers are indispensable, as they are the main source of knowledge and technology generation. In this sense, the knowledge and technology transfer between academia and industry is indispensable for innovation as it allows the adaptation of scientific research results to their application in the marketing, sale, and use of goods and services to meet economic and social demands (Miśkiewicz, 2018; de Wit-de Vries et al., 2019; Thomas and Paul, 2019). Within this context, knowledge and technology transfer is a key element for the development of a low-carbon electricity sector (Fernandez Sanchez et al., 2016; Miśkiewicz, 2018; IRENA, 2020).
For its part, the development of human capital is indispensable for the success of the energy transition. In coordination with the government, strategic planning and collaboration between educational institutions and renewable energy industries are necessary for the development of integrated cooperative education, training, and learning systems to reduce mismatches between the demand and supply of skills (Lucas et al., 2018; Maier et al., 2019; IRENA, 2020). Having conveyed the previous ideas, the following hypotheses are explained:
H2: A-Academia -> G-Government
H3: A-Academia -> ET-Energy Transition
H4: A-Academia -> NE-Natural Environment
H5: A-Academia -> CS-Civil Society
H6: A-Academia -> I-Industry.
2.2.3. Economic system (industry)
This helix, also called industry, comprises firms, services, and industries, and therefore focuses on the economic or financial capital, that is, money, technology, services, products, etc. of a nation-state (Barth, 2011; Carayannis et al., 2012). The growing energy demand must be accompanied by increased investment in renewable energy to meet this demand, diversify the electricity supply, and thus reduce GHG emissions (Chapman et al., 2018). Energy infrastructure and distribution grid systems require large-scale investment because they are the basis for electrification, capacity expansion, energy flexibility, and demand management (Monitor Deloitte, 2021; BloombergNEF, 2022). Similarly, investments offer benefits to society such as sustainability, incentivizing competitiveness by contributing to the decrease in the price of electricity, increasing employment, and contributing to the development of the economy (Fragkos and Paroussos, 2018; IRENA, 2020). The deployment of renewable energies in the electric power industry generates direct, indirect, and induced jobs, which exceed the loss of jobs in conventional technologies. Jobs arise from activities related to electricity generation (renewable energies, energy efficiency, power grids, and energy flexibility) (Fragkos and Paroussos, 2018; IRENA, 2020).
In turn, innovation in the electric industry reduces costs and creates additional investment and business opportunities, in addition to making this sector a more efficient, competitive, and sustainable environment (DigitalES, 2019; IRENA, 2019c; United in Science, 2019). Innovation in areas such as grid digitalization, the Internet of Things (IoT), clean technologies, energy efficiency, electric energy storage, services, and business models, brings disruptive solutions that redefine and streamline the future of the low-carbon electricity industry (Kuzemko et al., 2016; Hoekstra et al., 2017; Olkkonen et al., 2017). In this sense, digitalization is a key factor for the transformation of the electricity sector, as it allows for managing large amounts of data, optimizing the electricity system, improving the performance and quality of service for consumers, and reducing costs and GHG emissions (IRENA, 2020; Torres and Eguia, 2020). It also facilitates the transformation to a system with decentralized renewable generation based on smart grids, increasing the number of connected devices, i.e., distributed generation (prosumers), smart meters, devices (IoT), etc. (Pacte industrial de la Regió Metropolitana de Barcelona, 2016; DigitalES, 2019). Therefore, the implementation of smart meters stands out, which allows the exchange of information regarding the state of the grid, reduces the time of supply interruption, and facilitates the active participation of the consumer in the electricity market by encouraging the use of renewable resources (Gil et al., 2017; DigitalES, 2019; Torres and Eguia, 2020). In connection with the previous text, the following hypotheses are introduced:
H7: I-Industry -> ET-Energy Transition
H8: I-Industry -> NE-Natural Environment
H9: I-Industry -> CS-Civil Society.
2.2.4. Media-based and culture-based public (civil society)
The subsystem of the media-based and culture-based public, known as civil society, integrates and combines social capital and information capital. On the one hand, it contains social capital through the culture-based public, i.e., traditions, lifestyles, customs, and values (Barth, 2011; Carayannis et al., 2012). This capital is important and intangible (Makridisid et al., 2021) and includes the connections between individuals, social networks, and forms of reciprocity and trust that improve the efficiency of society by facilitating coordinated actions (Messner et al., 2004; Kawachi, 2006; Yip et al., 2007). Societies with higher levels of social capital function better and are more democratic, safer, wealthier, healthier, happier, and less corrupt (Makridisid et al., 2021). On the other hand, it has information capital, such as news, social communication, etc. using public based on media, for example, television, radio, newspapers, etc. (Barth, 2011; Carayannis et al., 2012).
The transition to a sustainable electricity sector can be achieved if citizens are empowered as active agents of change and if citizen-centered action steps are taken (Pel et al., 2021; Wahlund and Palm, 2022). Energy citizenship is related to meaningful public engagement and increased awareness of the need for a rapid, just, and inclusive energy transition focused on behavioral change and ways of active participation of individuals in energy systems (Vanegas Cantarero, 2020; Beauchampet and Walsh, 2021). Active participation of citizens refers to individual practices such as the adoption of renewable technologies, energy efficiency measures, use of household energy technologies, joining energy communities, supporting local initiatives, participating in energy decision-making, etc. Similarly, the active participation of prosumers (consumers + producers) in energy production contributes to the decentralization of the energy system, encourages energy efficiency, and reduces GHG emissions (Hoekstra et al., 2017; IRENA, 2019a; Yang et al., 2019). The digitalization of the electric system makes it easier for consumers to know how their consumption habits affect their electricity bills, giving them the ability to control and optimize their energy consumption (IRENA, 2020; Pel et al., 2021). After having brought up those statements, the next hypotheses are conveyed:
H10: CS-Civil Society -> ET-Energy Transition
H11: CS-Civil Society -> G-Government
H12: CS-Civil Society -> NE-Natural Environment.
2.2.5. Natural environment
The natural environment provides natural capital, which is made available to the people (Barth, 2011). It also guides decisions and provides information about sustainable development (Carayannis et al., 2012). The natural environment is the space where the life of living beings develops; it is a system formed by natural and artificial elements that interrelate and are modified by human action (Shende et al., 2015). To encourage and raise awareness in society and governments about the importance of caring for the environment, the sustainable use of its natural resources, and the social good, several non-governmental organizations (NGOs) and associations have emerged (Robina-Ramirez et al., 2022). These organizations are committed to the defense of nature and the environment; therefore, they provide expert advice to the government (Kacar and Kartal, 2014; Roa and Alonso, 2015), i.e., secretariats, public institutions, city councils, politicians, etc. on various topics. In addition, they foster dialogue, agreements, and lobbying mechanisms to make the government comply with the commitments established in the constitution and/or international agreements and public policies to respond to the needs and demands of society (Bobadilla Díaz and Barreto Huamán, 2014; Servos and Servos, 2019).
NGOs and associations incentivize the transformation of the electric power industry and climate change mitigation. For example, distributed renewable energy sources projects provide local distributed employment integrating renewable energy needs with the interests of local communities contributing to ecology, sustainability of their economies, and encouraging self-consumption (Scholten and Bosman, 2016; Campos and Marín-González, 2020; IRENA, 2020). These challenges require citizen awareness and participation, which is why they carry out informative campaigns, conferences, debates, etc. through various mass media (Yanfei and Zhao, 2008; Kacar and Kartal, 2014; Roa and Alonso, 2015; Dai et al., 2017). Having conveyed the previous ideas, H13 and H14 are introduced:
H13: NE-Natural Environment -> ET-Energy Transition
H14: NE-Natural Environment -> G-Government.
