- 1Temple Heart and Vascular Institute, Temple University Hospital, Philadelphia, PA, United States
- 2Jefferson Heart Institute, Thomas Jefferson University, Philadelphia, PA, United States
- 3Department of Medicine-Cardiovascular Division, University of Wisconsin Hospital, Madison, WI, United States
Cardiopulmonary exercise testing allows for a comprehensive assessment of the mechanism of exercise limitation in pulmonary arterial hypertension and chronic thromboembolic pulmonary hypertension. Competitive pathophysiologic mechanisms may affect the clinical interpretation of cardiopulmonary disease as they relate to dyspnea, leg fatigue, and exercise intolerance.
1 Introduction
Cardiopulmonary exercise testing (CPET) is a diagnostic tool used to investigate functional capacity and characterize exercise limitations related to circulatory impairment and ventilatory inefficiency (1, 2). It is a durable and versatile test that provides invaluable information on a patient's cardiovascular and pulmonary disease. Traditionally the 6 min walk distance is routinely used to assess the functional capacity of patients with PH for risk stratification, but can lack cardiopulmonary specificity. Therefore current ESC/ERS guidelines (3) recommend CPET for further evaluation in symptomatic patients with intermediate echocardiographic findings of pulmonary hypertension (PH), therefore we will try to provide key parameters to understand and interpret those findings. CPET allows the analysis of gas exchange at rest as well as during exercise and recovery, using breath-by-breath analysis of oxygen uptake (VO2), carbon dioxide output (VCO2), and ventilation (VE). This data is integrated with measurements of symptoms, heart rate, blood pressure, work rate, and electrocardiographic findings.
The definition of PH was recently updated to emphasize early disease detection in the guidelines from the World Health Organization (WHO) -European Society of Cardiology (3). Currently, PH is defined as a mean pulmonary artery pressure (mPAP) of greater than 20 mmHg and pulmonary vascular resistance (PVR) of greater than 2 Wood Units. There are 5 clinical categories based on the etiology of PH, and we will focus on pulmonary arterial hypertension (PAH)- WHO Group 1, and chronic thromboembolic pulmonary hypertension (CTEPH)- WHO Group 4.
PAH is precapillary PH characterized by endothelial dysfunction leading to increased pulmonary vascular resistance (3). It can be idiopathic, hereditary, or associated with predisposing diseases. CTEPH is an uncommon complication of pulmonary embolism (PE) defined by the presence of precapillary PH with angiographic evidence (i.e., computed tomography of the chest, magnetic resonance imaging of the pulmonary arteries, catheter-based pulmonary angiography) of organized thrombotic residua within the pulmonary arteries that are present in spite of at least 3 months of anticoagulation therapy. For those with imaging evidence of chronic PE in the absence of rest-hemodynamic evidence of PH, the diagnosis of chronic thromboembolic disease (CTED), or most recently the proposed designation, chronic thromboembolic pulmonary disease (CTEPD), is suggested.
In both PAH and CTEPH, there are multiple variables affecting cardiac function and pulmonary gas exchange leading to exercise intolerance and exertional dyspnea. These changes reflect the underlying exercise pathophysiology and can be evaluated via CPET. This review summarizes the developments and evidence on the application of CPET in PH.
2 Practical considerations and key parameters of cardiopulmonary exercise testing
The usefulness of the CPET depends on an individual's capacity to perform physical exercise, evaluating the ability of the pulmonary system's ability to clear CO2 and the cardiovascular system's ability to supply oxygen to the muscles. There is a close evaluation of the delivery and removal systems (i.e., ventilation, diffusion, transport of O2 and CO2 in the blood, and capillary gas exchange). The increase in oxygen uptake by the peripheral muscles is a response to an increase in cardiac output (CO = heart rate × stroke volume), which increases 6 times with exercise. The cardiac output is distributed to skeletal muscles and shunted away from the non-active tissues (e.g., splanchnic circulation). There is an increase in blood flow to the lung in addition to vasodilation of the pulmonary vessel, with increased extraction of O2 from the blood to the muscles, resulting in an increased arteriovenous oxygen difference. Ventilation (VE) increases proportionally with the work rate. When inhalation takes place, the air in the alveoli, known as the tidal volume (Vt), accounts for the gas exchange. The air not participating in this exchange, is known as the dead space (Vd). During exercise, both dilation of the respiratory passages and the effective areas of gas exchange (tidal volume) increase, therefore gas exchange remains intact. The increase in VE should be matched with an increase in blood flow, hence an appropriate cardiac output is important to maintain gas exchange. The point at which oxygen uptake is unable to increase adequately for peripheral oxygen demand marks the aerobic-anaerobic transition at which lactic acidosis will begin to occur. This is called the anaerobic threshold. If at any point there is a mismatch in this supply-demand chain there will be a clinical symptom.
