- 1Systems Ecology and Ecological Modelling Laboratory, Department of Zoology, Visva-Bharati University, Santiniketan, West Bengal, India
- 2Aquatic Ecology and Fish Biology Laboratory, Department of Zoology, Visva-Bharati University, Santiniketan, West Bengal, India
- 3Department of Environmental Studies, Delhi School of Journalism, University of Delhi, New Delhi, India
Loss of native habitat has been identified as one of the crucial drivers of the growing biodiversity crisis, acting toward the deleterious effects on individual fitness, functional diversity, changes in species richness and community structure. The objective of the study was to review the species distribution modeling works done in the Indian subcontinent throughout the last decade (2012–2021) to highlight the urgent issues of biodiversity conservation and sustainable management. Species distribution model (SDM), ecological niche model (ENM), and habitat suitability model (HSM) are, in general, used to predict the niche of a species. The Scopus search engine result was used for conducting the literature search of peer-reviewed articles. Topics examined included estimation of species' potential distribution area, spread of introduced species, deadly diseases or pests, habitat loss or future range shift of species due to anthropogenic alterations and climate change. The analysis showed that the majority of the works were focused on predicting native species distribution patterns (n = 119, 82%) rather than the invasion of alien species in the native ecosystem (n = 26, 18%) indicating the further scope of research in these areas. The scientific articles highlighted that the modeling results could be utilized efficiently to design conservation areas if not biased toward charismatic species. A coordinating institute at the inter-ministerial level can help to undertake policy-related planning for sustainable management and execution of decisions.
Introduction
The existence of life on Earth has always been determined by the difference between origination and extinction (Jablonski, 2008). The current extinction rates are around 1,000 times higher than the background extinction rates, and in future, this will continue to increase (Pimm et al., 2014). Loss of native habitat has been identified as one of the crucial drivers of the growing biodiversity crisis, acting to the deleterious effects on individual fitness, functional diversity, changes in species richness, and community structure. The alarming implication of habitat loss or degradation is that many species can become regionally or locally extinct with a time lag, even if they do not face further habitat loss. Such extinction debt poses a significant and unnoticed challenge for biodiversity conservation across ecosystems and associated communities. For instance, species having populations near the extinction threshold and long generation times are prone to show extinction debt (Kuussaari et al., 2009). The Red list is said to be heavily biased toward evaluating the birds and mammalian species, but very little part of invertebrate species has been examined against those conservation criteria. If one considers the actual number of invertebrate species extinctions, it can be concluded by the estimation that the sixth mass extinction is underway (Cowie et al., 2022). Hence, understanding about habitat requirement for controlling native biodiversity loss and ecosystem functionality are crucial for applied research initiatives (Boesing et al., 2018).
Globally, the number of species having restricted suitable habitat ranges is quickly increasing, and it is also applicable to familiar taxa. These species are usually geographically restricted and disproportionately threatened or expected to be extinct. It is assumed that many unknown species also share similar patterns of crisis. Unfortunately, widely distributed species dominate the maps for conservation, whereas most species with small ranges are almost invisible (Pimm et al., 2014). South Asia today is one of the main centers of anthropogenic activities. Its complex biogeography creates zonation in regional biotic patterns and threatens species' existence (Hughes, 2017; Chowdhury et al., 2022).
The Indian subcontinent is a physiographical region in the southern part of Asia, consisting of countries namely Bangladesh, Bhutan, India, Nepal, Pakistan, Sri Lanka, and the Maldives (Kumar, 2012; Mann, 2014). There are four global biodiversity hotspots in the Indian subcontinent (Eastern Himalayas, Western Ghats-Sri Lanka, Indo-Burma, Sundaland, and Nicobar Islands) (Mittermeier et al., 1998). For instance, the four countries, namely—Bangladesh, India, Sri Lanka, and the Maldives, harbor a substantial number of mangroves of exceptional biodiversity value, including the world's largest mangrove forest—the Sundarbans. Conservation of these regions becomes more challenging as the taxonomic uncertainty is highly and significantly associated with many taxa that is greater than any other parts of the world (Hughes, 2017). Agriculture dependent economy of this region accelerated extreme competition for acquiring land that has eventually resulted in a fast habitat clearance as well as native resource exploitation (Chowdhury et al., 2022). Along with that, changing climate and invasive alien species (IAS) are two other significant contributors to biodiversity loss.
Distribution modeling aims to understand and visualize species spatial distribution under the future, present, or past climatic scenarios by relating its occurrence to the predictor variables (Guisan and Zimmermann, 2000). Species distribution model (SDM), ecological niche model (ENM), and habitat suitability model (HSM) are usually used for predicting suitable habitats, environmental tolerances, and species responses toward climate change (Guisan and Thuiller, 2005; Iverson et al., 2008; Sunday et al., 2012; Morán-Ordóñez et al., 2017; Bryn et al., 2021). Although SDM is used to predict the actual geographic ranges where a species may be found, ENM estimates the Grinnellian niche of the species. Predictions from the models can be applied to make inferences about community assemblages, evolution, and dynamics that would otherwise not be available to researchers working with local field data only (Murphy and Smith, 2021). The sustainable management of Indian subcontinental flora and fauna is an essential issue of global concern for its richness and presence of several endemic species. Thus, the objective of the study was to review the species distribution modeling works done in the Indian subcontinent throughout the last decade (2012–2021) to estimate the effects of climate change and anthropogenic alterations on native species, and determine the chance of invasive alien species, or disease infestation.
Materials and methods
Literature search and paper selection
For collating the literature from the Indian subcontinent, a two-stage process was followed. Firstly, the Scopus search engine was used for conducting scientific literature searches of peer-reviewed journals that focussed on the distribution models (a similar method was followed by Urbina-Cardona et al., 2019; Feldman et al., 2021). The search spanned a period of 10 years in the last decade, considering papers from 2012 until 31st December 2021. The articles were searched using the following combination of keywords: (“species distribution modeling” OR “Habitat suitability modeling” OR “Ecological niche modeling”) AND (“Bangladesh” OR “Bhutan” OR “India” OR “Nepal” OR “Pakistan” OR “Sri Lanka”) AND (“English”). The initial search gave about 199 articles (on 1st January 2022).
Secondly, the title and the abstract were thoroughly checked for all the articles from the initial list. For considering an appropriate paper for the review, three conditions were followed, (1) the region of the study was situated within the Indian subcontinent, (2) the occurrence data were the presence or abundance points of the selected species, and (3) relationship between species occurrence points with environmental data was estimated by any statistical method followed by a proper validation method. In this way, repeated or same literature and overwhelmingly non-relevant works were eliminated.
After an initial scan, 41 articles were eliminated before the following analysis. The complete reading of these articles showed that they were outside the selection criteria, or the full text was not accessible online. One hundred fifty-eight papers formed the basis of this analysis, and the extracted information included in our review is listed in Appendix 1. The search process is shown in Figure 1. From each paper, the following details were gathered: (1) name of the journal, (2) year of publication, (3) name of the focal taxa, (4) taxonomic details of the taxa, (5) region, (6) country, (7) scope or central objective of the study (e.g., keywords) (8) type of data used (species abundance, presence, or presence-absence), (9) statistical method, (10) the method of collection of occurrence coordinates (e.g., primary or other sources), (11) validation technique, (12) environmental layers used, and (13) name of the packages and software used. Based on that, the papers were grouped into three main categories. They were—(1) speciation or ancestral distribution estimation, (2) estimation of climate change effect and anthropogenic alterations on native species, and (3) determining the chance of invasive alien species, disease, or pest spread (Table 1). As the research objective of this study was to review the articles with an intention for the conservation-related recommendations and their applications in the Indian subcontinent, only the last two topics were elaborately discussed through case studies from the countries. For that, before analysis, two papers contributing to understanding species' evolutionary aspects were eliminated from counting.
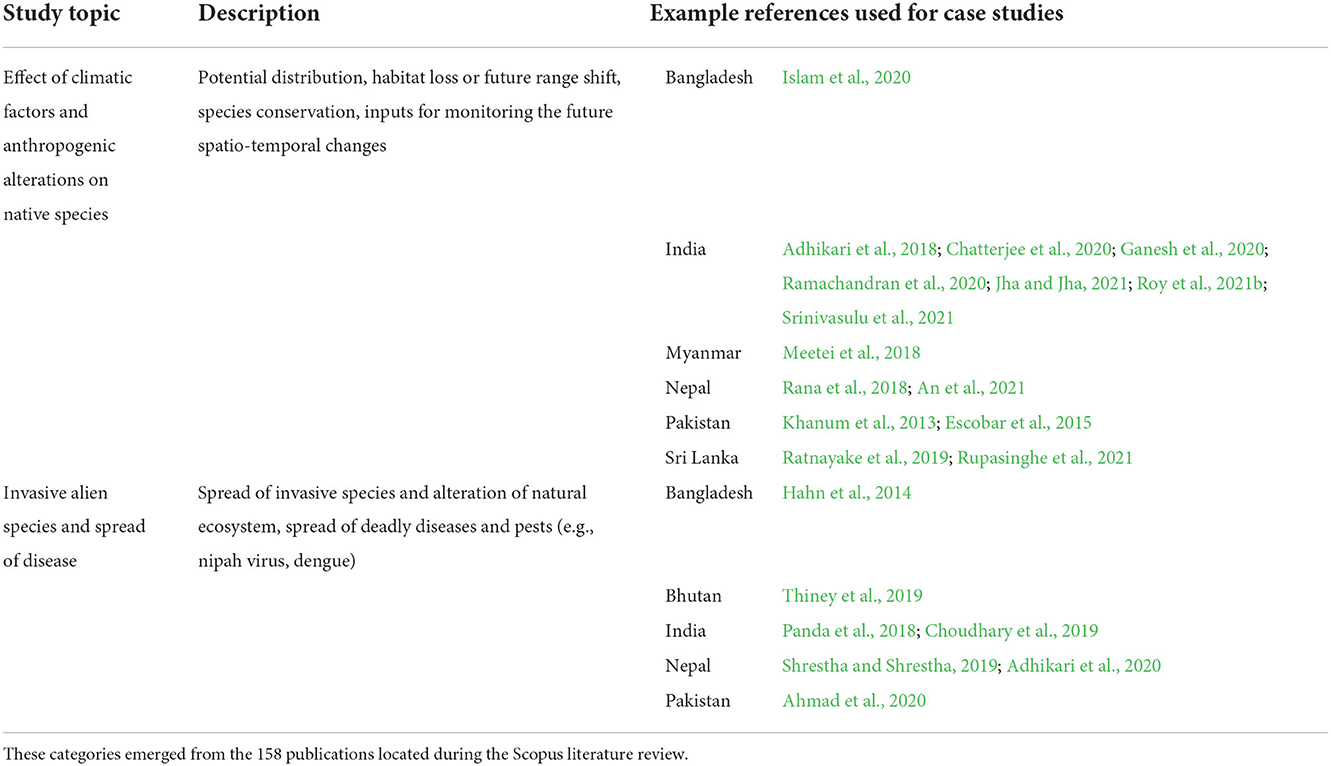
Table 1. Classification of study topics, including sample references from each country (if available).
Generalized linear model, generalized boosted models, classification tree analysis, artificial neural networks, surface response envelope, flexible discriminant analysis, multivariate adaptive regression splines, random forest, and maximum entropy—were used as statistical algorithms in most of the articles. Occurrence data points were collected by field survey (e.g., observation, quadrat sampling, camera trapping etc.), followed by online data sources (e.g., iNaturalist, India biodiversity portal, Fish Base, literature, and herbarium survey etc.). In most cases, true skill statistic, Cohen's kappa, and area under the curve were used as the validation techniques. R (packages: Biomod2, Dismo, ENMeval, HMSC, mgcv, virtualspecies, ENMEval, spThin, usdm, BiodiversityR, AdehabitatHS etc.), DIVA-GIS, QGIS, and ArcGIS software were used for analysis.
Data analysis
Among 156 research papers, some described the species distribution patterns in the entire world, including the Indian subcontinent (n = 11) (Ranjitkar et al., 2014; Ashraf et al., 2018). Apart from that, most of the research works were from India, followed by Nepal, Sri Lanka, Pakistan, Bangladesh, Bhutan, and Myanmar (Figure 2). The majority of the works were focused on predicting the potential distribution of species, habitat loss in future climatic scenarios, estimating future range shifts, and providing valuable suggestions for monitoring species conservation (n = 119, 82%). The research papers predicting the invasion of alien species in the native ecosystem and disease spread together were 26 in no (18%) (Figure 3).