2.3. Sample and population
According to Lepkowski (2008), the choice of the target sample was directed toward sectors directly related to the object of the sample. The respondents were recruited using purposive sampling (Roeters, 2014). For this purpose, several Mexican government agencies directly related to the electric power sector were selected, such as the Energy Regulatory Commission (CRE, for its acronym in Spanish), the National Commission for the Efficient Use of Energy (CONUEE, for its acronym in Spanish), the Ministry of Energy (SENER, for its acronym in Spanish), and the National Energy Control Center (CENACE, for its acronym in Spanish). In addition to incorporating these specific energy production bodies, we include the Federal Chamber of Deputies and the Senate as transmitter vehicles and connectors of the rest of the parts of the helix model, since in both chambers there are bodies representing the “Academia,” “Industry,” “Civil Society,” and “Natural-Environment.”
The distribution of employees in each of these bodies is as follows: CRE had 91 employees, CONEEE had 105 employees, SENER had 499 employees, CENACE had 639 employees, Federal Chamber of Deputies had 500 employees, and the Senate had 128 employees. In total there were 1,962 employees. In April, a letter explaining the objective of the investigation was sent to each of the presidents of the Commissions requesting their collaboration. The lack of response from some commissions led to a second, and sometimes a third letter, being sent. They were asked to send the final questionnaire by internal communication to all their employees, and a representation of 15 employees per Commission was asked to help carry out the pre-test of the study. The questionnaire was validated by 47 employees from all the commissions, accepting the indicators and making modifications to the wording of the questions in the final questionnaire. The questionnaires were sent out during the month of July. A total of 995 questionnaires were collected, representing 51% of the total sample. Table 1 shows the demographic variables such as gender, age, and education of the employees.
2.4. Selection of indicators
The Quintuple Helix Model as an analytical and decision-making framework was implemented through the involvement of the different stakeholders of each helix or subsystem in the electric power sector in Mexico, as follows: 1. Government: CRE, CENACE, CONUEE, SENER, Federal Chamber of Deputies and the Senate; 2. Academia: Researchers and academics from various universities, research centers, and educational institutions for technical professionals and high school technical professionals; 3. Industry: Staff from CFE and personnel from private electricity generation companies; 4. Civil Society: Consumers; and, 5. Natural Environment: Environmental NGOs and renewable energy associations. The conceptual model is formed by six constructs and twenty-four indicators, all of which were obtained from the literature and are summarized in Table 2.
2.5. PLS-SEM data methods
Partial least squares structural equation modeling (PLS-SEM) is a second-generation multivariate method that can be defined as a combination of simultaneous factor analysis and multiple regression analysis (Wong, 2013; Ravand and Baghaei, 2016). Its objective is to test the degree of fit of an observed data set to a hypothesized model represented through a plot of trajectories. This methodology has gained greater emphasis in recent years in various research areas because it overcomes the weaknesses of first-generation multivariate techniques, such as multiple regression, cluster analysis, or analysis of variance (Haenlein and Kaplan, 2004). It allows the simultaneous examination of a series of dependence relationships between independent and dependent variables (Gefen et al., 2000), between observed and latent variables, as well as between latent variables, and takes measurement error into account (Ravand and Baghaei, 2016). For the use of structural equation modeling (SEM), a strong theoretical foundation and justification of the studied phenomenon are required to achieve the specification of the dependence relationships and for the estimation of the proposed model (Mulaik, 2009). Therefore, SEM can be used to test theoretical assumptions with empirical data (Haenlein and Kaplan, 2004).
In SEM, two types or approaches can be found, the covariance-based (CB-SEM), recommended for testing, contrasting, or confirming theories, and the based-on variance or partial least squares method (PLS-SEM), which aims to maximize the explained variance of the dependent variables by adopting an ordinary least squares estimation method and is recommended when the objective is the prediction and development of new theories (Chin, 2010; Hair et al., 2011; Wong, 2013; Ravand and Baghaei, 2016). In addition, PLS-SEM is a more flexible modeling methodology since (1) it has no assumptions regarding the multivariate normality distribution of data because it is a non-parametric method; (2) it is robust to the presence of missing values; (3) it can use minimum sample sizes; (4) the number of items of each construct measured can be only one or more and in the relationships between latent constructs and their indicators (observable variables) reflective and formative measurement methods can be incorporated; (5) it assesses the reliability and validity of measurement models using various criteria; and (6) there is a high degree of statistical power (Dijkstra and Henseler, 2015; Ravand and Baghaei, 2016).
For the development of this model, the following decisions were made about the nature of the constructs and indicators involved in it: (1) the 5-helix model was modeled as a composite (Bollen, 2011; Bollen and Bauldry, 2011; Henseler, 2017) that can be estimated in Mode A or correlation weights (Henseler et al., 2014; Sarstedt et al., 2016), i.e., a composite whose indicators are expected to correlate with each other; (2) the presented model was measured across six reflexive common factor constructs (Henseler et al., 2016b), as they are behavioral constructs of the analyzed individuals (Henseler, 2017); and (3) given the nature of the indicators, they were constructed as reflexive common factor models, measurement models in which the variance of the indicators is assumed to be fully explained by the latent variable and random errors, these errors being uncorrelated with each other.
The evaluation of the measurement model and the structural model were developed using structural equation modeling (SEM), with a causal-predictive analysis approach (Shmueli et al., 2016; Hair et al., 2017, 2019). We used the multivariate PLS technique to process the information obtained from the questionnaires. Specifically, the study used the partial least squares (PLS) technique, through the Smart PLS V3 2.6 program. This version is especially recommended for composite models (Rigdon et al., 2017). This technique is ideal in social science analysis (Henseler, 2017) due to the precision of its predictions; this means that the model can be replicated in other settings (Carmines and Zeller, 1979).
2.6. Hypothesis and model
Based on the documentary compilation and the literature review which were carried out, a set of hypotheses were provisionally established as the basis of the research to respond in an alternative way and with a scientific basis to the problem of the energy transition in the electricity industry in Mexico. According to this series of assertions and the PLS-SEM methodology, a model was designed that proposes the sum of strategic interactions between government, academia, industry, civil society, and the natural environment to promote the sustainable development of the electricity sector in Mexico. The fourteen working hypotheses to be statistically validated are:
1) H1. The government (G) shapes the energy transition (ET).
2) H2. Academia (A) influences the government (G).
3) H3. Academia (A) shapes the energy transition (ET).
4) H4. Academia (A) affects the natural environment (NE).
5) H5. Academia (A) influences civil society (CS).
6) H6. Academia (A) affects the industry (I).
7) H7. The industry (I) shapes the energy transition (ET).
8) H8. Industry (I) influences the natural environment (NE).
9) H9. Industry (I) affects civil society (CS).
10) H10. Civil society (CS) shapes the energy transition (ET).
11) H11. Civil society (CS) influences the government (G).
12) H12. Civil society (CS) affects the natural environment (NE).
13) H13. The natural environment (NE) shapes the energy transition (ET).
14) H14. The natural environment (NE) influences the government (G).
Figure 1 shows the research model to convey the relationships among the constructs, indicators, and hypotheses.
3. Results
3.1. Measurement model results
In this section, we analyze the model's reliability and validity (Hair et al., 2017). The first one analyzes simple correlations of the measurements with their respective latent variables (≥0.7 was accepted; see Table 3). For this reason, all indicators (GHG emissions, price, taxes and subsidies, high-skilled human capital, green jobs, energy citizenship, and advising, among others) associated with a particular construct or latent variable (energy transition, government, academia, industry, civil society, or natural environment) are highly correlated with each other.