The assessment of exercise capacity can be performed via a treadmill or stationary cycle ergometer. The selection of the exercise modality will depend on the patient and center's availability. Treadmills are preferred. Meanwhile, cycle ergometers are preferred in cases of gait and balance instability, severe obesity, orthopedic limitations, or when simultaneous right heart catheterization or echocardiogram is planned. The Bal and Ware, Naughton, and individualized ramp protocol involve increments in work rate per stage. They are recommended, as they allow to establish relationships between measured VO2 and work rate. Individuals should be expected to exercise for 8–12 min (4). Table 1 summarizes the CPET variables that are measured and calculated during the study (1, 3). Normal values may be affected in some individuals depending on their age, gender, volitional effort, and comorbidities.
3 Physiology of exercise in PAH and CTEPH
During exercise, peripheral muscles have increased oxygen demand that is achieved by peripheral vasodilation and increased oxygen extraction. When the normal individual exercises, the cardiac output should increase to match this oxygen delivery for the increasing demand of peripheral muscles (10). In the lungs increased tidal volume and pulmonary blood flow (achieved via vasodilation and pulmonary capillary recruitment) allows for a proportional increase in alveolar oxygen uptake. The maximal achievable oxygen uptake is labeled as the peak O2 (peak VO2) (5). In the alveoli, the carbon dioxide is cleared by a corresponding increase in minute ventilation (VE = Vt × respiratory rate). The point at which oxygen uptake is unable to increase adequately for peripheral oxygen demand marks the aerobic-anaerobic transition at which lactic acidosis will begin to occur. This point is called the anaerobic threshold.
The physiological derangements in PH originate from obliteration and obstruction of pulmonary arteries (Figure 1). In PAH, vascular inflammation, remodeling, and endothelial dysfunction precipitate an increase in pulmonary vascular resistance. There is an imbalance in the production of pulmonary vasodilators and vasoconstrictors, abnormal proliferation of cells in the walls of the pulmonary arteries and arterioles, and intraluminal thrombus (11, 12). In CTEPH, residual thrombotic material causes pulmonary arterial obstruction, hindering pulmonary vasodilation and increasing dead space ventilation. In PAH and CTEPH, there is a decrease in the vasodilatory capacity, as well as in the distensibility and patency of the pulmonary vasculature. This hypoperfusion to ventilated lung regions is reflected as low PETCO2. In both entities, PAH and CTEPH, there is an increase in afterload, or pulmonary vascular resistance, which limits the increased stroke volume needed for effective circulation at the time of exercise (10, 13, 14). The pulmonary vascular bed of PH patients is unable to be recruited and distended, leading to increased pulmonary artery pressures (10). The sustained increased afterload causes adaptive myocardial hypertrophy, which is initially reflected by RV wall hypertrophy, and later on by RV dilation (15, 16). There can be RV ischemia related to imbalanced oxygen supply and demand associated with hypertrophy, increased RV workload, and metabolic demands, without an increase in blood supply or microcirculation (17). In addition, there is uncoupling between the pulmonary vascular bed and the right ventricle, leading to right heart failure at peak exercise and inability to augment CO. In addition, elevated right ventricular pressure and dilation can cause interventricular septal shifting, which further reduces the pulmonary venous return to the left atrium, thereby decreasing left ventricular diastolic filling, systemic cardiac output, and tissue oxygen delivery (18–20).
