- 1FIFA Clairefontaine Medical Center, French Football Federation, Clairefontaine-en-Yvelines, France
- 2CIAMS Laboratory, Université Paris-Saclay, Orsay, France
- 3CIAMS Laboratory, Université D'Orléans, Orléans, France
- 4French Football Federation Research Centre, Clairefontaine National Football Centre, Clairefontaine-en-Yvelines, France
- 5Laboratory Sport, Expertise and Performance (EA 7370), French Institute of Sport (INSEP), Paris, France
Purpose: This study investigated the evolution of neuromotor control during a typical short sport-specific rehabilitation program (SSR) in professional soccer players who had incurred a major lower-limb injury (n = 15, chondral and muscle injuries, ACL-reconstruction).
Methods: All injured participants (n = 15) were in the on-field rehabilitation phase of their specific sport rehabilitation process, prior to return to play. An experimental group (EG, chondral and muscle injuries, ACL-reconstruction) followed a 3-week SSR-program composed of muscular and core strengthening (weightlifting, functional stability, explosivity and mobility exercises), running and cycling, neuromotor reprogramming, cognitive development and specific soccer on-field rehabilitation (acceleration, braking, cutting, dual-contact, high-speed-running, sprint, jump, drills with ball). Neuromotor control via analysis of movement kinematics, muscle activation and kinetic parameters was evaluated using a single-leg Countermovement-Jump, pre- and post- rehabilitation program. A control group (n = 22) of healthy soccer players of similar standards performed the same single-leg Countermovement-Jump to provide reference values regarding the level to be attained by the injured players for return to play.
Results: In the experimental group, almost all kinetic analyses values progressed during the program and significantly for concentric Rate-of-Force-Development (p < 0.05), height jump (p < 0.001) and Reactive-Strength-Index Modified (p < 0.001) but remained lower than control group values for RSI-Mod (p < 0.05) and RFDconcentricLate (p < 0.001). Activation changed (p < 0.05) for all muscles except for rectus femoris and medial gastrocnemius in the pushing phase and rectus femoris during landing in the EG. Activation of all muscles decreased for EG, except for semitendinous which increased. Regarding kinematic analyses during the landing phase, there were a significant decrease in peak trunk flexion (p < 0.001) and lateroflexion (p < 0.001) and an increase in peak knee flexion (p < 0.001) for both legs. Trunk flexion (p < 0.001) and lateroflexion (p < 0.001) values were again higher for EG while knee flexion remained significantly lower than the CG (p < 0.001).
Conclusion: The SSR generally improved neuromotor control suggesting that the present specific sport rehabilitation program, albeit of only three weeks duration, was effective in aiding elite footballers recover their neuromotor qualities although this was potentially insufficient to return to the values observed in healthy players.
Level of evidence: Therapeutic studies of level II.
Introduction
Contemporary elite soccer is imposing ever-increasing levels of stress on players notably due to increases in the frequency of matches and competitive physical demands (1). While over the last 20-years the incidence of joint and ligament injuries has decreased, hamstring and ligament injury severity has concomitantly increased (2, 3). Injury recurrence rates are high, attaining values of 36% for knee chondral injuries (CH), 17.5% for lower-limb muscle injuries (MI) and 6.6%–10% for anterior cruciate ligament ruptures (4) (ACL). These injuries generate various alterations including arthrogenic muscular inhibition (AMI) (5), pain interference (6), detraining or sensorimotor impairments (7). These can have a strong impact on lower-limb functioning. Indeed, RFD deficits ranging from 10% to 57% have been reported up to 24 months after ACL reconstruction in the injured and non-injured leg (8, 9). For thigh muscle strains, a weakness in eccentric force production has been observed, notably in external muscle range of motion post hamstring injury (10). Moreover, a chondral injury generates inappropriate activation and muscle imbalances leading to impaired dynamic coordination (11).
As such, sport-specific rehabilitation programs (SSR) (12–15) aim to ensure complete restoration of any affected functions and safe and efficient return-to-play phases (RTP) (16). SSR generally include neuromotor training and reprogramming (17–19) (strengthening, postural-work, core-training, mobility, motor-learning, locomotor exercises), physiological energy system conditioning, cognitive work (17) and specific on-field rehabilitation exercises. Buckthorpe et al. (17) suggest that on-field rehabilitation is constructed around 4 pillars: fitness, movement quality, sport-specific skills, and training load. The sport-specific rehabilitation phase can be organized in 5 distinct phases where the intensity, volume, complexity and specificity of the exercises and sessions on the field are progressively increased. One example is the “control-chaos continuum” (CCC) proposed by Taberner (14). The athlete must be able to perform all the movements occurring in their sport (cutting, shifting, jumping, landing, shooting, contact, sprinting, braking, acceleration, processing information and decision-making), all at maximum intensity, repeatedly over time, and with quality movement (18, 20).
To support decision-making during RTP, medical and reconditioning staff frequently utilize information derived from motor evaluations (17, 21, 22) (e.g., hop, landing, isokinetic, agility tests) commonly performed in clinical settings. However, these tests might not be considered discriminating enough to specifically assess any motor deficits that might persist in injured athletes (23) during RTP. As such, RTP assessment batteries frequently including multiple tests have been proposed (22). However, it is not always practically or logistically easy to perform several tests. One test, the Countermovement-Jump (CMJ) is useful as a performance measure (24), a means to evaluate neuromotor control deficits, and also a readiness to play measure (24, 25) while limiting core and limb compensations.
During RTP processes, the between-leg (a)symmetry derived from analyses of neuromotor control is commonly investigated using a “leg symmetry index” (LSI, percentage difference in values for a selected variable between both legs) when performing a locomotor task (20, 26). The LSI-method is used to evaluate neuromotor control impairment and recovery (21, 27) in injured athletes performing lower-limb tests. Recovery is generally considered “complete” if LSI = 100% (20, 26). However, it has been suggested that the LSI overestimates players’ progress in returning to play (28, 29). The utilization of “normative” values is relevant where comparisons of the athlete's current post-injury state can be made with reference values that are both reliable and level-appropriate in cohorts of healthy players (14, 30, 31).