Table 4 shows the main parameters. Cronbach's alpha coefficient was used as a reliability index of the latent variables. In addition, the composite reliability was calculated. To measure validity, the mean-variance extracted (AVE), known as “convergent validity” (accepted > 0.5), was evaluated (see Table 4). The discriminant validity was verified using the Fornell-Larcker criterion (Fornell and Bookstein, 1982). This was accepted as the square root of the AVE of each item exceeded the correlations with the other latent variables.
Furthermore, according to Henseler et al. (2015), the best technique to detect the lack of discriminant validity is known as the heterotrait-monotrait ratio (HTMT). Henseler et al. (2015) proposed testing the correlations between variables using the HTMT parameter. Since all values are < 0.90, as observed in Table 5, this condition is accepted (Henseler, 2017). Table 5 reveals that the HTMT ratios for each pair of factors were < 0.90 (Henseler, 2017).
3.2. Structural model results
PLS-SEM aims to maximize the amount of variance explained through the coefficient of determination (R2). The structural evaluation of the model also analyzes the predictive relevance (Q2), the size, and the significance of the standardized regression coefficients or path coefficients. The basic algorithm of the PLS follows a two-step approach. The first step is the iterative estimation of the scores of the latent variables, and the second step is the final estimation of the weights, loads, and path coefficients using the estimation of ordinary least squares p-value of >0.05 (Henseler et al., 2015) (Table 6). Figure 2 shows the results of the PLS algorithm.
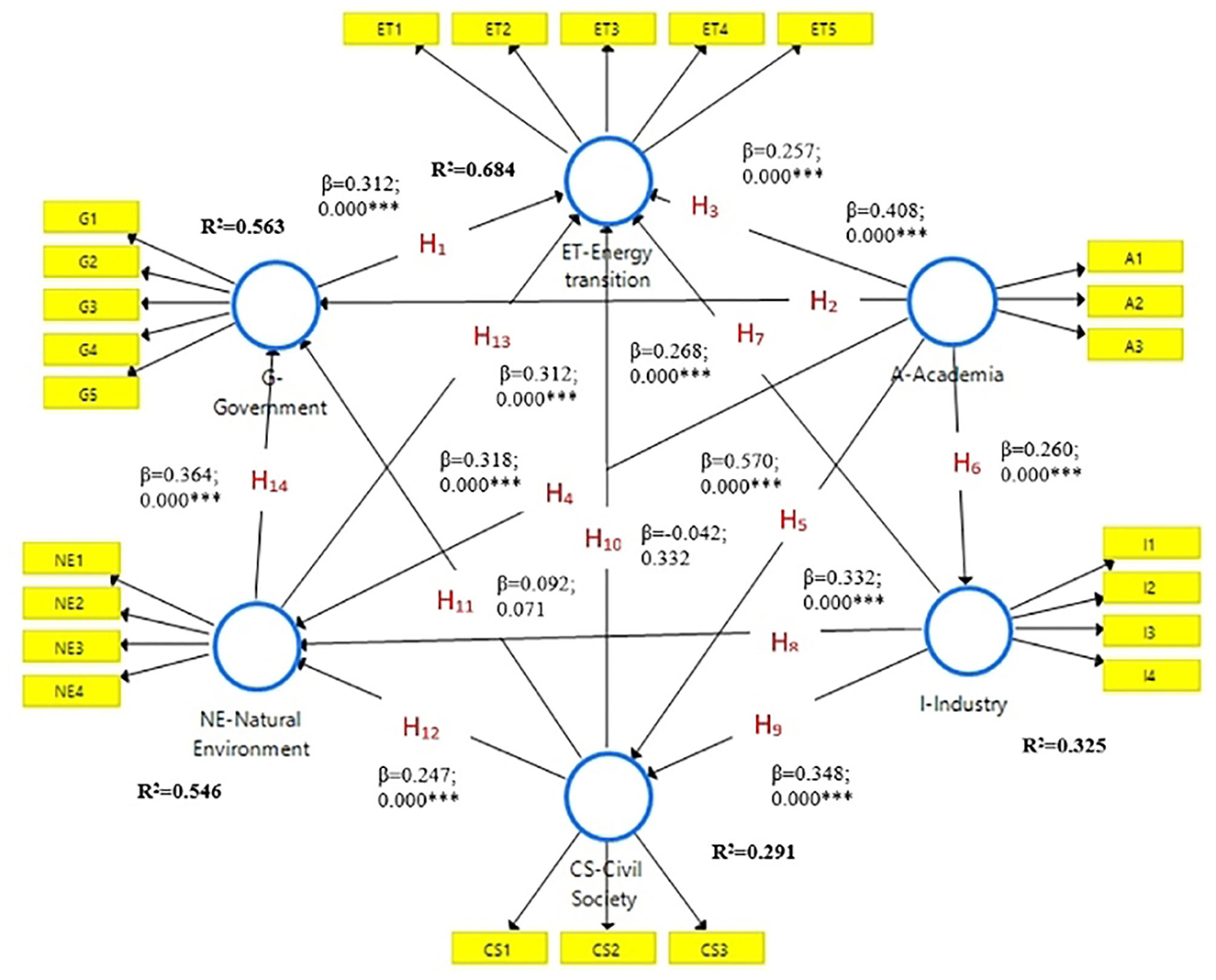
Figure 2. Results of the PLS algorithm. Statistical significance: p < 0.05; p < 0.01; p < 0.001; n.s.: not significant.
According to Henseler et al. (2016a), the best-fit criterion for the global model is the residual root mean square normalization (SRMR) (Hu and Bentler, 1998, 1999). A model with an adequate fit is considered when the values are <0.08. Therefore, a value of 0 for SRMR would indicate a perfect fit and, in general, an SRMR value <0.05 indicates an acceptable fit (Byrne, 2008). A recent simulation study shows that a correctly specified model implies SRMR values >0.06 (Henseler et al., 2016a). SRMR is 0.065, so the model is appropriate for the empirical data used (Hair et al., 2017).
The R2 values (see Table 7) obtained for the investigation led to the following conclusions: 0.67 = “Substantial”, 0.33 = “Moderate”, and 0.19 = “Weak” (Chin, 1998). The result obtained for the main dependent variable, Energy Transition (ET), in the intention to use the model (DCM) was R2= 68.4%.
From these data, it is clear that the model has a predictive capacity (Chin, 1998). Following Stone-Geisser (Q2) (Geisser, 1974; Stone, 1974), all endogenous constructions comply with Q2 > 0, as can be seen in Table 7. Hair et al. (2017) also establish values of 0.02 as small, values of 0.15 as medium, and values of 0.35 as large in their predictive validity of the model. In our case, all the values exceed the maximum threshold, ET, G, and NE indicate a high predictive relevance, while I and CS have an intermediate predictive relevance.
4. Discussion
The cooperation model proposed for the strategic interaction between industry, academia, government, society, and environment is highly significant R2 = 0.684 (Chin, 1998). This means that the model is valid for solving transdisciplinary problems related to sustainability and social ecology (Carayannis and Campbell, 2010; Barth, 2011). If the contribution of each of the elements in the variable “Energy Transition” is analyzed, it is observed in Table 8 as terms of the variance explained, the “Government” contributes to better explain the variable “Energy Transition” (Var = 0.234). Hence, our results suggest that the government may act as the driver of the energy transition, which is a common result in the literature as pointed out by Yanfei and Zhao (2008), Abdmouleh et al. (2015), Kuzemko et al. (2016), Vargas et al. (2016), Hoekstra et al. (2017), Zhao et al. (2017), INECC SEMARNAT (2018), Liu and Chu (2019), IRENA (2020), and CEPAL (2022). In other words, the government is the entity that can lead the energy transition process by modifying the electricity sector.