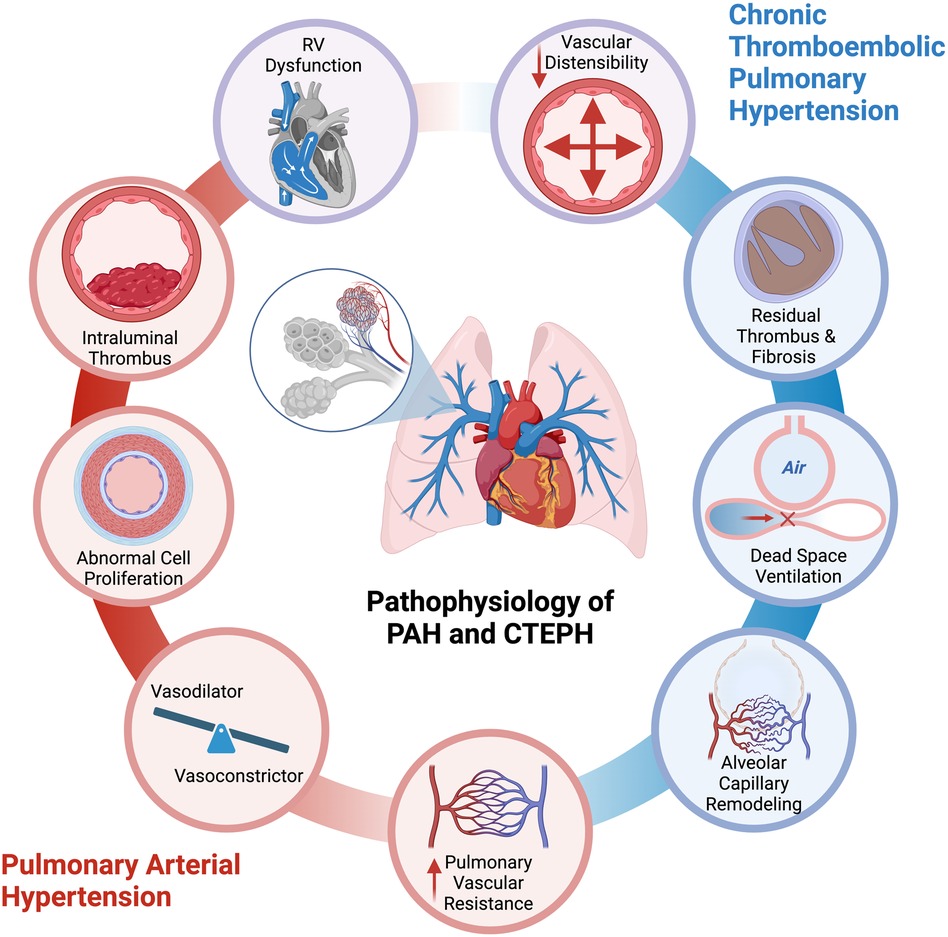
Figure 1. Pathogenesis of exercise limitation in pulmonary arterial hypertension and chronic thromboembolic pulmonary hypertension. (Created with biorender).
The resulting hypoxia leads to vasoconstriction and impaired tissue oxygen delivery to peripheral muscles, causing lactic acidosis, lowered peak VO2, and a reduction in the anaerobic threshold. This leads to the production of VCO2 and an excessive increase in ventilation during exercise (increased VE/VCO2). This results in the characteristic CPET pattern of PH with low PETCO2, high ventilatory equivalent for carbon dioxide (VE/VCO2), and low peak O2 uptake (peak VO2).
3.1 Indications and contraindications for CPET
Patients with PH can have nonspecific symptoms, such as fatigue, dyspnea, and exercise intolerance. CPET can help identify the source of exercise intolerance, monitoring disease progression or treatment response, and aiding in prognosis. Ultimately, CPET allows for physiologic risk stratification. Table 2 includes the absolute and relative contraindications. CPET can provide reproducible cardiovascular-specific information as you can record heart rate, electrocardiogram, blood pressure, and gas exchange patterns. The primary measurements made are respiratory rate, end-tidal pO2, and pCO2. Other parameters, such as O2 pulse, and ventilatory equivalents for O2 and CO2 are also obtained. Individuals should reach an RER >1.05. There are some limitations with the interpretation of the data depending on the volitional effort of the individual, but the interpretation can be done in the context of the maximum HR and RER achieved. The patient does not require prior training to achieve better performance. CPET can rule out other factors affecting the ability to exercise, such as anemia, pulmonary mechanical pathology, or skeletal muscle myopathy. CPET in patients with PH is highly reproducible and provide robust endpoints to assess exercise capacity (21).
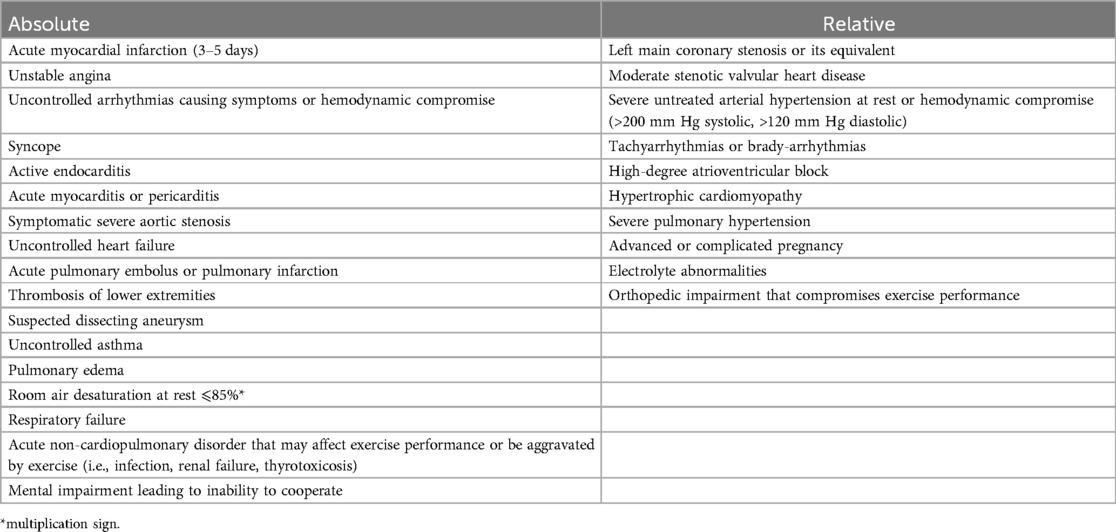
Table 2. Absolute and relative contraindications for cardiopulmonary exercise testing (adapted from ATS/ACCP statement on cardiopulmonary exercise testing) (1).