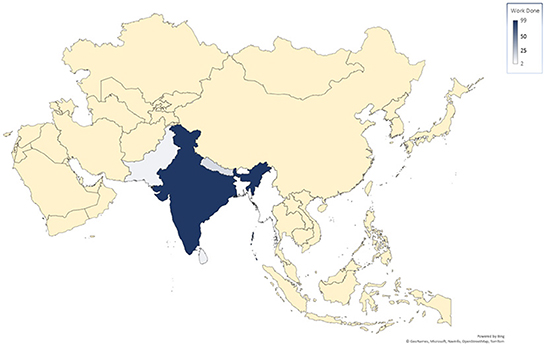
Figure 2. Indian subcontinental countries that contributed research articles on distribution modeling (2012–2021 in Scopus) are highlighted on the world map.
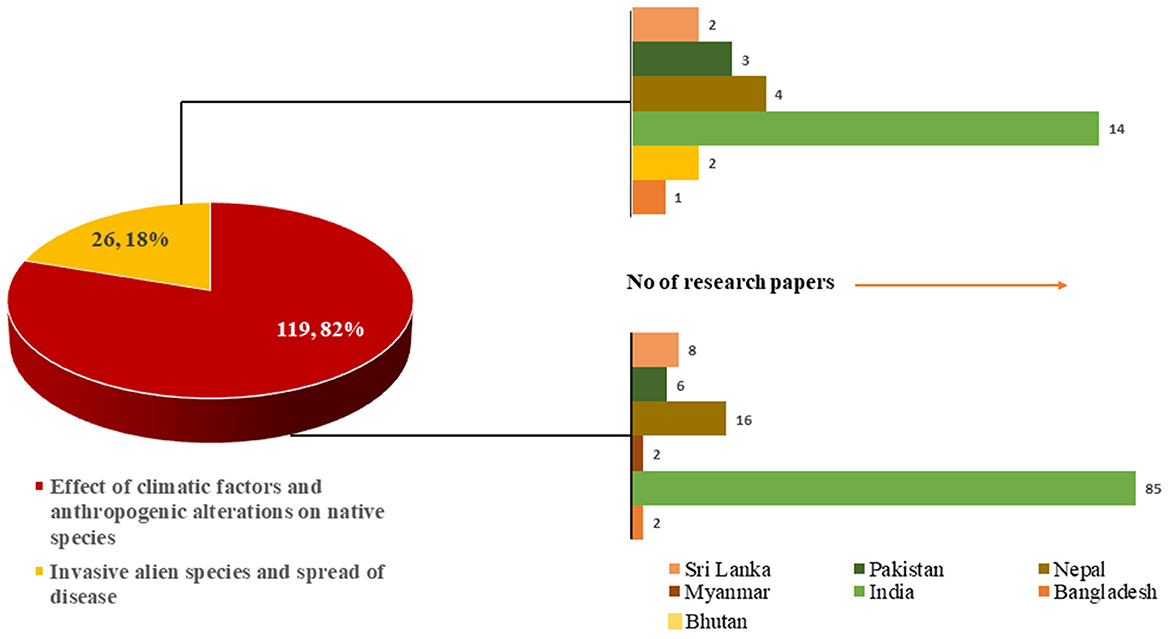
Figure 3. Diagram showing total no of research articles (country-specific) used for review and analysis. The highest no of research papers is published from India, followed by Nepal, Sri Lanka, Pakistan, Bangladesh, Bhutan, and Myanmar.
Studies on plant species distribution patterns
Among the 156 research papers, the works on plant species distribution were 84 in number, among which research on native plants species distribution was higher (e.g., sal, Himalayan alder, Himalayan rhubarb, Himalayan birch, chir pine, garjan, teak, olive, sandalwood, kamala tree, coffee, rubber, wild strawberry, potato, wheat, maize, wild rice, wild banana, kalmegh etc.; n = 67) than the invasive alien plants (e.g., sage, mesquite, common water hyacinth, kariba weed, billygoat weed, bitter vine etc.; n = 17). Investigations were also done for understanding fungi (e.g., Chinese caterpillar fungus; n = 2), lichen (n = 1), phytoplankton (n = 1), and macrofungi (n = 1) distribution pattern.
Studies on animal species distribution patterns
Research works describing the patterns of aniaml distribution was total 67 in number, among which research on mammal was highest in no (e.g., Bengal tigers, greater one-horned rhinoceros, Asiatic black bear, red panda, bat, sangai, Himalayan brown bear, Asiatic wildcat, jungle cat, Indian fox, golden-jackal, leopard, Malayan giant squirrel, brown mongoose, Himalayan Langur, desert hamster, Royle's pika, Asian elephants, Assamese macaque, Asiatic black bear, Kashmir musk deer, and Gaur; n = 28), followed by birds (e.g., frogmouth, Nilgiri flycatcher, tree-nesting colonial waterbirds, wooly-necked stork, vulture, cotton pygmy goose, red-crested pochard, Indian peafowl, Western tragopan, great Indian bustard, forest owlet etc.; n = 13), insects (e.g., screwworm, desert locust, Aedes mosquito, guava fruit fly etc.; n = 8), reptiles (e.g., Himalayan pit viper, mountain pit viper, king cobra, monocled cobra, short-tailed ground agama, Indian skink etc.; n = 6), amphibia (e.g., Sri Lankan torrent toads, rock toad, Kirtisinghe's rock frog, and Gannoruwa shrub frog; n = 4), fish (e.g., snow trout, mola, and copper mahseer; n = 3), mollusca (e.g., giant African land snail etc.; n = 3), nematoda (n = 1), and benthic invertebrates (n = 1).
Case studies on the effect of climatic factors and anthropogenic alterations on native species
Some important studies were described as case studies to discuss species conservation status, future needs for protection and sustainable management in their native countries. The case studies, if available, were selected from each country and divided into two sections—one explaining the native plant species distribution and the other one about native animal species distribution. As India has contributed a significant number of research articles (n = 85) on the study topics, examples were carefully selected from available documents based on the scientific importance of the focal taxa and modeling technique. For plant species distribution, one example was from Northeast India, the Himalayas, and Jammu and Kashmir region, and another was from the Eastern Ghats region. For animal species, one example from each vertebrate taxa (e.g., fish, amphibia, reptilia, bird, mammal) was selected.
Plant species distribution
Country—Bangladesh
(I) Species: Garjan, Dipterocarpus turbinatus.
Study predicting species range shift in changing climate.
Bangladesh is vulnerable to climate change for its geographic location (MOEF, 2008). Climate change causes changes in the tree phenology, forest cover and spatial distribution. Dipterocarpus turbinatus, locally known as Garjan, is one of the evergreen tree species which is globally valuable as well as vulnerable. It is native to the countries like Bangladesh, India, Myanmar, and Sri Lanka. Garjan forests are one of the vital shelters for threatened native wildlife species. Garjan requires a mean annual temperature range of 6–45°C and an annual rainfall range of about 2,000–4,000 mm for proper growth. Although valuable, the population seemed to have a declining trend due to land cover land-use change, forest fragmentation, illegal felling, improper management, and species invasion (Islam et al., 2001; Biswas and Misbahuzzaman, 2008; Ashton and Kettle, 2012). Islam et al. (2020) conducted SDM to predict species' future range shifts in Bangladesh. The prediction indicated that the habitat would shift toward the south-eastern corner of the country, which has a comparatively high altitude. The annual temperature range would be the most significant bioclimatic variable. D. turbinatus might become extinct in the north-eastern parts of the country due to increased stress in future.
Country—India
(II) Species: native tree, herb, and pteridophyte species in Northeast India, Western and Eastern Himalaya, and Jammu and Kashmir.
Study for improving threat assessment and categorization process.
The pre-requisite for conservation planning is prioritizing species conservation through threat assessment. Adhikari et al. (2018) assessed the conservation status of five trees, eight herbs, and one pteridophyte (e.g., Acer hookeri, Angelica glauca, Angiopteris evecta, Aquilegia nivalis, Bhesa robusta, Begonia satrapis, Corydalis cashmeriana, Gynocardia odorata, Ilex venulose, Lagerstroemia minuticarpa, Rheum austral etc.) species in Northeast India, Western and Eastern Himalaya, and Jammu and Kashmir. They utilized the potential distribution prediction maps of ENM as a tool to guide surveys responsible for the discovery of new populations of threatened species. It was further used to improve threat assessment and for categorization. The species status based on this study showed that all species fell under the critically endangered and endangered category. For example, B. robusta, earlier, was classified as least concerned, fell into the critically endangered conservation category.
(III) Species: rare, endangered, and threatened plant species (RET) and endemic species of conservation value in the Eastern Ghats.
Study predicting the future of range-restricted species.
Intense deforestation in the Eastern Ghats over decades has affected its rich and unique species diversity (Balaguru et al., 2006; Ramachandran et al., 2018). Its “broken-chain topography” further enhances the chance for this region to be overexploited and degraded. Although, very few studies are available for the region that analyse the climate and land use land cover (LULC) change aspects (Remya et al., 2015). Ramachandran et al. (2020) studied that aspect by using SDM. The suitable habitats for a group of endemics (28 in no) and RET (22 in no) species were stimulated for 2050 and 2070 in the Eastern Ghats region. From the model result, the bioclimatic variables like the mean temperature of wettest quarter, isothermality, precipitation of wettest, coldest and warmest quarter, and annual precipitation were found to have a significant percentage of influence on endemic species distribution. Similarly, RET species were mainly dependent on climatic factors like rainfall and LULC and geographic factors like slope. Most suitable habitats for the future occurrence of the endemic and RET species would be available in the core forest areas and high-elevation areas of less disturbance. However, the restricted distribution of these species' groups might increase their chances of future extinction.
Country—Nepal
(IV) Species: Himalayan alder, Alnus nepalensis and Alnus nitida.
Study for recommending conservation area for an alder tree-based agroforestry system development.
Himalayan alder species are ecologically important nitrogen-fixing trees helpful both in traditional or contemporary agroforestry practice and sloping land restoration (Sharma et al., 2007). Therefore, understanding their distribution and potential plantation zones could be helpful for the agroforestry sector. Rana et al. (2018) modeled habitat suitability for identifying the climatic zones of Alnus nepalensis and Alnus nitida. The model result indicated that the most favorable condition for A. nepalensis persistence was in the moist northwest-facing slope of central Nepal, whereas for A. nitida, it was in western Nepal. The major environmental factors contributing to Alnus distribution were precipitation of the warmest quarter (A. nepalensis) and precipitation of the driest quarter (A. nitida).
Further, future climatic forecast indicated significant changes in the potential distribution of both species by 2050; there would be expansions and reductions in suitability. A. nepalensis population would decline, whereas A. nitida population would secure suitability toward the northeast. In addition to climate, mountains and increasing elevation in northern aspect of the country create an obstacle for A. nepalensis seed dispersal and their establishment (Salick et al., 2009). In such cases, A. nitida could be an alternative. It would be advantageous in the future first to check the compatibility between cardamom or tea plantation and Alnus and then establish this nitrogen-fixing, rare shade tree for the agroforestry systems in a stable area. The potentially suitable area predicted by the model could finally be designated as the conservation area for recommending an alder tree-oriented agroforestry system.
Country—Pakistan
(V) Species: Asclepiads.
Study predicting the range shrinks of medicinally important Asclepiads distribution.
Khanum et al. (2013) modeled the distribution of three medicinally important Asclepiads, namely Tylophora hirsuta, Pentatropis spiralis, and Vincetoxicum arnottianum, in future climate change scenarios. The Indian subcontinent, especially India and Pakistan, is a secondary center for Asclepiad's diversity. Asclepiads are highly dependent on the local climate. Model prediction showed that the precipitation variables mainly affected the current distributions of P. spiralis and V. arnottianum, whereas temperature regulated T. hirsuta distribution. Overall modeling results suggested that the future distribution of medicinal plants might decrease under future climate change scenarios, resulting in local extinction. Habitat loss risk would be greatest for T. hirsuta, followed by P. spiralis and V. arnottianum. Range expansions in the case of V. arnottianum and T. hirsute could be seen toward the comparatively higher peak of the country, which might be accelerated through adaptations.
Country—Sri Lanka
(VI) Species: Neglected and Underutilized Fruit Species (NUFS).
Study for understanding the future challenges of global food security.