The purpose of this study was to assess the effects of a typical 3-week SSR-program on neuromotor control recovery in the injured and non-injured legs of elite soccer players. Analyses of kinematic, kinetic and EMG (electromyography) variables derived from a single-leg CMJ (SLCMJ) before and after the SSR-program would help determine the variables most impacted during the players' rehabilitation over the 3-week period. The hypothesis forwarded is that the SSR would have a positive impact on all variables, thanks to its comprehensive, functional, and systematic approach whilst bringing the results in both legs of injured players closer to reference values observed in a control group of healthy players.
Materials and methods
Experimental approach to the problem
The effect of a 3-week SSR-program (13–15) on neuromotor control was investigated in injured male professional soccer players. Two groups were formed: an experimental (EG, n = 15) comprised of players with a unilateral lower-limb injury and a control group (CG, n = 22) comprised of uninjured (healthy) players of the same playing standard. All players performed 3-unilateral CMJ using each leg; before and after the SSR-program for the EG and during a single-session for the CG. Metrics included whole-body kinematics, kinetics, and lower-limb muscle activation.
Participants
The cohort included an EG composed of players having sustained a lower-limb injury (Chondropathy n = 4, Muscle Injury n = 4, Anterior Cruciate Ligament-rupture n = 7) and receiving treatment at the Clairefontaine FIFA Medical Center of the French Football Federation, and a CG. The CG (n = 22) included players who had not incurred any significant injury (absence longer than one week) during the six months before the study. All injured participants were in the advanced part of their rehabilitation, the final “on-field rehabilitation” phase, the aim of which, irrespective of the injury, is to regain the ability to meet the demands of competitive practice in all areas. The groups presented similar anthropometric characteristics (Table 1). This study complied with the Declaration of Helsinki (1964) and permission was obtained from French national ethics committee for sports science research (CERSTAPS n°IRB00012476-2020-24-03-48).
Experimental task and protocol
The experiments were performed between 2:00 and 3:30 PM in a training-room, at 20°C. The protocol began with a 10-min warm-up on an ergocycle followed by a progressive increase in power from 100W to 200W. Two maximal isometric voluntary contractions (MVIC) of the leg muscles were then performed for EMG normalization, followed by series of unmeasured SLCMJ trials (three per leg) on a force-plate. These blank trials were performed to ensure familiarization with the experimental task, apparatus, and instructions. Following a one-minute rest period, the two series of SLCMJ were repeated and recorded.
Participants performed barefoot with their hands fixed on their hips. They started in a static position with their stance leg stretched and the contralateral leg slightly flexed with the foot a few centimeters above the force-plate. Participants returned to the same posture following SLCMJ. They were instructed to “jump as high and as quickly as possible and stabilize themselves three-seconds in the final posture”.
Following this first series of tests (pre-SSR), EG participants followed the SSR-program over a 3-week period, which corresponds to a micro-cycle work unit duration within a typical rehabilitation program (13, 14). It also corresponds to the average duration of an injured player's stay at the present Football Medical Center. Post-program, the EG repeated the testing protocol (post-SSR). CG only performed a single test as conducting the same tests twice in healthy top-level footballers is difficult notably due to logistics regarding their training and competition schedules in addition to the effect of the associated loading. In addition, some of the players were no longer able to perform the test battery as they no longer met the inclusion criteria on being injury free. A pilot study in the twenty-two CG subjects showed that the raw experimental variables did not differ significantly when SLCMJ was performed on their dominant or non-dominant leg (p > 0.05). As such, only the dominant leg was tested in the CG.
SSR-program
The SSR-program (13, 14, 32) was composed of muscular strengthening (weightlifting and functional exercises), physiological energy system conditioning running and cycling, neuromotor reprogramming and specific soccer on-field rehabilitation (acceleration, braking, cutting, dual-contact, high-speed-running, sprint, jump, drills with ball) on the pitch, core-training, mobility and cognitive development (2, 20, 32–36). EG players performed the program approximately 5-h per day, 5-days a week, during 3-weeks consecutively. On each day of the on pitch SSR-program, players performed mobility, specific lower-limb activation, neuromotor control and specific soccer rehabilitation in the morning and lower-limb strengthening, core-training and specific care in the afternoon. The soccer-specific rehabilitation part included general and specific drills and soccer movement (accelerations, decelerations, cutting, jump, landing, dribble, shift), high-speed-running and sprinting, short and long passes, duels and physical contact work, and cognitive work (information analysis and decision making). A progressive augmentation in the volume, intensity and complexity of the content of the pitch sessions was implemented over the 3weeks following previous recommendations for on-field rehabilitation (12, 20, 32–34, 36, 37). The external and internal workloads and intensities were monitored and adapted in relation to progression, according to the characteristics of the injury and the individual's response to the programme. This was done to respond as effectively as possible to the inter-individual differences in adaptation and recovery times. The exercises were ceased if the player deemed the pain was greater than 3/10 using a numerical rating scale (38). The decision criteria for changing training focus depended upon the progress made during strength training assessments and GPS tracking data. The program was monitored by a certified physical trainer specialized in rehabilitation and a team of sport physiotherapists working under the responsibility of three medical doctors specialized in sport rehabilitation. A weekly planning of the SSR program is available in the appendixes.
Data recordings
SLCMJ movement was analyzed though kinetic, kinematic and EMG data recording (Figure 1). Kinetic data was obtained using a force-plate (9260AA6 Kistler Instruments, Hampshire, UK) that provided ground reaction force (GRFZ), and moments applied at its surface. Kinematic data for knee flexion (KF), trunk flexion (TF) and lateroflexion (TLF) of the stance leg was obtained using the Humantrak system (Vald Performance, Brisbane, Australia) with a Kinect-v2 camera (Microsoft Corp., Redmond, WA, USA). Kinematic positional data was processed through a dual Butterworth filter to remove residual noise. Electrical activity of lower-limb muscles was recorded with 12-channel Delsys Trigno (39) wireless surface Ag/AgCl sensors (27 mm × 35 mm, Trinoma, Lyon, France): vastus medialis (VM), rectus femoris (RF), biceps femoris (BF), semitendinous (ST), gluteus medius (GM) and medial gastrocnemius (MG). SENIAM (40) recommendations were applied for sensors location. EMG signals were filtered by a 10-Hz bandpass filter (41) and by a Butterworth filter in EMGworks 4.4 software (Delsys, Inc.) via Root Mean Square. All recordings were sampled at 1,000 Hz.