Although CPET is generally well-tolerated, contraindications include physical barriers to exercise or decompensated disease state including but not limited to active myocardial ischemia, uncontrolled arrhythmia, severe aortic stenosis, COPD exacerbation, uncontrolled asthma, or other acute respiratory failure (22). Barriers for implementation can be related to confidence in interpreting results and lack of equipment or trained technicians.
3.2 Cardiopulmonary exercise testing in pulmonary arterial hypertension
The goal of CPET in PAH is to provide an early diagnosis, help stratify individuals with intermediate risk PH, identify peripheral muscle abnormalities related to PAH, and prognosticate and evaluate the efficacy of therapeutic interventions (23, 24) (Figure 2). The use of CPET in PAH was derived from its use in left heart failure (25). The mechanisms that impair exercise in PAH are: (1) inability to vasodilate the pulmonary vascular bed (i.e., impaired distensibility and vasodilation and smaller vessels) (26); (2) reduction in the RV contractility (19); (3) chronotropic impairment (27); (4) abnormal gas exchange and hypoxemia; (5) chemoreceptor activation; (6) endothelial dysfunction; and (7) skeletal muscle myopathy. All of these are measurable with CPET. The main abnormalities recognized in PAH are called ventilatory inefficiencies and are determined by an abnormally high ratio of ventilation to CO2 production (VE/VCO2), as well as a low end-tidal pCO2 (PETCO2), and thus dead space ventilation, which in turn have proven to be reliable physiologic signatures of pulmonary vascular disease across a variety of PH conditions (28–31). Peak VO2 is prognostic in PAH. Wensel et al. (32) first reported the prognostic capability of CPET in PAH showing that a low peak VO2 of 10.4 mL/kg/min and a low peak exercise systolic blood pressure of less than 120 mmHg were associated with worse survival as compared to those with one or none of those factors.
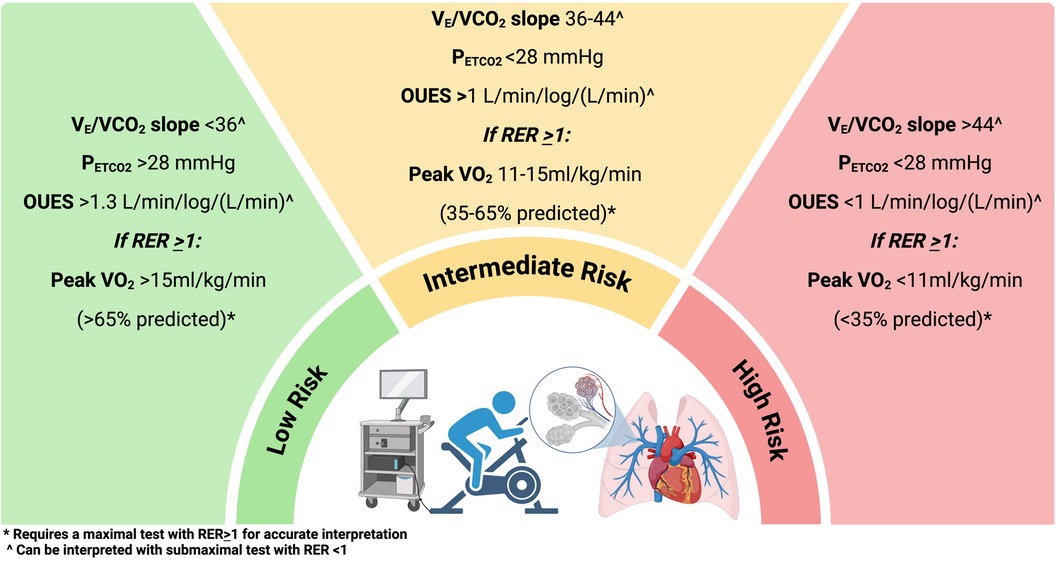
Figure 2. Cardiopulmonary exercise testing stratification in pulmonary arterial hypertension and chronic thromboembolic pulmonary hypertension. (Created with biorender).
