- 1Stroke, Department of Acute Medicine, Nottingham University Hospitals NHS Trust, Nottingham, United Kingdom
- 2Stroke, Academic Unit of Mental Health and Neuroscience, University of Nottingham, Nottingham, United Kingdom
- 3Department of Neurology, Boston Medical Centre, Boston University Chobanian and Avedisian School of Medicine, Boston, MA, United States
- 4Department of Stroke, University Hospitals Birmingham NHS Foundation Trust, Birmingham, United Kingdom
- 5College of Dental and Medical Sciences, Institute of Applied Health Research, University of Birmingham, Birmingham, United Kingdom
- 6Department of Medicine, Faculty of Medicine, National University of Malaysia, Kuala Lumpur, Malaysia
- 7Department of Neurosurgery, Nottingham University Hospitals NHS Trust, Nottingham, United Kingdom
- 8Department of Stroke, Derby Royal NHS Foundation Trust, Derby, United Kingdom
- 9Stroke Research in Stoke, Institute of Science and Technology, Keele University, Stoke-on-Trent, United Kingdom
- 10Department of Clinical Pharmacology, School of Medicine, Medical Sciences and Nutrition, University of Aberdeen, Aberdeen, United Kingdom
- 11Institute of Cardiovascular and Medical Sciences, University of Glasgow, Glasgow, United Kingdom
- 12Department of Brain Repair and Rehabilitation, UCL Stroke Research Centre, London, United Kingdom
- 13UCL Institute of Neurology, National Hospital for Neurology, National Hospital for Neurology and Neurosurgery, London, United Kingdom
Acute intracerebral hemorrhage is the most devastating stroke subtype and is associated with significant morbidity and mortality. Poor prognosis is associated with primary brain injury from the presenting hematoma, and despite advances in clinical trials of evacuation or reducing expansion, management is largely limited to supportive care and secondary prevention. Recent research has led to a better understanding of the pathophysiology of the cerebral edema surrounding the hematoma (perihematomal edema) and the identification of treatment targets and potential interventions. Some therapies have progressed to testing in phase 2 and 3 clinical trials, while novel agents are in development. This review focuses on the pathogenesis of perihematomal edema and its natural history and summarizes the results of potential interventions including preclinical and clinical studies. This review also lists the gaps in the current knowledge and suggests directions for future trials of perihematomal edema that could potentially change clinical practice.
Introduction
Spontaneous intracerebral hemorrhage (ICH) accounts for approximately 20% of all strokes and affects about 3 million patients worldwide each year (GBD 2019 Stroke Collaborators, 2019). ICH is devastating, with a mortality of ~40% at 1 month, and more than two-thirds of survivors remain dependent, requiring long-term care (van Asch et al., 2010; Krishnamurthy et al., 2020). Compared to advances in the management of acute ischemic stroke, the treatment for ICH lags significantly. Poor prognosis is associated with the hematoma size, location, and intraventricular hemorrhage. Hence, randomized clinical trials have assessed limiting the hemorrhage size and expansion through hemostasis, lowering blood pressure (BP), and surgery (Broderick et al., 1993; Mendelow et al., 2005, 2013; Anderson et al., 2008; Investigators, 2015; NCT02880878, 2023). Except for surgical evacuation of a hematoma in a highly selected group of patients (Ratcliff et al., 2023), no other intervention has been shown to improve functional outcomes. Therefore, the management of ICH remains largely supportive. Survivors of ICH are at risk of death or severe disability due to the cerebral edema that surrounds the hematoma (perihematomal edema [PHE]) (Venkatasubramanian et al., 2011; Balami and Buchan, 2012; Yang J. et al., 2015; Hurford et al., 2019). In a large-volume ICH, the accompanying swelling can increase the mass effect and lead to brain herniation (Zazulia et al., 1999). Hence, PHE is emerging as a key component of secondary brain injury and a potential surrogate outcome measure for preclinical and clinical trials (Venkatasubramanian et al., 2011; Yang Z. et al., 2015). Understanding the mechanisms and natural history of PHE is therefore important, and the development of potential therapeutic agents is of interest to clinical trialists and researchers.
The pathogenesis of PHE and accompanying brain injury are not yet fully understood but include vasogenic, cytotoxic, inflammatory, and oxidative mechanisms accompanied by disruption of the blood-brain barrier (BBB) (Figure 1). In this narrative review, we focus on these mechanisms, the natural history of PHE, neuroimaging measures, and laboratory parameters that may be associated with the pathogenesis, and summarize potential interventions, including data from translational and clinical studies. We also list the gaps in the current knowledge and suggest directions for future trials of cerebral edema that could potentially change clinical practice.
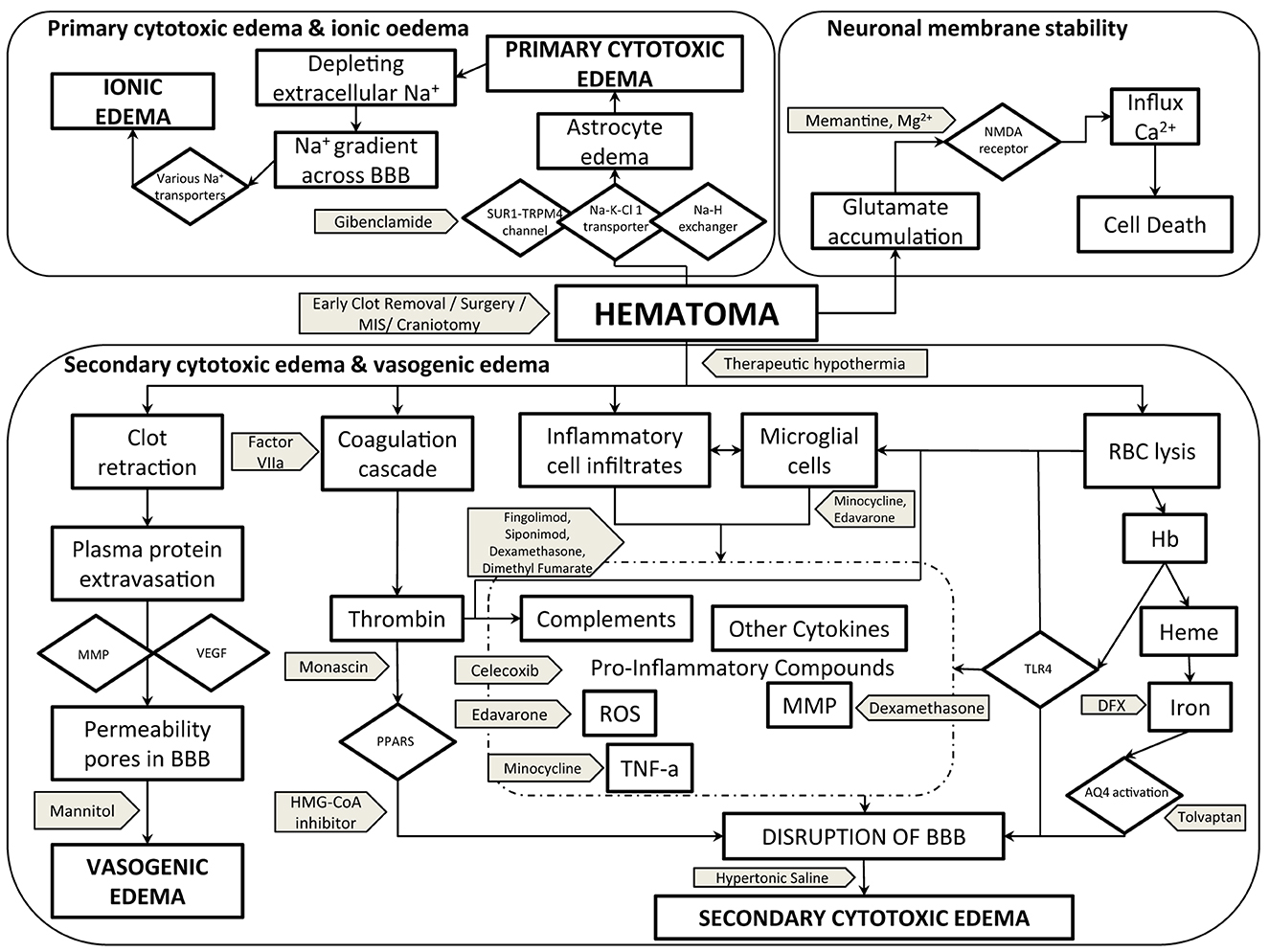
Figure 1. Pathogenesis of PHE cerebral edema with potential therapeutic targets and interventions. AQ4, aquaporin-4; BBB, blood-brain barrier; Ca2+, calcium ion; DFX, deferoxamine; Hb, hemoglobin; Heme, HMG-CoA-3-hydroxy-3-methylglutaryl-coenzyme A; MIS, minimal invasive surgery; MMP, matrix metalloproteinase; Mg2+, magnesium ion; Na+, sodium ion; Na-H exchanger, sodium–hydrogen exchanger; Na-K-Cl-1 transporter, sodium–potassium–chloride-1 transporter; NMDA, N-methyl-D-aspartate; PPAR, peroxisome profilerator-activated receptor; RBC, red blood cells; ROS, reactive oxygen species; SUR1-TRPM4 channel, sulfonylurea receptor-1–transient receptor potential melastatin 4 channel; TNF-α, tissue necrosis factor–alpha; TLR4, toll-like receptor-4; VEGF, vascular endothelial growth factor.
Natural history and pathogenesis of cerebral edema
The precise natural history of PHE is unclear, but it is thought to evolve over three phases.
The hyperacute phase
This phase of edema begins within the first hours of ictus, with the mechanism being vasogenic from activation of the coagulation cascade, leading to a retraction of the presenting hematoma (Xi et al., 1998), a decrease in hydrostatic pressure in the PHE region, and an exudation of serum plasma proteins (Reulen et al., 1977). The oncotic pressure gradient generated by the exudated proteins leads to increased brain swelling from the movement of water into the perihematomal space (Wagner et al., 1996). Such changes can be identified visually in the first few hours of stroke on a plain computed tomography (CT) or magnetic resonance imaging (MRI) scan as an area of low attenuation or high T2 or fluid attenuation inversion recovery signal surrounding the hematoma, respectively (Enzmann et al., 1981; Linfante et al., 1999). Preclinical studies have shown that the BBB is intact for the first few hours during this period and that permeability gradually increases (Yang et al., 1994; Xi et al., 1998). Therefore, a change in PHE volume during this phase is likely to be mediated by hematoma contraction and the pressure gradients generated by plasma proteins (Wagner et al., 1996).
As retraction of the hematoma continues, there is a further increase in PHE. Large swelling could increase intracranial pressure and decrease blood flow to the perihematomal region if the collateral circulation is not recruited and able to compensate. Research has shown that the perihematomal region is in a state of reduced metabolism (“hibernation”) and that autoregulation is preserved in the first few hours after ictus (Kim-Han et al., 2006; Ironside et al., 2019).
In addition to vasogenic edema, there is cellular/cytotoxic swelling because of a failure of the energy-dependent ion channel sodium-potassium adenosine triphosphatase (Na+K+ATPase) (Kahle et al., 2009). Na+K+ATPase is responsible for maintaining the transmembrane electrochemical balance and failure or dysfunction leads to excess influx of intracellular sodium and water (Kahle et al., 2009). Studies indicate that the sulfonyl-receptor-1–transient receptor potential melastatin 4 (SUR1-TRPM4) channel is also involved (Simard et al., 2006). Once activated, SUR1-TRPM4 not only leads to water accumulation and ionic dysfunction but also mediates BBB damage and the lining of endothelial cells (Simard et al., 2006, 2009). As cytotoxic edema continues, more water enters the brain through aquaporin-4 channels (AQP4), which are located on astrocytes (Qing et al., 2009). The activation of AQP4 induces water influx to compensate for the ionic imbalance from failure of Na+K+ATPase (Pollay et al., 1983). Studies suggest that the opening of AQP4 is linked to SUR1-TRPM4 (Stokum et al., 2018).
Cytotoxic edema is also potentiated through activation of the Na+-K+Cl cotransporter, which is a membrane protein on brain endothelial cells and excess extracellular glutamate after stroke (Shuaib et al., 2003). Glutamate is an important neurotransmitter and is normally released into the neuronal synaptic cleft (Collingridge et al., 2013). Its reuptake occurs at the presynaptic terminals and adjacent glial cells. In acute ICH, excess glutamate binds to N-methyl D aspartate (NMDA) receptors on postsynaptic neurons, which, in turn, facilitates the uncontrolled entry of calcium, causing apoptosis or death (Zheng et al., 2016; Ironside et al., 2019). Furthermore, glutamate can bind to other receptors facilitating an influx of sodium and water (Kritis et al., 2015).
It is important to highlight that vasogenic and cytotoxic edema are not exclusive and that the mechanisms are interlinked (Ironside et al., 2019).
The intermediate phase
The next phase of PHE continues over the next 24–48 h of ictus when the volume has been shown to increase and could reach the maximum (Olivot et al., 2010; Parry-Jones et al., 2015; Zheng et al., 2016). Studies have reported that this time window could be longer and the variation could be explained in the timing of follow-up scans and measures used to assess edema (Arima et al., 2009; Venkatasubramanian et al., 2011; Parry-Jones et al., 2015). Observational data indicate that edema in lobar ICH can increase quickly in the first few days and reach a larger volume than deep ICH (Gebel et al., 2002; Grunwald et al., 2016; Wu T. Y. et al., 2017). This could be because lobar regions in the brain have more space and fewer adjacent tightly packed white matter tracts compared to deep ICH, so there is less resistance to extending the swelling (Grunwald et al., 2016).
Pathophysiologically, the intermediate phase is characterized by events including the breakdown of the hematoma, the activation of thrombin, inflammation, and microglial/macrophage activation (Xi et al., 2003; Zhao et al., 2007a, 2015a; Keep et al., 2012). This results in damage to the BBB, which, in turn, increases inflammation by promoting leukocyte infiltration, ionic imbalance, and the entry of toxic substances into the brain interstitium. Studies have shown that the increased permeability of the BBB is facilitated by cytotoxic edema and ionic failure that occurred in the hyperacute phase (Reulen et al., 1977; Kahle et al., 2009). There are a number of molecules and signaling pathways involved in the intermediary phase, and the key components are discussed.
Thrombin
The immediate response to intracerebral hemorrhage is activation of the coagulation cascade to limit bleeding and here, thrombin has an important role. However, experimental studies have shown that in high concentrations as in acute stroke, thrombin induces edema independent of fibrinogen and causes the release of nitric oxide and cytokines, including tumor necrosis factor–alpha (TNF-α), interleukin (IL)-12, and IL-6 (Xi et al., 1998, 2003). In addition, thrombin promotes recruitment and infiltration of neutrophils, lymphocytes, and macrophages into the perihematomal region by inducing chemotactic substances and adhesion molecules (Xi et al., 2003). Thrombin also directly stimulates microglia through specific proteinase-activated receptors (PARs) (Chen et al., 2022).
The activation of thrombin can cause an opening of the BBB through PARs and damage the basement membrane by activating metalloproteinases (MMPs) (Chen et al., 2022). MMPs are proteolytic enzymes, which degrade components of the basement membrane, including collagen, gelatin, laminin, and fibronectin. Thrombin can also trigger activation of Src kinase (a proto-oncogene protein with kinase activity), which, in turn, potentiates damage to astrocytes and endothelial cells, the main cellular component of the BBB (Xi et al., 2003).