More than 60 varieties of NUFS grow in Sri Lanka (Haq, 2002). NUFS are a vital source of local food which contributes to nutrition security, traditional medicine, and the country's economy. Ratnayake et al. (2019) modeled the potential areas for four popular NUFS, namely Aegle marmelos, Annona muricata, Tamarindus indica, and Limonia acidissima occurrence under future and current climatic scenarios. Understanding their spatial distribution is required to deal with the cultivation success and future global food security challenges (Delêtre et al., 2012). Results suggested that soil contributed most significantly to A. muricata and L. acidissima distributions, whereas the minimum temperature of the coldest month and precipitation of the driest month for T. indica and A. marmelos distribution, respectively. The suitable area laid mainly around Kandy, Kalutara, Kurunegala, Gampaha, and Kegalle districts. Overall, all four NUFS were expected to decrease by more than 40% in future and would confine to wet zone regions, thus suggesting the requirement of immediate management concerns.
Animal species distribution
Country—India
(I) Species: least concerned small indigenous freshwater fish Amblypharyngodon mola.
Study for identifying indicator variables regulating indigenous fish distribution.
Amblypharyngodon mola is one of the important members of small indigenous freshwater fish species (SIF) and belongs to the family Cyprinidae (Dubey et al., 2014; Ahamed et al., 2017). Although their current IUCN status is least concerned, they are facing today risk of extinction due to negligence. They often remain unnoticed under the species of high economic value. The potential suitability areas of A. mola were predicted in the current climatic situation by Roy et al. (2021b). Variations of annual upstream precipitation, mean upstream temperature of the driest quarter and soil pH in water across elevational gradients determine fish distribution patterns. Clear zonation was found to be present between highly suitable and other categories. Assam, Bangladesh, Kerala, Tripura, West Bengal, and eastern coastal parts of Orissa were predicted to have optimal conditions. Despite high freshwater fish species richness, the Ganges delta and plain ecoregion are most vulnerable in the World. The delta is facing extensive threats due to the high converted land percentage, sea-level rise, human footprint, large cities and megacities, lesser urban land cover, surface water consumption, irrigation together with issues of climatic disasters (Abell et al., 2008).
(II) Species: endemic rock toad Duttaphrynus hololius.
Study for covering lacunae of data deficient species distribution.
The rock toad (Günther, 1876)1 is a data-deficient and uncommon species endemic to the Deccan plateau. The new modeling and analyses by Ganesh et al. (2020) produced a better and more clarified picture of its distribution. The map showed higher probabilities of occurrence (about 77–92%), notably in the Mysore plateau, which is further surrounded by low probability zones (about 23–31%) of south Cauvery and north Penner Rivers. Despite being hilly, the Western Ghats region was projected to be a totally unsuitable zone. Altogether, the new information on the occurrence and range projections covered some lacunae on the only probable amphibian endemic to the Indian peninsula's dry zone.
(III) Species: endemic threatened squamates.
Study for investigating the future distribution and recommending conservation area network.
Squamates are one of the most environmentally sensitive species. Srinivasulu et al. (2021) have modeled 22 threatened and endemic squamates distribution in the Western Ghat hotspot. For the study, the nine terrestrial ecoregions of the Western Ghats were considered—the Deccan thorn scrub forests, Malabar coast moist forests, Khathiar-Gir dry deciduous forests, Narmada Valley dry deciduous forests, North Western Ghats montane rainforests, North Western Ghats moist deciduous forests, South Western Ghats moist deciduous forests, South Western Ghats montane rainforests, and South Deccan Plateau dry deciduous forests (Olson et al., 2001).
A decrease in climatically suitable areas in future for the majority of the studied species was observed. The greatest declines were predicted for Eurylepis poonaensis (97.9%), followed by Cnemaspis indica (85.92%). There was no significant relationship between trends in suitable climatically areas and taxonomic groups. Temperature seasonality had the maximum contribution across all species. The South Western Ghats montane forest and the Malabar coast moist forest had optimal conditions for becoming diversity hotspots. In changing climatic scenarios, hotspots of species diversity were predicted to be condensed in the southern and north-central Western Ghats. The final recommendation of the analysis was to develop a conservation area network which has 97 clusters (for 10% target species representation) with an area of ~21,442 sq km.
(IV) Species: extinction-prone vulture population.
Study on the future distribution of the resident and migratory population.
Vultures are obligate scavengers and one of the most extinction-prone bird groups in the world (Sekercioglu et al., 2004; Straub et al., 2015; Phipps et al., 2017; Anoop et al., 2020). In India, there are nine old world vultures; most belong to the critically endangered and endangered category. The Gangetic-Thar-Deccan region (GTDR, administrative units are Uttar Pradesh, Rajasthan, and Madhya Pradesh) in India supports the bulk of the migratory and resident populations (Jha, 2018).
Jha and Jha (2021) have done species distribution modeling to estimate the current range of their habitat, predict potential future habitat, identify the vulnerable areas and finally find out a variation in global and local scale modeling results. First, the vulture occurrences were grouped according to the Forest Department's management strategy. Global and local species distribution models were generated to compare and analyse the difference in habitat suitability. The local models were developed separately for each individual region. Bioclimatic (temperature and precipitation variables) and bioenvironmental (climatic, LULC, human Footprint, normalized differential vegetation index, aspect, and elevation variables) models were generated globally as well as locally. The significant bioclimatic environmental variables were the mean diurnal range of temperature (6.55–18.50°C), temperature seasonality (361.67–818.56°C), isothermality (36.16–54.29°C), annual precipitation (104–2,124 mm), precipitation of the driest month (0–12 mm) and the precipitation seasonality (103.61–175.05 mm).
For the future, the top climatic factors that contributed significantly to the distribution of all vultures were isothermality, temperature seasonality, annual precipitation, precipitation seasonality, precipitation of driest month, and warmest quarter. For the resident vultures, these were isothermality, precipitation and temperature seasonality, annual precipitation, precipitation of driest month, and warmest quarter. Lastly, for the migrants, these variables were annual precipitation, isothermality, precipitation of the warmest quarter, mean diurnal range temperature, and precipitation seasonality. The unsuitable area of all vultures would increase in future for a moderate emission scenario.
(V) Species: near-threatened Malayan giant squirrel, Ratufa bicolor.
Study predicting the future habitat loss.
Malayan giant squirrel Ratufa bicolor is an arboreal, diurnal, herbivorous, and near-threatened rodent species found mainly in the semi-evergreen and evergreen forest areas of north-eastern India. They are known as the indicator species for forest health and habitat quality (Koprowski and Nandini, 2008; Bahuguna and Singh, 2013). Along with climatic and anthropogenic factors, the long generation length (between 8 and 9 years) has put the species in more vulnerable conditions. Chatterjee et al. (2020) have studied the factors governing their distribution within India and modeled the suitable existing areas for occurrence and vulnerability.
Precipitation of wettest month was found to have the highest power for predicting the distribution at the current time, along with elevation, LULC, and vegetation pattern. Among different LULC and vegetation patterns, most of the species' occurrences were found in the tropical semi-evergreen, tropical moist deciduous, and subtropical evergreen forests. More than 33% of occurrences were between 1,001 to 1,500 m elevation. In future, precipitation of wettest month would contribute to maximum species distribution, followed by the mean temperature of warmest quarter and the precipitation seasonality. With changing climate by 2050, it was predicted that only 2.94% area of its distribution range would remain stable, and the other 97.1% would become unfavorable. By this way, species' suitable habitat would vanish by more than 90%.
Country—Myanmar
(VI) Species: Ptyas doriae.
Study on species potential distribution.
Meetei et al. (2018) analyzed Ptyas doriae distribution to determine the Grinnellian niche. The result predicted a larger section in Southeast Asia, including parts of north-eastern India, China, northern Myanmar, Laos, Thailand, and Vietnam, had an optimal suitable climate for this species persistence. The variable contributing the highest influence in determining their distribution was the precipitation of the warmest quarter. The summer months might be essential for the maintenance of forest covers, which could act as an important determinant of distribution.
Country—Nepal
(VII) Species: Bengal tiger, Panthera tigris.
Study on sex-specific distribution models for more informative trail maps.
Tigers are one of the iconic species among the 31 largest and globally important mammalian carnivores. They are sparsely distributed across the Asia (Ripple et al., 2016). Despite that, fewer than 4,000 animals are living in the wild, and inhabiting <7% of their past geographic range (WWF, 2016). Range restrictions of the species are primarily because of human activities and development, prey depletion, hunting, and illegal killing. An et al. (2021) generated sex-specific distribution models for Bengal tigers using two analytical frameworks for more informative and detailed maps based on camera trapping data within Chitwan National Park in Nepal and its surrounding zones.
Results of MaxEnt analysis suggested that about 8% area fell under the least suitable category and about 85% for moderately suitable habitats. The suitable and highly suitable classes accounted for about 7%. Lowland floodplains had the highest suitable area, while most of the regions of Churia hills had moderately suitable areas. For female tigers, prey abundance and sal forest cover percentage were most influential, contributing 35 and 31%, respectively. Female tigers had a more restricted range of suitable habitats. Results also indicated differences between male and female tigers with respect to the proximity to human settlements. Male tigers were found less averse to human settlements suggesting probable concerns for tiger conservation at the social and ecological intersection.
Country—Pakistan
(VIII) Species: Asian black bear, Ursus thibetanus.
Study on the species distribution with night time satellite imagery.
The Asian black bear is a red-listed species of broad geographic range, preferring highland areas of low temperature and high precipitation, which has been fragmented today drastically by land use change (Servheen, 1990; Garshelis and Steinmetz, 2008). Despite several protection plans at the international and national level, their population are declining rapidly, mainly due to competition for resources and human-bear conflicts. According to the IUCN (2014) map, U. thibetanus occurs in restricted areas of Pakistan, northern parts of Nepal, and India (e.g., Himachal Pradesh, Uttarakhand, Assam, Manipur, and Mizoram). Escobar et al. (2015) integrated ENM with night time satellite imagery for better and more reliable prediction. The night time light reflectance values were determined. The highest value attached to the bear occurrence tracks was the criterion to indicate the threshold, above which the species avoid artificial light. Based on overall results, the species was considered as a habitat generalist. Thus, threats to the conservation of U. thibetanus should be managed at fine geographic scales, e.g., in areas of low human disturbance and ideal environmental conditions.
Country—Sri Lanka
(IX) Species: endemic and endangered frog, Pseudophilautus zorro.
Study on the range-restricted distribution of the species.
The shrub frog Pseudophilautus zorro is an endemic, endangered, and range-restricted species which is found in Sri Lanka's central highlands. There is vast knowledge gap about the basic ecology of the species, which is critical for proposing conservation strategies. Rupasinghe et al. (2021) assessed the niche of the species to identify potential areas of occupancy and, after that, calculated the area of occupancy and the extent of occurrence. Kandy, Kegalle, and Matale districts in the Sabaragamuwa and Central zones had highly suitable regions. Mean diurnal range had the maximum contribution, followed by precipitation of the driest month and temperature seasonality. The reproduction and survival of P. zorro depend on leaf litter moisture. High environmental temperature thus increases evaporation from litter (Kreye et al., 2018), reducing species survival.
Case studies on invasive alien species and the spread of disease
Plant species distribution
Country—Bhutan
(I) Species: Ageratum conyzoides, Aegratina adenophora, Chromolaena odorata, Mikania micrantha, Lantana camara, and Parthenium hysterophorus.
Study on alien invasive weeds distribution.
Thiney et al. (2019) studied the distributions of six globally noxious alien species in Bhutan. The study proved that even the mountain environment, despite its barriers of sharp slopes, limited niches, and harsh climate, was threatened by invasion in the changing environmental conditions. The study tested the hypothesis that the invasive species were highly resilient to various environmental conditions, e.g., a topographically variable landscape or highly heterogeneous ecoregions. Results suggested that there would be a significant increase in invasion regions during future climate scenarios, except for P. hysterophorus. It would experience a northerly shift and decrease in extent. Among the six studied species, C. odorata might become widespread over time. Despite that, most species would have restricted expansion to the country's southern region.
Country—India
(II) Species: Cassia tora and Lantana camara.
Study on the distribution of invasive annual and perennial species.
The species-specific invasive potentials of Cassia tora (Fabaceae) and Lantana camara (Verbenaceae) in climate-changing scenarios were studied by Panda et al. (2018). These two species have different patterns of the life cycle (annual and perennial, respectively), but the similar origin and life forms. C. tora is native to eastwards Polynesia (Singh, 1968), and L. camara is native to South and Central America (Day et al., 2003). They are familiar weeds in the jhum fallows and dry forests in India (Sharma et al., 2005). These alien species together increase native ecosystem vulnerability to climatic stressors (Vitousek, 1986; Burgiel and Muir, 2010).