Oxygen pulse reflects the capacity and efficiency of the heart to deliver oxygen per heartbeat and can be related to stroke volume or peripheral arterial extraction. Reduction in O2 pulse as the work rate increases suggests an issue with oxygen delivery. The ratio of change in oxygen consumption to changes in work rate (exercise factor) is usually 10 mL/min/W in healthy individuals. If this ratio cannot be achieved, it suggests impairments in the cardiac output with exercise. The gas exchange parameters will imply the presence of PH, but ultimately cardiac catheterization will be required to make the diagnosis of PAH.
With regards to diagnosis, current ESC/ERS guidelines include CPET among the recommended evaluations for patients with unexplained exertional dyspnea with indeterminate echo findings for PH (3). In symptomatic patients with systemic sclerosis, CPET with normal peak VO2 suggests against the presence of PH, reducing the need for screening RHC (33). Similarly, for patients with connective-tissue diseases, HIV, or portal hypertension, CPET is a recommended modality to screen for PAH. Additionally, CPET as an endpoint in clinical trials has been used in many consensus working groups.
3.3 Cardiopulmonary exercise testing in chronic thromboembolic pulmonary disease
Patients with persistent symptoms after an acute pulmonary embolism with a “post-PE syndrome” can benefit from further characterization of their impairment (34). Patients with CTEPH and CTEPD can have similar symptoms and perfusion defects, but the difference is in the lack of resting PH in the latter. Investigators have described in CTEPD a marked reduction in peak VO2, high VE/VCO2 at anaerobic threshold, and slightly decreased PETCO2 (35). In a study combining CPET with right heart catheterization and stress echocardiography, Peak VO2 was negatively correlated with mPAP and PVR, while VE/VCO2 at AT was positively correlated with mPAP and PVR (35).
Regarding patients with concerns for CTEPH, CPET is included in the recommended workup along with echocardiography and BNP/NT-proBNP (36). This is corroborated by data from Held et al. (37), who described that a score combining P(A−a)O2, P(c−ET)CO2 (capillary to end tidal carbon dioxide gradient), PETCO2 at anaerobic threshold, and VE/VCO2 slope, had a sensitivity of 83.3% and a specificity of 92.2% for the diagnosis of CTEPH, even in patients whose echocardiogram was normal. Zhu et al. (38) divided CTEPH patients by levels of severity defined by mean pulmonary artery criteria, and described the parameters associated with significant differences in oxygen uptake efficiency slope and plateau, VE/VCO2, peak VO2 and VE at anaerobic threshold depending of the pulmonary artery pressure.
CPET is also useful to monitor response to treatment and assess disease severity in CTEPH. It has been used to monitor response in surgical patients post pulmonary thromboendarterectomy, balloon pulmonary angioplasty and inoperable patients medically managed with PDE5 inhibitors or endothelin receptor agonists (39, 40).
The differences between PAH and CTEPH have been described by some authors. Sun et al. (41) have described lower PETCO2 than in PAH when compared to CTEPH patients. Other parameters that have been compared is the response of oxygen uptake efficiency at peak and anaerobic threshold, and patient with PAH have higher values than patients with CTEPH not in proportion with hemodynamic severity (42).
4 Conclusion
As PAH and CTEPH diagnosis and management continue to improve the evaluation of the exercise capacity of individuals with the use of CPET may be a key tool to understand the true source of their limitations. Abnormal responses to exercise can be better characterized with the use of CPET and allow for physiologically tailored interventions. We can help stratify the disease via this comprehensive and reproducible method.
Author contributions
AC: Writing – review & editing, Writing – original draft. SL: Writing – review & editing, Writing – original draft, Visualization, Resources. GR: Writing – original draft, Investigation. YB: Writing – review & editing, Visualization, Resources, Data curation. FR: Writing – review & editing, Visualization, Supervision, Resources, Investigation, Funding acquisition, Conceptualization. EO: Writing – review & editing, Writing – original draft, Visualization, Validation, Supervision, Software, Resources, Project administration, Methodology, Investigation, Funding acquisition, Formal Analysis, Data curation, Conceptualization.
Funding
The author(s) declare financial support was received for the research, authorship, and/or publication of this article. This study was supported from the AHA Career Grant 23CDA1057697 (FR) and NIH/NCATS ICTR KL2TR002374-07 (FR). The content is solely the responsibility of the authors and does not necessarily represent the official views of the AHA or NIH.
Conflict of interest
The authors declare that the research was conducted in the absence of any commercial or financial relationships that could be construed as a potential conflict of interest.