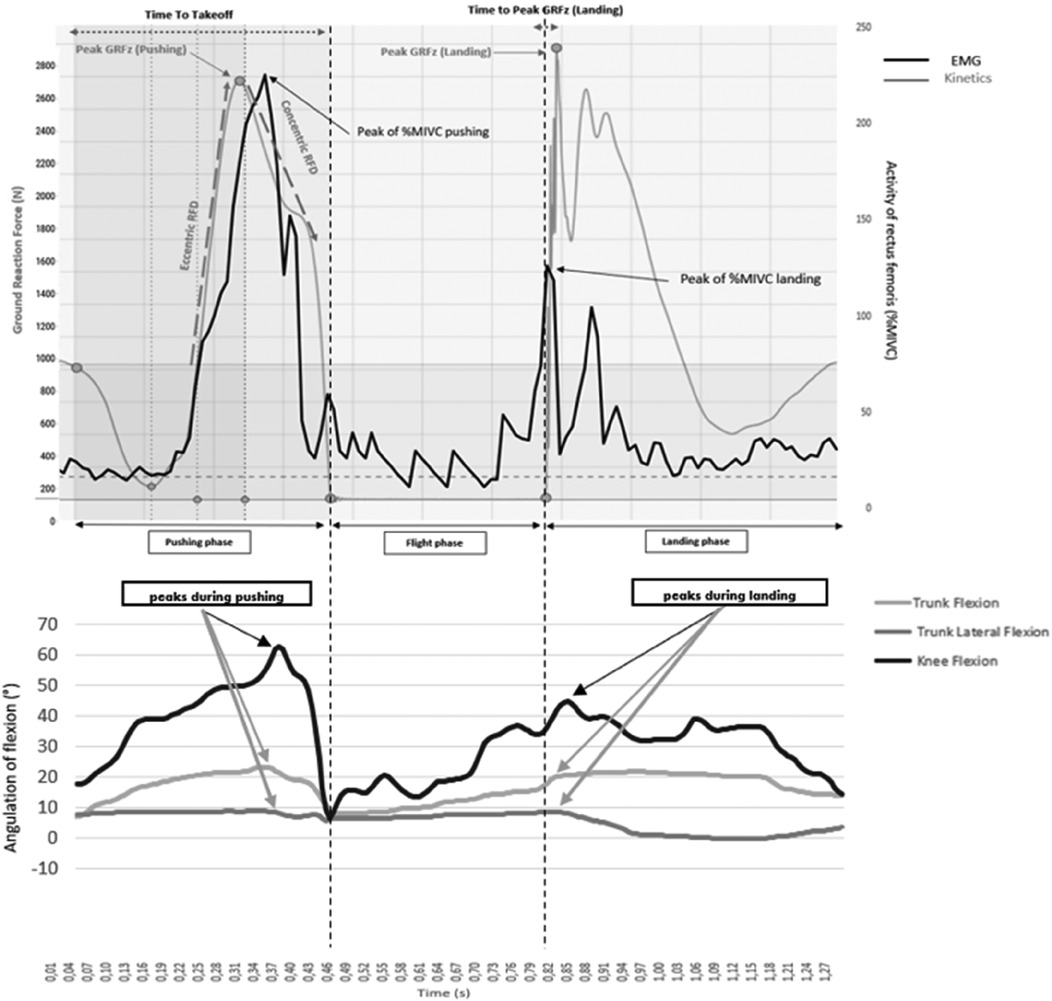
Figure 1. Example of electromyogram, kinematics and kinetics curve of a control group athlete during a single-leg countermovement jump on a strength platform. For the EMG and kinetic curves, a drop occurs in the curve corresponding to the countermovement (the unloading of athlete's weight), then the curves rise during the athlete's reloading phase (end of the eccentric) to the top of the curves. The curves then drop again (concentric thrust phase), then there is a slight plateau (with zero values for the kinetic) corresponding to the flight time, then finally the curves rise again during the jump landing phase before plateauing again.
EMG normalization
The electrical activity of each muscle obtained during propulsion and landing phases of SLCMJ series was normalized with respect to maximal voluntary isometric contraction (MVIC) (41). EMG activity during MVIC was evaluated by two successive MVIC for 5-s separated by a 30-s rest interval for each muscle studied in both legs, before each test session. The highest maximal averaged value obtained on the sliding 0.5-s periods (the highest average recorded over a period of 0.5 during the 5-s isometric contraction) was considered MVIC (41). This was assessed during specific analytical exercises carried out on a guide machine, with the targeted muscle contracting against an over-maximal resistance. MVIC was evaluated with the leg extended at 45° knee flexion for the vastus medialis and rectus femoris and for the leg curl at 45° knee flexion for the biceps femoris and semitendinous using a fixed pulley at 25° of abduction for the gluteus medius and on the calf press with the leg extended and neutral ankle position for the medial gastrocnemius (40).
Raw experimental variables
The following spatio-temporal and kinetics variables were obtained from the force-plate (Figure 1):
- Peaks of upward vertical ground reaction force (peak vGRF, in Newton) produced during the pushing phase and during the landing phase of the single-leg-CMJ (24). The landing phase corresponds to the dynamic phase after the flight phase.
- Jump height (in cm) represents the maximal altitude attained by the athlete during the single-leg-CMJ, estimated by the force-plate software through flight duration. Values are a functional measure of the athlete's neuromotor performance (24).
- The Reactive Strength Index Modified (RSI-mod, in m/s) is the ratio of jump height to time to take-off (countermovement duration). This metric reflects lower-limb explosiveness (24).
- Rate-of-Force Development (RFD, in N/s) during both the eccentric (RFDeccentric) and concentric (RFDconcentric) phases of the pushing motion (see Figure 1). RFDeccentric is determined by the slope of the line between the return to the athlete's body weight while ascending the ground reaction force (GRF) and the first upward peak of the vertical GRF trace. RFDconcentric is defined as the slope of the line from this first upward peak to take-off time (23). These parameters reflect lower-limb explosiveness.