The “Industry” (Var = 0.188) and “Academia” (Var = 0.179) contribute to explaining the variable “Energy Transition” to a lesser degree. Our results indicate that the industry is a key factor for the development of the energy transition through innovation and digital solutions, adaptation to market trends, competitiveness, and generation of employment and social welfare, in accordance with Gil et al. (2017), Chapman et al. (2018), Fragkos and Paroussos (2018), DigitalES (2019), IRENA (2019c, 2020), Torres and Eguia (2020), Monitor Deloitte (2021), and BloombergNEF (2022). For its part, academia can provide and enhance education and information to foster a critical and participatory attitude toward climate change and the development of a sustainable electricity sector, as referred to by FAES (2018), Lucas et al. (2018), Miśkiewicz (2018), de Wit-de Vries et al. (2019), Maier et al. (2019), Thomas and Paul (2019), IRENA (2020), Lantz et al. (2021), and Sandin and Benner (2022).
Similarly, the other two variables with significant coefficient determinations are NE-Natural Environment R2 = 0.546 and G-Government R2 = 0.563. In the first case, the influence of “Industry” (Var = 0.211) and “Academia” (Var = 0.197) are determinants. According to Doh et al. (2012) and Abenoza et al. (2015), the industry has the capital and the influence of consumers. The knowledge, experience, and capabilities of industry and NGOs are different and complementary, together they can achieve more than they could alone. For its part, linking academia with NGOs in developing countries provides innovation in ideas, concepts, technologies, and projects, which, increases the impact, influence, and efficiency of NGOs and associations (MacLeod, 2009; Leege and Mcmillan, 2016). In the second case, the changes produced in the “Government” construct are explained thanks to the effect of “Academia” (Var = 0.276) and “Natural Environment” (Var = 0.243). According to authors Parker Gumucio (2014) and Vargas et al. (2016), academia provides expertise to the government for the development of policies, regulatory and investment frameworks, and the change toward a cleaner mode of production and consumption patterns. Likewise, NGOs and associations communicate and explain to the government the opinions, needs, and demands of society and ask for responses to them (Díaz and Bel, 2003; Roa and Alonso, 2015).
On the other hand, in terms of variance explained, “Civil Society” does not contribute to explaining the variable “Energy Transition” (V = −0.019), likewise, its influence on the variable “Government” is minimal (V = 0.044). These results can be explained by the fact that citizen participation in Mexico is very low in this type of issue due to several factors: the lack of information, the fact that most of these issues are solved by the citizens themselves due to low governmental performance, distrust in institutions, and because they do not feel represented, they consider it unnecessary to get more involved. Some others think that the solution to them is only the government's responsibility (Serrano Rodríguez, 2015; Del Tronco and Ramírez, 2021). However, “Civil Society” influences the variable “Natural Environment” (V = 0.138) since when citizens are members of associations, NGOs, etc. they tend to participate more in the solution of community problems (Del Tronco and Ramírez, 2021).
If the hypotheses are analyzed, twelve are significant and two are not. This indicates that the model, in general terms, has established relationships between constructs that are largely accepted by the large sample that participated in the study. Above all the hypotheses, the close relationship between the variables “Academia” and “Industry” (H6: A I, β: 0.570, t: 10.069) stands out. This relationship is grounded in the fact that the transfer of knowledge and technology between academia and industry creates an intangible network of support, which drives innovation, growth, and prosperity of the economy (de Wit-de Vries et al., 2019; Thomas and Paul, 2019). A key element for the development of a low-carbon power sector (Miśkiewicz, 2018; IRENA, 2020).
There is also a special significance to the relationship between “Academia” and “Government” (H2: A G, β: 0.531, t: 6.324). It is logical to think that academia provides scientific support, empirical evidence, and expert knowledge to the government on technological, social, and economic issues so that it can establish the legal framework, national objectives, and strategic decision-making necessary to incentivize the transformation of the electricity sector (Vargas et al., 2016; Glied et al., 2018).
The statistical analysis rejects hypotheses H10 (CS ET, β: 0.050, t: 0.970) and H11 (CS G, β: 0.182, t: 1.809). The poor relationship between the variables of the two hypotheses is explained because the participation of Mexican citizens in matters related to community problems is infrequent due to distrust in institutions, lack of information, and because they consider that public problems should be solved by the government (Serrano Rodríguez, 2015; Del Tronco and Ramírez, 2021).
In general, the statistical analysis indicates that in Mexico it is feasible to adopt a five-helix approach, but it is not fully developed, much less consolidated. So, returning to particular relationships:
• It is clear that the government plays a fundamental role in Mexico's energy transition. From the results, one could interpret the Mexican government as the intermediary driver. This contrasts with other countries where academia has driven this process (the Netherlands, for example). This would imply that the government should strengthen the structural change of the energy market by promoting the activities of CENACE, CRE, and CNH (National Hydrocarbons Commission, for its acronym in Spanish), but the opposite is currently happening. Possibly, with these results, it could be suggested that CENACE should promote the updating of energy infrastructure, CRE should encourage competition to increase consumer welfare, and CNH should promote technology transfer with academia.
• The work reflects the lack of social participation. This is an expected result because public policies focused on the consumer only reward those who consume less. In other words, the CFE promotes lower consumption but is not a substitution for clean electricity. There is also no clear strategy for people to produce energy. These types of public policies only benefit small and medium-sized companies. It is important to emphasize that social mechanisms for participation in the energy transition practically do not exist; those that do exist only integrate certain types of companies. Then, in addition to the subsidy for low consumption, Mexico could implement subsidies for the connection of solar panels to the grid or withdraw the subsidy received by the CFE to encourage the search for other suppliers (which exist but are not developed due to lack of demand).
• The network of technology transfer offices in Mexico is an initiative with an “appropriate origin”, but it has never been consolidated. Perhaps, due to the relationship between government and academia, it would be convenient to change the agent that controls them. Its administration should be transferred to academia, and the development of academic programs focused on energy transition should be promoted.
• Another point that can be discussed is the lack of development in the energy market. In recent years, it has returned to a monopolistic situation, instead of promoting short, medium, and long-term markets.
5. Conclusion
Due to the wealth of renewable resources in Mexico, as well as the urgent need to achieve the transformation of the electricity sector and the mitigation of climate change, an exploratory model based on the Quintuple Helix Model was proposed as an analytical and decision-making framework to promote the production and consumption of clean/renewable electricity and reduce GHG emissions. It proposes a cooperation system and transfer of knowledge, know-how, and innovation through the active and committed collaboration of government, academia, industry, civil society, and the environment through the sum of strategic interactions to achieve the sustainable development of the electricity industry in Mexico, as well as to provide relevant information to decision-makers in the energy transition of the electricity industry and similar transition processes.
This study concludes that the five-helix approach is valid to solve transdisciplinary problems related to sustainability and social ecology, in this case, to evolve from a state of greenhouse gas (GHG) emissions due to electricity generation through the use of fossil fuels to one where electricity generation is carried out through renewable energy sources and can be replicated in scenarios with similar socioeconomic characteristics. In Mexico, it is possible to adopt such an analytical and decision-making framework, however, it is not fully developed and consolidated. In addition, it became clear that the government is the most appropriate intermediary driving agent for the development of the energy transition in the electricity industry, i.e., the government is the one that can lead and drive the energy transition process by modifying the electricity sector.
Likewise, industry and academia are key players in achieving the energy transition. The importance of industry lies in the fact that innovation, competitiveness, the generation of green jobs, and social welfare are fundamental to encouraging the sustainable development of the electricity industry. Likewise, the collaboration of academia is indispensable since it is the main source of knowledge and technology generation, which are indispensable for the training of highly qualified human capital; advising the government for the development of policies, regulatory and investment frameworks, and the change toward a cleaner mode of production and consumption patterns, as well as facilitating and enhancing information to promote collective actions and a critical and participatory attitude of society to achieve the energy transition in the electricity industry.