Publisher's note
All claims expressed in this article are solely those of the authors and do not necessarily represent those of their affiliated organizations, or those of the publisher, the editors and the reviewers. Any product that may be evaluated in this article, or claim that may be made by its manufacturer, is not guaranteed or endorsed by the publisher.
References
1. American Thoracic S, American College of Chest P. ATS/ACCP statement on cardiopulmonary exercise testing. Am J Respir Crit Care Med. (2003) 167(2):211–77. doi: 10.1164/rccm.167.2.211
2. Lewis GD, Docherty KF, Voors AA, Cohen-Solal A, Metra M, Whellan DJ, et al. Developments in exercise capacity assessment in heart failure clinical trials and the rationale for the design of METEORIC-HF. Circ Heart Fail. (2022) 15(5):e008970. doi: 10.1161/CIRCHEARTFAILURE.121.008970
3. Humbert M, Kovacs G, Hoeper MM, Badagliacca R, Berger RMF, Brida M, et al. 2022 ESC/ERS guidelines for the diagnosis and treatment of pulmonary hypertension. Eur Heart J. (2022) 43(38):3618–731. doi: 10.1093/eurheartj/ehac237
4. Balady GJ, Arena R, Sietsema K, Myers J, Coke L, Fletcher GF, et al. Clinician’s guide to cardiopulmonary exercise testing in adults. Circulation. (2010) 122(2):191–225. doi: 10.1161/CIR.0b013e3181e52e69
5. Glaab T, Taube C. Practical guide to cardiopulmonary exercise testing in adults. Respir Res. (2022) 23(1):9. doi: 10.1186/s12931-021-01895-6
6. Davies LC, Wensel R, Georgiadou P, Cicoira M, Coats AJS, Piepoli MF, et al. Enhanced prognostic value from cardiopulmonary exercise testing in chronic heart failure by non-linear analysis: oxygen uptake efficiency slope. Eur Heart J. (2005) 27(6):684–90. doi: 10.1093/eurheartj/ehi672
7. Tang Y, Luo Q, Liu Z, Ma X, Zhao Z, Huang Z, et al. Oxygen uptake efficiency slope predicts poor outcome in patients with idiopathic pulmonary arterial hypertension. J Am Heart Assoc. (2017) 6(7):e005037. doi: 10.1161/JAHA.116.005037
8. Cardoso DM, Gass R, Sbruzzi G, Berton DC, Knorst MM. Effect of the expiratory positive airway pressure on dynamic hyperinflation and exercise capacity in patients with COPD: a meta-analysis. Sci Rep. (2020) 10(1):13292. doi: 10.1038/s41598-020-70250-4
9. Datta D, Normandin E, ZuWallack R. Cardiopulmonary exercise testing in the assessment of exertional dyspnea. Ann Thorac Med. (2015) 10(2):77–86. doi: 10.4103/1817-1737.151438
10. Janicki JS, Weber KT, Likoff MJ, Fishman AP. The pressure-flow response of the pulmonary circulation in patients with heart failure and pulmonary vascular disease. Circulation. (1985) 72(6):1270–8. doi: 10.1161/01.CIR.72.6.1270
11. Humbert M, Morrell NW, Archer SL, Stenmark KR, MacLean MR, Lang IM, et al. Cellular and molecular pathobiology of pulmonary arterial hypertension. J Am Coll Cardiol. (2004) 43(12 Suppl S):13s–24. doi: 10.1016/j.jacc.2004.02.029
12. Michelakis ED, Wilkins MR, Rabinovitch M. Emerging concepts and translational priorities in pulmonary arterial hypertension. Circulation. (2008) 118(14):1486–95. doi: 10.1161/CIRCULATIONAHA.106.673988
13. Naeije R, Vanderpool R, Dhakal BP, Saggar R, Saggar R, Vachiery JL, et al. Exercise-induced pulmonary hypertension: physiological basis and methodological concerns. Am J Respir Crit Care Med. (2013) 187(6):576–83. doi: 10.1164/rccm.201211-2090CI
14. Groepenhoff H, Westerhof N, Jacobs W, Boonstra A, Postmus PE, Vonk-Noordegraaf A. Exercise stroke volume and heart rate response differ in right and left heart failure. Eur J Heart Fail. (2010) 12(7):716–20. doi: 10.1093/eurjhf/hfq062
15. Haddad F, Hunt SA, Rosenthal DN, Murphy DJ. Right ventricular function in cardiovascular disease, part I: anatomy, physiology, aging, and functional assessment of the right ventricle. Circulation. (2008) 117(11):1436–48. doi: 10.1161/CIRCULATIONAHA.107.653576