- The vertical ground reaction force value at t = 50 ms after foot landing (vGRF at 50 ms landing, in Newtons). This moment is known to coincide with the peak risk of knee injury (42, 43).
- Time to peak vertical ground reaction force during the landing phase (in ms), indicating the duration between foot landing and the peak of the vertical ground reaction force.
The EMG parameters encompassed both the peak and mean values of electrical activity in the leg muscles, expressed as a percentage of the activity observed during maximal isometric voluntary contraction, throughout both phases of the single-leg-CMJ (see Figure 1).
Kinematic variables included peak knee flexion, peak trunk flexion, and peak trunk lateroflexion angles (in degrees) recorded during both phases of the single-leg-CMJ (see Figure 1) (7, 44).
Statistics
Group means and standard deviations were computed for VARIL, VARNIL and VARCT raw variables in pre- and post-SSR. The Shapiro-Wilk test was used to check the normality of the data distribution. To assess the neuromotor capacity of IL and NIL, repeated measures (RM) ANOVAs included the method (3-levels: VARIL, VARNIL vs. VARCT) and SSR (2 levels: pre-SSR vs. post-SSR) as within subject factors were used on each VARIL, VARNIL and VARCT. A significant outcome was followed by the Tukey post hoc test to assess pairwise statistical differences between methods and both SSR conditions. A student T-test was used to compare anthropometric data between the two experimental groups. Kinematic values remain expressed in degrees (°) as values can be positive or negative, so percentage methods were not relevant in this context. The significance threshold was set at p < 0.05. Cohen's d was used to determine the effect sizes for differences in mean values (classified as trivial: <0.2, small: 0.2–0.49, medium: 0.5–0.79, and large: ≥0.8).
Results
Comparison of anthropometric characteristics between groups
No significant differences were observed in inter-group characteristics (p > 0.05).
Impact of the SSR-program on IL and NIL according to the estimation method chosen
Kinetic analysis
Results reported no differences for all RFDeccentric and peak vGRF pushing phase values (p > 0.05) for both legs. In contrast, a significant impact of the SSR was observed for RFDconcentric (F = 7.8, p < 0.01), RFDconcentricEarly (F = 4.4, p < 0.05), RFDconcentricLate (F = 5.9, p < 0.05), RSI-Mod (F = 12.4, p < 0.001), jump height (F = 13.6, p < 0.001), time to peak of vGRF landing (F = 4.0, p < 0.05) and vGRF at 50 ms of landing (F = 8.7, p < 0.01). Post hoc tests revealed that the SSR-program increased RSI-Mod in IL (p < 0.01, d = 1.1), jump height in IL (p < 0.001, d = 0.8) and vGRF at 50 ms during landing in NIL (p < 0.05, d = 0.6).
There were a significant group*SSR interaction between IL, NIL and CT for jump height (F = 5.4, p < 0.01) and RSI-Mod (F = 4.4, p < 0.05). Post hoc tests revealed that post-SSR, there was no significant difference between IL, NIL and CT, except for the RSI-Mod and RFDconcentricLate where IL was lower than CT (p < 0.05, d = 0.6 and p < 0.001, d = 0.8), underlining progression in both legs towards the values observed in the healthy players (see Figure 2).
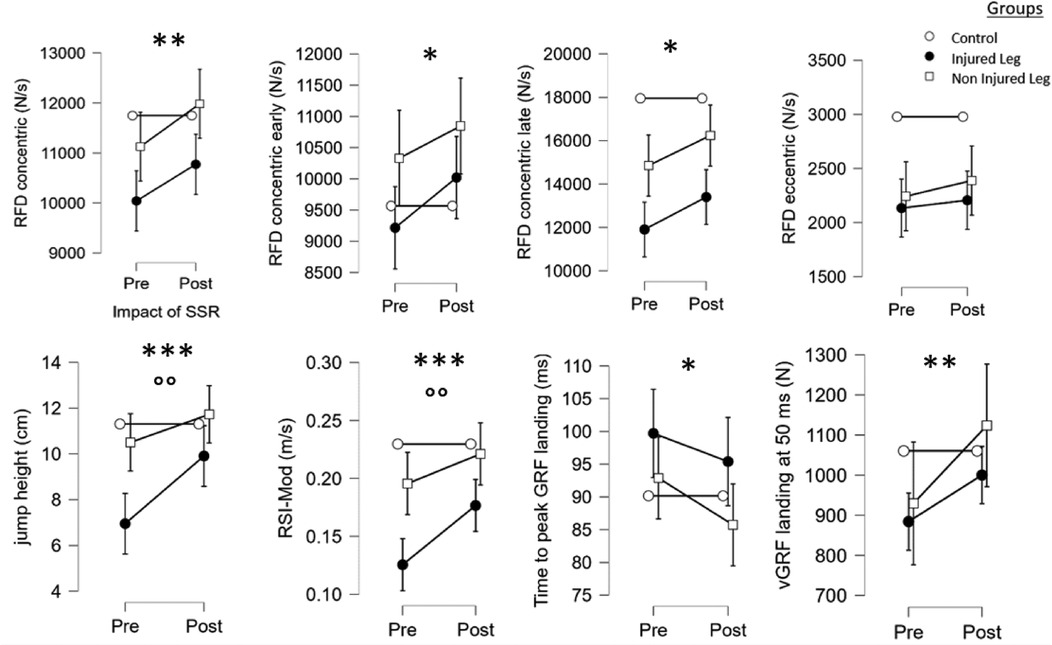
Figure 2. Main effects of the SSR program and the group on the kinetics variables of the single-leg-CMJ. *, **, ***: significant effect of the SSR program (pre vs. post-SSR) with p < 0.05, p < 0.01 and p < 0.001, respectively. °, °°,°°°: significant effect of the group with p < 0.05, p < 0.01 and p < 0.001, respectively.