In the particular case of Mexico, the civil society, namely consumers, do not contribute to the energy transition in the electricity industry, but as part of NGOs or associations, they participate and have the influence to achieve the transformation of the electricity sector. Consequently, in Mexican society, it is important to encourage citizen participation in matters related to community problems. The government must emphasize the creation of public policies and mechanisms that motivate civic involvement in social problems and decision-making processes and facilitate access to information to transform the prevailing civic culture. On the other hand, it is convenient that academia encourages the sensitization and awareness of the population on the importance of the change to a sustainable electric industry and climate change mitigation through its technical training programs, university, and postgraduate programs, as well as in basic and high school education programs.
5.1. Future research lines
The results drawn from the study have allowed us to understand the importance of developing other lines of research. It is suggested that future researchers explore a temporal approach to citizen participation in the energy transition in the electric power industry, that is, how citizen participation accelerates or delays the change to a sustainable electricity sector. It is also recommended that future researchers investigate, in detail, the current patterns of social participation in communal issues to know the type of mechanisms necessary to implement a changE to a low-carbon electricity sector and/or similar transition processes.
Data availability statement
The raw data supporting the conclusions of this article will be made available by the authors, without undue reservation.
Ethics statement
Ethical review and approval was not required for the study on human participants in accordance with the local legislation and institutional requirements. Written informed consent from the participants was not required to participate in this study in accordance with the national legislation and the institutional requirements.
Author contributions
VG-C conceived the ideas and designed the research with critical suggestions from RR-R. VG-C and RR-R collected, processed the data, and carried out the modeling. VG-C, RR-R, and D-EG-R analyzed the data and led the writing of the manuscript. D-EG-R and MS-O contributed critically to the drafts. All authors reviewed the manuscript and gave final approval for publication.
Conflict of interest
The authors declare that the research was conducted in the absence of any commercial or financial relationships that could be construed as a potential conflict of interest.
Publisher's note
All claims expressed in this article are solely those of the authors and do not necessarily represent those of their affiliated organizations, or those of the publisher, the editors and the reviewers. Any product that may be evaluated in this article, or claim that may be made by its manufacturer, is not guaranteed or endorsed by the publisher.
References
Abdmouleh, Z., Alammari, R. A., Gastli, A. (2015). Review of policies encouraging renewable energy integration and best practices. Renew and Susta Ene Rev. 45, 249–262.
Abenoza, S., Carreras, I., and Sureda, M. (2015). Colaboraciones ONG y empresa que transforman la sociedad. Barcelona: Instituto de Innovación Social; Universidad Ramón Llull.
Araújo, K. (2014). The emerging field of energy transitions: progress, challenges, and opportunities. Energy Res. Soc. Sci. 1, 112–121. doi: 10.1016/j.erss.2014.03.002
Barbosa, L. F. (2019). La cuarta hélice: ciudadanía en el sistema de innovación. Centro de Estudios de Ciencia, Comunicación y Sociedad, Centro de Estudios de Ciencia, Comunicación y Sociedad Universitat Pompeu Fabra Barcelona. Available online at: http://ccs.upf.edu/la-cuarta-helice-ciudadania-en-el-sistema-de-innovacion/ (accessed March 27, 2022).
Barth, T. D. (2011). The idea of a green new deal in a Quintuple Helix Model of knowledge, know-how and innovation. Int. J. Soc. Ecol. Sustain. Dev. 2, 1–14. doi: 10.4018/jsesd.2011010101
Beauchampet, I., and Walsh, B. (2021). Energy citizenship in the Netherlands: the complexities of public engagement in a large-scale energy transition. Energy Res. Soc. Sci. 76, 102056. doi: 10.1016/j.erss.2021.102056
Bobadilla Díaz, P., and Barreto Huamán, E. (2014). Las ONGs y el Estado en torno a las políticas sociales. Rev. Cienc. Soc. 6. doi: 10.31876/rcs.v6i3.25080
Bollen, K. A. (2011). Evaluating effect, composite, and causal indicators in structural equation models. MIS Q. Manag. Inf. Syst. 35, 359–372. doi: 10.2307/23044047
Bollen, K. A., and Bauldry, S. (2011). Three Cs in measurement models: causal indicators, composite indicators, and covariates. Psychol. Methods 16, 265–284. doi: 10.1037/a0024448
Bracho, R., Guerra Fernandez, O. J., Brancucci, C., Peluso, A., Alvarez Guerrero, J. D., and Flammini, M. (2022). Impacts Analysis of Amendments to Mexico's Unit Commitment and Dispatch Rules. Golden, CO: National Renewable Energy Laboratory. NREL/TP-6A50-81350. Available online at: https://www.nrel.gov/docs/fy22osti/81350.pdf (accessed July 16, 2022).
Byrne, B. M. (2008). Testing for multigroup equivalence of a measuring instrument: a walk through the process. Psicothema 20, 872–882.
Cámara de Diputados LXV Legislatura (2021). Iniciativa de Decreto por el que se reforman los artículos 25, 27 y 28 de la Constitución Política de los Estados Unidos Mexicanos. Available online at: http://gaceta.diputados.gob.mx/PDF/65/2021/oct/20211001-I.pdf#page=2 (accessed May 5, 2022).
Campos, I., and Marín-González, E. (2020). People in transitions: energy citizenship, prosumerism and social movements in Europe. Energy Res. Soc. Sci. 69. doi: 10.1016/j.erss.2020.101718
Carayannis, E. G. (2020). Democracy and the Environment Are Endangered Species, Interview with Dr. Prof. Elias Carayannis by Charlotte Koldbye. RiConfigur. Available online at: http://riconfigure.eu/wp-content/uploads/2020/01/Interview-with-Elias-Carayannis_2020_Final.pdf (accessed July 20, 2022).
Carayannis, E. G., Barth, T. D., and Campbell, D. F. J. (2012). The Quintuple Helix innovation model: global warming as a challenge and driver for innovation. J. Innovat. Entrepreneur. 1, 1–12. doi: 10.1186/2192-5372-1-2
Carayannis, E. G., and Campbell, D. F. J. (2009). “Mode 3” and “Quadruple Helix”: Toward a 21st century fractal innovation ecosystem. Int. J. Technol. Manag. 46, 201–234. doi: 10.1504/IJTM.2009.023374
Carayannis, E. G., and Campbell, D. F. J. (2010). Triple helix, quadruple helix and quintuple helix and how do knowledge, innovation and the environment relate to each other? A proposed framework for a trans-disciplinary analysis of sustainable development and social ecology. Int. J. Soc. Ecol. Sustain. Dev. 1, 41–69. doi: 10.4018/jsesd.2010010105
Carayannis, E. G., Campbell, D. F. J., and Grigoroudis, E. (2022). Helix trilogy: the triple, quadruple, and quintuple innovation helices from a theory, policy, and practice set of perspectives. J. Knowl. Econ. 13, 2272–2301. doi: 10.1007/s13132-021-00813-x
Carayannis, E. G., Cherepovitsyn, A. E., and Ilinova, A. A. (2017). Sustainable development of the Russian Arctic zone energy shelf: the role of the Quintuple Innovation Helix Model. J. Knowl. Econ. 8, 456–470. doi: 10.1007/s13132-017-0478-9
CEPAL (2022). Temas estadísticos de la CEPAL No 5. La energía de América Latina y el Caribe: acceso renovabilidad y eficiencia. Available online at: https://cepalstat-prod.cepal.org/cepalstat/tabulador/ConsultaIntegrada (accessed August 2, 2022).