16. Haddad F, Doyle R, Murphy DJ, Hunt SA. Right ventricular function in cardiovascular disease, part II: pathophysiology, clinical importance, and management of right ventricular failure. Circulation. (2008) 117(13):1717–31. doi: 10.1161/CIRCULATIONAHA.107.653584
17. Naeije R, Manes A. The right ventricle in pulmonary arterial hypertension. Eur Respir Rev. (2014) 23(134):476–87. doi: 10.1183/09059180.00007414
18. Nootens M, Wolfkiel CJ, Chomka EV, Rich S. Understanding right and left ventricular systolic function and interactions at rest and with exercise in primary pulmonary hypertension. Am J Cardiol. (1995) 75(5):374–7. doi: 10.1016/S0002-9149(99)80557-8
19. Holverda S, Gan CT, Marcus JT, Postmus PE, Boonstra A, Vonk-Noordegraaf A. Impaired stroke volume response to exercise in pulmonary arterial hypertension. J Am Coll Cardiol. (2006) 47(8):1732–3. doi: 10.1016/j.jacc.2006.01.048
20. Kasner M, Westermann D, Steendijk P, Dröse S, Poller W, Schultheiss HP, et al. Left ventricular dysfunction induced by nonsevere idiopathic pulmonary arterial hypertension: a pressure-volume relationship study. Am J Respir Crit Care Med. (2012) 186(2):181–9. doi: 10.1164/rccm.201110-1860OC
21. Hansen JE, Sun XG, Yasunobu Y, Garafano RP, Gates G, Barst RJ, et al. Reproducibility of cardiopulmonary exercise measurements in patients with pulmonary arterial hypertension. Chest. (2004) 126(3):816–24. doi: 10.1378/chest.126.3.816
22. Albouaini K, Egred M, Alahmar A, Wright DJ. Cardiopulmonary exercise testing and its application. Postgrad Med J. (2007) 83(985):675–82. doi: 10.1136/hrt.2007.121558
23. Markowitz DH, Systrom DM. Diagnosis of pulmonary vascular limit to exercise by cardiopulmonary exercise testing. J Heart Lung Transplant. (2004) 23(1):88–95. doi: 10.1016/S1053-2498(03)00064-0
24. Tolle JJ, Waxman AB, Van Horn TL, Pappagianopoulos PP, Systrom DM. Exercise-induced pulmonary arterial hypertension. Circulation. (2008) 118(21):2183–9. doi: 10.1161/CIRCULATIONAHA.108.787101
25. Mancini DM, Eisen H, Kussmaul W, Mull R, Edmunds LH Jr., Wilson JR. Value of peak exercise oxygen consumption for optimal timing of cardiac transplantation in ambulatory patients with heart failure. Circulation. (1991) 83(3):778–86. doi: 10.1161/01.CIR.83.3.778
26. Provencher S, Hervé P, Sitbon O, Humbert M, Simonneau G, Chemla D. Changes in exercise haemodynamics during treatment in pulmonary arterial hypertension. Eur Respir J. (2008) 32(2):393–8. doi: 10.1183/09031936.00009008
27. Provencher S, Chemla D, Hervé P, Sitbon O, Humbert M, Simonneau G. Heart rate responses during the 6-minute walk test in pulmonary arterial hypertension. Eur Respir J. (2006) 27(1):114–20. doi: 10.1183/09031936.06.00042705
28. Claeys M, Claessen G, La Gerche A, Petit T, Belge C, Meyns B, et al. Impaired cardiac reserve and abnormal vascular load limit exercise capacity in chronic thromboembolic disease. JACC Cardiovasc Imaging. (2019) 12(8 Pt 1):1444–56. doi: 10.1016/j.jcmg.2018.07.021
29. Kikuchi H, Goda A, Takeuchi K, Inami T, Kohno T, Sakata K, et al. Exercise intolerance in chronic thromboembolic pulmonary hypertension after pulmonary angioplasty. Eur Respir J. (2020) 56(1):1–11. doi: 10.1183/13993003.01982-2019
30. Howden EJ, Ruiz-Carmona S, Claeys M, De Bosscher R, Willems R, Meyns B, et al. Oxygen pathway limitations in patients with chronic thromboembolic pulmonary hypertension. Circulation. (2021) 143(21):2061–73. doi: 10.1161/CIRCULATIONAHA.120.052899
31. Ewert R, Ittermann T, Schmitt D, Pfeuffer-Jovic E, Stucke J, Tausche K, et al. Prognostic relevance of cardiopulmonary exercise testing for patients with chronic thromboembolic pulmonary hypertension. J Cardiovasc Dev Dis. (2022) 9(10):1–33. doi: 10.3390/jcdd9100333
32. Wensel R, Opitz CF, Anker SD, Winkler J, Hoffken G, Kleber FX, et al. Assessment of survival in patients with primary pulmonary hypertension: importance of cardiopulmonary exercise testing. Circulation. (2002) 106(3):319–24. doi: 10.1161/01.CIR.0000022687.18568.2A