Species with different phenological cycles (e.g., perennial or annual) use resources differently. Perennials need resources throughout the year, whereas annuals are during each growing season (Leffler and Ryel, 2012). The modeling result indicated that distributions of both species were significantly related to solar radiation of the warmest and wettest quarter. Radiation of the wettest quarter and the mean diurnal temperature range were highly correlated to L. camara distribution, compared with C. tora. Irradiative surfaces, open spaces, deforestation, fire, and agriculture could further help to establish invasive species (O'Donnell and Ignizio, 2012).
In future, precipitation seasonality would significantly contribute to C. tora distribution. Precipitation variations had negative effect on L. camara but positive impact on C. tora. There are chances that Central India might be most suitable for C. tora occurrence in changing climate scenarios. On the contrary, L. camara was found to have comparatively more colonizing capacity due to its higher seed dormancy and yielding capability. For that, the potential of L. camara invasion of the Western Himalayas and the Western Ghats would be higher.
Country—Nepal
(III) Species: 24 invasive alien plants.
Study for highlighting geographic hotspots of invasion.
Shrestha and Shrestha (2019) conducted ensemble species distribution models to predict the potential regions suitable for the 24 selected invasive alien species (IAPs) in future (2050 for RCP 6) and current climate change scenarios. The geographic hotspots of invasion were highlighted after considering climatic zones, physiography, land cover, and federal states. A much higher degree of overlapping area would be between invasion hotspots and agricultural lands/forests. The maximum invasion hotspots were predicted in the Terai-Duar savanna and grasslands' tropical zone (situated below 1,000 m). In the current climatic situation, no potential invasion hotspots were predicted in the subalpine region (3,000–4,000 m). But the hotspots could expand toward higher elevations areas of temperate and subalpine zones in the future. The analysis result thus highlighted the need to integrate biological invasions into subtropical climatic country Nepal's climate change policies, especially in the case of the Himalayas.
Animal species distribution
Country—Bangladesh
(I) Species: threatened bat Pteropus giganteus.
Study on the relationship between Pteropus giganteus roosts and human Nipah virus spread.
Hahn et al. (2014) analyzed threatened bat Pteropus giganteus roosts characteristics and developed an ecological niche model for identifying suitable habitat in Bangladesh and their association with human Nipah virus cases. Bat habitat range is primarily disturbed due to habitat loss, hunting and deforestation in Bangladesh. Additionally, the misconception about P. giganteus that it is a pest and animal reservoir of the deadly Nipah virus creates a clash between its conservation initiative and public health. Results indicated that human population and road density were significant predictors in the ENM. It was identified by modeling that 2–17% of the land area was suitable for roosting. The species' habitat preference is dependent upon the characteristics of trees, percentage of forest fragmentation, level of human disturbance (e.g., human population density, distance to roads), annual precipitation and elevation. It was concluded that the primary agricultural practices facilitated the deleterious effects. To prevent the spread of zoonotic diseases, one must improve basic knowledge about the Pteropus species' life history and resource utilization patterns.
Country—India
(II) Species: guava fruit fly, Bactrocera correcta.
Study on the infestation by the polyphagous pest.
Bactrocera correcta is a polyphagous fruit fly of the Tephritidae family, predominant in tropical and subtropical areas. Choudhary et al. (2019) predicted the habitat suitability of the species in future climate change scenarios (2050 and 2070) in India. The significant variables for the species distribution were precipitation of driest month, temperature seasonality and annual precipitation. Under future and present climate change conditions, a highly suitable area would be in the coastal parts of Gujarat, Karnataka, Kerala, Tamilnadu, and Maharashtra (>0.6). In contrast, the majority of the area of central India (Madhya Pradesh, Odisha, Chhattisgarh, and Rajasthan) would be with low to mild infestation. Overall, the model projected a shift in species distribution and suitability by the end of this century.
Country—Nepal
(III) Species: giant African land snail (GALS), Achatina fulica.
Study on distribution patterns of the world's worst alien invasive species.
GALS is one of the world's worst alien invasive species (Lowe et al., 2000), posing threats to native biodiversity and humans by causing economic loss (Budha, 2015). Their increased colonization and associated risks are primarily accelerating due to anthropogenic disturbances, including the extension and expansion of roads (Pimentel et al., 2001). The transporting materials of the vehicles have caused the introduction of this alien species in new areas. Adhikari et al. (2020) modeled the distribution of GALS and identified that the distance to the nearest road (35%) had the greatest percentage contribution, followed by mean temperature of driest quarter (33%), and annual precipitation (10%). The probability of GALS' presence was higher near the road, within about 900 m of roads. The result indicated potential risks of GALS on agricultural production in the lowlands, which serve as the rice bowl of Nepal.
Country—Pakistan
(IV) Species: Aedes albopictus and Aedes aegypti.
Study on mosquito distribution and dengue prevalence by geostatistical model.
Aedes albopictus and Aedes aegpti are two known mosquito species and dengue vectors (Akiner et al., 2016). There are numerous efforts made by the government and healthcare bodies, but it is still challenging to control the epidemic (Kannan et al., 2019). Determining the effects of climate change on future distributions of the mosquitoes can provide a visual insight into their distribution which would be helpful in understanding and controlling the epidemic (Campbell et al., 2015). Ahmad et al. (2020) performed a geostatistical analysis of the dengue fever risk in Khyber Pakhtunkhwa and estimated the maps after incorporating associated uncertainty. Geostatistical models can quantify the prediction probabilities and uncertainties in rate evaluations without any higher computational load and reduce the noise of the dataset (Hampton et al., 2011). One of the methods used in the study was the binomial Kriging method. The Maxent analysis showed that human density and distance to roads were the most critical factors for dengue infections.
Discussion
The highlighted case studies described the urgent need for species conservation in each country. Asia's remarkable economic growth brought many benefits through income and employment but fuelled threats to its biodiversity and ecosystems through habitat degradation (Squires, 2013). Economic interests are preferred over environmental concerns in Asia. That is obvious from the rising social-cost bill or stated in the “Asia and the Pacific SDG Progress Report 2020” (Yorifuji et al., 2015; United Nations Economic Social Commission for Asia and the Pacific, 2020). The variables, e. g., non-renewable energy usage, per capita income, fertility rate, urbanization, and population density, are some crucial drivers that affected the ecological footprint in the eight Asian developing countries from 1990 to 2015 (Sharma et al., 2020). The re-evaluation of the existing policies on the environment and economy is necessary for Asian countries as they struggle to achieve sustainable development goals.
Need for re-evaluation of existing policies and barriers to implementation
For developing proper climate change mitigation and adaptation, the conversion of suitable land for agricultural purposes must be estimated at the country level (Zabel et al., 2014). The fast-growing research on the farming domain in Asian countries has mainly concentrated on expanding agricultural lands by converting natural areas (Laurance et al., 2014; Chaplin-Kramer et al., 2015). Expansion poses a substantial question of balance between food security and conservation of wildlife for governments (Horgan and Kudavidanage, 2020). Some Asian countries have introduced national agroforestry policies in recent years (India in 2014, followed by Nepal in 2019) (Chavan et al., 2015; Atreya et al., 2021). Agroforestry is generally associated together with the concepts of multifunctionality at the tree, field, landscape, and farm levels. Agroforests are an essential category of planted forests on crops or pastureland, which have the potential for providing a wide range of forest-related sustainable ecosystem services to human society (Noordwijk et al., 2003). The system can potentially enhance the resilience of smallholder farmers in the future (Lasco et al., 2014; Bettles et al., 2021).
Climate change can negatively impact agriculture and leads to circumstances of food insecurity at a global and regional level. For example, the agriculture sector of Pakistan accounts for about 19.5% of gross domestic product, where it employs about 42.3% of the labor force (Finance Division, 2018). Although, in the Global Food Security Index, the country ranks 77th (Khanum et al., 2013; World Bank, 2017; Ministry of National Food Security and Research, 2018). Agroforestry-based products have a significant potential for providing employment opportunities, improvements in soil, air and water quality, carbon storage, building materials, medicines as well as biodiversity benefits (Dhyani et al., 2005, 2007; Barrios et al., 2012; Duguma et al., 2019; Chapman et al., 2020; Bettles et al., 2021). It is an important topic now in global and national agendas considering sustainable development. It is internationally recognized as a climate-smart agricultural system by the Intergovernmental Panel on Climate Change (IPCC) and the United Nations Framework Convention on Climate Change (UNFCCC) (Park et al., 2022).
Although agroforests are less diverse than native forests, they contain numerous animal and plant species (Murdiyarso et al., 2002). They provide ecological resilience, relieve pressure on harvesting native forests and maintain beneficial ecological functions (Angelsen and Kaimowitz, 2001; Noordwijk et al., 2003). Rahman et al. (2021) assessed the effects of tree cover and its diversity on carbon storage in Bangladesh. Analysis indicated that tree diversity increased total carbon storage in home gardens representing a potential nature-based solution. Generally, the preferred species are namely Acacia, Ailanthus, Albizia, Bamboo, Casuarina, Eucalyptus, Gmelina, Kadam, Melia, Populus, Shisham, and Teak (Singh and Dhyani, 2014).
Agroforestry growth and development are influenced directly or indirectly by economic policies, e.g., credit, power, taxation, trade, transport etc. Despite all the benefits, there are constraints in implementing agroforestry system climate goals. There is a need for better coordination between the Center-State agriculture departments, agriculture and forest departments, research organizations, financial institutions, panchayats, NGOs, the corporate sectors, and farmers. A coordinating institute for support at the inter-ministerial level can help to undertake policy-related planning and execution decisions. The system is also limited due to the lack of technical and functional knowledge, lack of participation of people, absence of separate policy, and market development about the process. For instance, in India, after agriculture, the second largest land use sector is represented by forestry, although it contributes only about 1% of GDP, reflecting the inert potential of the industry (Singh and Dhyani, 2014; Park et al., 2022).
The re-evaluation of existing policies for diminishing the risks of invasive species is also crucial in this regard. The “Integrated Forest Management (IFM)” (2009) scheme, initiated by the Ministry of Environment, Forest, and Climate Change (MoEFCC) in India for eradicating and controlling the pest species in forested areas, failed primarily because of a lack of proper sustainable management (Kannan et al., 2013). Identification and implementation of the invasion pathways and the biosecurity measures are essential for policy formulation (Nuñez et al., 2020). Although stern trading policies frequently face strong objections due to their economic benefits. Additionally, the increasing tendency of invasive species denialism, absences from the quarantine lists and the unresolved conflict of interest among stakeholders become significant roadblocks in the decision-making process (Russell and Blackburn, 2017; Banerjee et al., 2021). The small farmers face high risk for species invasion and effects of climate change on horticulture and agriculture. So, there is an urgent need to study and estimate the invasion cost.
Climatic change, economic development, population growth, and related land use changes all together impact directly on another resource, the freshwater ecosystem (Immerzeel and Bierkens, 2012), which raises concerns for freshwater biodiversity. Worldwide lack of monitoring stations and water quality data, poor integration of existing remote sensing data into inland water sciences led toward decreased allocation of resources, weak management, and lack of coordination for conservation (Dube et al., 2015; Ross et al., 2019). Water security is hindered due to the lack of an integrated management plan among countries sharing river basins. There is also a need for range-wide environmental data of freshwater realms to quantify the correlation between occurrence and abiotic environment. Freshwater-specific datasets are often restricted to individual watersheds or a country. Additionally, the terrestrial environmental variables cannot well explain the directional flows of freshwater (Domisch et al., 2015). The spatial detail is thus crucial for determining the variability of physical and socio-economic phenomena at a finer scale and planning the hot spot areas for developments (Giupponi and Gain, 2016).
Species distribution models as a cost-effective and sound tool for biodiversity conservation and sustainable management
SDM can be utilized as a sound and cost-efficient planning tool to critically assess biodiversity for sustainable development (Edenius and Mikusiński, 2006). SDM quantify species' functional niche at different spatial scales. Analysis and results can act as the spatial templates used to address population viability, the distribution range or habitat contraction of a species, and natural habitats for (re)introduction in climate change scenarios. The recent increasing trends in species distribution modeling works in ecology have supported this statement (Figure 4). Moderate to high suitability stable areas should be prioritized for conservation (Vaidyanathan, 2018). Prediction results can also be used as an easy warning system that identifies potential invasion areas, hence giving the early opportunity to prioritize the vulnerable region (Sarma et al., 2015). For instance, the dense forest canopy cover can be maintained, and continued plantation can be done to check the invasion of shadow-phobic species. Continuous uprooting of some weeds involving local people may help control their colonization (Panda et al., 2018). “A weed risk assessment protocol” could be included in the national policy framework to identify species' invasiveness and adaptability to climate change. Formulating legal provisions can engage landowners in ecosystem management actions. Shifting focus from alien to exclusively native species should be encouraged by promoting the businesses (Banerjee et al., 2021).