Chapman, A. J., Mclellan, B. C., and Tezuka, T. (2018). Prioritizing mitigation efforts considering co-benefits, equity and energy justice: Fossil fuel to renewable energy transition pathways. doi: 10.1016/j.apenergy.2018.03.054
Chin, W. W. (1998). “The partial least squares approach for structural equation modeling,” in Modern Methods for Business Research, ed G. A. Marcoulides (Hoboken, NJ: Lawrence Ed.), 295–336.
Dai, J., Zeng, F., and Wang, Y. (2017). Publicity strategies and media logic: communication campaigns of environmental NGOs in China. Chin. J. Commun. 10, 38–53. doi: 10.1080/17544750.2016.1267024
de Wit-de Vries, E., Dolfsma, W. A., van der Windt, H. J., and Gerkema, M. P. (2019). Knowledge transfer in university–industry research partnerships: a review. J. Technol. Transfer 44, 1236–1255. doi: 10.1007/s10961-018-9660-x
Defeuilley, C. (2019). Energy transition and the future(s) of the electricity sector. Util. Policy J. 57, 97–105. doi: 10.1016/j.jup.2019.03.002
Del Tronco, J., and Ramírez, A. M. (2021). La democracia participativa en México: “compensa o profundiza la desigualdad política? Andamios 18, 171–203. doi: 10.29092/uacm.v18i,46.842
Díaz, J., and Bel, C. (2003). Las ongs y sus relaciones con la administración. Reflexiones para un debate. Papeles Geogr. 38, 77–102.
DigitalES (2019). La Digitalización En El Sector Energía: Transformación Tecnológica Y Energética. DigitalES Asociación Española para la Digitalización. Available online at: https://www.digitales.es/wp-content/uploads/2019/06/energia-y-digitalizacion.pdf (accessed May 05, 2022).
Dijkstra, T. K., and Henseler, J. (2015). Consistent partial least squares path modeling. MIS Q. 39, 297–316. doi: 10.25300/MISQ/2015/39.2.02
Doh, J., London, T., and Kilibarda, V. (2012). Building and Scaling a Cross-Sector Partnership: Oxfam America and Swiss Re Empower Farmers in Ethiopia, Oikos. Available online at: http://globalens.com/casedetail.aspx?cid=1429185http://www.oikos-international.org/academic/case-collection/ (accessed August 28, 2022).
Etzkowitz, H., and Leydesdorff, L. (2000). The dynamics of innovation: From National Systems and “mode 2” to a Triple Helix of university-industry-government relations. Res. Policy 29, 109–123. doi: 10.1016/S0048-7333(99)00055-4
FAES (2018). Claves de éxito de la transición energética. Available online at: https://fundacionfaes.org/file_upload/news/pdfs/20180306233756.pdf (accessed October 24, 2019).
Fernandez Sanchez, M. R., Sanchez-Oro, M., and Robina Ramirez, R. (2016). La evaluacion de la competencia digital en la docencia universitaria: el caso de los grados de empresariales y economicas. doi: 10.21501/22161201.1726
Fornell, C., and Bookstein, F. L. (1982). Two structural equation models: LISREL and PLS applied to consumer exit-voice theory. J. Market Res. 19, 440–452.
Fragkos, P., and Paroussos, L. (2018). Employment creation in EU related to renewables expansion. Appl. Energy 230, 935–945. doi: 10.1016/j.apenergy.2018.09.032
Fulghum, N. (2021). México lidera al G20 en la reducción de carbón el año pasado, pero tres cuartos de su electricidad aún provienen de combustibles fósiles. Ember, 14. Available online at: https://ember-climate.org/wp-content/uploads/2021/03/Global-Electricity-Review-2021-Mexico-Translated.pdf (accessed May 12, 2022).
Fundación Laboral de la Construcción (2021). Construye2020+. Available online at: http://construye2020plus.eu/ (accessed July 28, 2022).
Gefen, D., Straub, D., and Boudreau, M. C. (2000). structural equation modeling and regression: guidelines for research practice. Commun. Assoc. Inf. Syst. 4. doi: 10.17705/1CAIS.00407
Geisser, S. (1974). A predictive approach effect to the random model. Biometrika 61, 101–107. doi: 10.1093/biomet/61.1.101
Gil, J. B., Díaz, E. M., and Ríos, J. J. A. (2017). La seguridad de suministro eléctrico durante la transición energética. Cuadernos de energía. 53, 49–55.
Glied, S., Wittenberg, R., and Israeli, A. (2018). Research in government and academia: the case of health policy. Isr. J. Health Policy Res. 7, 1–8. doi: 10.1186/s13584-018-0230-3
Guillén, D. (2018). Cuádruple Hélice en la gestión territorial. Available online at: http://www.ciumataro.cat/wp-content/uploads/2018/03/Article-de-la-Regidora-Dolors-Guillén-Cuádruple-Hélice-en-la-gestión-territorial.pdf (accessed April 5, 2022).
Haenlein, M., and Kaplan, A. M. (2004). A beginner's guide to partial least squares analysis. Understand. Stat. 3, 283–297. doi: 10.1207/s15328031us0304_4
Hair Jr, J. F., Sarstedt, M., Hopkins, L., and Kuppelwieser, V. G. (2014). Partial least squares structural equation modeling (PLS-SEM): An emerging tool in business research. Eur. Bus. Rev. 26, 106–121.
Hair, J., Hollingsworth, C. L., Randolph, A. B., and Chong, A. Y. L. (2017). An updated and expanded assessment of PLS-SEM in information systems research. Ind. Manag. Data Syst. 117, 442–458. doi: 10.1108/IMDS-04-2016-0130
Hair, J. F., Ringle, C. M., Gudergan, S. P., Fischer, A., Nitzl, C., and Menictas, C. (2019). Partial least squares structural equation modeling-based discrete choice modeling: an illustration in modeling retailer choice. Bus. Res. 12, 115–142. doi: 10.1007/s40685-018-0072-4
Hair, J. F., Ringle, C. M., and Sarstedt, M. (2011). PLS-SEM: indeed a silver bullet. J. Market. Theory Pract. 19, 139–152. doi: 10.2753/MTP1069-6679190202
Henseler, J. (2017). Bridging design and behavioral research with variance-based structural equation modeling. J. Advert. 46, 178–192. doi: 10.1080/00913367.2017.1281780
Henseler, J., Dijkstra, T. K., Sarstedt, M., Ringle, C. M., Diamantopoulos, A., and Straub, D. W. (2014). Common beliefs and reality about PLS: comments on Rönkkö and Evermann. Org. Res. Methods 17, 182–209. doi: 10.1177/1094428114526928
Henseler, J., Hubona, G., and Ray, P. A. (2016a). Using PLS path modeling in new technology research: updated guidelines. Ind. Manag. Data Syst. 116, 2–20. doi: 10.1108/IMDS-09-2015-0382
Henseler, J., Ringle, C. M., and Sarstedt, M. (2015). A new criterion for assessing discriminant validity in variance-based structural equation modeling. J. Acad. Mark. Sci. 43, 115–135. doi: 10.1007/s11747-014-0403-8
Henseler, J., Ringle, C. M., and Sarstedt, M. (2016b). Testing measurement invariance of composites using partial least squares. Int. Market. Rev. 33, 405–431. doi: 10.1108/IMR-09-2014-0304
Hoekstra, A., Steinbuch, M., and Verbong, G. (2017). Creating agent-based energy transition management models that can uncover profitable pathways to climate change mitigation. Complexity. doi: 10.1155/2017/1967645
Honorable Congreso de la Unión (2015). Ley de Transición Energética. Available online at: https://www.diputados.gob.mx/LeyesBiblio/pdf/LTE.Pdf (accessed October 7, 2019).