Neuroinflammation
In response to thrombin activation and damage to the BBB, leukocytes enter the perihematomal region. Studies have shown that the activation of a specific receptor, toll-like receptor 4 (TLR-4), on the surface of neutrophils and macrophages is important for infiltration (Yang J. et al., 2015). The activation of TLR-4 begins within hours after a stroke and continues for about a week (Yang J. et al., 2015). By inducing the expression of a transcription factor, nuclear factor–kappa B (NF-κB), on microglia and macrophages, TLR-4 signaling also stimulates the release of pro-inflammatory cytokines (e.g., IL-1, IL-6, TNF-α, and IL-β), signaling pathways generating free radicals, and glutamate (Sansing et al., 2007; Yang J. et al., 2015).
Neutrophils are the earliest to reach the perihematomal region, and studies suggest that the peak activity is at ~72 h. Neutrophils seem to contribute to cerebral edema by generating reactive oxygen species (ROS) and pro-inflammatory protease enzymes that damage the BBB (Wang and Dore, 2007). The activation of microglia occurs later, and studies have shown this to continue for ~2 weeks (Zheng et al., 2020). The inflammation mediated by microglia is driven by its M1 phenotype through the release of cytokines, chemokines, ROS, and nitric oxide (Wang and Dore, 2007). The activity of the M1 phenotype is maintained by activated astrocytes and lymphocytes (T1 helper cells) (Tschoe et al., 2020). At ~1 week of ictus, the M1 phenotype converts to the M2 phenotype, and this begins the process of resolution of inflammation, scar formation, and brain repair (Wu J. et al., 2017). The conversion from the M1 to the M2 phenotype is potentiated by anti-inflammatory cytokines (IL-4, IL-10, IL-13), lymphocyte T2 helper cells and a number of transcription factors (Chhor et al., 2013). Hence, the effects of microglia after ICH are either pro-inflammatory or neuroprotective and time-dependent. The anti-inflammatory effect of the M2 phenotype has led to testing of potential treatments, which is discussed later.
The disruption to the BBB also leads to the activation of the complement system in the perihematomal region (Lee et al., 1995). Both complement C3a and C5a are anaphylatoxins and induce endothelial cells and infiltration of pro-inflammatory cells (Lee et al., 1995; Holste et al., 2021). Complement activation also leads to the formation of the membrane attack complex (MAC), which causes erythrolysis. MAC is known to be directly toxic to neurons, glial cells, astrocytes, and the BBB (Holste et al., 2021).
The late phase
Although erythrolysis begins early after ICH, the late phase of edema is mainly medicated by the release of toxic products, including hemoglobin and iron, and phagocytosis (Ironside et al., 2019).
Hemoglobin has been shown to inhibit the enzyme Na+K+ATPase and induce the release of ROS and peroxidation of membrane lipids, leading to neuronal death (Bautista et al., 2021). Haem has been shown to activate microglia through TLR-4, and this, in turn, can induce NF-κB through a complex system of gene expression or signaling pathways (Tschoe et al., 2020). This process leads to more release of inflammatory cytokines and brain injury. (Tschoe et al., 2020).
Following the breakdown of the hematoma, iron is first observed in the perihematomal region at day 1, reaching peak level at approximately 7 days, and can continue to remain at that level for approximately 2 weeks (Qing et al., 2009). There is significant evidence that excess iron leads to brain damage (Xi et al., 2002). One mechanism is through the release of toxic free radicals (“ferroptosis”) (Bautista et al., 2021). Experimental studies have also shown that iron induces inflammation by generating ROS and MMP-9 and BBB dysfunction and increases cytotoxic swelling by activating AQP4 (Qing et al., 2009).
Phagocytosis leads to brain repair and is mediated by the infiltrated microglia and macrophages in the perihematomal region (Galloway et al., 2019). Phagocytosis includes the following steps: anchoring, internalization, and, finally, processing of the products of hematoma breakdown (Hochreiter-Hufford and Ravichandran, 2013). The process itself is complex and regulated by multiple receptors and enzymes that are present on the surface of the microglia and macrophage itself (Hochreiter-Hufford and Ravichandran, 2013; Galloway et al., 2019). As phagocytosis continues, ROS and toxic free radicals are generated, which can lead to more neurotoxicity (Hu et al., 2016). Preclinical studies have shown that accelerating phagocytosis could limit the period of exposure of the brain to toxic products and lead to recovery (Zhao et al., 2007a, 2015a).
Hormones, peptides, and toxic free radicals
It is suggested that, after ICH, excess levels of the antidiuretic hormone vasopressin induce edema and inflammation by activating astrocytes (Hertz et al., 2014). Evidence also indicates that vasopressin increases BBB permeability and water accumulation through the expression of AQP4 (Hertz et al., 2014; Zhao et al., 2015b).
Recent work has shown that endothelin-1, a hormone that is released by endothelial cells, increases within hours after stroke and correlates with BBB hyperpermeability (Li et al., 2022). In addition, endothelin-1 is linked to genes that regulate the secretion of cytokines, inflammation, the release of toxic free radicals, and iron metabolism (Wang et al., 2013).
An in vivo microdialysis study showed that apart from glutamate, there is an excessive extracellular accumulation of amino acids, including taurine, glycine, and asparagine in acute ICH (Shuaib et al., 2003). The role of these amino acids in the pathogenesis of inflammation and prognosis is unclear and needs further exploration (Kanthan et al., 1995; Shuaib et al., 2003).
Following the third phase of cerebral edema, some studies suggest a gradual decline in volume while others suggest otherwise. One study found that edema volume at 1 month was similar to that observed early after ictus (Fung et al., 2016). Peng et al. showed that edema volume at 2–3 weeks was much higher than baseline and was associated with poor outcomes (Peng et al., 2019). Another observational study reported that PHE can last up to 2 months (Chen et al., 2021).
Blood pressure
BP is elevated in ~70% of patients with acute stroke and is associated with poor outcomes (Leonardi-Bee et al., 2002). Following the loss of cerebral autoregulation, high BP in ICH is associated with increased rebleeding, hematoma expansion, and PHE (Willmot et al., 2004). A history of high BP is also relevant as shown by analysis from the Intensive Blood Pressure Reduction in Acute Cerebral Hemorrhage (INTERACT) trial, which showed a significant association with an increase in edema at 72 h compared to baseline (Arima et al., 2009), In addition, BP variability is linked to the pathogenesis of edema in the hyperacute and subacute phases, but the mechanisms are not fully understood (Sykora et al., 2009; Havenon et al., 2018). One explanation is that the variation in BP directly increases the hydrostatic pressure and oncotic pressure in the PHE region (Sykora et al., 2009). Another explanation is that significant fluctuation in BP induces the release of pro-inflammatory cytokines, hyperglycemia, BBB dysfunction, and vasogenic edema (Sykora et al., 2009).
To date, no studies have prospectively tested the effects of BP lowering on PHE, but post-hoc analyses of two trials, INTERACT-2 and the Antihypertensive Treatment in Acute intracerebral hemorrhage (ATACH-2) trial, suggest that the rate of edema expansion was less in participants randomized to intensive treatment (Yang J. et al., 2015; Leasure et al., 2019). It is noteworthy that the majority of participants in INTERACT-2 and ATACH-2 had deep ICH, and one reason for the observed result could be that there is a difference in hematoma dynamics when compared to other brain locations (Seiffge et al., 2022). The trials excluded patients presenting in later-time windows, with large ICH volume and very high systolic BP (>200 mm Hg), and so the pathogenesis of PHE in such patients needs further research (Anderson et al., 2013; Bath et al., 2019).
Neuroimaging measures of PHE
Studies have used various measures of PHE to assess the effect on prognosis. Some studies have used absolute PHE volume in the first hours after stroke and others have suggested the change from baseline to be useful (Sansing et al., 2003; Appelboom et al., 2013; Yang J. et al., 2015). Some authors have postulated using the ratio of PHE per mL of ICH size/volume (relative edema), but it can be difficult to estimate the effects if the hematoma itself is large (Staykov et al., 2011; Appelboom et al., 2013).
Recent work has proposed that the absolute or relative edema change per hour could be more useful as it reflects the actual speed at which the pathogenesis occurs, but this needs to be tested in larger studies (Grunwald et al., 2016).
It may be that examining the peak/highest value of PHE is more appropriate, but performing sequential scans in unwell ICH patients may not be practical (Staykov et al., 2011; Venkatasubramanian et al., 2011). However, the change in PHE is dependent on the hematocrit, which is the proportion of blood volume occupying an erythrocyte, and is known to be higher in males (Venkatasubramanian et al., 2011). A higher hematocrit could therefore expose the brain to more products of erythrolysis over time, and this could delay the peaking of edema. By comparison, in intraventricular hemorrhage, the peak value of edema can be reached more quickly as the hematocrit is diluted by the volume of cerebrospinal fluid (Venkatasubramanian et al., 2011).
Because the development of PHE is linked to ICH volume, it is difficult to assess the independent effects in the first few hours of stroke. Parry-Jones et al. suggested that edema extension distance (EED) or measuring the change in OED over time might be able to overcome this issue (Parry-Jones et al., 2015). OED is the linear measure of the extension of swelling from the boundary of the hematoma, and so theoretically, it should be independent of the hematoma. However, OED is based on the assumption that a hematoma is elliptical in shape and so may not be applicable to irregular ICH (Parry-Jones et al., 2015).
Laboratory testing for PHE
Studies have shown that high serum glucose at baseline is linked with the pathogenesis of PHE (Esposito et al., 2002; Qureshi et al., 2011). Hyperglycemia has been shown to mediate this effect through the release of TNF-α and IL-1 (Esposito et al., 2002). Other laboratory measures, including a high red blood cell count, increased platelet count, and increased hematocrit, are associated with an increase in PHE in the first week after symptom onset (Sansing et al., 2003; Venkatasubramanian et al., 2011). It is suggested that an increase in thromboplastin time correlates with the expansion of PHE, which implies the activation of the coagulation system (Sansing et al., 2003). A meta-analysis including 2,176 patients analyzed the prognostic effect of neutrophils and lymphocytes on outcomes after ICH (Liu et al., 2019). The results showed that a high neutrophil-to-lymphocyte ratio predicted an increased risk of early death, death, or disability at day 90 (Liu et al., 2019). Pathophysiologically, this can be explained as ongoing inflammation in a patient with a weakened immune system who is likely to have a poor outcome.
Prognostic effects of PHE
With advances in understanding the natural history of PHE, there is interest in assessing the prognostic effects. The mechanisms are not yet understood, and one reason is because the pathogenesis of PHE is linked to the presenting hematoma, which could confound the clinical effects (Gebel et al., 2002; Arima et al., 2009; Wu J. et al., 2017). In patients with ICH volume <30 cm3, observational data have shown that the presence of PHE at baseline increases the risk of death or disability at day 90 (Appelboom et al., 2013; Murthy et al., 2015). One explanation is that in small hematomas, a modest increase in the mass effect from PHE could lead to clinical deterioration. However, such patients could be potentially eligible for testing interventions for edema, which, in turn, could improve outcomes.
After adjusting for ICH volume, studies have used other measures of PHE and assessed the effect on clinical outcomes. Gebel et al. reported that there was an inverse correlation between relative PHE and death or disability at day 90 but other authors found no association (Gebel et al., 2002; Arima et al., 2009; Staykov et al., 2011; Volbers et al., 2016). Another study examined EED and found that a large deviation induced by swelling increased the risk of midline shift and brain herniation (Wu J. et al., 2017). Recently, Urday et al. showed that the peak increase in PHE between baseline and 24 h was independently associated with death at day 90 (Urday et al., 2016). Studies have also attempted to assess the effects of PHE beyond the hyperacute phase and shown that an increase up to 72 h predicts poor functional outcomes (Sansing et al., 2011a; Li et al., 2013; Bakhshayesh et al., 2014; Yang J. et al., 2015; Urday et al., 2016).
Research suggests that the prognostic effects of PHE depend on the location of the stroke itself (Appelboom et al., 2013; Grunwald et al., 2016; Leasure et al., 2019). This could be highly relevant in deep hematomas in the thalamus or basal ganglia, as these structures are important for maintaining consciousness, movement, and executive function. It is therefore intuitive that PHE or an increase in volume in these regions could worsen damage and result in poor outcomes (Grunwald et al., 2016; Leasure et al., 2019).
It is important to highlight that the aforementioned studies have been retrospective; varied in inclusion criteria, the modality of neuroimaging, the timing of scanning, and outcome measures; and utilized heterogeneous measures of PHE. Furthermore, little is reported on the late phase of edema and how this affects outcomes after ICH. More research is needed to understand these temporal relationships in prospective studies, including a large number of patients.
Potential interventions for cytotoxic edema
Targeting SUR1-TRPM4 channels
Glyburide, also known as glibenclamide, a sulfonylurea receptor (SUR1) antagonist and potent inhibitor of TRPM4 channel in the brain, has emerged as a potential treatment for cerebral edema (Simard et al., 2006). In acute stroke, the low pH in the brain resulting from the lack of energy and hypoxia facilitates the entry of glibenclamide through the BBB (Simard et al., 2006). In one preclinical model, glibenclamide reduced PHE, protected the BBB, and reduced the expression of MMP. In addition, treatment was associated with improved neurological function (Zhou et al., 2018). However, Kung et al. reported that glibenclamide had no effect, but this could be explained by the different ICH model that was used (Kung et al., 2021) (Table 1). In a small clinical trial, treatment using a patented form of intravenous glibenclamide in ischemic stroke was associated with a reduction in the midline shift and MMP-9 levels measured at 24–72 h compared to controls (Sheth et al., 2016). Based on these results, a recent phase 2 trial tested the safety and efficacy of oral glibenclamide in basal ganglia ICH-associated cerebral edema within 72 h of symptom onset (NCT03741530, 2022). The results showed that treatment significantly reduced the proportion of poor outcomes at day 90, but glibenclamide was associated with a higher incidence of hypoglycemia, although this was asymptomatic (Zhao et al., 2022).
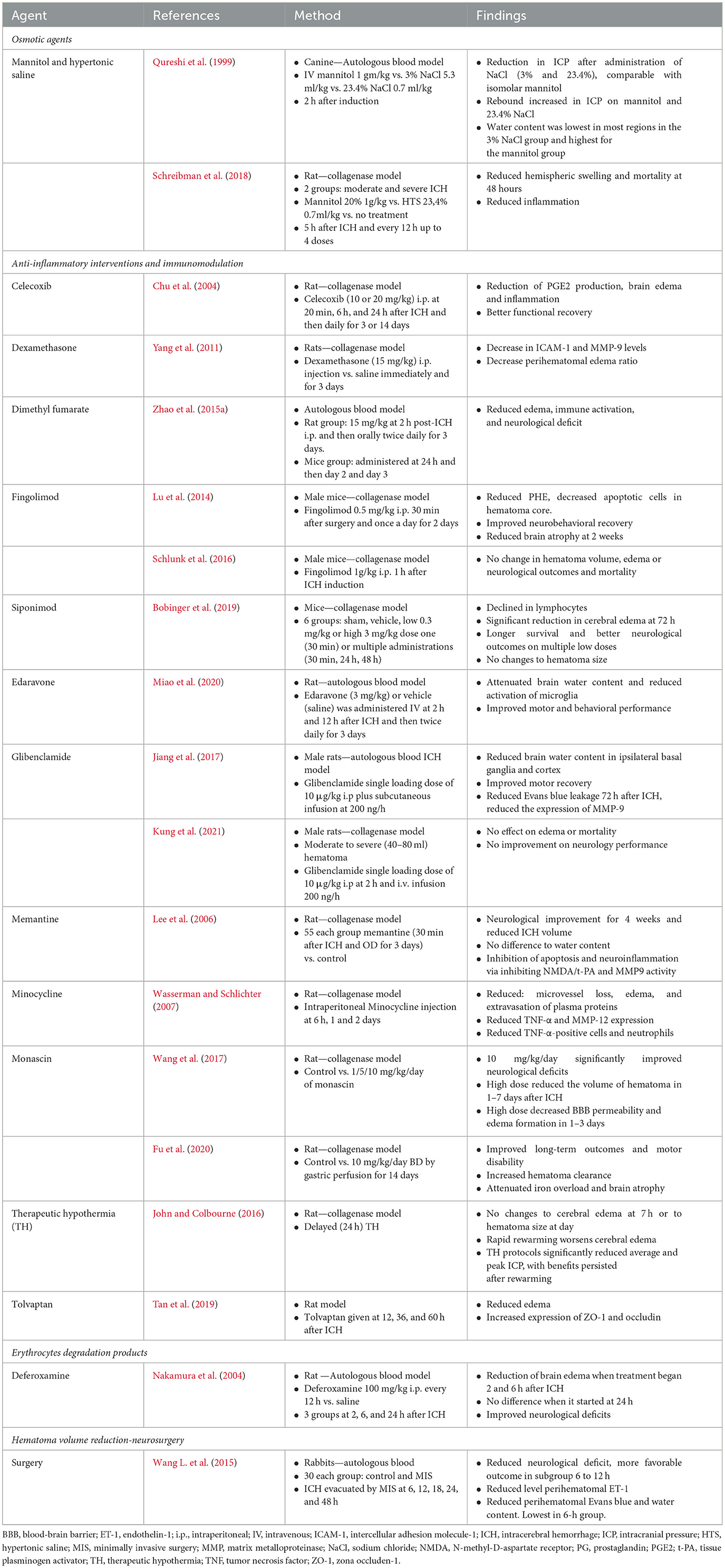
Table 1. Summary of key preclinical studies testing interventions for cerebral edema in intracerebral hemorrhage.