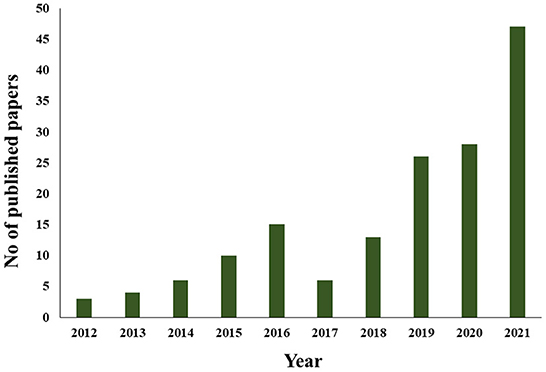
Figure 4. Increasing trend of research works in the field of species distribution modeling in the Indian subcontinent throughout the last decade (2012–2021).
The niche models are species-specific and dynamic. So, there is need for updating the occurrence dataset after every field visit (Adhikari et al., 2018). This intensive field validation has to be continuously followed to offer the scope to improve the model prediction. Long-term conservation of biodiversity requires community-based management programs. The decision-makers and non-government organizations worldwide are using today's citizens to volunteer and enhance modeling ability and investigate the depleting natural resources, identify endangered species, and conserve protected areas (Conrad and Hilchey, 2011). Formulating sustainable plans and policies for future management would be good if focused beyond 50 years after identifying global and regional trends of conservation approach (Vaidyanathan, 2018).
Endemic, range-restricted, non-charismatic, and neglected species can be conserved through a protected area network (Abraham and Kelkar, 2012). These potential new conservation areas should be designated aiming toward higher species representation targets as an alternative to a flagship or umbrella species-based approach (Ratnayake et al., 2019; Roy et al., 2021a; Srinivasulu et al., 2021). A protected area can be defined as—“An area of land and/or sea especially dedicated to the protection and maintenance of biological diversity, and of natural and associated cultural resources, and managed through legal or other effective means” (IUCN, 1994)2. SDM prediction can provide knowledge about the long-term persistence of a protected species in future climatic scenarios (Roy et al., 2021b).
Combining climate with remote sensing data can further improve the predictive ability of the historical ranges of a species (e. g., models based on environmental plus night time light data). Night time light imagery is an excellent alternative for species' habitat range estimation, corridor and protected area designing, threatened species prioritization, policy implementation, and human-wildlife conflict area determination (Escobar et al., 2015). Further, the time window and the selection of the locations of allowed disruptive activities (i.e., film shooting or tourism) in protected areas need more scientific considerations to minimize the negative impacts of conflict. Like, identifying the difference between male and female carnivores' breeding habitat choices through SDM can provide essential insights into their preference (An et al., 2021).
Some shortcomings of the models and recommendations for improvement
SDMs predict species' geographic ranges with the assumption of the niche conservatism theory that niche changes very slowly across space and time to sustain their populations (Soberón and Nakamura, 2009; Wiens et al., 2010). Several transferred SDMs were generated by transferring the data obtained from one region to other areas or to another time (Jeschke and Strayer, 2008; Werkowska et al., 2017). The results of the studies are widely helpful in forecasting the possibility of distributions under past environmental conditions, disease breakout, and species responses to climate change (Nogués-Bravo, 2009; Morán-Ordóñez et al., 2017; Yates et al., 2018). Although several recent studies reported that the range of species' niches changes rapidly when transported to a new geographic region (Broennimann et al., 2007; Pearman et al., 2008; Guisan et al., 2014; Atwater and Barney, 2021). So, understanding how the changed species-environment relationship affects the spatiotemporal transferability of SDMs is crucial for the future application of conservation design and improvement (Pearman et al., 2008; Petitpierre et al., 2017; Atwater and Barney, 2021). It is essential to consider data quality as well as niche change to improve the transferability of SDMs in the case of invasive species to support better conservation management and policy decisions (Liu et al., 2022). There is a need for an additional extended assessment of habitat suitability, especially for the range-shifting species, through an integrated SDM database (iSDMdb) (Frans et al., 2022).
A fundamental limitation of SDM is that they do not consider species interactions like competition or predation. It assumes that a species' existence depends only on abiotic factors (Peres-Neto and Legendre, 2010; Dormann et al., 2012; Wisz et al., 2013). The joint species distribution model (JSDM), a community-level and novel modeling framework, recently emerged as a promising technique to integrate the possibility of species interactions additionally into metacommunity and macroecology studies (Leibold et al., 2021; Pichler and Hartig, 2021). Model predictions can be applied to large-scale and regional metacommunity analyses (Gilbert and Bennett, 2010; Mittelbach and Schemske, 2015; Leibold and Chase, 2017). For instance, Zhang et al. (2020) found that community-level data improved the rare species' distributions to a certain extent.
One of the key assumptions of SDM is that species are at climatic equilibrium in their current distribution range. However, in reality, biotic interactions can prevent that through dispersal limitation or human disturbance, hence underestimating climatic tolerance (Hutchinson, 1957; Richmond et al., 2010). So, the model predictions relying on the climatic tolerance derived from unevenly sampled and biased species distributions have limited utility. One of the probable solutions could be to develop SDMs based on species' physiological tolerance data or growth requirement estimates (e.g., USDA PLANTS database). Considering species occurrences outside the natural range is needed to closely predict species' fundamental niche and range shifts in response to climate change. However, exhaustive field and lab work is essential to measure the tolerance limits (Booth et al., 2015; Curtis and Bradley, 2016).
For the accuracy of predictions and to maximize the performance of SDMs, selecting the most appropriate predictor variable is crucial (Araújo and Guisan, 2006; Braunisch et al., 2013; Barbet-Massin and Jetz, 2014). As expert knowledge is not much available, the choice of predictors remains a crucial question when executing SDMs. Another associated challenge of SDM is to unify multi-scale variables that may contribute to species distribution at different spatial resolutions. Species–environment relationships are strongly scale-dependent, and thus it reduces model accuracy and appropriateness for producing environmental suitability maps (Virkkala et al., 2005). Fournier et al. (2017) showed that after integrating multiple drivers, the modeling result had a smaller potential range of species than predicted using the climatic model alone, suggesting an overestimation.
Conclusion
Most of the works in the Indian subcontinent were focused on predicting native species' potential distribution, habitat loss, or future range shifts in changing climate (n = 119, 82%) rather than the invasion of alien species in the native ecosystem (n = 26, 18%) indicating the further scope of research in these areas. The scientific articles highlighted that modeling results could be utilized efficiently to design conservation areas like the native tree-based agroforestry system or protected area network for range-restricted, endemic, endangered, or threatened species to cope with the challenges of climate change scenarios. But analysis should not be biased toward charismatic species because that can affect the conservation-related decision-making process. Encouragement should be given to study the data-deficient species from molluscs, fish, amphibia, insects, lichen, fungi, or plankton. Modeling such species can help understand the future challenges of global food security and eradicating zoonotic disease spread. The population status of a species in the wild should be examined at regular intervals against the conservation criteria to understand how much native land is needed to control biodiversity loss and ecosystem functionality. Most importantly, the re-evaluation of existing policies and identification of geographic hotspots of invasion in subcontinental countries will be crucial to eradicate alien species infestation. A coordinating institute at the inter-ministerial level can help to undertake policy-related planning for sustainable management and execution of decisions.
Data availability statement
The original contributions presented in the study are included in the article/Supplementary material, further inquiries can be directed to the corresponding author.
Author contributions
SRo: conceptualization, data analysis, and writing—reviewing original draft. AS: data analysis and reviewing original draft. SRa: conceptualization, supervision, and resources. SS: supervision and resources. All authors contributed to the article and approved the submitted version.
Funding
The authors acknowledge University Grants Commission, New Delhi, for financial support through the UGC-BSR fellowship [Ref. F. No. 25-1/2014-15(BSR)/5-132/2007(BSR)].
Acknowledgments
The authors acknowledge the Scopus search facility provided to AS by the University of Delhi.
Conflict of interest
The authors declare that the research was conducted in the absence of any commercial or financial relationships that could be construed as a potential conflict of interest.
Publisher's note
All claims expressed in this article are solely those of the authors and do not necessarily represent those of their affiliated organizations, or those of the publisher, the editors and the reviewers. Any product that may be evaluated in this article, or claim that may be made by its manufacturer, is not guaranteed or endorsed by the publisher.
Supplementary material
The Supplementary Material for this article can be found online at: https://www.frontiersin.org/articles/10.3389/fsrma.2022.1031646/full#supplementary-material
Footnotes
References
Abell, R., Thieme, M. L., Revenga, C., Bryer, M., Kottelat, M., Bogutskaya, N., et al. (2008). Freshwater ecoregions of the world: a new map of biogeographic units for freshwater biodiversity conservation. BioScience 58, 403–414. doi: 10.1641/B580507
Abraham, R. K., Kelkar, N. (2012). Do terrestrial protected areas conserve freshwater fish diversity? Results from the Western Ghats of India. Oryx 46, 544–553. doi: 10.1017/S0030605311000937
Adhikari, D., Reshi, Z., Datta, B. K., Samant, S. S., Chettri, A., Upadhaya, K., et al. (2018). Inventory and characterization of new populations through ecological niche modelling improve threat assessment. Curr. Sci. 114, 519. doi: 10.18520/cs/v114/i03/519-531
Adhikari, S., Sharma, H. P., Rimal, B., Belant, J. L., Katuwal, H. B. (2020). Road as a major driver for potential distribution of the invasive giant African land snail in Nepal. Trop. Ecol. 61, 583–588. doi: 10.1007/s42965-020-00115-4
Ahamed, F., Rasel, M., Saha, N., Ara, M. G., Ahmed, Z. F., Hossain, M., et al. (2017). Biological aspects of the mola carplet Amblypharyngodon mola (Cyprinidae) in the Payra river, southern Bangladesh. Int. J. Fish. Aquat. Stud. 5, 336–339.
Ahmad, H., Ali, A., Fatima, S. H., Zaidi, F., Khisroon, M., Rasheed, S. B., et al. (2020). Spatial modeling of dengue prevalence and kriging prediction of dengue outbreak in Khyber Pakhtunkhwa (Pakistan) using presence only data. Stoch. Environ. Res. Risk Assess. 34, 1023–1036. doi: 10.1007/s00477-020-01818-9
Akiner, M. M., Demirci, B., Babuadze, G., Robert, V., Schaffner, F. (2016). Spread of the invasive mosquitoes Aedes aegypti and Aedes albopictus in the black sea region increases risk of chikungunya, dengue, and zika outbreaks in Europe. PLoS Negl. Trop. Dis. 10, e0004664. doi: 10.1371/journal.pntd.0004664
An, L., Bohnett, E., Battle, C., Dai, J., Lewison, R., Jankowski, P., et al. (2021). Sex-specific habitat suitability modeling for Panthera tigris in Chitwan national park, Nepal: broader conservation implications. Sustainability 13, 13885. doi: 10.3390/su132413885
Angelsen, A., Kaimowitz, D. (2001). Agricultural Technologies and Tropical Deforestation. Wallingford, CT: CABI. doi: 10.1079/9780851994512.0000
Anoop, N. R., Babu, S., Nagarajan, R., Sen, S. (2020). Identifying suitable reintroduction sites for the white-rumped vulture (Gyps bengalensis) in India's Western Ghats using niche models and habitat requirements. Ecol. Eng. 158, 106034. doi: 10.1016/j.ecoleng.2020.106034
Araújo, M. B., Guisan, A. (2006). Five (or so) challenges for species distribution modelling. J. Biogeogr. 33, 1677–1688. doi: 10.1111/j.1365-2699.2006.01584.x
Ashraf, U., Chaudhry, M. N., Ahmad, S. R., Ashraf, I., Arslan, M., Noor, H., et al. (2018). Impacts of climate change on Capparis spinosa L. based on ecological niche modeling. PeerJ 6, e5792. doi: 10.7717/peerj.5792
Ashton, P., Kettle, C. J. (2012). Dipterocarp biology as a window to the understanding of tropical forest structure: where are we looking now? Biotropica 44, 575–576. doi: 10.1111/j.1744-7429.2012.00913.x
Atreya, K., Subedi, B. P., Ghimire, P. L., Khanal, S. C., Charmakar, S., Adhikari, R. (2021). Agroforestry for mountain development: prospects, challenges and ways forward in Nepal. Arch. Agric. Environ. Sci. 6, 87–99. doi: 10.26832/24566632.2021.0601012
Atwater, Z. D., Barney, J. N. (2021). Climatic niche shifts in 815 introduced plant species affect their predicted distributions. Glob. Ecol. Biogeogr. 30, 1671–1684. doi: 10.1111/geb.13342
Bahuguna, A., Singh, A. (2013). A review on the molecular study of the species of family Sciuridae (Rodentia: Mammalia). Biol. Forum 5, 37–46.