EMG analysis
Results showed that the SSR-program led to significant differences for%MIVC mean of semitendinous (F = 15.9, p < 0.001), %MIVC max of vastus medialis (F = 6.0, p < 0.05), rectus femoris (F = 5.7, p < 0.05) and gluteus medius (F = 7.6, p < 0.01) muscles during pushing. Some significant differences were observed for%MIVC mean of biceps femoris (F = 6.3, p < 0.05), medial gastrocnemius (F = 7.6, p < 0.01) and for%MIVC max of vastus medialis (F = 6.6, p < 0.05), biceps femoris (F = 5.8, p < 0.05), semitendinous (F = 23.6, p < 0.001), gluteus medius (F = 11.2, p < 0.001) and medial gastrocnemius (F = 9.5, p < 0.01) muscles during landing. Post hoc tests revealed that the SSR-program led to an increase in pushing phase mean%MIVC for the semitendinous in the IL (p < 0.05, d = 0.5) and NIL (p < 0.01, d = 0.6), in%MIVC max for the semitendinous in the IL (p < 0.01, d = 0.7) during landing. Decreased max%MIVC of the gluteus medius for the NIL (p < 0.01, d = 0.6) and of medial gastrocnemius for NIL (p < 0.001, d = 0.7) were observed during landing.
There was a significant group*SSR interaction for%MIVC mean of semitendinous during pushing (F = 4.5, p < 0.01), for%MIVC max of gluteus medius (F = 4.4, p < 0.05) and medial gastrocnemius (F = 5.5, p < 0.05) during landing (see Figure 3).
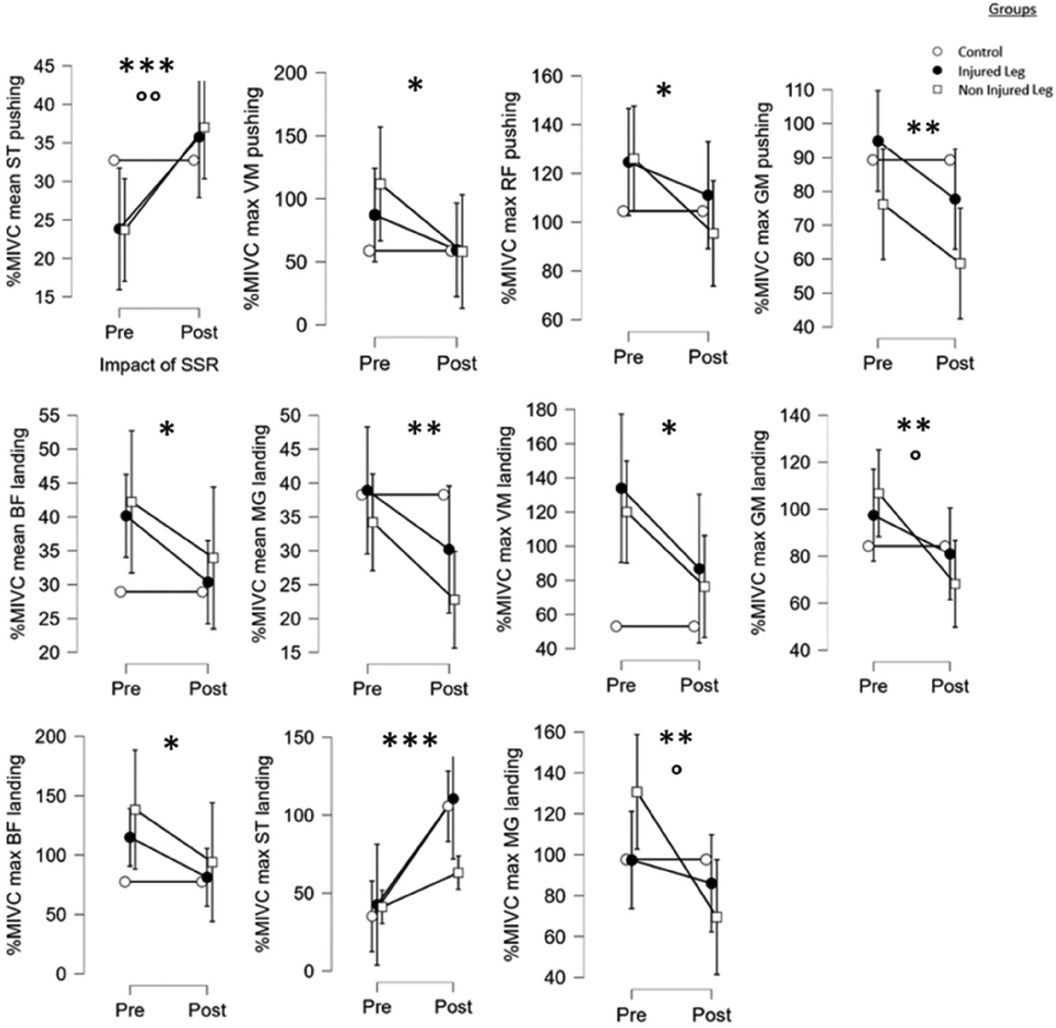
Figure 3. Main effects of the SSR program and the group on the EMG variables of the single-leg-CMJ. *, **, ***: significant effect of the SSR program (pre vs. post-SSR) with p < 0.05, p < 0.01 and p < 0.001, respectively. °, °°, °°°: significant effect of the group with p < 0.05, p < 0.01 and p < 0.001, respectively.
Kinematic analysis
Results showed that SSR-program led to significant differences in active knee flexion at push (F = 5,4, p < 0.05,), at landing (F = 26,4, p < 0.001); and in trunk flexion (F = 50,7, p < 0.001) and trunk lateroflexion (F = 405,9, p < 0.001) during the landing phase. Post hoc tests revealed that the SSR-program led to a decrease in knee flexion of NIL at push (p < 0.001, d = 0.5), a knee flexion of NIL increase at landing (p < 0.001, d = 1.0) and a decrease in IL and NIL values, respectively for trunk flexion (p < 0.001, d = 3.2 and p < 0.001, d = 2.5) and trunk lateroflexion of IL (p < 0.001, d = 1.1) during the landing phase.
Finally, there were a significant group*SSR interaction between IL, NIL and CT for knee flexion (F = 9.5, p < 0.001), trunk flexion (F = 16.3, p < 0.001) and trunk lateral flexion (F = 123.3, p < 0.001) during landing (see Figure 4).