Hu, L., and Bentler, P. M. (1998). Fit indices in covariance structure modeling: sensitivity to underparameterized model misspecification. Psychol. Methods 3, 424–453. doi: 10.1037/1082-989X.3.4.424
Hu, L., and Bentler, P. M. (1999). Cutoff criteria for fit indexes in covariance structure analysis: conventional criteria versus new alternatives. Struct. Eq. Model. 6, 1–55. doi: 10.1080/10705519909540118
Huh, T., Yoon, K.-Y., and Chung, I. R. (2019). Drivers and ideal types towards energy transition: anticipating the futures scenarios of OECD countries. Int. J. Environ. Res. Public Health 16. doi: 10.3390/ijerph16081441
INECC and SEMARNAT (2018). México, Sexta Comunicación Nacional y Segundo Informe Bienal de Actualización ante la Convención Marco de las Naciones Unidas sobre el Cambio Climático.
IPCC (2014). Climate Change 2014: Impacts, Adaptation, and Vulnerability. Part A: Global and Sectorial Aspects. Contribution of Working Group II to the Fifth Assessment Report of the Intergovernmental Panel on Climate Change. New York, NY: Cambridge University Press. Available online at: https://www.ipcc.ch/site/assets/uploads/2018/02/WGIIAR5-PartA_FINAL.pdf (accessed June 04, 2022).
IRENA (2019a). Global Energy Transformation: A Roadmap to 2050 (2019 Edn.). International Renewable Energy Agency.
IRENA (2019b). Renewable Capacity Statistics 2019. International Renewable Energy Agency (IRENA). Available online at: https://www.irena.org/publications/2019/Mar/Renewable-Capacity-Statistics-2019 (accessed June 12, 2022).
IRENA (2019c). Renewable Power Generation Costs in 2018, International Renewable Energy Agency. Abu Dhabi.
IRENA (2020). Global Renewables Outlook: Energy Transformation 2050. International Renewable Energy Agency. Available online at: https://www.irena.org/publications/2020/Apr/Global-Renewables-Outlook-2020 (accessed June 22, 2022).
Kacar, I., and Kartal, B. (2014). An outlook on social marketing campaigns of environmental NGOs. Eur. J. Sci. Res. 119, 438–451.
Kawachi, I. (2006). No Commentary: Social capital and health: making the connections one step at a time. Int. J. Epidemiol. 35, 989–993. doi: 10.1093/ije/dyl117
Knaut, A., Tode, C., Lindenberger, D., Malischek, R., Paulus, S., and Wagner, J. (2016). The reference forecast of the German energy transition-An outlook on electricity markets. Energy Policy 92, 477–491. doi: 10.1016/j.enpol.2016.02.010
Kuzemko, C., Lockwood, M., Mitchell, C., and Hoggett, R. (2016). Governing for sustainable energy system change: politics, contexts and contingency. Energy Res. Soc. Sci. 12, 96–105. doi: 10.1016/j.erss.2015.12.022
Lantz, T. L., Ioppolo, G., Yigitcanlar, T., and Arbolino, R. (2021). Understanding the correlation between energy transition and urbanization. Environ. Innovat. Soc. Transit. 40, 73–86. doi: 10.1016/j.eist.2021.06.002
Leege, D. M., and Mcmillan, D. E. (2016). Building more robust NGO-university partnerships in development: lessons learned from catholic relief services. J. Poverty Alleviat. Int. Dev. 7.
Lepkowski, J. (2008). Concepto sobre la población. in Metodología de la investigación. 6°. Mc Graw Hill.
Liu, P., and Chu, P. (2019). Renewables finance and investment: How to improve industry with private capital in China. J. Modern Power Syst. Clean Energ. 7, 1385–1398. doi: 10.1007/s40565-018-0465-6
Lucas, H., Pinnington, S., and Cabeza, L. F. (2018). Education and training gaps in the renewable energy sector. Solar Energy 173, 449–455. doi: 10.1016/j.solener.2018.07.061
Maier, S., Narodoslawsky, M., Borell-Damián, L., Arentsen, M., Kienberger, M., Bauer, W., et al. (2019). Theory and practice of European cooperative education and training for the support of energy transition. Energy Sustain. Soc. 9. doi: 10.1186/s13705-019-0213-4
Makridisid, C. A., Wuid, C., and Carey, W. P. (2021). How social capital helps communities weather the COVID-19 pandemic. PLoS ONE 16, e0245135. doi: 10.1371/journal.pone.0245135
Messner, S. F., Rosenfeld, R., and Baumer, E. P. (2004). Dimensions of social capital and rates of criminal homicide. Am. Sociol. Rev. 69, 882–903. doi: 10.1177/000312240406900607
Miller, C. A., Iles, A., and Jones, C. F. (2013). The social dimensions of energy transitions. Sci. Cult. 22, 135–148. doi: 10.1080/09505431.2013.786989
Miśkiewicz, R. (2018). The importance of knowledge transfer on the energy market. Polit. Energ. 21, 49–62. doi: 10.33223/epj/96208
Monitor Deloitte (2021). Connecting the Dots: Distribution Grid Investments to Power the Energy Transition.
Olkkonen, L., Korjonen-Kuusipuro, K., and Grönberg, I. (2017). Redefining a stakeholder relation: finnish energy “prosumers” as co-producers. Environ. Innovat. Soc. Transit. 24, 57–66. doi: 10.1016/j.eist.2016.10.004
Pacte industrial de la Regió Metropolitana de Barcelona (2016). Guía de Iniciativas Locales Hacia la Transición Energética en Los Polígonos Industriales.
Parker Gumucio, C. (2014). El mundo académico y las políticas públicas frente a la urgencia del desarrollo sustentable en América Latina y el Caribe. Polis 13, 175–201. doi: 10.4067/S0718-65682014000300009
Pel, B., Debourdeau, A., Kemp, R., Dumitru, A., and Schäfer, M. (2021). Proactive Strategies and Policies for Energy Citizenship Transformation. doi: 10.3030/101022492
Pikk, P., and Viiding, M. (2014). The dangers of marginal cost based electricity pricing. Balt. J. Econ. 13, 49–62. doi: 10.1080/1406099X.2013.10840525
Ramirez, R. R., and Palos-Sanchez, P. R. (2018). Environmental firms?f better attitude towards nature in the context of corporate compliance. Sustainability. 10, 3321. doi: 10.3390/su10093321
Ravand, H., and Baghaei, P. (2016). Partial least squares structural equation modeling with R. Pract. Assess. Res. Eval. 21, 11.
Rigdon, E. E., Sarstedt, M., and Ringle, C. M. (2017). On comparing results from CB-SEM and PLS-SEM: five perspectives and five recommendations. Marketing 39, 4–16. doi: 10.15358/0344-1369-2017-3-4
Roa, J. C., and Alonso, J. (2015). Las Organizaciones Civiles Mexicanas hoy. UNAM. Available online at: http://biblioteca.clacso.edu.ar/Mexico/ceiich-unam/20170426043823/pdf_1267.pdf (accessed August 14, 2022).
Robina-Ramirez, R., Fernandez-Portillo, A., and Diaz-Casero, J. C. (2019). Green start-ups?f attitudes towards nature when complying with the corporate law. Complexity. 2019, 1–17. doi: 10.1155/2019/4164853
Robina-Ramirez, R., Sanchez, M. S. O., Jimenez-Naranjo, H. V., and Castro-Serrano, J. (2022). Tourism governance during the COVID-19 pandemic crisis: A proposal for a sustainable model to restore the tourism industry. Environ. Develop. Sustain. 24, 6391–6412. doi: 10.1007/s10668-021-01707-3
Roeters, A., and Michalos, A. (2014). “Work stress,” in Encyclopedia of Quality of Life and Well-Being Research. p. 7196–7198.