Balaguru, B., John britto, S. J. S., Nagamurugan, N., Natarajan, D., Soosairaj, S. (2006). Identifying conservation priority zones for effective management of tropical forests in Eastern Ghats of India. Biodivers. Conserv. 15, 1529–1543. doi: 10.1007/s10531-004-6678-1
Banerjee, A. K., Khuroo, A. A., Dehnen-Schmutz, K., Pant, V., Patwardhan, C., Bhowmick, A. R., et al. (2021). An integrated policy framework and plan of action to prevent and control plant invasions in India. Environ. Sci. Policy 124, 64–72. doi: 10.1016/j.envsci.2021.06.003
Barbet-Massin, M., Jetz, W. (2014). A 40-year, continent-wide, multispecies assessment of relevant climate predictors for species distribution modelling. Divers. Distrib. 20, 1285–1295. doi: 10.1111/ddi.12229
Barrios, E., Sileshi, G. W., Shepherd, K. D., Sinclair, F. (2012). “Agroforestry and soil health: linking trees, soil biota, and ecosystem services,” in Soil Ecology and Ecosystem Services, ed D. H. Wall (Oxford: Oxford University Press), 315–330. doi: 10.1093/acprof:oso/9780199575923.003.0028
Bettles, J., Battisti, D. S., Cook-Patton, S. C., Kroeger, T., Spector, J. T., Wolff, N. H., et al. (2021). Agroforestry and non-state actors: a review. For. Policy Econ. 130, 102538. doi: 10.1016/j.forpol.2021.102538
Biswas, S. R., Misbahuzzaman, K. (2008). Tree species diversity and regeneration traits of the dominant species in a dipterocarp forest in Bangladesh: implications for conservation. Int. J. Biodivers. Sci. Manage. 4, 81–91. doi: 10.3843/Biodiv.4.2:2
Boesing, A. L., Nichols, E., Metzger, J. P. (2018). Biodiversity extinction thresholds are modulated by matrix type. Ecography 41, 1520–1533. doi: 10.1111/ecog.03365
Booth, T. H., Broadhurst, L. M., Pinkard, E., Prober, S. M., Dillon, S. K., Bush, D., et al. (2015). Forest ecology and management native forests and climate change: lessons from eucalypts. Ecol. Manage. 347, 18–29. doi: 10.1016/j.foreco.2015.03.002
Braunisch, V., Coppes, J., Arlettaz, R., Suchant, R., Schmid, H., Bollmann, K. (2013). Selecting from correlated climate variables: a major source of uncertainty for predicting species distributions under climate change. Ecography 36, 971–983. doi: 10.1111/j.1600-0587.2013.00138.x
Broennimann, O., Treier, U. A., Müller-Schärer, H., Thuiller, W., Peterson, A. T., Guisan, A. (2007). Evidence of climatic niche shift during biological invasion. Ecol. Lett. 10, 701–709. doi: 10.1111/j.1461-0248.2007.01060.x
Bryn, A., Bekkby, T., Rinde, E., Gundersen, H., Halvorsen, R. (2021). Reliability in distribution modeling—a synthesis and step-by-step guidelines for improved practice. Front. Ecol. Evol. 9, 658713. doi: 10.3389/fevo.2021.658713
Budha, P. B. (2015). Current state of knowledge on invasive and alien fauna of Nepal. J. Inst. Sci. Tech. 20, 68–81. doi: 10.3126/jist.v20i1.13913
Burgiel, S. W., Muir, A. A. (2010). Invasive Species, Climate Change and Ecosystem-Based Adaptation: Addressing Multiple Drivers of Global Change. Washington, DC: Global Invasive Species Programme.
Campbell, L. P., Luther, C., Moo-Llanes, D., Ramsey, J. M., Danis-Lozano, R., Peterson, A. T. (2015). Climate change influences on global distributions of dengue and chikungunya virus vectors. Phil. Trans. R. Soc. B 370, 20140135. doi: 10.1098/rstb.2014.0135
Chaplin-Kramer, R., Sharp, R. P., Mandle, L., Sim, S., Johnson, J., Butnar, I., et al. (2015). Spatial patterns of agricultural expansion determine impacts on biodiversity and carbon storage. Proc. Natl. Acad. Sci. U.S.A. 112, 7402–7407. doi: 10.1073/pnas.1406485112
Chapman, M., Walker, W. S., Cook-Patton, S. C., Ellis, P. W., Farina, M., Griscom, B. W., et al. (2020). Large climate mitigation potential from adding trees to agricultural lands. Glob. Change Biol. 26, 4357–4365. doi: 10.1111/gcb.15121
Chatterjee, P., Tripathy, B., Chandra, K., Saha, G. K., Mondal, K. (2020). Climate change alarms the survival of near threatened species Malayan giant squirrel (Ratufa bicolor Sparrman, 1778) in India. Mammal Study 45, 289–302. doi: 10.3106/ms2020-0011
Chavan, S. B., Keerthika, A., Dhyani, S. K., Handa, A. K., Newaj, R., Rajarajan, K. (2015). National agroforestry policy in India: a low hanging fruit. Curr. Sci. 108, 1826–1834.
Choudhary, J. S., Kumari, M., Mali, S. S., Dhakar, M. K., Das, B., Singh, A. K. (2019). Predicting impact of climate change on habitat suitability of guava fruit fly, Bactrocera correcta (Bezzi) using MaxEnt modeling in India. J. Agrometeorol. 21, 24–30. doi: 10.54386/jam.v21i1.199
Chowdhury, S., Alam, S., Labi, M. M., Khan, N., Rokonuzzaman, M., Biswas, D., et al. (2022). Protected areas in South Asia: status and prospects. Sci. Total Environ. 811, 152316. doi: 10.1016/j.scitotenv.2021.152316
Conrad, C. C., Hilchey, K. G. (2011). A review of citizen science and community-based environmental monitoring: issues and opportunities. Environ. Monit. Assess. 176, 273–291. doi: 10.1007/s10661-010-1582-5
Cowie, R. H., Bouchet, P., Fontaine, B. (2022). The sixth mass extinction: fact, fiction or speculation? Biol. Rev. 97, 640–663. doi: 10.1111/brv.12816
Curtis, C. A., Bradley, B. A. (2016). Plant distribution data show broader climatic limits than expert-based climatic tolerance estimates. PLoS ONE 11, e0166407. doi: 10.1371/journal.pone.0166407
Day, M. D., Wiley, C. J., Playford, J., Zalucki, M. P. (2003). Lantana: Current Managemet Status and Future Prospects. Canberra, ACT: Australian Centre for International Agricultural Research.
Delêtre, M., Gaisberger, H., Arnaud, E. (2012). Agrobiodiversity in Perspectives—A REVIEW of Questions, Tools, Concepts and Methodologies. Rome: Biodiversity International.
Dhyani, S. K., Samra, J. S., Ajit, H., Uma, A. K. (2007). Forestry to support increased agricultural production: focus on employment generation and rural development. Agric. Econ. Res. Rev. 26, 179–202. doi: 10.22004/ag.econ.47328
Dhyani, S. K., Sharda, V. N., Samra, J. S. (2005). Agroforestry for sustainable management of soil, water and environmental quality: looking back to think ahead. Range Manag. Agrofor. 26, 71–83.
Domisch, S., Amatulli, G., Jetz, W. (2015). Near-global freshwater-specific environmental variables for biodiversity analyses in 1 km resolution. Sci. Data 2, 150073. doi: 10.1038/sdata.2015.73
Dormann, C. F., Schymanski, S. J., Cabral, J., Chuine, I., Graham, C., Hartig, F., et al. (2012). Correlation and process in species distribution models: bridging a dichotomy. J. Biogeogr. 39, 2119–2131. doi: 10.1111/j.1365-2699.2011.02659.x
Dube, T., Mutanga, O., Seutloali, K., Adelabu, S., Shoko, C. (2015). Water quality monitoring in sub-Saharan African lakes: a review of remote sensing applications. Afr. J. Aquat. Sci. 40, 1–7. doi: 10.2989/16085914.2015.1014994
Dubey, S. K., Trivedi, R. K., Rout, S. K., Chand, B. K., Choudhury, A. (2014). Median lethal salinity (mls96 h) of two small indigenous fish species Amblypharyngodon mola and Pethia ticto from Indian Sundarban. J. Aquac. Res. Dev. 5, 249. doi: 10.4172/2155-9546.1000249
Duguma, L. A., Minang, P. A., Otsyina, R., Mpanda, M., Kimaro, A. A. (2019). “Shinyanga: blending old and new agroforestry to integrate development, climate change mitigation and adaptation in Tanzania,” in Sustainable Development through Trees on Farms: Agroforestry in its Fifth Decade, ed M. Van Noordwijk [Bogor: World Agroforestry (ICRAF)], 139–151.
Edenius, L., Mikusiński, G. (2006). Utility of habitat suitability models as biodiversity assessment tools in forest management. Scand. J. For. Res. 21, 62–72. doi: 10.1080/14004080500486989
Escobar, L. E., Awan, M. N., Qiao, H. (2015). Anthropogenic disturbance and habitat loss for the red-listed Asiatic black bear (Ursus thibetanus): using ecological niche modeling and nighttime light satellite imagery. Biol. Conserv. 191, 400–407. doi: 10.1016/j.biocon.2015.06.040
Feldman, M. J., Imbeau, L., Marchand, P., Mazerolle, M. J., Darveau, M., Nicole, J., et al. (2021). Trends and gaps in the use of citizen science derived data as input for species distribution models: a quantitative review. PLoS ONE 16, e0234587. doi: 10.1371/journal.pone.0234587
Finance Division (2018). Pakistan Economic Survey 2017–2018. Islamabad: Economic Adviser's Wing, Finance Division, Government of Pakistan. Available online at: www.finance.gov.pk/survey_1718.html
Fournier, A., Barbet-Massin, M., Rome, Q., Courchamp, F. (2017). Predicting species distribution combining multi-scale drivers. Glob. Ecol. Conserv. 12, 215–226. doi: 10.1016/j.gecco.2017.11.002
Frans, V. F., Augé, A. A., Fyfe, J., Zhang, Y., McNally, N., Edelhoff, H., et al. (2022). Integrated SDM database: enhancing the relevance and utility of species distribution models in conservation management. Methods Ecol. Evol. 13, 243–261. doi: 10.1111/2041-210X.13736
Ganesh, S. R., Brihadeesh, S., Narayana, H. S., Kumar, G. C. (2020). A contribution on morphology and distribution of the rock toad Duttaphrynus hololius (Günther, 1876) with first report on deformity, calling and breeding behaviours (Amphibia: Anura: Bufonidae). Asian J. Conserv. Biol. 9, 71–78.
Garshelis, D., Steinmetz, R. (2008). “Ursus thibetanus,” in IUCN SSC Bear Specialist Group, The IUCN Red List of Threatened Species (Version 2014.2). IUCN/SSN Bear Specialist Group. Available online at: http://www.iucnredlist.org/details/22824/0
Gilbert, B., Bennett, J. R. (2010). Partitioning variation in ecological communities: do the numbers add up? J. Appl. Ecol. 47, 1071–1082. doi: 10.1111/j.1365-2664.2010.01861.x
Giupponi, C., Gain, A. K. (2016). Integrated spatial assessment of the water, energy and food dimensions of the sustainable development goals. Reg. Environ. Change 17, 1881–1893. doi: 10.1007/s10113-016-0998-z
Guisan, A., Petitpierre, B., Broennimann, O., Daehler, C., Kueffer, C. (2014). Unifying niche shift studies: insights from biological invasions. Trends Ecol. Evol. 29, 260–269. doi: 10.1016/j.tree.2014.02.009
Guisan, A., Thuiller, W. (2005). Predicting species distribution: offering more than simple habitat models. Ecol. Lett. 8, 993–1009. doi: 10.1111/j.1461-0248.2005.00792.x
Guisan, A., Zimmermann, N. E. (2000). Predictive habitat distribution models in ecology. Ecol. Model. 135, 147–186. doi: 10.1016/S0304-3800(00)00354-9
Hahn, M. B., Epstein, J. H., Gurley, E. S., Islam, M. S., Luby, S. P., Daszak, P., et al. (2014). Roosting behaviour and habitat selection of Pteropus giganteus reveal potential links to nipah virus epidemiology. J. Appl. Ecol. 51, 376–387. doi: 10.1111/1365-2664.12212
Hampton, K. H., Serre, M. L., Gesink, D. C., Pilcher, C. D., Miller, W. C. (2011). Adjusting for sampling variability in sparse data: geostatistical approaches to disease mapping. Int. J. Health Geogr. 10, 54. doi: 10.1186/1476-072X-10-54
Haq, N. (2002). “Fruits for the future in Asia,” in Proceedings of a Regional Consultation Meeting on Utilization of Tropical Fruit Trees in Asia (Bangkok: Crops for the Future).