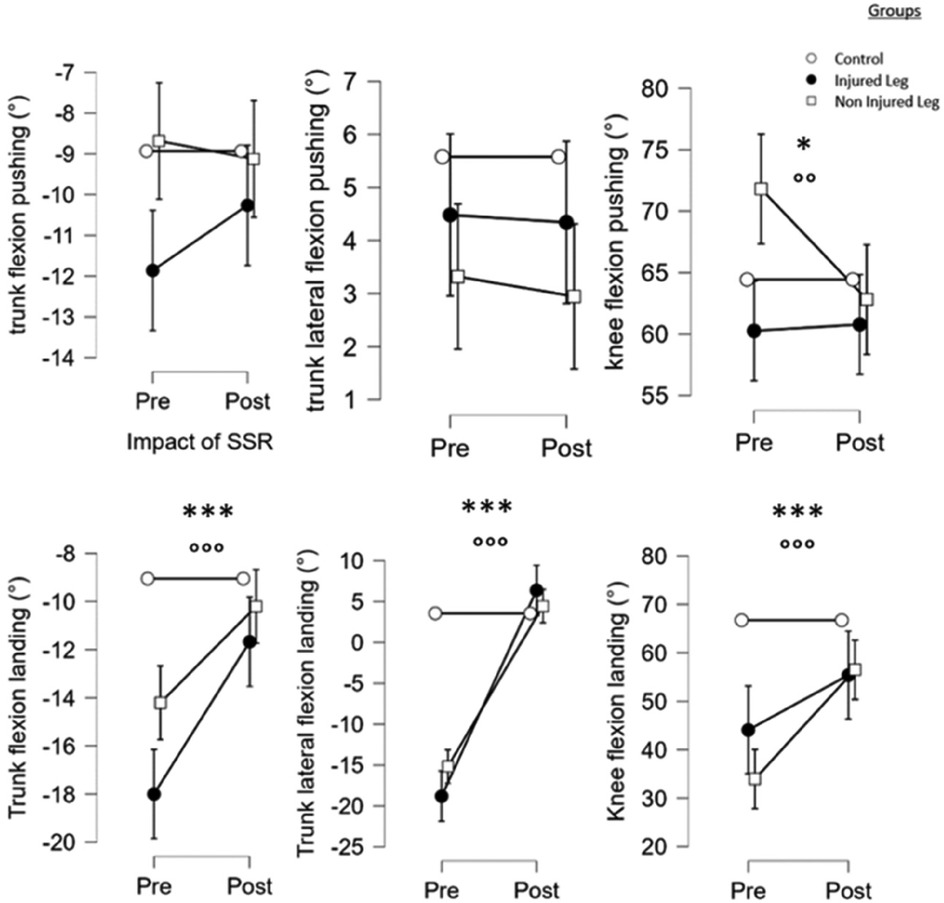
Figure 4. Main effects of the SSR program and the group on the kinematics variables of the single-leg-CMJ. *, **, ***: significant effect of the SSR program (pre vs. post-SSR) with p < 0.05, p < 0.01 and p < 0.001, respectively. °, °°, °°°: significant effect of the group with p < 0.05, p < 0.01 and p < 0.001, respectively.
Discussion
Impact of the SSR-program
The present study firstly assessed the effectiveness of a typical 3-week SSR-program on neuromotor control for the injured leg (IL) in a group of professional soccer players. The SSR-program positively impacted several neuromotor parameters for both the IL and non-injured leg (NIL). Similar results have previously been observed related to the impact of neuromuscular training but with an eccentric dominance during muscle-strengthening program (35). Here, strength conditioning and neuromotor control work coupled with high-intensity and soccer-specific movements generated significant gains in RFD, despite the program's short duration (3-weeks). Improvement in neural aspects in the RFDEarly phase including muscle activation, reduction of recruitment threshold and increase in the rate of motor-unit discharge or a facilitation of spinal and supraspinal outputs can potentially explain this gain (8, 9, 45–47). Structural contractile factors are preponderant for the RFDLate phase (8, 9, 45–47). These include muscle fiber architecture, composition, and strength level. Concentric gains are achieved more quickly because the coordination, muscle recruitment and neural adaptations specific to this contraction regime are less complex (35). In contrast, no progress was observed in the injured players for RFDEccentricLate. To our knowledge, this variable has not yet received any attention in the literature. Nevertheless, we can hypothesize that a 3-week period is potentially insufficient to establish nerve force adaptations unlike structural ones (21, 35). Other possible explanations include a lack of quality eccentric explosiveness based on muscular pre-activation and pre-synaptic facilitation (48, 49); or self-confidence to descend quickly during a post-injury ballistic movement. As such, players must continue neuromotor training to improve inter and intramuscular coordination and optimize this eccentric-concentric transition period (48–50).
Regarding muscle activation, the semitendinous was the muscle most positively impacted by the SSR, with a significant increase observed. In relation to the associated literature, one would expect a significant increase in muscle activation following neuromotor training (45, 51, 52). This is linked to improvements in corticomotor excitability, motor-unit synchronization and a reduction in inhibitory processes, and even after only 1-week of targeted work (52). To generate the same force, an eccentric contraction requires less muscle activation than a concentric one (53). An increase in discharge frequency and recruitment of slow motor-units to distribute the mechanical stress applied to the muscle can be responsible for stimulating EMG activity (53). These observations were observed only for the semitendinous and rectus femoris (not significatively) in IL and may partly be explained by the general rehabilitation program performed pre-SSR (11–13, 19). Indeed, this enabled the players to resume running and perform one-leg jumps without pain as nerve adaptations related to activation had certainly already been stimulated. Hamstrings and quadriceps are preferential targets of central and peripheral inhibitions (AMI) (5) and pain adaptation (6) potentially explaining this evolution. Research has previously discussed the compensation capacity of the BF when ST is inhibited (54). Here, SSR enabled a higher activation of the semitendinous avoiding overactivation of the BF and can therefore be partly responsible for any stagnation. RF and MG did not change with this result possibly explained by the quadriceps being targeted at an early stage during rehabilitation in order to eliminate any possible inhibition (5), and the athletes use better coordination reducing the compensations by the ankle and therefore the MG. Research, albeit limited (55), has also shown a lack of change in muscle activation after a neuromotor program and no correlation between jump height and muscle activity (20).