Targeting Na-K-Cl cotransporters
Bumetanide, a Na-K-Cl-cotransporter inhibitor, is used routinely to treat peripheral edema from congestive cardiac failure. In experimental studies, bumetanide has been shown to reduce cytotoxic edema in ischemic stroke, traumatic brain injury, and liver failure (Bautista et al., 2021). However, bumetanide has shown no effect on cytotoxic edema associated with ICH (Wilkinson et al., 2019).
Targeting aquaporin channels
Tolvaptan is a selective oral Vasopressin V2-receptor antagonist that has been shown to reduce the expression of the AQP4 channel and promote the excretion of excess brain water (Tan et al., 2019). In a preclinical model, administration of Tolvaptan at 12 h, 36 h, and 60 h reduced cerebral edema, prevented degradation of the BBB by downregulating MMP-9, and increased expression of tight junction proteins, occludin and zonula occludens-1 (Tan et al., 2019). Similarly, treatment with Conivaptan has correlated with reduced expression of AQP4 (Corry et al., 2020).
There is preliminary evidence that a novel agent, (nicotinamide)-1,3,4-thiadiazole, TGN-020, and piroxicam, a nonsteroidal anti-inflammatory drug, could also inhibit AQP4 channels (Zhang et al., 2016; Li et al., 2019). More studies are required before translation to clinical settings.
Interventions for vasogenic edema
Osmotic agents
Mannitol is one of the most widely used osmotic agents and lowers intracranial pressure by two mechanisms: an immediate effect through plasma expansion, which decreases plasma viscosity, which, in turn, improves regional brain perfusion and oxygenation (Sorani et al., 2008; Diringer et al., 2012; Rickard et al., 2014). Through its osmotic effect, mannitol reduces cerebral edema by drawing water from the brain interstitium into the intravascular compartment (Sorani and Manley, 2008). As a free radical scavenger, mannitol also acts as a neuroprotectant (Bereczki et al., 2007).
Most preclinical studies of mannitol have been in traumatic brain injury, and few studies have tested the effects in acute ICH. One study compared mannitol with two doses of hypertonic saline whereas another tested intravenous infusion of 20% of mannitol every 12 h for 4 doses in total (Qureshi et al., 1999; Schreibman et al., 2018). In both studies, mannitol reduced intracranial pressure and death (Qureshi et al., 1999; Schreibman et al., 2018).
Clinical studies assessing cerebral blood flow have reported varying results from no change with low-dose mannitol (0.9 g/kg) to transient increase using a higher dose (1.5 g/kg) (Misra et al., 2007). A post-hoc analysis of a large BP lowering trial showed that mannitol was associated with better outcomes in patients with ICH volume >15 mls (Wang X. et al., 2015; Shah et al., 2018). However, observational data from another study showed no difference in functional outcome at 3 months (Shah et al., 2018).
The precise dose of mannitol for cerebral edema is unknown, but multiple doses can cause severe hypernatremia and high osmolarity, which, in turn, can cause neurological complications, such as osmotic demyelination syndrome (Rickard et al., 2014).
A systematic review assessed the effects of mannitol vs. no mannitol within 24 h of symptom onset (Sun et al., 2018) (Table 2). The conclusion was that treatment should be used only in large hematomas and in patients with cerebral edema or raised intracranial pressure (Sun et al., 2018). However, the studies were mainly retrospective and included a small number of patients, so prospective randomized trials testing the appropriate dose of mannitol, timing, and duration of treatment are warranted (Sun et al., 2018).
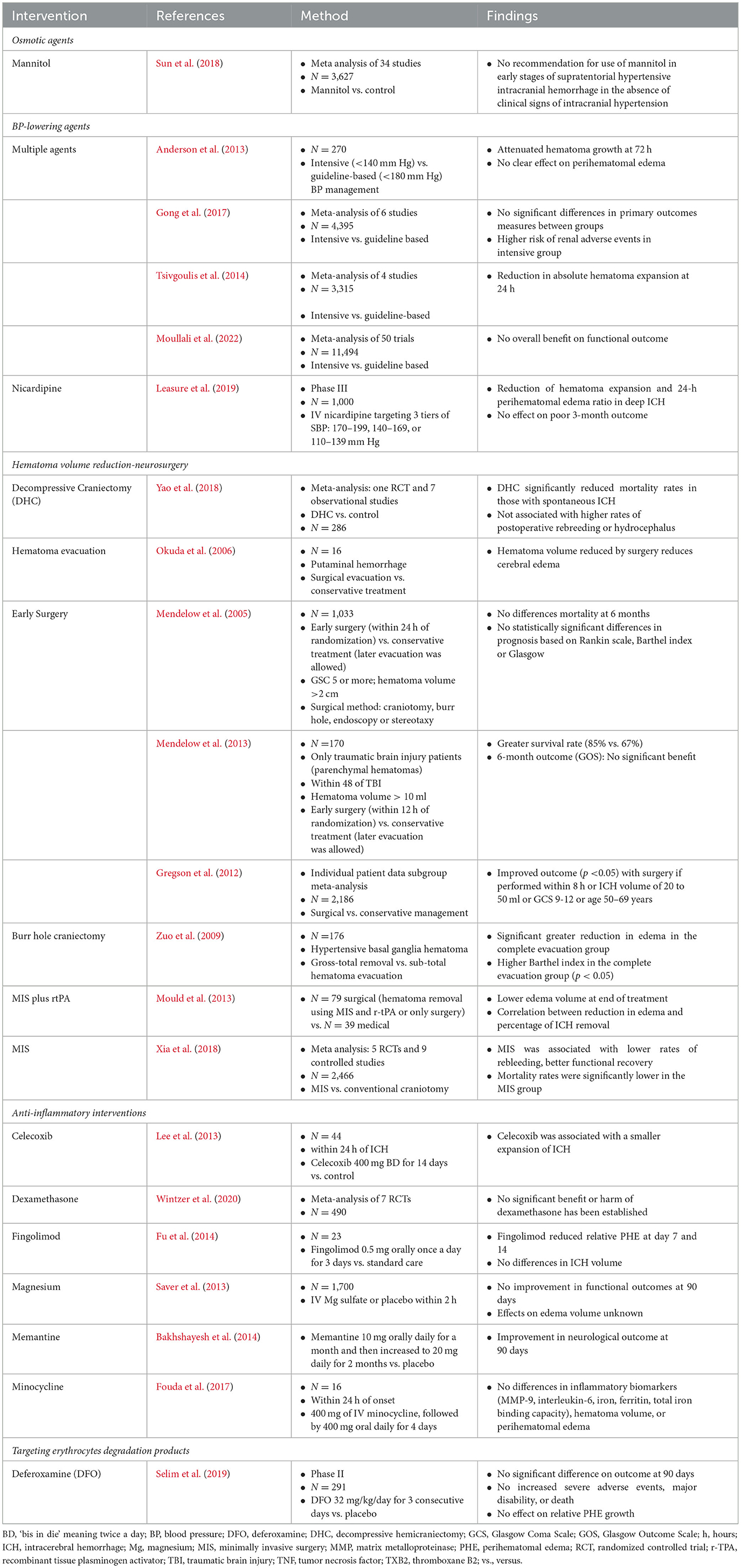
Table 2. Summary of key clinical trials and meta-analysis of cerebral edema in acute intracerebral hemorrhage.
Another osmotic agent, hypertonic saline, has also been assessed. One preclinical study found that intravenous hypertonic saline 3% and 23.4% were equally effective in reducing intracranial pressure (Qureshi et al., 1999). Schreibman et al. combined hypertonic saline with mannitol in moderate to severe ICH and found that there was reduced mortality and swelling at 48 h (Schreibman et al., 2018). In that study, repeated doses of treatment reduced activation of microglia, free-radical formation, and inflammation in the PHE region and the contralateral hemisphere (Schreibman et al., 2018). Despite this evidence, there are few data on the clinical effects of hypertonic saline, and no randomized trials have been performed (Wagner et al., 2011). Hypertonic saline can cause electrolyte imbalance, volume overload, coagulopathy, and rebound increase in intracranial pressure, so patients have to be carefully monitored (Diringer and Zazulia, 2004; Rickard et al., 2014).
Preclinical studies have shown that the combination of furosemide and mannitol can be more effective in reducing intracranial pressure and reversing the osmotic gradient in ICH than mannitol alone (Thenuwara et al., 2002). Similar results have been observed with furosemide and hypertonic saline compared to hypertonic saline (Mayzler et al., 2006). There is a risk of electrolyte disturbance and neurological complications, but it is thought to be not higher with two osmotic agents compared to one (Pollay et al., 1983; Thenuwara et al., 2002; Diringer and Zazulia, 2004). This might mean that combining two agents for cerebral edema is synergistic but needs to be evaluated further.
Thrombin inhibition
There is evidence that suggests that inhibition of thrombin can reduce vasogenic edema after acute ICH (Xi et al., 2003). Treatment with a thrombin inhibitor such as argatroban might seem counterintuitive as thrombin is essential for coagulation. However, thrombin which is bound to fibrin for the first few days after stroke is released afterwards leading to delayed cerebral edema (Kitaoka et al., 2002). So, starting treatment after a hematoma has stabilized may be useful. In a small study, intravenous argatroban was tested 24 h after ictus in a rat ICH model and the results showed that treatment was associated with a reduction in perihematomal edema (Nagatsuna et al., 2005). In addition, thrombin was also found to suppress the infiltration of neutrophils and macrophages (Nagatsuna et al., 2005). This suggests that argatroban might be a potential therapeutic option for ICH edema but studies are required to assess the appropriate dose, safety, and efficacy (Bath, 2012).
Anti-inflammatory agents
During the inflammatory phase of edema, the selective depletion of neutrophils has been shown to reduce BBB permeability, MMP-9 expression, and axonal damage and improve outcomes in experimental models (Moxon-Emre and Schilchter, 2011; Sansing et al., 2011b). Similarly, inhibiting MMP or treatment with toxic free radical scavengers or a TNF-α antibody could reduce perihematomal edema, but prospective clinical studies are awaited (Imai et al., 2019; Lattanzi et al., 2020).
Minocycline is a second-generation tetracycline antibiotic that is able to enter the brain through the BBB following a stroke (Wasserman and Schlichter, 2007). Minocycline inhibits the activation of microglia, and in one preclinical study, the treatment reduced BBB permeability, prevented extravasation of plasma proteins, and showed antioxidant and antiapoptotic properties (Wasserman and Schlichter, 2007; Chang et al., 2017). Studies have also shown that minocycline reduces iron overload and associated brain damage (Zhao et al., 2011; Chang et al., 2017; Cao et al., 2018). In a clinical trial, treatment with minocycline was safe and tended to reduce MMP-9 after 3–5 days (Fouda et al., 2017). However, the study was unable to assess the effect on edema volume or functional outcome (Fouda et al., 2017).
Tranexamic acid is a well-known antifibrinolytic agent that has been shown to be effective in reducing bleeding after trauma and postpartum hemorrhage (Shakur et al., 2010; Trial Collaborators WOMAN, 2017). It has been tested in acute ICH, and in a large trial, the Tranexamic Acid for Hyperacute Primary Intracerebral Hemorrhage (TICH-2) trial, treatment within 8 h was associated with reduced hematoma expansion and early death, but there was no difference in functional outcome at day 90 (Sprigg et al., 2018). Due to the mechanism of action, there is a risk of brain ischemia, but a substudy of TICH-2 reported no difference in patients who received tranexamic acid compared to those who received placebo (Josephson et al., 2022). Experimental studies suggest that tranexamic acid also has anti-inflammatory effects by decreasing TNF-α, IL-1β, and M1 macrophages and increasing the levels of the M2 macrophage phenotype (Barrett et al., 2019; Yoshizaki et al., 2019). Whether these putative effects translate into the clinical setting needs to be assessed.
Celecoxib, a well-known cyclo-oxygenase-2 inhibitor, has been tested in acute ICH and shown to reduce inflammation and edema associated with prostaglandin E2 (Chu et al., 2004). In a pilot, clinical trial, patients randomized to 14 days of celecoxib (400mg twice daily) had a significant reduction in edema volume compared to controls (Lee et al., 2013). However, the time from ictus to the first CT scan was different between the two groups, which could have impacted the results (Lee et al., 2013).
The rationale for using steroids such as dexamethasone is to minimize the mass effect of edema and inflammation (Lee et al., 2015). Dexamethasone could promote recovery after ICH because it decreases apoptotic cell death and inhibits the expression of MMP-9 (Yang et al., 2011; Lee et al., 2015). Similar to celecoxib, preclinical studies of dexamethasone have shown positive effects (Yang et al., 2011; Lee et al., 2015), but this has not been translated to clinical trials. A meta-analysis including 248 patients found that there was no evidence of a benefit from dexamethasone, so its use for routine treatment for ICH edema is not recommended (Wintzer et al., 2020).
Experimental studies have shown that selective deactivation of the complement cascade could inhibit brain inflammation (Xi et al., 2002; Gong et al., 2005). For example, N-acetyl heparin, which is a derivative of heparin, inhibits complement without any effect on coagulation (Gong et al., 2005). It is also known to inhibit thrombin-induced complement activation (Gong et al., 2005). This proof of concept was shown in one small preclinical study, with a reduction in brain edema at 24 and 72 h. Work on this has since been reproduced and extended to effects including the reduction of brain atrophy at 1 month after ICH (Gong et al., 2005).
There is emerging evidence that peroxisome proliferator-activated receptor–gamma (PPAR-γ), a transcription factor, is important for modulating inflammation and phagocytosis. Therefore, testing PPAR-γ agonists such as pioglitazone and rosiglitazone could lead to reduction of hematoma and enhance recovery (Zhao et al., 2006, 2007a,b). These agents have been tested in preclinical studies, but there is little clinical data for ICH (Zhao et al., 2007a; Wu et al., 2015). Preliminary work indicates that through specific inhibition of TLR-4 on macrophages, outcomes could improve, but more research is needed (Wu J. et al., 2017).