Sánchez, L., Vásquez, C., Viloria, A., and Rodríguez Potes, L. (2018). Greenhouse Gases Emissions and Electric Power Generation in Latin American Countries in the Period 2006–2013, Lecture Notes in Computer Science (including subseries Lecture Notes in Artificial Intelligence and Lecture Notes in Bioinformatics). Springer International Publishing.
Sánchez-Hernández, M. I., Stankevičiūtė, Ž., Robina-Ramirez, R., and Díaz-Caro, C. (2020). Responsible job design based on the internal social responsibility of local governments. Int. J. Environ. Res. Public Health. 17, 3994. doi: 10.3390/ijerph17113994
Sandin, S., and Benner, M. (2022). Research evaluations for an energy transition? Insights from a review of Swedish research evaluation reports. Res. Eval. 31, 80–92. doi: 10.1093/reseval/rvab031
Sarstedt, M., Hair, J. F., Ringle, C. M., Thiele, K. O., and Gudergan, S. P. (2016). Estimation issues with PLS and CBSEM: Where the bias lies!. J. Bus. Res. 69, 3998–4010. doi: 10.1016/j.jbusres.2016.06.007
Scholten, D., and Bosman, R. (2016). The geopolitics of renewables; exploring the political implications of renewable energy systems? Technol. Forecast. Soc. Change 103, 273–280. doi: 10.1016/j.techfore.2015.10.014
SENER (2016). Inventario Nacional de Energías Limpias (INEL). Available online at: https://dgel.energia.gob.mx/inel/ (accessed August 2, 2021).
Serrano Rodríguez, A. (2015). La participación ciudadana en México. Estudios políticos (México). 34, 93–116.
Servos, C. M., and Servos, C. M. (2019). Las organizaciones no gubernamentales para el desarrollo (ONGD) en España. Rev. Int. Sociol. doi: 10.3989/ris.2000.i25.784
Shende, V. A., Janbandhu, K. S., and Patil, K. G. (2015). Impact of human beings on environment. Int. J. Res. Biosci. Agric. Technol. 23–28.
Shmueli, G., Ray, S., Estrada, J. M. V., and Chatla, S. B. (2016). The elephant in the room: predictive performance of PLS models. J. Bus. Res. 69, 4552–4564. doi: 10.1016/j.jbusres.2016.03.049
Singh, H. V., Bocca, R., Gomez, P., Dahlke, S., and Bazilian, M. (2019). The energy transitions index: an analytic framework for understanding the evolving global energy system. Energy Strat. Rev. 26. doi: 10.1016/j.esr.2019.100382
Stone, M. (1974). Cross-validation and multinomial prediction. Biometrika 61, 509–515. doi: 10.1093/biomet/61.3.509
Tabares Quiroz, J., and Correa Vélez, S. (2014). Tecnología y sociedad: una aproximación a los estudios sociales de la tecnología. Rev. CTS 26, 129–144.
Taratori, R., Rodriguez-Fiscal, P., Pacho, M. A., Koutra, S., Pareja-Eastaway, M., and Thomas, D. (2021). Unveiling the evolution of innovation ecosystems: an analysis of triple, quadruple, and quintuple helix model innovation systems in european case studies. Sustainability 13. doi: 10.3390/su13147582
Taylor, B. Y. M. (2020). Energy subsidies: Evolution in the global energy transformation to 2050. International Renewable Energy Agency.
Thomas, A., and Paul, J. (2019). Knowledge transfer and innovation through university-industry partnership: an integrated theoretical view. Knowl. Manag. Res. Pract. 17, 436–448. doi: 10.1080/14778238.2018.1552485
Torres, E. A., and Eguia, P. (2020). Proyecto : Digitalizacion “El valor de la digitalización en las redes eléctricas”.
UNEP (2021). The Production Gap Report 2021. SEI IISD ODI, E3G Available online at: www.productiongap.org (accessed March 1, 2022).
United in Science (2019). High-Level Synthesis Report of Latest Climate Science Information Convened by the Science Advisory Group of the UN Climate Action Summit 2019. Available online at: https://wedocs.unep.org/bitstream/handle/20.500.11822/30023/climsci.pdf?sequence=1&isAllowed=y (accessed November 6, 2019).
Vanegas Cantarero, M. M. (2020). Of renewable energy, energy democracy, and sustainable development: a roadmap to accelerate the energy transition in developing countries. doi: 10.1016/j.erss.2020.101716
Vargas, A., Saavedra, O. R., Samper, M. E., Rivera, S., and Rodriguez, R. (2016). Latin American energy markets: investment opportunities in nonconventional renewables. IEEE Power Energy Mag. 14, 38–47. doi: 10.1109/MPE.2016.2573862
Wahlund, M., and Palm, J. (2022). The role of energy democracy and energy citizenship for participatory energy transitions: a comprehensive review. Energy Res. Soc. Sc. 87, 102482. doi: 10.1016/j.erss.2021.102482
Wehn, U., and Montalvo, C. (2018). Knowledge transfer dynamics and innovation: behaviour, interactions and aggregated outcomes. J. Clean. Prod. 171, S56–S68. doi: 10.1016/j.jclepro.2016.09.198
Wong, K. K. K. (2013). Partial least squares structural equation modeling (PLS-SEM) techniques using SmartPLS. Market. Bull. 24, 1–32.
Yanfei, S., and Zhao, D. (2008). Environmental Campaigns. Popular Protest in China, 144–162. Available online at: https://www.researchgate.net/publication/309106419 (accessed June 29, 2022).
Yang, J., Zhao, J., Qiu, J., and Wen, F. (2019). A distribution market clearing mechanism for renewable generation units with zero marginal costs. IEEE Transact. Ind. Informat. 15, 4775–4787. doi: 10.1109/TII.2019.2896346
Yip, W., Subramanian, S. V., Mitchell, A. D, Lee, D. T. S, Wang, J., and Kawachi, I. (2007). Does social capital enhance health and well-being? Evidence from rural China. Soc. Sci. Med. 64, 35–49. doi: 10.1016/j.socscimed.2006.08.027
Keywords: energy transition, electric power industry, Quintuple Helix Model, renewable energies, sustainable development, climate change
Citation: González-Carrasco V, Robina-Ramírez R, Gibaja-Romero D-E and Sánchez-Oro Sánchez M (2023) The Quintuple Helix Model: Cooperation system for a sustainable electric power industry in Mexico. Front. Sustain. Energy Policy 1:1047675. doi: 10.3389/fsuep.2022.1047675
Received: 18 September 2022; Accepted: 21 December 2022;
Published: 16 February 2023.
Edited by:
Sanya Carley, Indiana University Bloomington, United StatesReviewed by:
Magdalena Wójcik-Jurkiewicz, Kraków University of Economics, PolandAntonio Sanchez-Bayon, Rey Juan Carlos University, Spain
Copyright © 2023 González-Carrasco, Robina-Ramírez, Gibaja-Romero and Sánchez-Oro Sánchez. This is an open-access article distributed under the terms of the Creative Commons Attribution License (CC BY). The use, distribution or reproduction in other forums is permitted, provided the original author(s) and the copyright owner(s) are credited and that the original publication in this journal is cited, in accordance with accepted academic practice. No use, distribution or reproduction is permitted which does not comply with these terms.
*Correspondence: Rafael Robina-Ramírez, cnJvYmluYUB1bmV4LmVz
†ORCID: Damián-Emilio Gibaja-Romero orcid.org/0000-0002-3536-4117
Marcelo Sánchez-Oro Sánchez orcid.org/0000-0002-0837-9372