Regarding kinematic data, analysis of SLCMJ demonstrated noteworthy results. IL and NIL exhibited higher trunk flexion during pushing and landing probably to compensate for a lack of knee flexion, but these were less important post-SSR. When excessive trunk lateroflexion is observed, a deficit in pelvic control with a gluteus medius deficit in functionality is highlighted (56). These improvements show that the SSR-program had a positive impact on kinematic compensations linked to the injuries and particularly improved the neuromotor function of both the IL and NIL. This is possibly due to progress in activation qualities, muscle strength and confidence in one's leg movement. Indeed, poor neuromotor control of the trunk impacts dynamic knee stability, resulting in increased abduction and tension on the ligaments and joint (16, 56). The present SSR-program increased knee flexion at landing to dampen the movement, and knee flexion at push were very similar for IL, NIL and CG after SSR, demonstrating the effectiveness of the SSR-program effects on knee functionality. Knee flexion, knee valgus and hip abduction have previously been studied (41, 48) and reported the same observations as here (16, 56). The reduction in TLF may be due to a better utilization of knee flexion (linked to activation, strength and RFD), limiting distal instability and therefore compensation with the trunk. This improvement in trunk control is essential as postural stabilization deficit of the trunk is a key risk factor for joint and muscle injury (16, 56). Increasing knee flexion and trunk flexion during jump and landing actions would reduce GRFZ levels and therefore the stress on body structures to limit injury risk (56, 57). Dynamic postural deficits have been observed up to 9–12 months following ACL-reconstruction highlighting that there are still postural compensations that have not yet been normalized during rehabilitation, as reported in our results (8, 9). As such, the SSR-program seems to be efficient for ensuring intrinsic functionality recovery of the knee. The kinematic analyses underline compensations, orientate rehabilitation and prophylactic work, and evaluate players' capacity to return to play using qualitative control of movements (14, 56–58). Different characteristics related to neuromotor control were observed depending on the injuries studied. These are currently under investigation in a sister paper (entitled, Modeling the neuromotor capacities of professional soccer player with a lower-limb injury during a Countermovement Jump, submitted).
A recent review on return-to-play deplores that this process is too often based on subjective data and lacks objectivity, normalization, standardization and scientific consensus (59). To validate any RTP process, specialized literature including the Italian Consensus Conference recommend either no LSI deficit or values less than 10% and return-to-performance levels prior to injury (9, 14, 60–62). Our pre-SSR result of deficits in RFDconcentric (32%), jump height (8%) and RSI-Mod (17%) highlights that the NIL is also impacted (9) by injury to the contralateral leg and therefore also requires specific care and reconditioning during rehabilitation. This deconditioning of the NIL is consistent with the rate of reinjury reported in the contralateral leg over the two years following an initial ACLR injury (63). Moreover, if the NIL has not undergone specific conditioning training, RFD deficits of LSI (10%–57%) have been reported up to 24 months after surgery (8, 9) in the IL and NIL, which is outside the acceptability threshold for any RTP process. Once again comparison with healthy players seems to be of prime importance in guiding the rehabilitation staff on the program to be implemented for injured players. However, when this NIL training is performed, NIL values are closer to healthy players' performance, as our results showed with a reduction observed for deficits in RFDconcentric, jump height and RSI-Mod respectively of 24%, 0% and 4% also underlining the efficacity of the SSR-program on NIL.
This study has two main limitations. First, the cohort size which can be explained by limited access to elite standard participants. Secondly, the GE was made up of players with different types of injury (ACLR, muscle strain and chondral injury) who have different rehabilitation durations and severities of neuromuscular alteration, which may complicate analysis of the impact of the present SSR. Three weeks is a “normal” duration for the SSR of muscle strains, whereas according to the literature, ACL and CH require a longer period of SSR.
Conclusion
The main objective of any RTP process is to monitor and aid the athlete's return from injury and ultimately help them respond to the specific demands of competition (14, 55). SLCMJ tests during the RTP process are pertinent, useful and discriminating in the evaluation of neuromotor control (14). A SSR-program, even of 3-weeks duration, was effective in recovering neuromotor qualities in a group of high-level footballers although progress in their return to play programme seemed insufficient to attain the same level as healthy players. Moreover, results also showed a positive impact of the SSR-program on the players' NIL, underlying that it is essential to develop the capacities of the both the IL and NIL, to help avoid deficits on return to play with the NIL. Monitoring the progress of athletes during a SSR is important to refine the program and RTP strategies to minimize the risk of injury recurrence (20, 22, 64). Despite this, pending a scientific and clinical consensus on the objectification of SRR and the RTP processes, the impact of sport-specific rehabilitation program arguably depends mainly on the quality of the physical trainer in charge (59).
Data availability statement
The raw data supporting the conclusions of this article will be made available by the authors, without undue reservation.
Ethics statement
The studies involving humans were approved by national Ethics Committee for sports science research (CERSTAPS n°IRB00012476-2020-24-03-48. The studies were conducted in accordance with the local legislation and institutional requirements. The participants provided their written informed consent to participate in this study.
Author contributions
GM: Conceptualization, Data curation, Formal Analysis, Investigation, Methodology, Project administration, Resources, Supervision, Validation, Visualization, Writing – original draft, Writing – review & editing. CC: Formal Analysis, Methodology, Supervision, Validation, Visualization, Writing – review & editing. JB: Conceptualization, Investigation, Methodology, Resources, Validation, Writing – original draft. PM: Investigation, Project administration, Resources, Supervision, Visualization, Writing – review & editing. BT: Conceptualization, Methodology, Validation, Writing – review & editing. PF: Conceptualization, Data curation, Methodology, Writing – review & editing. EY: Conceptualization, Data curation, Formal Analysis, Methodology, Validation, Writing – review & editing.
Funding
The author(s) declare financial support was received for the research, authorship, and/or publication of this article. FIFA Medical Center of Clairefontaine.
Conflict of interest
The authors declare that the research was conducted in the absence of any commercial or financial relationships that could be construed as a potential conflict of interest.
Publisher's note
All claims expressed in this article are solely those of the authors and do not necessarily represent those of their affiliated organizations, or those of the publisher, the editors and the reviewers. Any product that may be evaluated in this article, or claim that may be made by its manufacturer, is not guaranteed or endorsed by the publisher.