33. Dumitrescu D, Nagel C, Kovacs G, Bollmann T, Halank M, Winkler J, et al. Cardiopulmonary exercise testing for detecting pulmonary arterial hypertension in systemic sclerosis. Heart. (2017) 103(10):774–82. doi: 10.1136/heartjnl-2016-309981
34. Dharmavaram N, Esmaeeli A, Jacobson K, Brailovsky Y, Raza F. Cardiopulmonary exercise testing, rehabilitation, and exercise training in postpulmonary embolism. Interv Cardiol Clin. (2023) 12(3):349–65. doi: 10.1016/j.iccl.2023.02.002
35. Kim MS, Jeon K, Kim EK, Park TK, Park HY, Yang JH, et al. Usefulness of cardiopulmonary exercise test combined with exercise stress echocardiography in mild chronic thromboembolic pulmonary disease. Echocardiography. (2024) 41(3):e15795. doi: 10.1111/echo.15795
36. Boon G, Ende-Verhaar YM, Bavalia R, El Bouazzaoui LH, Delcroix M, Dzikowska-Diduch O, et al. Non-invasive early exclusion of chronic thromboembolic pulmonary hypertension after acute pulmonary embolism: the InShape II study. Thorax. (2021) 76(10):1002–9. doi: 10.1136/thoraxjnl-2020-216324
37. Held M, Grun M, Holl R, Hubner G, Kaiser R, Karl S, et al. Cardiopulmonary exercise testing to detect chronic thromboembolic pulmonary hypertension in patients with normal echocardiography. Respiration. (2014) 87(5):379–87. doi: 10.1159/000358565
38. Zhu H, Sun X, Cao Y, Pudasaini B, Yang W, Liu J, et al. Cardiopulmonary exercise testing and pulmonary function testing for predicting the severity of CTEPH. BMC Pulm Med. (2021) 21(1):324. doi: 10.1186/s12890-021-01668-3
39. Charalampopoulos A, Gibbs JS, Davies RJ, Gin-Sing W, Murphy K, Sheares KK, et al. Exercise physiological responses to drug treatments in chronic thromboembolic pulmonary hypertension. J Appl Physiol (1985). (2016) 121(3):623–8. doi: 10.1152/japplphysiol.00087.2016
40. Shinkura Y, Nakayama K, Yanaka K, Kinutani H, Tamada N, Tsuboi Y, et al. Extensive revascularisation by balloon pulmonary angioplasty for chronic thromboembolic pulmonary hypertension beyond haemodynamic normalisation. EuroIntervention. (2018) 13(17):2060–8. doi: 10.4244/EIJ-D-17-00157
41. Sun X, Shi X, Cao Y, Zhu H, Pudasaini B, Yang W, et al. Variation of PetCO(2) during incremental exercise and severity of IPAH and CTEPH. BMC Pulm Med. (2022) 22(1):249. doi: 10.1186/s12890-022-02045-4
Keywords: pulmonary hypertension, chronic thromboembolic pulmonary disease, chronic thromboembolic pulmonary hypertension, cardiopulmonary exercise testing, pulmonary embolism
Citation: Coulis A, Levanon S, Randhawa G, Brailovsky Y, Raza F and Oliveros E (2024) Cardiopulmonary exercise testing in pulmonary arterial hypertension and chronic thromboembolic pulmonary hypertension. Front. Sports Act. Living 6:1477562. doi: 10.3389/fspor.2024.1477562
Received: 8 August 2024; Accepted: 31 October 2024;
Published: 20 November 2024.
Edited by:
Serafeim - Chrysovalantis Kotoulas, Hippokration General Hospital, GreeceReviewed by:
Mário Santos, University of Porto, PortugalHelen Triantafyllidi, National and Kapodistrian University of Athens, Greece
Xiujun Zhong, Shanghai University of Traditional Chinese Medicine, China
Qin Luo, Chinese Academy of Medical Sciences and Peking Union Medical College, China
Copyright: © 2024 Coulis, Levanon, Randhawa, Brailovsky, Raza and Oliveros. This is an open-access article distributed under the terms of the Creative Commons Attribution License (CC BY). The use, distribution or reproduction in other forums is permitted, provided the original author(s) and the copyright owner(s) are credited and that the original publication in this journal is cited, in accordance with accepted academic practice. No use, distribution or reproduction is permitted which does not comply with these terms.
*Correspondence: Estefania Oliveros, ZXN0ZWZhbmlhLm9saXZlcm9zQHRlbXBsZS5lZHU=