Recently, the recombinant human IL-1 receptor antagonist Anakinra has been shown to reduce inflammation in preclinical models of ICH (Smith et al., 2018). Based on these results, two phase 2 studies are testing its effects within 8 h of symptom onset (NCT04834388; NCT03737344).
Immune modulation
Fingolimod, a sphingosine 1-phosphate receptor analog (S1 P-R), is able to cross the BBB and act directly on neurons and glial cells (Fu et al., 2014). In preclinical studies using collagenase models of ICH, treatment with fingolimod was associated with less cerebral edema and lymphocytic proliferation and decreased apoptosis (La Mantia et al., 2016). Other studies have shown that fingolimod is neuroprotective and improves behavioral outcomes (Lu et al., 2014). A phase 2 clinical study has shown that treatment was associated with reduced perihematomal edema volume (Fu et al., 2014), and this has led to testing a single-dose 0.5 mg fingolimod as potential treatment within 24 h of ictus (NCT04088630) (Wolfe, 2022).
Siponimod is a more selective S1 P-R analog but has a shorter half-life compared to fingolimod. Experimental studies of siponimod showed that it reduced cerebral edema and improved neurological recovery and survival (Bobinger et al., 2019, 2020). However, a phase 2 randomized clinical trial (NCT03338998) was terminated due to a lack of efficacy (NCT03338998, 2022).
Putative neuroprotectants
Edaravone, which is a novel synthetic molecule with scavenging properties, is an attractive neuroprotectant. In one preclinical study, edaravone reduced brain water content, suppressed inflammation by blocking the release of NLRP3 [nucleotide binding oligomerization domain (NOD), leucine-rich repeat (LRR), and pyrin domain–containing protein 3], and improved motor and cognitive outcomes (Miao et al., 2020). Little is known of the clinical effects, and so a small trial (NCT04714177) has begun testing the effects of edaravone in hypertensive ICH patients within 48 h of onset (NCT04714177, 2021).
One study tested disufenton, a free-radical trapping agent, in patients with ICH, but there was no benefit (Lyden et al., 2007). The reasons could be reflected in the insufficient permeability of disufenton to enter through the BBB or that a single agent is not enough to neutralize the large numbers of free radicals that are generated (Lyden et al., 2007). Other agents such as pyrroloquinoline quinone and sulforaphane, which activate a protein nuclear factor (erythroid2-related factor 2), have been tested for their antioxidant properties and whether they enhance brain recovery in experimental stroke (Ma et al., 2015; Bautista et al., 2021). The clinical effects are yet to be established.
Given the inability of free radical scavengers to affect the neurological outcomes in patients with acute ischemic stroke (Serebruany, 2006; Shuaib et al., 2007) and the prominent role of pro-oxidant enzyme Nicotinamide adenine dinucleotide phosphate (NADPH) oxidase in cerebrovascular ROS generation (Serebruany, 2006; Shuaib et al., 2007; Allen and Bayraktutan, 2009), it is conceivable that the inhibition of this enzyme system or its functional subunits (p22-phox or gp91-phox) may prove efficacious. Indeed, suppression of NADPH oxidase has been shown to negate its effects in acute ischemia, hyperglycemia, or TNF-? on an in vitro model of human BBB through regulation of apoptosis, MMP-2/9 and the protein kinase C pathway (Abdullah and Bayraktutan, 2014; Rakkar et al., 2014; Shao and Bayraktutan, 2014).
Magnesium is appealing as a neuroprotectant because of its membrane-stabilizing effects, and it has been shown to increase cerebral blood flow, enhance recovery, and reduce damage from toxic free radicals (Avgerinos et al., 2019). Studies have shown that it is feasible to administer intravenous magnesium in acute stroke (Saver et al., 2004), but in one phase 3 trial, treatment within 2 h had no effect on neurological recovery or functional outcome (Saver et al., 2013). Potential explanations for these results include the small number of patients with ICH and the timing could have been too early for treatment to have any effect on edema (Saver et al., 2013).
Inhibition of excess accumulation of glutamate in acute ICH with memantine, a fast-acting, noncompetitive NMDA channel antagonist, has been tested (Lee et al., 2006). One preclinical trial of memantine reported an approximate 50% reduction in ICH volume coupled with the inhibition of MMP-9 following treatment for 14 days (Lee et al., 2006). In another study, 64 patients were allocated to either memantine (10 mg daily for a month and then increased to 20 mg daily for 2 months) or placebo within 24 h of ictus (Bakshayesh-Ebhbali and Hajinnori, 2015). At 3 months, patients treated with memantine reported better motor function compared to those receiving the placebo (Bakshayesh-Ebhbali and Hajinnori, 2015). Data also suggest that memantine may improve cognition and aphasia (Berthier et al., 2009), but this needs to be tested further.
Statins have been shown to be neuroprotective by modulating cellular pathways and controlling leukocyte proliferation, adhesion, migration, and angiogenesis (Tapia-Perez et al., 2013). Preclinical studies have shown that these effects might translate to clinical recovery through hematoma resolution and reduced cerebral edema (Jung et al., 2004; Yang et al., 2013; Chen et al., 2019). Based on these results and retrospective data showing lower mortality, the Statin for Neuroprotection in Spontaneous Intracerebral Hemorrhage trial (STATIC) is now testing the effect of Atorvastatin 20 mg in ICH-associated cerebral edema (Chen et al., 2019) (Appendix).
Other neuroprotective agents include monascin, which is a natural agonist of the PPAR. In preclinical models, monascin has been shown to reduce ICH volume and reduce cerebral edema and BBB permeability at higher doses (Wang et al., 2017; Fu et al., 2020). Further testing of this agent is awaited.
Hypothermia
Therapeutic hypothermia and fever prevention have the potential to be therapeutic options for ICH edema (Loach and Bayraktutan, 2016; Greer et al., 2021). Preclinical studies have shown promise, and a meta-analysis concluded that hypothermia reduced edema-associated BBB disruption through the suppression of excitotoxicity, inflammation, oxidative stress, and apoptosis (Staykov et al., 2010; John and Colbourne, 2016; Loach and Bayraktutan, 2016; Melmed and Lyden, 2017). Furthermore, therapeutic hypothermia has been shown to augment the BBB-reparative capacity of therapeutic agents targeting protein kinase C-β or the NADPH oxidase enzyme system (Kadir et al., 2021). One prospective clinical study tested endovascular mild hypothermia (35 degrees celcius for 10 days) in patients with moderate volume ICH (Staykov et al., 2013). The study reported that the volume of edema remained stable throughout treatment, and, interestingly, there was no rebound increase after patients had been rewarmed to normal temperature (Staykov et al., 2013). Therapeutic hypothermia is associated with complications such as pneumonia, coagulopathy, and bradycardia, which appear to be directly related to the depth and duration of cooling (Zhao et al., 2007a; John and Colbourne, 2016). Therefore, optimizing cooling protocols might prevent such complications, but this needs to be assessed in future studies (Staykov et al., 2010; Loach and Bayraktutan, 2017).
Targeting erythrolysis and products of degradation
Deferoxamine has been shown to be neuroprotective and improve outcomes in experimental ICH (Staykov et al., 2010; Selim et al., 2011). As a chelator, it reduces the amount of free iron that is available to cause cerebral edema and neurotoxicity. Deferoxamine has also been shown to have other effects: it has antioxidant properties that directly reduce neuronal damage; downregulate cytokines, particularly TNF-α; and activate the enzyme cyclooxygenase, thereby reducing brain inflammation and inhibiting toxicity caused by hemoglobin (Nakamura et al., 2004; Okauchi et al., 2009). A meta-analysis analyzing the effect of deferoxamine in preclinical models of ICH found that treatment reduced brain water by 85% and improved neurobehavioral outcomes (Okauchi et al., 2009). In addition, this analysis reported that treatment was effective if started within the first few hours of ictus (Okauchi et al., 2009).
Based on these results, a phase II clinical trial tested deferoxamine at a dose of 32 mg/kg/day (Selim et al., 2019). Participants were enrolled within 24 h of ICH, and treatment was given for 3 consecutive days (Selim et al., 2019). The results showed that deferoxamine was safe, but there was no difference in the primary outcome of death or dependency at day 90 between deferoxamine compared to placebo (34% vs. 33%) (Selim et al., 2019). A post-hoc analysis suggested a treatment effect at 6 months, indicating that the benefits with deferoxamine might be observed after a longer period but there was no effect on cognition, mood, or PHE (Wei et al., 2021). The results do not support testing of deferoxamine on its own in a future study, but combining it with another intervention could be an option (Wei et al., 2021).
Endothelial progenitor cells
Cell therapy with endothelial progenitor cells (EPCs), bone marrow–derived circulating stem cells, may induce therapeutic angiogenesis and potentially improve outcomes after ICH. Translational and clinical studies indicate that EPCs may potentiate endothelial cell regeneration, a prerequisite for effective BBB repair and neovascularization (Bayraktutan, 2019; Kadir et al., 2022a,b). Indeed, EPC levels are associated with good neurological outcomes and reduced hematoma volume in ICH, implying the potential as a novel therapeutic option for patients (Pías-Peleteiro et al., 2016).
Surgery
Surgery for ICH has theoretical benefits for the management of edema: it reduces mass effect, lowers intracranial pressure (ICP), and removes inflammatory and neurotoxic blood products. Open craniotomy is the most studied approach, but two well-powered randomized controlled trials did not show a benefit of surgery compared to medical management alone (Mendelow et al., 2005, 2013). A pooled analysis of the two trials with results of previous studies suggested that early surgery irrespective of the technique was associated with a survival benefit in a subgroup of patients (e.g., those who deteriorate neurologically from hematoma expansion or superficial, lobar ICH with no intraventricular extension) (Gregson et al., 2012). It is important to highlight that the two trials were based on clinical equipoise, i.e. patients thought to benefit from surgery at the outset were excluded (Mendelow et al., 2005, 2013). Moreover, the protocols allowed cross-over of patients to craniotomy if the patient deteriorated (Mendelow et al., 2005, 2013). As a result, the actual benefit of delayed surgery in ICH is unclear and could have been underestimated.
Preclinical studies have reported that early minimally invasive surgery is associated with reduced extracellular glutamate, perihematomal endothelin-1 levels, BBB permeability, and brain water content (Wu et al., 2013; Wang L. et al., 2015). In a small study, patients with putaminal hematomas who underwent surgery had significantly reduced cerebral edema on follow-up scans (Okuda et al., 2006). However, a decrease in edema has been shown to depend on the amount of hematoma that is evacuated (Horowitz et al., 2021). Another analysis compared the effect of hematoma drainage by burr hole craniectomy on severity of cerebral edema (Zuo et al., 2009). The results showed that at 3 weeks, surgery significantly reduced levels of TNF-α and products released by the coagulation cascade (Zuo et al., 2009). Although hematoma evacuation is suggested to reduce perihematomal edema, it is unclear whether it affects outcomes. One study analyzed the relationships between the ratio of residual hematoma volume to edema, inflammation, and prognosis (Zuo et al., 2009). At 1 year, patients who had complete evacuation of hematoma had better functional outcomes (Zuo et al., 2009). A post-hoc analysis of a phase 3 trial reported a 35% reduction in perihematomal edema, but there was no improvement in functional outcomes (Hanley et al., 2019).
One factor that can increase edema is intraventricular hemorrhage (IVH) because there is an imbalance in the absorption of cerebrospinal fluid. This often leads to acute hydrocephalus, which, in turn, worsens edema by increasing ICP, reduces brain perfusion, and induces ventricular wall stretch, white matter injury, and inflammation (Holste et al., 2022). Therefore, placement of an external ventricular drain (EVD) could reduce these effects. Observational data suggest that placement of EVD in IVH is associated with reduced mortality, but the effect on long-term outcomes is unclear (Lovasik et al., 2016). It is also important to highlight that placement of EVD has its challenges including blockage, malpositioning, overdrainage, the need for prolonged drainage, and infection (Jamjoom et al., 2018). To date, there have been no randomized trials of EVD in ICH, therefore research into the potential benefits is needed.
Decompressive hemicraniectomy (DHC) refers to the removal of a window of bone with wide durotomy or dural expansion to allow for normalization of ICP and mass effect. DHC is routinely performed in malignant middle cerebral artery (MCA) infarction, but it is less established for the treatment of spontaneous ICH (Yao et al., 2018). The effect of DHC for edema after ICH is unclear, and the indication must be balanced against the complications, including hematoma expansion (~25% of cases) and an additional procedure, that is, cranioplasty (Gopalakrishnan et al., 2018). One randomized trial is evaluating whether DHC with the best medical treatment improves outcomes vs. best medical treatment alone (NCT02258919, 2023) (Appendix).
Conclusion and future perspectives
As of yet, there is no established treatment for prevention or reducing PHE, but information on which ones show promise and those that may not be effective is emerging. Some drugs used in other medical conditions have properties that could be useful in treating cerebral edema and offer the possibility of being repurposed.
Lowering BP is recommended after acute ICH, but its routine use and for secondary prevention means that specific testing for effects on PHE might necessitate a comparison of intensity (standard vs. guideline) or agent. Osmotic agents have biologically plausible mechanisms that might be effective for treating PHE, but more work is needed on the optimal dose, treatment duration, safety, and efficacy. Because hematoma volume is strongly linked with cerebral edema, surgery may still have a role, but more research is needed on the timing and technique to identify which patients benefit.
The involvement of AQP4 channels in the pathogenesis of cytotoxic edema is clear, but more work is needed to develop effective agents. The SuR1-TRPM4 channel seems to be a key target, and glyburide as a potential inhibitor is promising. One concern is that treatment might increase the risk of hypoglycemia, although this was not observed in a small phase 2 trial testing the effects of glyburide in ischemic stroke (Sheth et al., 2016).
Agents that are neuroprotective and remove toxic free radicals are promising as are treatments that attenuate inflammation and infiltration of leucocytes into the perihematomal region after ICH. With careful selection, it is possible that some of these could translate from preclinical to clinical studies. This review also highlights that a better understanding of the role of inflammation and the immune system after stroke is needed as the potential for modulation could have profound implications for clinical management.
As highlighted, the mechanisms of PHE are complex with the magnitude time-dependent, and this should be taken into consideration when designing future clinical studies. For example, a trial could test reducing hemorrhage expansion first and, when the bleeding has stopped, then assess evacuation followed by an anti-inflammatory or neuroprotective agent. In the acute phase, it may be that osmotic agents complement BP lowering, hemostatic therapy, or surgery for ICH. Such an innovation would warrant streamlining of trial protocols, careful patient selection, and incorporation of specific outcomes to test response and recovery. This work will need close collaboration between clinicians, researchers, pharmaceutical companies, and industry.
The present review also highlights that there is variation in the measurement of PHE and the modality of brain imaging, therefore more research is needed to identify a standard to assess change or severity. Evidence suggests that brain repair and recovery can continue for months after ICH (Selim et al., 2019), therefore testing the long-term effects of potential treatments for cerebral edema is warranted.
Author contributions
KK: Conceptualization, Writing—original draft, Data curation, Methodology, Resources. PC: Writing—original draft, Data curation, Resources. TN: Writing—review and editing. CT: Data curation, Writing—original draft, Resources. SC: Data curation, Writing—original draft, Resources. JA: Writing—review and editing. ZL: Writing—original draft, Resources. MH: Writing—original draft. MK: Writing—review and editing. TE: Writing—review and editing. CR: Writing—review and editing. MM: Writing—review and editing. JD: Writing—review and editing. UB: Writing—original draft. DW: Writing—review and editing. NS: Writing—review and editing. PB: Writing—review and editing.
Funding
The author(s) declare that no financial support was received for the research, authorship, and/or publication of this article.
Conflict of interest
The authors declare that the research was conducted in the absence of any commercial or financial relationships that could be construed as a potential conflict of interest.