Horgan, F. G., Kudavidanage, E. P. (2020). Farming on the edge: farmer training to mitigate human-wildlife conflict at an agricultural frontier in south Sri Lanka. Crop Prot. 127, 104981. doi: 10.1016/j.cropro.2019.104981
Hughes, A. C. (2017). Understanding the drivers of Southeast Asian biodiversity loss. Ecosphere 8, e01624. doi: 10.1002/ecs2.1624
Hutchinson, G. E. (1957). Concluding remarks. Cold Spring Harb. Symp. Quant. Biol. 22, 415–427. doi: 10.1101/SQB.1957.022.01.039
Immerzeel, W. W., Bierkens, M. F. P. (2012). Asia's water balance. Nat. Geosci. 5, 841–842. doi: 10.1038/ngeo1643
Islam, K., Rahman, M. F., Islam, K. N., Nath, T. K., Jashimuddin, M. (2020). Modeling spatiotemporal distribution of Dipterocarpus turbinatus Gaertn. F in Bangladesh under climate change scenarios. J. Sustain. For. 39, 221–241. doi: 10.1080/10549811.2019.1632721
Islam, K. R., Ahmed, M. R., Bhuiyan, M. K., Badruddin, A. (2001). Deforestation effects on vegetative regeneration and soil quality in tropical semi-evergreen degraded and protected forests of Bangladesh. Land Degrad. Dev. 12, 45–56. doi: 10.1002/ldr.418
IUCN. (2014). Available online at: https://www.iucnredlist.org/ja/species/22824/166528664
Iverson, L. R., Prasad, A. M., Matthews, S. N., Peters, M. (2008). Estimating potential habitat for 134 Eastern US tree species under six climate scenarios. For. Ecol. Manag. 254, 390–406. doi: 10.1016/j.foreco.2007.07.023
Jablonski, D. (2008). Extinction and the spatial dynamics of biodiversity. Proc. Natl. Acad. Sci. U.S.A. 105, 11528–11535. doi: 10.1073/pnas.0801919105
Jeschke, J. M., Strayer, D. L. (2008). Usefulness of bioclimatic models for studying climate change and invasive species. Ann. N. Y. Acad. Sci. 1134, 1–24. doi: 10.1196/annals.1439.002
Jha, K. K. (2018). “Mapping and management of vultures in an Indian stronghold,” in Geomatics and Conservation Biology, ed M. O. Campbell (New York, NY: Nova Science Publishers), 45–75.
Jha, R., Jha, K. K. (2021). Habitat prediction modelling for vulture conservation in Gangetic-Thar-Deccan region of India. Environ. Monit. Assess. 193, 532. doi: 10.1007/s10661-021-09323-4
Kannan, R., Ramakrishnan, K., Ojo, A. O. (2019). “Social networking sites as communication tool for dengue related healthcare and wellness information,” in Proceedings of the 2nd International Conference on Software Engineering and Information Management (ACM), 204–207.
Kannan, R., Shackleton, C., Shaanker, R. U. (2013). Playing with the forest: invasive alien plants, policy and protected areas in India. Curr. Sci. 104, 1159–1165.
Khanum, R., Mumtaz, A. S., Kumar, S. (2013). Predicting impacts of climate change on medicinal asclepiads of Pakistan using maxent modeling. Acta Oecol. 49, 23–31. doi: 10.1016/j.actao.2013.02.007
Koprowski, J. L., Nandini, R. (2008). Global hotspots and knowledge gaps for tree and flying squirrels. Curr. Sci. 95, 851–856.
Kreye, J. K., Hiers, J. K., Varner, J. M., Hornsby, B., Drukker, S., O'Brien, J. J. (2018). Effects of solar heating on the moisture dynamics of forest floor litter in humid environments: composition, structure, and position matter. Can. J. For. Res. 48, 1331–1342. doi: 10.1139/cjfr-2018-0147
Kumar, D., (Ed.). (2012). Genomics and Health in the Developing World. Oxford: Oxford University Press.
Kuussaari, M., Bommarco, R., Heikkinen, R. K., Helm, A., Krauss, J., Lindborg, R., et al. (2009). Extinction debt: a challenge for biodiversity conservation. Trends Ecol. Evol. 24, 564–571. doi: 10.1016/j.tree.2009.04.011
Lasco, R. D., Delfino, R. J. P., Catacutan, D. C., Simelton, E. S., Wilson, D. M. (2014). Climate risk adaptation by smallholder farmers: the roles of trees and agroforestry. Curr. Opin. Environ. Sustain. 6, 83–88. doi: 10.1016/j.cosust.2013.11.013
Laurance, W. F., Sayer, J., Cassman, K. G. (2014). Agricultural expansion and its impacts on tropical nature. Trends Ecol. Evol. 29, 107–116. doi: 10.1016/j.tree.2013.12.001
Leffler, A. J., Ryel, R. J. (2012). “Resource pool dynamics: conditions that regulate species interactions and dominance,” in Invasive Plant Ecology and Management: Linking Processes to Practice, eds T. A. Monaco, and R. L. Sheley (Wallingford, CT: CABI), 57–78. doi: 10.1079/9781845938116.0057
Leibold, M. A., Chase, J. M. (2017). Metacommunity Ecology. Princeton University Press. doi: 10.2307/j.ctt1wf4d24
Leibold, M. A., Rudolph, J., Blanchet, F. G., Meester, L. D., Gravel, D., Hartig, F., et al. (2021). The internal structure of metacommunities. bioRxiv. 2022. doi: 10.1101/2020.07.04.187955
Liu, C., Wolter, C., Courchamp, F., Roura-Pascual, N., Jeschke, J. M. (2022). Biological invasions reveal how niche change affects the transferability of species distribution models. Ecology 103, e3719. doi: 10.1002/ecy.3719
Lowe, S., Browne, M., Boudjelas, S., De Poorter, M. (2000). 100 of the World's Worst Invasive Alien Species: A Selection From the Global Group. Auckland: The Invasive Species Specialist Group (ISSG) a specialist group of the Species Survival Commission (SSC) of the World Conservation Union (IUCN).
Mann, M. (2014). South Asia's Modern History: Thematic Perspectives. London: Routledge. doi: 10.4324/9781315754550
Meetei, A. B., Das, S., Campbell, P. D., Raha, S., Bag, P. (2018). A study on Ptyas doriae (Boulenger, 1888) with comments on the status of Ptyas hamptoni (Boulenger, 1900) (Squamata: Colubridae: Colubrinae). Zootaxa 4457, 537. doi: 10.11646/zootaxa.4457.4.3
Ministry of National Food Security and Research (2018). National Food Security Policy. Islamabad: Government of Pakistan.
Mittelbach, G. G., Schemske, D. W. (2015). Ecological and evolutionary perspectives on community assembly. Trends Ecol. Evol. 30, 241–247. doi: 10.1016/j.tree.2015.02.008
Mittermeier, R. A., Myers, N., Thomsen, J. B., da Fonseca, G. A. B., Olivieri, S. (1998). Biodiversity hotspots and major tropical wilderness areas: approaches to setting conservation priorities. Conserv. Biol. 12, 516–520. doi: 10.1046/j.1523-1739.1998.012003516.x
MOEF (2008). Bangladesh Climate Change Strategy and Action Plans 2008. Dhaka: Ministry of Environment and Forests Government of the People's Republic of Bangladesh.
Morán-Ordóñez, A., Lahoz-Monfort, J. J., Elith, J., Wintle, B. A. (2017). Evaluating 318 continental-scale species distribution models over a 60-year prediction horizon: what factors influence the reliability of predictions?: Temporal transferability of species distribution model predictions. Glob. Ecol. Biogeogr. 26, 371–384. doi: 10.1111/geb.12545
Murdiyarso, D., Van Noordwijk, M., Wasrin, U. R., Tomich, T. P., Gillison, A. N. (2002). Environmental benefits and sustainable land-use options in the Jambi transect, Sumatra, Indonesia. J. Veg. Sci. 13, 429–438. doi: 10.1111/j.1654-1103.2002.tb02067.x
Murphy, S. J., Smith, A. B. (2021). What can community ecologists learn from species distribution models? Ecosphere 12, e03864. doi: 10.1002/ecs2.3864
Nogués-Bravo, D. (2009). Predicting the past distribution of species climatic niches. Glob. Ecol. Biogeogr. 18, 521–531. doi: 10.1111/j.1466-8238.2009.00476.x
Noordwijk, M., Roshetko, J. M., Murniati, A. M. D., Suyanto, Fay, C., Tomich, T. P. (2003). Agroforestry is a Form of Sustainable Forest Management: Lessons From South East Asia. ICRAF Southeast Asia Working Paper No. 2003_2. Bogor: World Agroforestry Centre—ICRAF, SEA Regional Office.