TN declared that they were an editorial board member of Frontiers, at the time of submission. This had no impact on the peer review process and the final decision.
Publisher's note
All claims expressed in this article are solely those of the authors and do not necessarily represent those of their affiliated organizations, or those of the publisher, the editors and the reviewers. Any product that may be evaluated in this article, or claim that may be made by its manufacturer, is not guaranteed or endorsed by the publisher.
References
Abdullah, Z., and Bayraktutan, U. (2014). NADPH oxidase mediates TNF-?-evoked in vitro brain barrier dysfunction: roles of apoptosis and time. Mol. Cell. Neurosci. 61, 2. doi: 10.1016/j.mcn.2014.06.002
Allen, C. L., and Bayraktutan, U. (2009). Oxidative stress and its role in the pathogenesis of ischaemic stroke. Int. J. Stroke. 4, 387. doi: 10.1111/j.1747-4949.2009.00387.x
Anderson, C. S., Heeley, E., and Huang, Y. (2013). Rapid blood-pressure lowering in patients with acute intracerebral hemorrhage. N. Engl. J. Med. 2013, 2355–65. doi: 10.1056/NEJMoa1214609
Anderson, C. S., Huang, Y., Wang, J. G., Arima, H., Neal, B., Peng, B., et al. (2008). Intensive blood pressure reduction in acute cerebral haemorrhage trial (INTERACT): a randomised pilot trial. Lancet Neurol. 7, 391–399. doi: 10.1016/S1474-4422(08)70069-3
Appelboom, G., Bruce, S. S., Hickman, Z. L., Zacharia, B., Carpenter, A., Vaughan, K., et al. (2013). Volume-dependent effect of perihematomal oedema on outcome for spontaneous intracerebral haemorrhage. JNNP. 84, 488–493. doi: 10.1136/jnnp-2012-303160
Arima, H., Wang, J. G., Huang, Y., Heeley, E., Skulin, C., Parsons, M., et al. (2009). Significance of perihematomal edema in acute intracerebral haemorrhage: the INTERACT trial Neurology 73, 1963–1968. doi: 10.1212/WNL.0b013e3181c55ed3
Avgerinos, K. I., Chatzisotiriou, A., Haidich, A. B., Tsapas, A., and Lioulas, V. (2019). Intravenous magnesium sulphate in acute stroke. Stroke 50, 931–938. doi: 10.1161/STROKEAHA.118.021916
Bakhshayesh, B., Hosseininezhad, M., Saadat, S. N. S., Ansar, M. M., Ramezani, H., and Saadat, S. M. S. (2014). Iron overload is associated with perihematomal growth following intracerebral haemorrhage that may contribute to in-hospital mortality and long-term functional outcome. Curr Neuro Res. 11, 248–253. doi: 10.2174/1567202611666140530124855
Bakshayesh-Ebhbali, B., and Hajinnori, M. (2015). The effect of memantine and placebo on clinical outcome of intracranial haemorrhage: a randomized double blind clinical trial. Caspian J Neurol Sci. 1, 11–18. doi: 10.18869/acadpub.cjns.1.3.11
Balami, J. S., and Buchan, A. M. (2012). Complications of intracerebral haemorrhage. Lancet Neurol. 11, 101–118. doi: 10.1016/S1474-4422(11)70264-2
Barrett, C. D., Moore, H. B., Kong, Y., Chapman, M. P., Sriram, G., Lim, D., et al. (2019). Tranexamic acid mediates proinflammatory and anti-inflammatory signalling via complement C5a regulation in a plasminogen activator-dependent manner. J. Trauma Acute Care Surg. 86, 101–107. doi: 10.1097/TA.0000000000002092
Bath, P. M. (2012). The argatroban and tissue-type plasminogen activator stroke study: final results of a pilot safety study. Stroke (2012) 2012, 640557. doi: 10.1161/STROKEAHA.111.640557
Bath, P. M., Woodhouse, L. J., and Krishnan, K. (2019). Prehospital transdermal glyceryl trinitrate for ultra-acute intracerebral haemorrhage: data from the RIGHT-2 trial. Stroke 50, 3064–3071. doi: 10.1161/STROKEAHA.119.026389
Bautista, W., Adelson, P. D., Bicher, N., Themistocleous, M., Tsivgoulis, G., Chang, J. J., et al. (2021). Secondary mechanisms of injury and viable pathophysiological targets in intracerebral haemorrhage. Adv Neurol Disord. 14, 1–16. doi: 10.1177/17562864211049208
Bayraktutan, U. (2019). Endothelial progenitor cells: potential novel therapeutics for ischaemic stroke. Pharmacol. Res. 144, 181–191. doi: 10.1016/j.phrs.2019.04.017
Bereczki, D., Fekete, I., and Prado, G. F. (2007). Mannitol for acute stroke. Cochrane Data. Syst. Rev. 18, CD001153. doi: 10.1002/14651858.CD001153.pub2
Berthier, M. L., Green, C., Lara, J. P., Higueras, C., Barbancho, M. A., Dávila, G., et al. (2009). Memantine and constraint-induced aphasia therapy in chronic poststroke aphasia. Ann. Neurol. 65, 577–585. doi: 10.1002/ana.21597
Bobinger, T., Bäuerle, T., Seyler, L., Horsten, S. V., Schwab, S., Huttner, H. B., et al. (2020). A sphingosine-1-phosphate receptor modulator attenuated secondary brain injury and improved neurological functions of mice after ICH. Oxi Med Cell Longev. 2020, 3214350. doi: 10.1155/2020/3214350
Bobinger, T., Manaenko, A., Burkardt, P., Beuscher, V., Sprügel, M. I., Roeder, S. S., et al. (2019). Siponimod (BAF-312) attenuates perihemorrhagic edema and improves survival in experimental intracerebral haemorrhage. Stroke 50, 3246–3254. doi: 10.1161/STROKEAHA.119.027134
Broderick, J. P., Brott, T. G., Duldner, J. E., Tomsick, T., and Huster, G. (1993). Volume of intracerebral hemorrhage. A powerful and easy-to-use predictor of 30-day mortality. Stroke 24, 987–93. doi: 10.1161/01.STR.24.7.987
Cao, S., Hua, Y., Keep, R. F., Chaudhary, N., and Xi, G. (2018). Minocycline effects on intracerebral haemorrhage-induced iron overload in aged rats. Stroke 49, 995–1002. doi: 10.1161/STROKEAHA.117.019860
Chang, J. J., Kim-Tenser, M., Emanuel, B. A., Jones, G. M., Chapple, K., Alikhani, A., et al. (2017). Minocycline and matrix metalloproteinase inhibition in acute intracerebral haemorrhage: a pilot study. Stroke 48, 2885–2887. doi: 10.1111/ene.13403
Chen, C., Ding, D., Ironside, N., Buell, T., Elder, L., Warren, A., et al. (2019). for neuroprotection in spontaneous intracerebral haemorrhage. Neurology 93, 1056–1066. doi: 10.1212/WNL.0000000000008627
Chen, X., Zhang, H., Hao, H., Zhang, X., Song, H., He, B., et al. (2022). Thrombin induces morphological and inflammatory astrocytic responses via activation of PAR1 receptor. Cell Death Discov. 8 doi: 10.1038/s41420-022-00997-4
Chen, Y., Chen, S., Chang, J., Wei, J., Feng, M., Wang, R., et al. (2021). Perihematomal oedema after intracerebral haemorrhage: an update on pathogenesis, risk factors, and therapeutic advances. Front. Immunol. 12, 740632. doi: 10.3389/fimmu.2021.740632
Chhor, V., Charpentier, T. L., Lebon, S., Oré, M., Celador, I. L., Josserand, J., et al. (2013). Characterisation of phenotype markers and neuronotoxic potential of polarised primary microglia in vitro. Brain Behav. Immun. 32, 70–85. doi: 10.1016/j.bbi.2013.02.005
Chu, K., Jeong, S., Jung, K-. H., Han, S., Lee, S-. T., Kim, M., et al. (2004). Celecoxib induces functional recovery after intracerebral haemorrhage with reduction of brain edema and perihematomal cell death. J. Cereb. Blood Flow Metabol. 24, 926–933. doi: 10.1097/01.WCB.0000130866.25040.7D
Collingridge, G. L., Volianskis, A., Bannister, N., France, G., Hanna, L., Mercier, M., et al. (2013). The NMDA receptor as a target for cognitive enhancement. Neuropharmacology 64, 13–26. doi: 10.1016/j.neuropharm.2012.06.051
Corry, J. J., Asaithambi, G., Shaik, A. M., Lassig, J. P., Marino, E. H., Ho, B. M., et al. (2020). Conivaptan for the reduction of cerebral edema in intracerebral haemorrhage: a safety and tolerability study. Clin. Drug Investig. 40, 9. doi: 10.1007/s40261-020-00911-9
Diringer, M. N., Scalfani, M. T., Zazulia, A. R., Videen, T. O., Dhar, R., and Powers, W. J. (2012). Effect of Mannitol on cerebral blood volume in patients with head injury. Neurosurgery 70, 1215–1219. doi: 10.1227/NEU.0b013e3182417bc2
Diringer, M. N., and Zazulia, A. R. (2004). Osmotic therapy: fact and fiction. Neurocrit. Care. 1, 219–233. doi: 10.1385/NCC:1:2:219
Enzmann, D. R., Britt, R. H., Lyons, B. E., Buxton, J. L., and Wilson, D. A. (1981). Natural history of experimental intracerebral haemorrhage: sonography, computed tomography and neuropathology. AJNR Am. J. Neuroradiol 2, 517–526.
Esposito, K., Nappo, F., Marfella, R., Glugliano, G., Glugliano, F., Ciotola, M., et al. (2002). Inflammatory cytokine concentrations are acutely increased by hyperglycaemia in humans: role of oxidative stress. Circulation 106, 2067–2072. doi: 10.1161/01.CIR.0000034509.14906.AE
Fouda, A. Y., Newsome, A. S., Spellicy, S., Waller, J., Zhi, W., Hess, D., et al. (2017). Minocycline in acute cerebral haemorrhage: an early phase randomised trial. Stroke 48, 2885–2887. doi: 10.1161/STROKEAHA.117.018658
Fu, P., Liu, J., Bai, Q., Sun, X., Yao, Z., Liu, L., et al. (2020). Long-term outcomes of monascin—a novel dual peroxisome proliferator-activated receptor γ/nuclear factor-erythroid 2 related factor-2 agonist in experimental intracerebral haemorrhage. Adv. Neurol. Disord. 13, 1756286420921083. doi: 10.1177/1756286420921083
Fu, Y., Hao, J., Zhang, N., Ren, L., Sun, N., Li, Y., et al. (2014). Fingolimod for the treatment of intracerebral hemorrhage: a 2-arm proof-of-concept study. JAMA Neuro.71, 1092–1101. doi: 10.1001/jamaneurol.2014.1065
Fung, C., Murek, M., Kiinger-Gratz, P. P., Feichter, S., Graggen, W., Gautchi, O., et al. (2016). Effect of decompressive craniectomy on perihematomal oedema in patients with intracerebral haemorrhage. PLoS ONE. 11, e149169. doi: 10.1371/journal.pone.0149169
Galloway, D. A., Phillips, A. E. M., and Owen, D. R. J. (2019). Phagocytosis in the brain: homeostasis and disease. Front. Immunol. 10, 790. doi: 10.3389/fimmu.2019.00790
GBD 2019 Stroke Collaborators (2019). Global, regional, and national burden of stroke and its risk factors, 1990-2019: a systematic analysis for the Global Burden of Disease Study 2019. Lancet Neurol. 20, 795–820.
Gebel, J. M. G., Jauch, E. C., Brott, T. G., Khoury, J., Sauerbeck, L., Salisbury, S., et al. (2002). Natural history of perihematomal edema in patients with hyperacute spontaneous intracerebral hemorrhage. Stroke 33, 2631–2635. doi: 10.1161/01.STR.0000035284.12699.84
Gong, S., Lin, C., Zhang, D., Kong, X., Chen, J., Wang, C., et al. (2017). Effects of intensive blood pressure reduction on acute intracerebral hemorrhage: a systematic review and meta-analysis. Sci Rep. 7, 10694. doi: 10.1038/s41598-017-10892-z
Gong, Y., Xi, G. H., Keep, R. F., Hoff, J. T., and Hua, Y. (2005). Complement inhibition attenuates brain oedema and neurological deficits induced by thrombin. Acta Neurochir. Suppl. 95, 389–392. doi: 10.1007/3-211-32318-X_79
Gopalakrishnan, M. S., Shanbhag, N. C., Shukla, D. P., Konar, S., Bhat, D., and Devi, B. (2018). Complications of decompressive craniectomy. Front. Neurol. 9, 977. doi: 10.3389/fneur.2018.00977
Greer, D. M., Ritter, J., Helbok, R., Badjatia, N., Ko, S., Guanci, M., et al. (2021). Impact of fever prevention in brain-injured patients (INTREPID): study protocol for a randomized controlled trial. Neurocrit. Care. 35, 577–589. doi: 10.1007/s12028-021-01208-1
Gregson, B. A., Broderick, J. P., Auer, L. M., Batjer, H., Chen, X., Juvela, S., et al. (2012). Individual patient data subgroup meta-analysis of surgery for spontaneous supratentorial intracerebral hemorrhage. Stroke 43, 1496–1504. doi: 10.1161/STROKEAHA.111.640284
Grunwald, Z., Beslow, L. A., Urday, S., Vashkevich, A., Ayres, A., Greenberg, S. M., et al. (2016). Perihematomal oedema expansion rates and patient outcomes in deep and lobar intracerebral haemorrhage. Neurocrit. Care. 27, 205–212. doi: 10.1007/s12028-016-0321-3
Hanley, D. F., Thompson, R. E., Rosenblum, M., Yenokyan, G., Lane, K., McBee, N., et al. (2019). Efficacy and safety of minimally invasive surgery with thrombolysis in intracerebral haemorrhage evacuation (MISTIE III): a randomised, controlled, open-label, blinded endpoint phase 3 trial. Lancet 393, 1021–1032. doi: 10.1016/S0140-6736(19)30195-3
Havenon, A., Majersik, A., Stoddard, J. J., Wong, G., McNally, K., Smith, J. S., et al. (2018). Increased blood pressure variability contributes to worse outcome after intracerebral haemorrhage: an analysis of ATACH-2. Stroke 49, 1981–1984. doi: 10.1161/STROKEAHA.118.022133
Hertz, L., Xu, J., Chen, Y., Gibbs, M., Du, T., Hertz, L., et al. (2014). Antagonists of the vasopressin C1 receptpr and of the beta-1adrenoreceptor inhibit cytotoxic brain oedema in stroke by effects on astrocytes-but the mechanisms differ. Curr. Neuropharmacol. 12, 308–323. doi: 10.2174/1570159X12666140828222723
Hochreiter-Hufford, A., and Ravichandran, K. S. (2013). Clearing the dead: apoptotic cell sensing, recognition, engulfment, and digestion. Cold Spring Harb. Perspect. Biol. 5, a008748. doi: 10.1101/cshperspect.a008748
Holste, K., Xia, F., Garton, H. J. L., Wan, S., Hua, Y., Keep, S., et al. (2021). The role of complement in brain injury following intracerebral hemorrhage: a review. Exp. Neuro. 340, 113654. doi: 10.1016/j.expneurol.2021.113654
Holste, K. G., Xia, F., Ye, F., Keep, R. F., and Xi, G. (2022). Mechanisms of neuroinflammation in hydrocephalus after intraventricular hemorrhage: a review. Fluids Barriers CNS. 19, 324. doi: 10.1186/s12987-022-00324-0
Horowitz, M. E., Ali, M., and Chartrain, A. G. (2021). Definition and time course of pericavity oedema after minimally invasive endoscopic intracerebral haemorrhage evacuation. J. Neurointerv. Surg. 14, 149–154. doi: 10.1136/neurintsurg-2020-017077
Hu, X., Tao, C., Gan, Q., Zhen, J., Li, H., and You, C. (2016). Oxidative stress in intracerebral hemorrhage: sources, mechanisms, and therapeutic targets. Oxid. Med. Cell Longev. 2016, 3215391. doi: 10.1155/2016/3215391
Hurford, R., Vail, A., Heal, C., Ziai, W. C., Dawson, J., Murthy, S. B., et al. (2019). Oedema extension distance in intracerebral haemorrhage: association with baseline characteristics and long-term outcome. European Stroke Journal. 4, 263–270. doi: 10.1177/2396987319848203
Imai, T., Iwata, S., Miyo, D., Nakamura, S., Shimazawa, M., and Hara, H. (2019). A novel free radical scavenger, NSP-116, ameliorated the brain injury in both ischaemic and haemorrhagic stroke models. J. Pharmacol. Sci. 141, 119–126. doi: 10.1016/j.jphs.2019.09.012
Investigators, E. T. (2015). Efficacy of nitric oxide, with or without continuing antihypertensive treatment, for management of high blood pressure in acute stroke (ENOS): a partial-factorial randomised controlled trial. Lancet 385, 617–628. doi: 10.1016/S0140-6736(14)61121-1
Ironside, N., Chen, C. J., Ding, D., Mayer, S. A, and Connelley, S. E. (2019). Perihematomal oedema after spontaneous intracerebral haemorrhage. Stroke 50, 1626–1633. doi: 10.1161/STROKEAHA.119.024965
Jamjoom, A. A. B., Joannides, A. J., Poon, M. T., Chari, A., Zaben, M., Abdulla, M. A. H., et al. (2018). Prospective, multicentre study of external ventricular drainage-related infections in the UK and Ireland. JNNP. 89, 120–126. doi: 10.1136/jnnp-2017-316415
Jiang, B., Li, L., Chen, Q., Tao, Y., Yang, L., Zhang, B., et al. (2017). Role of glibenclamide in brain injury after intracerebral haemorrhage. Transl. Stroke Res. 8, 183–193.
John, R. F., and Colbourne, F. (2016). Delayed localised hypothermia reduces intracranial pressure following collagenase-induced intracerebral hemorrhage in rat. Brain Res. 1633, 27–36. doi: 10.1016/j.brainres.2015.12.033
Josephson, S. A., Keezer, M. A., and Wiebe, S. (2022). Inducers and cardiovascular risk-potential role for lowered drug exposure-reply. JAMA Neurology 79, 468–477. doi: 10.1001/jamaneurol.2022.0044
Jung, K., Chu, K., Jeong, S-. W., Han, S., Lee, S-. T., Kim, J. -Y., et al. (2004). HMG-CoA reductase inhibitor, atorvastatin, promotes sensorimotor recovery, suppressing acute inflammatory reaction after experimental intracerebral haemorrhage. Stroke. 35, 1744–1749. doi: 10.1161/01.STR.0000131270.45822.85
Kadir, R. R. A., Alwjwaj, M., and Bayraktutan, U. (2022a). Protein kinase C-β distinctly regulates blood-brain barrier-forming capacity of Brain Microvascular endothelial cells and outgrowth endothelial cells. Metab. Brain Dis. 37, 1815–1827. doi: 10.1007/s11011-022-01041-1
Kadir, R. R. A., Alwjwaj, M., and Bayraktutan, U. (2022b). Treatment with outgrowth endothelial cells protects cerebral barrier against ischemic injury. Cytotherapy 24, 5. doi: 10.1016/j.jcyt.2021.11.005
Kadir, R. R. A., Alwjwaj, M., McCarthy, Z., and Bayraktutan, U. (2021). Therapeutic hypothermia augments the restorative effects of PKC-β and Nox2 inhibition on an in vitro model of human blood–brain barrier. Metab. Brain Dis. 36, 1817–1832. doi: 10.1007/s11011-021-00810-8
Kahle, K. T., Simard, J. M., Staley, K. J., Nahed, B. V., Jones, P. S., Sun, D., et al. (2009). Molecular mechanisms of ischaemic cerebral oedema: role of electroneutral ion transport. Physiology (Bethesda) 24, 257–265. doi: 10.1152/physiol.00015.2009
Kanthan, R., Shuaib, A., Griebel, R., el-Alazounni, H., Miyashita, H., and Kalra, J. (1995). Intracerebral human microdialysis: invivo study of an acute focal ischaemic model of the human brain. Stroke 26, 870–873. doi: 10.1161/01.STR.26.5.870
Keep, R. F., Hua, Y., and Xi, G. (2012). Intracerebral haemorrhage: mechanisms of injury and therapeutic targets. Lancet Neurol. 11, 720–731. doi: 10.1016/S1474-4422(12)70104-7
Kim-Han, J. S., Kopp, S. J., and Dugan, L. L. (2006). Perihematomal mitochondrial dysfunction after intracerebral hemorrhage. Stroke 2006, 2457–62. doi: 10.1161/01.STR.0000240674.99945.4e
Kitaoka, T., Hua, Y., Xi, G., Hoff, J. T., and Keep, R. F. (2002). Delayed argatroban treatment reduces edema in rat model of intracerebral haemorrhage. Stroke 33, 3012–3018. doi: 10.1161/01.STR.0000037673.17260.1B
Krishnamurthy, R. V., Ikeda, T., and Feigin, V. L. (2020). Global, regional and country specific burden of ischaemic stroke, intracerebral haemorrhage and subarachnoid haemorrhage: a systematic analysis of the global burden of disease study 2017. Neuroepidemiology 54, 171–179. doi: 10.1159/000506396
Kritis, A., Stamols, E. G., Paniskaki, K. A., and Vavilis, T. D. (2015). Researching glutamate—induced cytotoxicity in different cell lines: a comparative/collective analysis/study. Front. Cell. Neurosci. 9, 91. doi: 10.3389/fncel.2015.00091
Kung, T. F. C., Wilkinson, C. M., Dirks, C. A., Jickling, G. C., and Colbourne, F. (2021). Glibenclamide does not improve outcome following severe collagenase-induced intracerebral hemorrhage in rats. PLoS ONE 16, e0252584. doi: 10.1371/journal.pone.0252584
La Mantia, L., Tramacere, I., Firwana, B., Pacchetti, I., Palumbo, R., and Fillippini, G. (2016). Fingolimod for relapsing-remitting multiple sclerosis. cochrane database of systematic reviews. 4, CD009371. doi: 10.1002/14651858.CD009371.pub2
Lattanzi, S., Napoli, M. D., Ricci, S., and Divani, A. (2020). Matrix metalloproteinases in acute intracerebral haemorrhage. Neurotherapeutics 17, 484–496. doi: 10.1007/s13311-020-00839-0
Leasure, A. C., Qureshi, A. I., Murthy, S. B., Kamel, H., Goldstein, J. N., Walsh, K. B., et al. (2019). Intensive blood pressure reduction and perihematomal oedema expansion in deep intracerebral haemorrhage. Stroke 50, 2016–2022. doi: 10.1161/STROKEAHA.119.024838
Lee, I. N., Cheng, W. C., Chung, C. Y., Lee, M., Lin, M., Kuo, C., et al. (2015). Dexamethasone reduces brain cell apoptosis and inhibits inflammatory response in rates with intracerebral haemorrhage. J Neuro Sci. 93, 178–188. doi: 10.1002/jnr.23454
Lee, K. R., Betz, A. L., and Kim, S. (1995). The role of the complement cascade in brain oedema formation after intracerebral haemorrhage. Acta Neurochir (WIen). 138, BF01420301. doi: 10.1007/BF01420301
Lee, S., Chu, K., Jung, K., Kim, J., Kim, E., Kim, S., et al. (2006). Memantine reduces hematoma expansion in experimental intracerebral hemorrhage, resulting in functional improvement. J. Cereb. Blood Flow Metabol. 26, 536–544. doi: 10.1038/sj.jcbfm.96002132006
Lee, S., Park, H-. K., Ryu, W., Lee, J-. S., Bae, H., Han, M-. K., et al. (2013). Effects of celecoxib on hematoma and edema volumes in primary intracerebral hemorrhage: a multicenter randomised controlled trial. Eur. J. Neurol. 20, 1161–1169. doi: 10.1111/ene.12140
Leonardi-Bee, J., Bath, P. M. W., Phillips, S. J., Sandercock, P. A. G., and Group, I. C. (2002). Blood pressure and clinical outcomes in the International Stroke Trial. Stroke 33, 1315–1320. doi: 10.1161/01.STR.0000014509.11540.66
Li, C., Meng, X., Wang, L., Wang, F., and Feng, F. (2022). Mitigating the effects of Endothelin-1 following a minimally invasive surgery reduces the blood-brain barrier permeability in a rabbit model of intracerebral haemorrhage. Brain Haemorrhages 3, 177–183. doi: 10.1016/j.hest.2022.06.004
Li, J., Jia, Z., Xu, W., Guo, W., Zhang, M., Bi, J., et al. (2019). TGN-020 alleviates oedema and inhibits astrocyte activation and glial scar formation after spinal cord compression in rats. Life Sci. 222, 148–157. doi: 10.1016/j.lfs.2019.03.007
Li, N., Worthmann, H., Heeren, M., Schuppner, R., Deb, M., Tryc, A. B., et al. (2013). Temporal pattern of cytotoxic oedema in the perihematomal region after intracerebral haemorrhage: a serial magnetic resonance imaging study. Stroke. 44, 1144–1146. doi: 10.1161/STROKEAHA.111.000056
Linfante, I., Llinas, R. H., Caplan, L. R., and Warach, C. (1999). MRI features of intracerebral haemorrhage wihin 2 hours from symptom onset. Stroke 30, 2263–2267. doi: 10.1161/01.STR.30.11.2263
Liu, S., Liu, X., Chen, S., Xiang, Y., and Zhuang, W. (2019). Neutrophil-lymphocyte ratio predicts the outcome of intracerebral haemorrhage. Medicine (Baltimore). 98, 26. doi: 10.1097/MD.0000000000016211
Loach, B., and Bayraktutan, U. (2016). Evaluation of molecular mechanisms involved in therapeutic hypothermia-mediated neurovascular protection. J. Neurol. Exp. Neural. Sci. 2016, 115. doi: 10.29011/JNNS-115.100015
Loach, B., and Bayraktutan, U. (2017). Hypothermia: a therapeutic option in management of stroke? Stroke Res Ther. 2017, 2.
Lovasik, B. P., McCracken, D. J., McCracken, C. E., McDougal, M., Frerich, J., Samuel, O., et al. (2016). The effect of external ventricular drain use in intracerebral haemorrhage. World Neurosurg. 94, 309–318. doi: 10.1016/j.wneu.2016.07.022
Lu, L., Barfejani, A. H., Qin, T., Dong, Q., Ayata, C., Waeber, C., et al. (2014). Fingolimod exerts neuroprotective effects in a mouse model of intracerebral haemorrhage. Brain Res. 1555, 89–96. doi: 10.1016/j.brainres.2014.01.048
Lyden, P. D., Shuaib, A., Lees, K. R., Davalos, A., Davis, S. M., Diener, H. C., et al. (2007). Safety and tolerability of NXY-059 for acute intracerebral hemorrhage: the CHANT trial. Stroke 38, 2262–2269. doi: 10.1161/STROKEAHA.106.472746
Ma, L., Xing, G-. P., Yu, Y., Liang, H., Yu, T., Zheng, W-. H., et al. (2015). Sulforaphane exerts neuroprotective effects via suppression of the inflammatory response in a rat model of focal cerebral ischaemia. Int. J. Clin. Exp. Med. 8, 17811–17817.
Mayzler, O., Leon, A., Eilig, I., Fuxman, Y., Benifla, M., Freixo, P. C., et al. (2006). The effect of hypertonic (3%) saline with and without furosemide on plasma osmolality, sodium concentration and brain water content after closed head trauma in rats. J. Neurosurg Anaesthesiol. 18, 24–31. doi: 10.1097/01.ana.0000188358.41284.cb
Melmed, K. R., and Lyden, P. D. (2017). Meta-analysis of pre-clinical trials of therapeutic hypothermia for intracerebral haemorrhage. Ther. Hypothermia Temp. Manag. 7, 141–146. doi: 10.1089/ther.2016.0033
Mendelow, A. D., Gregson, B. A., Fernandes, H. M., Murray, G. D., Teasdale, G. M., Hope, D. T., et al. (2005). Early surgery versus initial conservative treatment in patients with spontaneous supratentorial intracerebral hematomas in the International Surgical Trial in Intracerebral Haemorrhage (STICH): a randomised trial. Lancet. 365, 387–397. doi: 10.1016/S0140-6736(05)70233-6
Mendelow, A. D., Gregson, B. A., Rowan, E. N., Murray, G. D., Gholkar, A., Mitchell, P. M., et al. (2013). Early surgery versus initial conservative treatment in patients with spontaneous supratentorial lobar intracerebral hematomas (STICH II): a randomised trial. Lancet 382, 397–403. doi: 10.1016/S0140-6736(13)60986-1
Miao, H., Jiang, Y., Geng, J., Zhang, B., Zhu, G., Tang, J., et al. (2020). Administration confers neuroprotection after experimental intracerebral haemorrhage in rats via NLRP3 suppression. J. Stroke Cerebrovas. Dis. 29, 104468. doi: 10.1016/j.jstrokecerebrovasdis.2019.104468
Misra, U. K., Kalita, J., and Vajpayee, A. (2007). Effect of single manitol bolus in intracerebral haemorrhage. Eur. J. Neurol. 14, 1118–1123. doi: 10.1111/j.1468-1331.2007.01918.x
Mould, W. A., Carhuapoma, J. R., Muschelli, J., Lane, K., Morgan, T. C., McBee, N. A., et al. (2013). Minimally invasive surgery plus recombinant tissue-type plasminogen activator for intracerebral hemorrhage evacuation decreases perihematomal edema. Stroke. 44, 627–634.
Moullali, T. J., Wang, X., Sandset, E. C., Woodhouse, L. J., Law, Z, K., Arima, H., et al. (2022). Early lowering of blood pressure after acute intracerebral haemorrhage: a systematic review and meta-analysis of individual patient data. JNNP 93, 6–13.
Moxon-Emre, I., and Schilchter, L. C. (2011). Neutrophil depletion reduces blood-brain barrier breakdown, axon injury and inflammation after intracerebral haemorrhage. J. Neuropathol. Exp. Neurol. 70, 0b013e31820d94a5. doi: 10.1097/NEN.0b013e31820d94a5
Murthy, S. B., Moradiya, Y., Dawson, J., Lees, K. R., Hanley, D. F., Ziai, W. C., et al. (2015). Perihematomal oedema and functional outcomes in intracerebral haemorrhage: influence of hematoma volume and location. Stroke 46, 3088–3092. doi: 10.1161/STROKEAHA.115.010054
Nagatsuna, T., Nomura, S., Suehiro, E., Fujisawa, H., Koizumi, H., Suzuki, M., et al. (2005). Systemic administration of argatroban reduces secondary brain damage in a rat model of intracerebral hemorrhage: histopathological assessment. Cerebrovascular Dis. 2005, 192–200. doi: 10.1159/000083466
Nakamura, T., Keep, R. F., Hua, Y., Schallert, T., Hoff, J. T., Xi, G., et al. (2004). Deferoxamine-induced attenuation of brain edema and neurological deficits in a rat model of intracerebral hemorrhage. Neurosurgery 100, 672–678. doi: 10.3171/jns.2004.100.4.0672
NCT02258919 (2023). “Decompressive hemicraniectomy in intracerebral hemorrhage (SWITCH),” in Clinical Trialsgov.