Nuñez, M. A., Pauchard, A., Ricciardi, A. (2020). Invasion science and the global spread of SARS-CoV-2. Trends Ecol. Evol. 35, 642–645. doi: 10.1016/j.tree.2020.05.004
O'Donnell, M. S., Ignizio, D. A. (2012). Bioclimatic predictors for supporting ecological applications in the conterminous united states. US Geol. Survey Data Ser. 691, 10. doi: 10.3133/ds691
Olson, D. M., Dinerstein, E., Wikramanayake, E. D., Burgess, N. D., Powell, G. V. N., Underwood, E. C., et al. (2001). Terrestrial ecoregions of the world: a new map of life on Earth. Bioscience 51, 933–938. doi: 10.1641/0006-3568(2001)0510933:TEOTWA2.0.CO
Panda, R. M., Behera, M. D., Roy, P. S. (2018). Assessing distributions of two invasive species of contrasting habits in future climate. J. Environ. Manage. 213, 478–488. doi: 10.1016/j.jenvman.2017.12.053
Park, M. S., Baral, H., Shin, S. (2022). Systematic approach to agroforestry policies and practices in Asia. Forests 13, 635. doi: 10.3390/f13050635
Pearman, P. B., Guisan, A., Broennimann, O., Randin, C. F. (2008). Niche dynamics in space and time. Trends Ecol. Evol. 23, 149–158. doi: 10.1016/j.tree.2007.11.005
Peres-Neto, P. R., Legendre, P. (2010). Estimating and controlling for spatial structure in the study of ecological communities. Glob. Ecol. Biogeogr. 19, 174–184. doi: 10.1111/j.1466-8238.2009.00506.x
Petitpierre, B., Broennimann, O., Kueffer, C., Daehler, C., Guisan, A. (2017). Selecting predictors to maximize the transferability of species distribution models: lessons from cross continental plant invasions. Glob. Ecol. Biogeogr. 26, 275–287. doi: 10.1111/geb.12530
Phipps, W. L., Diekmann, M., MacTavish, L. M., Mendelsohn, J. M., Naidoo, V., Wolter, K., et al. (2017). Due south: a first assessment of the potential impacts of climate change on Cape vulture occurrence. Biol. Conserv. 210, 16–25. doi: 10.1016/j.biocon.2017.03.028
Pichler, M., Hartig, F. (2021). A new joint species distribution model for faster and more accurate inference of species associations from big community data. Methods Ecol. Evol. 12, 2159–2173. doi: 10.1111/2041-210X.13687
Pimentel, D., McNair, S., Janecka, J., Wightman, J., Simmonds, C., O'Connell, C., et al. (2001). Economic and environmental threats of alien plant, animal, and microbe invasions. Agric. Ecosyst. Environ. 84, 1–20. doi: 10.1016/S0167-8809(00)00178-X
Pimm, S. L., Jenkins, C. N., Abell, R., Brooks, T. M., Gittleman, J. L., Joppa, L. N., et al. (2014). The biodiversity of species and their rates of extinction, distribution, and protection. Science 344, 1246752. doi: 10.1126/science.1246752
Rahman, M. M., Kundu, G. K., Kabir, M. E., Ahmed, H., Xu, M. (2021). Assessing tree coverage and the direct and mediation effect of tree diversity on carbon storage through stand structure in homegardens of southwestern Bangladesh. Forests 12, 1661. doi: 10.3390/f12121661
Ramachandran, R. M., Roy, P. S., Chakravarthi, V., Joshi, P. K., Sanjay, J. (2020). Land use and climate change impacts on distribution of plant species of conservation value in Eastern Ghats, India: a simulation study. Environ. Monit. Assess. 192, 86. doi: 10.1007/s10661-019-8044-5
Ramachandran, R. M., Roy, P. S., Chakravarthi, V., Sanjay, J., Joshi, P. K. (2018). Long-term land use and land cover changes (1920–2015) in Eastern Ghats, India: pattern of dynamics and challenges in plant species conservation. Ecol. Indic. 85, 21–36. doi: 10.1016/j.ecolind.2017.10.012
Rana, S. K., Rana, H. K., Shrestha, K. K., Sujakhu, S., Ranjitkar, S. (2018). Determining bioclimatic space of Himalayan alder for agroforestry systems in Nepal. Plant Divers. 40, 1–18. doi: 10.1016/j.pld.2017.11.002
Ranjitkar, S., Kindt, R., Sujakhu, N. M., Hart, R., Guo, W., Yang, X., et al. (2014). Separation of the bioclimatic spaces of Himalayan tree rhododendron species predicted by ensemble suitability models. Glob. Ecol. Conserv. 1, 2–12. doi: 10.1016/j.gecco.2014.07.001
Ratnayake, S. S., Kumar, L., Kariyawasam, C. S. (2019). Neglected and underutilized fruit species in Sri Lanka: prioritisation and understanding the potential distribution under climate change. Agronomy 10, 34. doi: 10.3390/agronomy10010034
Remya, K., Ramachandran, A., Jayakumar, S. (2015). Predicting the current and future suitable habitat distribution of Myristica dactyloides Gaertn. using MaxEnt model in the Eastern Ghats, India. Ecol. Eng. 82, 184–188. doi: 10.1016/j.ecoleng.2015.04.053
Richmond, O. M. W., McEntee, J. P., Hijmans, R., Brashares, J. S. (2010). Is the climate right for pleistocene rewilding? Using species distribution models to extrapolate climatic suitability for mammals across continents emphasize the significance of findings. PLoS ONE 5, e12899. doi: 10.1371/journal.pone.0012899
Ripple, W. J., Chapron, G., López-Bao, J. V., Durant, S. M., Macdonald, D. W., Lindsey, P. A., et al. (2016). Saving the world's terrestrial megafauna. BioScience 66, 807–812. doi: 10.1093/biosci/biw092
Ross, M. R. V., Topp, S. N., Appling, A. P., Yang, X., Kuhn, C., Butman, D., et al. (2019). AquaSat: a data set to enable remote sensing of water quality for inland waters. Water Resour. Res. 55, 10012–10025. doi: 10.1029/2019WR024883
Roy, S., Debnath, S., Saikia, S. K. (2021a). “Threats to freshwater fish and application of community ecology studies in conservation,” in Current Approaches in Science and Technology Research Vol. 6, ed E. A. Makky (West Bengal: Book Publisher International), 69–76. doi: 10.9734/bpi/castr/v6/2462F
Roy, S., Ray, S., Saikia, S. K. (2021b). Indicator environmental variables in regulating the distribution patterns of small freshwater fish Amblypharyngodon mola in India and Bangladesh. Ecol. Indic. 120, 106906. doi: 10.1016/j.ecolind.2020.106906
Rupasinghe, U. A. L. D., Vandercone, R. P. G., Ukuwela, K. D. B. (2021). Habitat associations and distribution modeling of the endangered frog Pseudophilautus zorro in Sri Lanka. Herpetol. Conserv. Biol. 16, 38–46.
Russell, J. C., Blackburn, T. M. (2017). The rise of invasive species denialism. Trends Ecol. Evol. 32, 3–6. doi: 10.1016/j.tree.2016.10.012
Salick, J., Fang, Z., Byg, A. (2009). Eastern Himalayan alpine plant ecology, Tibetan ethnobotany, and climate change. Glob. Environ. Change 19, 147–155. doi: 10.1016/j.gloenvcha.2009.01.008
Sarma, R. R., Munsi, M., Neelavara Ananthram, A. (2015). Effect of climate change on invasion risk of giant african snail (Achatina fulica Férussac, 1821: Achatinidae) in India. PLoS ONE 10, e0143724. doi: 10.1371/journal.pone.0143724
Sekercioglu, Ç. H., Daily, G. C., Ehrlich, P. R. (2004). Ecosystem consequences of bird declines. Proc. Natl. Acad. Sci. U.S.A. 101, 18042–18047. doi: 10.1073/pnas.0408049101
Servheen, C. (1990). The Atatus and Conservation of the Bears of the World, 2nd Edn. Victoria, BC: International Association for Bear Research and Management, British Columbia.
Sharma, G. P., Raghubanshi, A. S., Singh, J. S. (2005). Lantana invasion: an overview. Weed Biol. Manage. 5, 157–165. doi: 10.1111/j.1445-6664.2005.00178.x
Sharma, R., Sinha, A., Kautish, P. (2020). Examining the impacts of economic and demographic aspects on the ecological footprint in South and Southeast Asian countries. Environ. Sci. Pollut. Res. 27, 36970–36982. doi: 10.1007/s11356-020-09659-3
Sharma, R., Xu, J., Sharma, G. (2007). Traditional agroforestry in the Eastern Himalayan region: land management system supporting ecosystem services. Trop. Ecol. 48, 1–12.
Shrestha, U. B., Shrestha, B. B. (2019). Climate change amplifies plant invasion hotspots in Nepal. Divers. Distrib. 25, 1599–1612. doi: 10.1111/ddi.12963
Singh, A. K., Dhyani, S. K. (2014). “Agroforestry policy issues and challenges,” in Agroforestry Systems in India: Livelihood Security and Ecosystem Services, eds J. C. Dagar, A. K. Singh, and A. Arunachalam (New Delhi: Springer), 367–372. doi: 10.1007/978-81-322-1662-9_12
Singh, J. S. (1968). Comparison of growth performance and germination behaviour of seeds of Cassia tora L. and C. obtusifolia L. Trop. Ecol. 9, 64–71.
Soberón, J., Nakamura, M. (2009). Niches and distributional areas: concepts, methods, and assumptions. Proc. Natl. Acad. Sci. U.S.A. 06, 19644–19650. doi: 10.1073/pnas.0901637106
Squires, D. (2013). Biodiversity conservation in Asia. Asia Pac. Policy Stud. 1, 144–159. doi: 10.1002/app5.13
Srinivasulu, A., Srinivasulu, B., Srinivasulu, C. (2021). Ecological niche modelling for the conservation of endemic threatened squamates (lizards and snakes) in the Western Ghats. Glob. Ecol. Conserv. 28, e01700. doi: 10.1016/j.gecco.2021.e01700
Straub, M. H., Kelly, T. R., Rideout, B. A., Eng, C., Wynne, J., Braun, J., et al. (2015). Seroepidemiologic survey of potential pathogens in obligate and facultative scavenging avian species in California. PLoS ONE 10, e0143018. doi: 10.1371/journal.pone.0143018
Sunday, J. M., Bates, A. E., Dulvy, N. K. (2012). Thermal tolerance and the global redistribution of animals. Nat. Clim. Change 2, 686–690. doi: 10.1038/nclimate1539
Thiney, U., Banterng, P., Gonkhamdee, S., Katawatin, R. (2019). Distributions of alien invasive weeds under climate change scenarios in mountainous Bhutan. Agronomy 9, 442. doi: 10.3390/agronomy9080442
United Nations Economic and Social Commission for Asia and the Pacific (2020). Asia and the Pacific SDG Progress Report. United Nations Economic and Social Commission for Asia and the Pacific. Available online at: https://www.unescap.org/publications/asia-and-pacific-sdg-progress-report-2020
Urbina-Cardona, N., Blair, M. E., Londono, M. C., Loyola, R., Vela'squez-Tibata, J., Morales-Devia, H. (2019). Species distribution modeling in Latin America: a 25-year retrospective review. Trop. Conserv. Sci. 12, 1–9. doi: 10.1177/1940082919854058
Vaidyanathan, G. (2018). Science and culture: imagining a climate-change future, without the dystopia. Proc. Natl. Acad. Sci. U.S.A. 115, 12832–12835. doi: 10.1073/pnas.1819792116
Virkkala, R., Luoto, M., Heikkinen, R. K., Leikola, N. (2005). Distribution patterns of boreal marshland birds: modelling the relationships to land cover and climate. J. Biogeogr. 32, 1957–1970. doi: 10.1111/j.1365-2699.2005.01326.x
Vitousek, P. M. (1986). “Biological invasions and ecosystem properties: can species make a difference?,” in Biological Invasions of North America and Hawaii, eds H. A. Mooney, and J. Drake (New York, NY: Springer) 163–176. doi: 10.1007/978-1-4612-4988-7_10
Werkowska, W., Marquez, A. L., Real, R., Acevedo, P. (2017). A practical overview of transferability in species distribution modeling. Environ. Rev. 25, 127–133. doi: 10.1139/er-2016-0045
Wiens, J. J., Ackerly, D. D., Allen, A. P., Anacker, B. L., Buckley, L. B., Cornell, H. V., et al. (2010). Niche conservatism as an emerging principle in ecology and conservation biology. Ecol. Lett. 13, 1310–1324. doi: 10.1111/j.1461-0248.2010.01515.x
Wisz, M. S., Pottier, J., Kissling, W. D., Pellissier, L., Lenoir, J., Damgaard, C. F., et al. (2013). The role of biotic interactions in shaping distributions and realised assemblages of species: implications for species distribution modelling. Biol. Rev. Camb. Philos. Soc. 88, 15–30. doi: 10.1111/j.1469-185X.2012.00235.x
World Bank (2017). Pakistan Development Update. Growth—A Shared Responsibility. Islamabad: World Bank. Available online at: https://openknowledge.worldbank.org/handle/10986/27544
Yates, K. L., Bouchet, P. J., Caley, M. J., Mengersen, K., Randin, C. F., Parnell, S., et al. (2018). Outstanding challenges in the transferability of ecological models. Trends Ecol. Evol. 33, 790–802. doi: 10.1016/j.tree.2018.08.001
Yorifuji, T., Bae, S., Kashima, S., Tsuda, T., Doi, H., Honda, Y., et al. (2015). Health impact assessment of PM10 and PM2.5 in 27 Southeast and east Asian cities. J. Occup. Environ. Med. 57, 751–756. doi: 10.1097/JOM.0000000000000485
Zabel, F., Putzenlechner, B., Mauser, W. (2014). Global agricultural land resources – a high resolution suitability evaluation and its perspectives until 2100 under climate change conditions. PLoS ONE 9, e107522. doi: 10.1371/journal.pone.0107522
Keywords: species niche, threats to biodiversity, species extinction, Scopus search, habitat conservation, sustainable management policy
Citation: Roy S, Suman A, Ray S and Saikia SK (2022) Use of species distribution models to study habitat suitability for sustainable management and conservation in the Indian subcontinent: A decade's retrospective. Front. Sustain. Resour. Manag. 1:1031646. doi: 10.3389/fsrma.2022.1031646
Received: 30 August 2022; Accepted: 18 November 2022;
Published: 19 December 2022.
Edited by:
Naser Valizadeh, Shiraz University, IranReviewed by:
Emran Dastres, Shiraz University, IranMohsen Edalat, Shiraz University, Iran
Arnab Banerjee, Indian Statistical Institute, India
Copyright © 2022 Roy, Suman, Ray and Saikia. This is an open-access article distributed under the terms of the Creative Commons Attribution License (CC BY). The use, distribution or reproduction in other forums is permitted, provided the original author(s) and the copyright owner(s) are credited and that the original publication in this journal is cited, in accordance with accepted academic practice. No use, distribution or reproduction is permitted which does not comply with these terms.
*Correspondence: Santanu Ray, santanu.ray@visva-bharati.ac.in
†Present address: Santanu Ray, Kerala University of Digital Sciences, Innovation and Technology, Thiruvananthapuram, Kerala, India