NCT02880878 (2023). “ENRICH: Early minimally invasive removal of intracerebral haemorrhage,” in Clinical Trialsgov.
NCT03338998 (2022). “Efficacy Safety and Tolerability of BAF312 Compared to Placebo in Patients With Intracerebral Haemorrhage,” in ClinicalTrialsgov.
NCT03741530 (2022). “Glibenclamide Advantage in Treating Edema After Intracerebral Hemorrhage (GATE-ICH),” in Clinical Trialsgov.
NCT04714177 (2021). “Edaravone Dexborneol for the Treatment of Hypertensive Cerebral Haemorrhage (ED-ICH),” in Clinical Trialsgov.
Okauchi, M., Hua, Y., Keep, R. F., Morgenstern, L. B., and Xi, G. (2009). Effects of deferoxamine on intracerebral haemorrhage-induced brain injury in aged rats. Stroke 40, 1858–1863. doi: 10.1161/STROKEAHA.108.535765
Okuda, M., Suzuki, R., Moriya, M., Fujimoto, M., Chang, C. W., and Fujimoto, T. (2006). The effect of hematoma removal for reducing the development of brain oedema in cases of putaminal haemorhage. Acta Neurochir. Suppl. 96, 74–77. doi: 10.1007/3-211-30714-1_18
Olivot, J. M., Mlynash, M., Kleinman, J. T., Strake, M., Venkatasubramiyam, C., Bammer, V., et al. (2010). MRI profile of the perihematomal region in acute intracerebral haemmorrhage. Stroke. 41, 2681–2683. doi: 10.1161/STROKEAHA.110.590638
Parry-Jones, A. R., Wang, X., Sato, S., Mould, W. A., Vail, A., Anderson, C. S., et al. (2015). Edema extension distance: outcome measure for phase i. clinical trials targeting edema after intracerebral haemorrhage. Stroke 46, e 137–e 140. doi: 10.1161/STROKEAHA.115.008818
Peng, W., Li, Q., and Tang, J. (2019). The risk factors and prognosis of delayed perihematomal edema in patients with spontaneous intracerebral hemorrhage. CNS Neurosci. Ther. 25, 1189–94. doi: 10.1111/cns.13219
Pías-Peleteiro, J., Pérez-Mato, M., López-Arias, E., Rodríguez-Yáñez, M., Blanco, M., Campos, F., et al. (2016). Increased endothelial progenitor cell levels are associated with good outcome in intracerebral haemorrhage. Sci. Rep. 6, srep28724. doi: 10.1038/srep28724
Pollay, M., Fullenwider, C., and Roberts, P. A. (1983). Effect of mannitol and furosemide on blood-brain osmotic gradient and intracranial pressure. JNS. 59, 945–950. doi: 10.3171/jns.1983.59.6.0945
Qing, W. G., Dong, Y. Q., Ping, T. Q., Lai, L. G., Fang, L. D., Min, H. W., et al. (2009). Brain oedema after intracerebral haemorrhage in rats: the role of iron overload and aquaporin-4. J. Neurosurg. 110, 462–468. doi: 10.3171/2008.4.JNS17512
Qureshi, A. I., Palesch, Y. Y., Martin, R., Novitzke, J., Cruz-Flores, S., Ehtisham, A., et al. (2011). Association of serum glucose concentrations during acute hospitalisation with hematoma expansion, perihematomal edema, and three month outcome among patients with intracerebral hemorrhage. Neurocrit. Care. 15, 428–435. doi: 10.1007/s12028-011-9541-8
Qureshi, A. I., Wilson, D. A., and Traystman, R. J. (1999). Treatment of elevated intracranial pressure in experimental intracerebral haemorrhage: comparison between mannitol and hypertonic saline. Neurosurgery 44, 1055–1064. doi: 10.1097/00006123-199905000-00064
Rakkar, K., Srivastava, K., and Bayraktutan, U. (2014). Attenuation of urokinase activity during experimental ischaemia protects the cerebral barrier from damage through regulation of matrix metalloproteinase-2 and NAD(P)H oxidase. Eur. J. Neurosci. 39, 12552. doi: 10.1111/ejn.12552
Ratcliff, J. J., Hall, A. J., Porto, E., Saville, B. R., Lewis, R. J., Allen, J. W., et al. (2023). Early minimally invasive removal of intracerebral hemorrhage (ENRICH): study protocol for a multi-centred two-arm randomised adaptive trial. Front. Neurol. 14, 1126958. doi: 10.3389/fneur.2023.1126958
Reulen, H. J., Graham, R., Spatz, M., and Klatzo, I. (1977). Role of pressure gradients and bulk flow in dynamics of vasogenic oedema. J. Neurosurg. 46, 24–35. doi: 10.3171/jns.1977.46.1.0024
Rickard, A. C., Smith, J. E., Newell, P., Bailey, A., Kehoe, A., and Mann, C. (2014). Salt or sugar for your injured brain? A meta-analysis of randomised controlled trials of mannitol versus hypertonic sodium solutions to manage raised intracranial pressure in traumatic brain injury. Emerg. Med. J. 31, 679–683. doi: 10.1136/emermed-2013-202679
Sansing, L. H., Harris, T. H., Kasner, S. E., Hunter, C. A., and Kariko, K. (2011a). Neutrophil depletion diminishes monocyte infiltration and improves functional outcome after experimental intracerebral haemorrhage. Acta Neurochir. Suppl. 111, 173–178. doi: 10.1007/978-3-7091-0693-8_29
Sansing, L. H., Harris, T. H., Welsh, F. A., Kasner, S., Hunter, C., Kariko, K., et al. (2007). Toll-like receptor 4 contributes to poor outcome after intracerebral haemorrhage. Ann. Neurol.70, 646–656. doi: 10.1002/ana.22528
Sansing, L. H., Kaznatcheeva, E. A., Perkins, C. J., Komaraff, E., Gutman, F., and Newman, G. (2003). Edema after intracerebral haemorrhage: correlation with coagulation parameters and treatment. J. Neurosurg. 98, 985–992. doi: 10.3171/jns.2003.98.5.0985
Sansing, L. H., Messe, S. R., Cucchiara, B. L., Lyden, P. D., and Kasner, S. (2011b). Antiadrenergic medications and oedema development after intracerebral haemorrhage. Neurocrit. Care. 14, 395–400. doi: 10.1007/s12028-010-9498-z
Saver, J. L., Kidwell, C., Eckstein, M., Starkman, S., and Investigators, F.-.M. P. T. (2004). Prehospital neuroprotective therapy for acute stroke. Results of the field administration of stroke therapy-magnesium (FAST-MAG) pilot trial. Stroke 35, 106–108. doi: 10.1161/01.STR.0000124458.98123.52
Saver, J. L., Starkman, S., Eckstein, M., Stratton, S. J., Pratt, F. D., Hamilton, S., et al. (2013). Prehospital use of magnesium sulfate as neuroprotection in acute stroke. N. Engl. J. Med. 372, 528–536. doi: 10.1056/NEJMoa1408827
Schlunk, F., Pfeilschifter, W., Yigitani, K., Lo, E. H., and Foerch, C. (2016). Treatment with FTY720 has no beneficial effects on short-term outcome in an experimental model of intracerebral hemorrhage. Exp. Transl. Stroke Med. 8, 1.
Schreibman, D. L., Hong, C. M., Keledjian, K., Ivanova, S., Tsymbalyuk, S., Gerzanich, V., et al. (2018). Mannitol and hypertonic saline reduce swelling and modulate inflammatory markers in a rat model of intracerebral haemorrhage. Neurocrit. Care. 29, 253–263. doi: 10.1007/s12028-018-0535-7
Seiffge, D. J., Polymeris, A. A., Law, Z. K., Krishnan, K., Zietz, A., Thilemann, S., et al. (2022). Cerebral amyloid angiopathy and the risk of hematoma expansion. Ann. Neurol. 92, 921–930. doi: 10.1002/ana.26481
Selim, M., Foster, L. D., Moy, C. S., Xi, G., Hill, M. D., Morgenstern, L. B., et al. (2019). Deferoxamine mesylate in patients with intracerebral haemorrhage (i-DEF): a multicentre, randomised, placebo-controlled, double-blind phase 2. Trial. 18, 5. doi: 10.1016/S1474-4422(19)30069-9
Selim, M., Yeatts, S., Goldstein, J. N., Gomes, J., Greenberg, S., Morgenstern, L. B., et al. (2011). Safety and tolerability of deferoxamine mesylat in patients with acute intracerebral hemorrhage. Stroke 42, 3067–3074. doi: 10.1161/STROKEAHA.111.617589
Serebruany, V. (2006). NXY-059 for acute ischemic stroke. N. Engl. J. Med. 354, 588–600. doi: 10.1056/NEJMoa052980
Shah, M., Birnbaum, L., Rasmussen, J., Sekar, P., Moomaw, C. J., Osborne, J., et al. (2018). Effect of hyperosmolar therapy on outcome following spontaneous intracerebral haemorrhage: Ethnic/Racial variations of Intracerebral Haemorrhage (ERICH) study. J. Stroke Cerebrovascular Dis. 27, 1061–1067. doi: 10.1016/j.jstrokecerebrovasdis.2017.11.013
Shakur, H., Roberts, I., Bautista, R., Caballero, J., Coats, T., Dewan, Y., et al. (2010). Effects of tranexamic acid on death, vascular occlusive events, and blood transfusion in trauma patients with significant haemorrhage (CRASH-2): a randomised, placebo-controlled trial. Lancet 376, 23–32. doi: 10.1016/S0140-6736(10)60835-5
Shao, B., and Bayraktutan, U. (2014). Hyperglycaemia promotes human brain microvascular endothelial cell apoptosis via induction of protein kinase C-βI and prooxidant enzyme NADPH oxidase. Redox Biol. 2, 694–701. doi: 10.1016/j.redox.2014.05.005
Sheth, K. N., Simard, J. M., Elm, J., Kronenberg, G., and Kunte, H. (2016). Human data supporting glyburide in ischaemic stroke. Acta Neurochir. Suppl. 121, 13–18. doi: 10.1007/978-3-319-18497-5_3
Shuaib, A., Kanthan, R., Goplen, G., Griebel, R., el-Azzouni, H., Miyashita, H., et al. (2003). Extracellular glutamate and other amino acids in experimental intracerebral haemorrhage: an invivo microdialysis study. Crit. Care Med. 31, 1482–1489. doi: 10.1097/01.CCM.0000063047.63862.99
Shuaib, A., Lees, K. R., Lyden, P., Grotta, J., Davalos, A., Davis, S. M., et al. (2007). NXY-059 for the treatment of acute ischemic stroke. N. Engl. J. Med. 357, 562–571. doi: 10.1056/NEJMoa070240
Simard, J. M., Chen, M., Tarasov, K. V., Bhatta, S., Ivanova, S., Melnitchenko, L., et al. (2006). Newly expressed SUR-1 regulated NC(ca-ATP) channel mediates cerebral oedema after stroke. Nat. Med. 12, 433–440. doi: 10.1038/nm1390
Simard, J. M., Geng, Z., Woo, S. K., Ivanova, S., Tosun, C., Melnichenko, L., et al. (2009). Glibenclamide reduces inflammation, vasogenic oedema and caspase-3 activation after subarachnoid haemorrhage. J. Cerebral Blood Flow Metabol. 29, 317–330. doi: 10.1038/jcbfm.2008.120
Smith, C. J., Hulme, S., Vail, A., Heal, C., Parry-Jones, A. R., Scarth, S., et al. (2018). SCIL-STROKE (subcutaneous interleukin-1 receptor antagonist in ischemic stroke): a randomised controlled phase 2 trial. Stroke 49, 1210–1216. doi: 10.1161/STROKEAHA.118.020750
Sorani, M. D., and Manley, G. T. (2008). Dose-response relationship of mannitol and intracranial pressure: a meta-analysis. J. Neurosurg. 108, 80–87. doi: 10.3171/JNS/2008/108/01/0080
Sorani, M. D., Morabito, D., Rosenthal, G., Giacomini, K. M., and Manley, G. T. (2008). Characterizing the dose-response relationship between mannitol and intracranial pressure in traumatic brain injury patients using a high-frequency physiological data collection system. J. Neurotrauma. 25, 291–298. doi: 10.1089/neu.2007.0411
Sprigg, N., Flaherty, K., Appleton, J. P., Salman, R. A., Bereczki, D., Beridze, M., et al. (2018). Tranexamic acid for hyperacute primary IntraCerebral Haemorrhage (TICH-2): an international randomised, placebo-controlled, phase 3 superiority trial. Lancet 10135, 2107–2115. doi: 10.1016/S0140-6736(18)31033-X
Staykov, D., Huttner, H. B., Köhrmann, M., Bardutzky, J., and Schellinger, P. D. (2010). Novel approaches to the treatment of intracerebral haemorrhage. Int. J. Stroke. 5, 457–465. doi: 10.1111/j.1747-4949.2010.00487.x
Staykov, D., Wagner, I., and Volbers, B. (2013). Mild prolonged hypothermia for large intracerebral haemorrhage. Neurocrit. Care. 18, 178–183. doi: 10.1007/s12028-012-9762-5
Staykov, D., Wagner, I., Volbers, B., Haueer, E., Doerfler, A., Schwab, S., et al. (2011). Natural course of perihaemorrhagic oedema after intracerebral haemorrhage. Stroke 42, 2625–2629. doi: 10.1161/STROKEAHA.111.618611
Stokum, J. A., Kwon, M. S., Woo, S. K., Tsymbalyuk, O., Vennekens, R., Gerzanich, V., et al. (2018). SUR1-TRPM4 and AQP4 form a heteromultimeric complex that amplifies ion/water osmotic coupling and drives astrocyte swelling. Glia 66, 108–125. doi: 10.1002/glia.23231
Sun, S., Li, Y., Zhang, H., Wang, X., She, L., Yan, Z., et al. (2018). The effect of mannitol in the early stage of supratentorial hypertensive intracerebral haemorrhage: a systematic review and meta-analysis. World Neurosurg. 24, 386–396. doi: 10.1016/j.wneu.2018.11.249
Sykora, M., Diedler, J., Turcani, P., Rupp, A., and Steiner, T. (2009). Subacute perihematomal oedema in intracerebral haemorrhage is associated with impaired blood pressure regulation. J. Neurol. Sci. 284, 108–112. doi: 10.1016/j.jns.2009.04.028
Tan, Q., Li, Y., Guo, P., Zhou, J., Jiang, Z., Liu, X., et al. (2019). Tolvaptan attenuated brain edema in experimental intracerebral haemorrhage. Brain Res. 1715, 41–46. doi: 10.1016/j.brainres.2019.01.033
Tapia-Perez, J. H., Rupa, R., Zilke, R., Gehring, S., Voellger, B., and Schneider, T. (2013). Continued statin therapy could improve the outcome after spontaneous intracerebral haemorrhage. Neurosurg. Rev. 36, 279–287. doi: 10.1007/s10143-012-0431-0
Thenuwara, K., Todd, M. M., and Brian Jr, J. E. (2002). Effect of mannitol and furosemide on plasma osmolality and brain water. Anaesthesiology 96, 416–421. doi: 10.1097/00000542-200202000-00029
Trial Collaborators WOMAN (2017). Effect of early tranexamic acid administration on mortality, hysterectomy, and other morbidities in women with post-partum haemorrhage (WOMAN): an international, randomised, double-blind, placebo-controlled trial. Lancet 389, 2105–2116. doi: 10.1016/S0140-6736(17)30638-4
Tschoe, C., Bushnell, C. D., Duncan, P. W., Miller, M., and Wolfe, S. (2020). Neuroinflammation after intracerebral haemorrhage and potential therapeutic targets. J. Stroke 22, 29–46. doi: 10.5853/jos.2019.02236
Tsivgoulis, G., Katsanos, A. H., Butcher, K. S., Boviatsis, E. J., Triantafyllou, N., Rizos, I., et al. (2014). Intensive blood pressure reduction in acute intracerebral hemorrhage: a meta-analysis. Neurology 83, 1523–1529. doi: 10.1212/WNL.0000000000000917
Urday, S., Beslow, L. A., and Dai, F. (2016). Rate of perihematomal oedema expansion predicts outcome after intracerebral haemorrhage. Crit. Care Med. 44, 790–797. doi: 10.1097/CCM.0000000000001553
van Asch, C., Luitse, M., Rinkel, G., van der Tweel, I., Algra, A., Klijn, C., et al. (2010). Incidence, case fatality, and functional outcome of intracerebral haemorrhage overtime, according to age, sex, and ethnic origin: a systematic review and meta-analysis. Lancet Neurol. 9, 167–176. doi: 10.1016/S1474-4422(09)70340-0
Venkatasubramanian, C., Mlynash, M., Finley-Caulfield, A., Eyngorn, I., Kalimuthu, R., Snider, R. W., et al. (2011). Natural history of perihematomal edema after intracerebral hemorrhage measured by serial magnetic resonance imaging. Stroke 42, 73–80. doi: 10.1161/STROKEAHA.110.590646
Volbers, B., Willfarth, W., and Kuramatsu, J. B. (2016). Impact of perihaemorrhagic oedema on short-term outcome after intracerebral haemorrhage. Neurocrit. Care. 24, 404–441. doi: 10.1007/s12028-015-0185-y
Wagner, I., Hauer, E. M., Staykov, D., Volbers, B., Dorfleirs, A., Schwab, S., et al. (2011). Effects of continous hypertonic saline infusion on perihaemorrhagic oedema evolution. Stroke 42, 1540–1545. doi: 10.1161/STROKEAHA.110.609479
Wagner, K. R., Xi, G., Hua, Y., Kleinholz, M., Courten-Myers, G. M., de Myers, R., et al. (1996). Lobar intracerebral hemorrhage model in pigs: rapid edema development in perihematomal white matter. Stroke 196, 490–497. doi: 10.1161/01.STR.27.3.490
Wang, J., and Dore, S. (2007). Inflammation after intracerebral haemorrhage. J. Cerebral blood Flow Metabol. 27, 894–908. doi: 10.1038/sj.jcbfm.9600403
Wang, J., Wang, G., Yi, J., Xu, Y., Duan, S., Li, T., et al. (2017). The effect of monascin on hematoma clearance and edema after intracerebral hemorrhage in rats. Brain Res. Bull. 134, 24–29. doi: 10.1016/j.brainresbull.2017.06.018
Wang, L., Wang, F., Wu, G., and Shi, J. (2015). Early-stage minimally invasive procedures decrease perihematomal endothelin-1 levels and improve neurological functioning in a rabbit model of intracerebral hemorrhage. Neurol. Res. 37, 320–327. doi: 10.1179/1743132814Y.0000000446
Wang, L. K., Hong, Z., and Wu, G. F. (2013). Perihematomal endothelin-1 level is associated with an increase in blood-brain barrier permeability in a rabbit model of intracerebral hematoma. Chin Med J (Engk). 126, 3433–3438.
Wang, X., Arima, H., Yang, J., et al. (2015). Mannitol and outcome in intracerebral haemorrhage. Stroke 46, 2767–2757. doi: 10.1161/STROKEAHA.115.009357
Wasserman, J. K., and Schlichter, L. C. (2007). Minocycline protects the BBB and reduces edema following intracerebral hemorrhage in the rat. Exp. Neurol. 207, 227–237. doi: 10.1016/j.expneurol.2007.06.025
Wei, C., Wang, J., Foster, L. D., Yeatts, S. D., Moy, C., Mocco, J., et al. (2021). Effect of deferoxamine on outcome according to baseline hematoma volume: a post hoc analysis of the I-DEF trial. Stroke 53, 1149–1156. doi: 10.1161/STROKEAHA.121.035421
Wilkinson, C. M., Fedor, B. A., Aziz, J. R., Nadeau, C. A., Brar, P. S., Clark, J. J. A., et al. (2019). Failure of bumetanide to improve outcome after intracerebral haemorrhage in rat. PLoS ONE. 14, e0210660. doi: 10.1371/journal.pone.0210660
Willmot, M., Leonardi-Bee, J., and Bath, P. M. W. (2004). High blood pressure in acute stroke and subsequent outcome—a systematic review. Hypertension 43, 18–24. doi: 10.1161/01.HYP.0000105052.65787.35
Wintzer, S., Hechmann, J. G., Huttner, H. B., and Schwab, S. (2020). Dexamethasone in patients with spontaneous intracerebral haemorrhage: an updated meta-analysis. Cerebrovasc Dis. 49, 495–502. doi: 10.1159/000510040
Wolfe, S. Q. (2022). “Fingolimod as a Treatment of Cerebral Edema After Intracerebral Hemorrhage (FITCH),” in ClInicaltrialsgovNCT04088630.
Wu, G., Wang, L., Wang, F., Feng, A., and Sheng, F. (2013). Minimally invasive procedures for intracerebral hematoma evacuation in early stages decrease perihematomal glutamate level and improve neurological function in a rabbit model of ICH. Brain Res. 1492, 140–147. doi: 10.1016/j.brainres.2012.11.023
Wu, G., Wu, J., Jiao, Y., Wang, L., Wang, F., Zhang, Y., et al. (2015). Rosiglitazone infusion therapy following minimally invasive surgery for intracerebral hemorrhage evacuation decreases matrix metalloproteinase-9 and blood–brain barrier disruption in rabbits. BMC Neurol. 15 doi: 10.1186/s12883-015-0287-3
Wu, J., Sun, L., Li, H., Shen, H., Zhai, W., Yu, Z., et al. (2017). Roles of programmed death protein 1/programmed death-ligand 1 in secondary brain injuyr after intracerebral haemorrhage in rats: selective modulation of microglia polarisation to anti-inflammatory phenotype. J. Neuroinflammation. 14 doi: 10.1186/s12974-017-0790-0
Wu, T. Y., Sharma, G., Strbian, D., Putaala, J., Desmound, P., Tatlisumak, T., et al. (2017). Natural history of perihematomal oedema and impact on outcomes after intracerebral haemorrhage. Stroke 48, 873–879. doi: 10.1161/STROKEAHA.116.014416
Xi, G., Hua, Y., Keep, R. F., Younger, J. G., and Hoff, J. T. (2002). Brain edema after intracerebral hemorrhage: the effects of systemic complement depletion. Acta Neurochir. Suppl. 81, 253–256. doi: 10.1007/978-3-7091-6738-0_66
Xi, G., Reiser, G., and Keep, R. F. (2003). The role of thrombin and thrombin receptors in ischemic, hemorrhagic and traumatic brain injury. J. Neurochem. 84, 3–9. doi: 10.1046/j.1471-4159.2003.01268.x
Xi, G., Wagner, K. R., Keep, R. F., Hua, Y., Courten-Myersde, G. M, Broderick, P., et al. (1998). Role of blood clot formation on early oedema development after experimental intracerebral haemorrhage. Stroke 29 doi: 10.1161/01.STR.29.12.2580
Xia, Z., Wu, X., Li, J., Liu, Z., Chen, F., Zhang, L., et al. (2018). Minimally invasive surgery is superior to conventional craniotomy in patients with spontaneous supratentorial intracerebral hemorrhage: a systematic review and meta-analysis. World Neurosurg. 115, 266–273.
Yang, D., Zhang, J., Han, Y., James, E., Chopp, M., and Seyfried, D. (2013). Acute statin treatment improves recovery after experimental intracerebral haemorrhage. World J. Neurosci. 3, 32010. doi: 10.4236/wjns.2013.32010
Yang, G. Y., Betz, A. L., Chenevert, T. L., Burnberg, J., and Hoff, J. (1994). Experimental intracerebral hemorrhage: relationship between brain edema, blood flow, and blood–brain barrier permeability in rats. J Neurosurg. 81, 93–102. doi: 10.3171/jns.1994.81.1.0093
Yang, J., Arima, H., Wu, G., Heeley, E., Delcourt, C., Zhou, J., et al. (2015). Prognostic significance of perihematomal oedema in acute intracerebral haemorrhage: pooled analysis from the intensive blood pressure reduction in acute cerebral haemorrhage studies. Stroke 46, 1009–1013. doi: 10.1161/STROKEAHA.114.007154
Yang, J., Lee, T-. H., Lee, I., Chung, C-. Y., Kuo, C., Weng, H-H., et al. (2011). Dexamethasone inhibits ICAM-1 and MMP-9 expression and reduces brain oedema in intracerebral haemorrhage rats. Acta Neurochir (WIen). 153, 2197–2203. doi: 10.1007/s00701-011-1122-2
Yang, Z., Liu, B., Zhong, L., Shen, H., Lin, C., Lin, L., et al. (2015). Toll-like receptor-4-mediated autophagy contributes to microglial activity and inflammatory injury in mouse models of intracerebral haemorrhage. Neuropathol. Appl. Neurobiol. 41, e95–e106. doi: 10.1111/nan.12177
Yao, Z., Ma, L., You, C., and He, M. (2018). Decompressive craniectomy for spontaneous intracerebral haemorrhage: a systematic review and meta-analysis. World Neurosurg. 110, 121–128. doi: 10.1016/j.wneu.2017.10.167
Yoshizaki, S., Kijima, K., Hara, M., Saito, T., Tamaru, T., Tanaka, M., et al. (2019). Tranexamic acid reduces haem cytotoxicity via the TLR/TNF axis and ameliorates functional recovery after spinal cord injury. J. Neuroinflammation. 16, 1536. doi: 10.1186/s12974-019-1536-y
Zazulia, A. R., Diringer, M. N., Derdeyn, C. P., and Powers, W. J. (1999). Progression of mass effect after intracerebral hemorrhage. Stroke 30, 1167–1173. doi: 10.1161/01.STR.30.6.1167
Zhang, M., Cui, Z., Cui, H., Cao, Y., Zhong, C., and Wang, Y. (2016). Astaxanthin alleviates cerebral oedema by modulating NKCC1 and AQP4 expression after traumatic brain injury. BMC Neurosci. 17, 2. doi: 10.1186/s12868-016-0295-2
Zhao, F., Hua, Y., Keep, R. F., Keep, R., and Xi, G. (2011). Minocycline-induced attenuation of iron overload and brain injury after experimental intracerebral haemorrhage. Stroke 42, 3587–3593. doi: 10.1161/STROKEAHA.111.623926
Zhao, J., Song, C., Li, D., Yang, X., Yu, L., Wang, K., et al. (2022). Efficacy and safety of glibenclamide therapy after intracerebral haemorrhage (GATE-ICH): a multicentre, prospective, randomised, controlled, open-label, blinded-endpoint, phase 2 clinical trial. eClinical Med. 53, 101666. doi: 10.1016/j.eclinm.2022.101666
Zhao, X., Sun, G., Ting, S., Song, S., Zhang, J., Edwards, N. J., et al. (2015a). Cleaning up after ICH: the role of Nrf2 in modulating microglia function and hematoma clearence. J. Neurochem. 133, 144–152. doi: 10.1111/jnc.12974
Zhao, X., Sun, G., and Zhang, J. (2007a). Transcription factor Nrf2 protects the brain from damage produced by intracerebral hemorrhage. Stroke 38, 3280–3286. doi: 10.1161/STROKEAHA.107.486506
Zhao, X., Sun, G., Zhang, J., Strong, R., Song, W., Gonzales, N., et al. (2007b). Hematoma resolution as a target for intracerebral haemorrhage treatment: role of peroxisone proliferator-activated gamma in microglia/macrophages. Ann. Neurol. 61, 352–362. doi: 10.1002/ana.21097
Zhao, X., Wu, C-. F., Yang, J., Gao, Y., Sun, F., Wang, D-X., et al. (2015b). Effect of arginine vasopressin on the cortex oedema in the ischaemic stroke of Mongolian gerbils. Neuropeptides.51, 55–62. doi: 10.1016/j.npep.2015.01.003
Zhao, X., Zhang, Y., Strong, R., Grotta, J. C., and Aronowski, J. (2006). 15.-d Prostaglandin activates peroxisome proliferator-activated receptor-gamma, promotes expression of catalase, and reduce inflammation, reduces inflammation, behavioural dysfunction, and neuronal loss after intracerebral haemorrhage in rats. J. Cereb. Blood Flow Metab. Clin. Exp. 26, 811–820. doi: 10.1038/sj.jcbfm.9600233
Zheng, H., Chen, C., Zhang, J., and Hu, Z. (2016). Mechanism and therapy of brain oedema after intracerebral haemorrhage. Cerebrovasc. Dis. 42, 155–169. doi: 10.1159/000445170
Zheng, Z. V., Lyu, H., Lam, S. Y. E., Lam, P. K., Poon, W. S., Wong, G. K. C., et al. (2020). The dynamics of microglial polarisation reveal the resident neuroinflammatory responses after subarachnoid hemorrhage. Transl. Stroke Res. 11, 433–449. doi: 10.1007/s12975-019-00728-5
Zhou, F., Liu, Y., Yang, B., and Hu, Z. (2018). Neuroprotective potential of glibenclamide is mediated by antioxidant and anti-apoptotic pathways in intracerebral haemorrhage. Brain Res. Bull. 142, 18–24. doi: 10.1016/j.brainresbull.2018.06.006
Zuo, Y., Cheng, G., Gao, D., Zhang, X., Zhen, H-. N., Zhang, W., et al. (2009). Gross-total hematoma removal of hypertensive basal ganglia haemorrhages: a long-term follow-up. J. Neuro. Sci. 287, 100–104. doi: 10.1016/j.jns.2009.08.046
Appendix
Keywords: intracerebral hemorrhage, cerebral edema, translation, clinical trial, treatment, pathophysiology
Citation: Krishnan K, Campos PB, Nguyen TN, Tan CW, Chan SL, Appleton JP, Law Z, Hollingworth M, Kirkman MA, England TJ, Roffe C, Macleod MJ, Dawson J, Bayraktutan U, Werring DJ, Sprigg N and Bath PM (2023) Cerebral edema in intracerebral hemorrhage: pathogenesis, natural history, and potential treatments from translation to clinical trials. Front. Stroke 2:1256664. doi: 10.3389/fstro.2023.1256664
Received: 11 July 2023; Accepted: 28 August 2023;
Published: 29 September 2023.
Edited by:
Benjamin Yq Tan, National University Hospital, SingaporeReviewed by:
Mervyn Jun Rui Lim, National University Hospital, SingaporeAlexander Tsiskaridze, Tbilisi State University, Georgia
Copyright © 2023 Krishnan, Campos, Nguyen, Tan, Chan, Appleton, Law, Hollingworth, Kirkman, England, Roffe, Macleod, Dawson, Bayraktutan, Werring, Sprigg and Bath. This is an open-access article distributed under the terms of the Creative Commons Attribution License (CC BY). The use, distribution or reproduction in other forums is permitted, provided the original author(s) and the copyright owner(s) are credited and that the original publication in this journal is cited, in accordance with accepted academic practice. No use, distribution or reproduction is permitted which does not comply with these terms.
*Correspondence: Kailash Krishnan, a2FpbGFzaC5rcmlzaG5hbkBudWgubmhzLnVr