References
1. Hostrup M, Bangsbo J. Performance adaptations to intensified training in top-level football. Sports Med. (2023) 53(3):577–94. doi: 10.1007/s40279-022-01791-z
2. Ekstrand J, Bengtsson H, Waldén M, Davison M, Khan KM, Hägglund M. Hamstring injury rates have increased during recent seasons and now constitute 24% of all injuries in men’s professional football: the UEFA elite club injury study from 2001/02 to 2021/22. Br J Sports Med. (2023) 57(5):292–8. doi: 10.1136/bjsports-2021-105407
3. Ekstrand J, Waldén M, Hägglund M. Hamstring injuries have increased by 4% annually in men’s professional football, since 2001: a 13-year longitudinal analysis of the UEFA elite club injury study. Br J Sports Med. (2016) 50(12):731–7. doi: 10.1136/bjsports-2015-095359
4. Sonnery-Cottet B, Saithna A, Cavalier M, Kajetanek C, Temponi EF, Daggett M, et al. Anterolateral ligament reconstruction is associated with significantly reduced ACL graft rupture rates at a Minimum follow-up of 2 years: a prospective comparative study of 502 patients from the SANTI study group. Am J Sports Med. (2017) 45(7):1547–57. doi: 10.1177/0363546516686057
5. Sonnery-Cottet B, Hopper GP, Gousopoulos L, Vieira TD, Thaunat M, Fayard JM, et al. Arthrogenic muscle inhibition following knee injury or surgery: pathophysiology, classification, and treatment. Video J Sports Med. (2022) 2(3):263502542210862. doi: 10.1177/26350254221086295
6. Hodges PW. Pain and motor control: from the laboratory to rehabilitation. J Electromyogr Kinesiol. (2011) 21(2):220–8. doi: 10.1016/j.jelekin.2011.01.002
7. Forelli F, Mazeas J, Linard M. Integration of postural alterations in the assessment and neuromotor rehabilitation after anterior cruciate ligament surgery A narrative review. J Orthop Sports Med. (2020) 02(01):29–34. doi: 10.26502/josm.511500019
8. Knezevic OM, Mirkov DM, Kadija M, Nedeljkovic A, Jaric S. Asymmetries in explosive strength following anterior cruciate ligament reconstruction. Knee. (2014) 21(6):1039–45. doi: 10.1016/j.knee.2014.07.021
9. Mirkov DM, Knezevic OM, Maffiuletti NA, Kadija M, Nedeljkovic A, Jaric S. Contralateral limb deficit after ACL-reconstruction: an analysis of early and late phase of rate of force development. J Sports Sci. (2017) 35(5):435–40. doi: 10.1080/02640414.2016.1168933
10. Fyfe JJ, Opar DA, Williams MD, Shield AJ. The role of neuromuscular inhibition in hamstring strain injury recurrence. J Electromyogr Kinesiol. (2013) 23(3):523–30. doi: 10.1016/j.jelekin.2012.12.006
11. Tayfur B, Charuphongsa C, Morrissey D, Miller SC. Neuromuscular joint function in knee osteoarthritis: a systematic review and meta-analysis. Ann Phys Rehabil Med. (2023) 66(2):101662. doi: 10.1016/j.rehab.2022.101662
12. Abdelatif H, Abdelhadi K, Nasredine G, Abdelkader Z, Fouad C, Mohamed Z. Effects of a reathletization protocol after ACL ligamentoplasty on performance of Algerian professional soccer player: a case study. Слобожанський Науково-Спортивний Вісник. (2022) 3(26):75–82.
13. Gaspar M. Intérêt de l’électromyographie de surface (EMG-S) dans la rééducation du sportif blessé, partie 1: installation, application, analyse et interprétation. Kinésithér Scient. (2021) 06(33):25–33.
14. Taberner M, Allen T, Cohen DD. Progressing rehabilitation after injury: consider the “control-chaos continuum”. Br J Sports Med. (2019) 53(18):1132–6. doi: 10.1136/bjsports-2018-100157
15. Della Villa S, Boldrini L, Ricci M, Danelon F, Snyder-Mackler L, Nanni G, et al. Clinical outcomes and return-to-sports participation of 50 soccer players after anterior cruciate ligament reconstruction through a sport-specific rehabilitation protocol. Sports Health. (2012) 4(1):17–24. doi: 10.1177/1941738111417564
16. Rambaud AJ, Neri T, Dingenen B, Parker D, Servien E, Gokeler A, et al. The modifying factors that help improve anterior cruciate ligament reconstruction rehabilitation: a narrative review. Ann Phys Rehabil Med. (2022) 65(4):101601. doi: 10.1016/j.rehab.2021.101601
17. Buckthorpe M. Optimising the late-stage rehabilitation and return-to-sport training and testing process after ACL reconstruction. Sports Med. (2019) 49(7):1043–58. doi: 10.1007/s40279-019-01102-z
18. Gokeler A, Neuhaus D, Benjaminse A, Grooms DR, Baumeister J. Principles of motor learning to support neuroplasticity after ACL injury: implications for optimizing performance and reducing risk of second ACL injury. Sports Med. (2019) 49(6):853–65. doi: 10.1007/s40279-019-01058-0
19. Grooms D, Appelbaum G, Onate J. Neuroplasticity following anterior cruciate ligament injury: a framework for visual-motor training approaches in rehabilitation. J Orthop Sports Phys Ther. (2015) 45(5):381–93. doi: 10.2519/jospt.2015.5549
20. Forelli F, Le Coroller N, Gaspar M, Memain G, Kakavas G, Miraglia N, et al. Ecological and specific evidence-based safe return to play after anterior cruciate ligament reconstruction in soccer players: a new international paradigm. Int J Sports Phys Ther. (2023) 18(2):526–40. doi: 10.26603/001c.73031
21. Gokeler A, Grassi A, Hoogeslag R, Van Houten A, Lehman T, Bolling C, et al. Return to sports after ACL injury 5 years from now: 10 things we must do. J Exp Orthop. (2022) 9(1):73. doi: 10.1186/s40634-022-00514-7
22. Gokeler A, Welling W, Zaffagnini S, Seil R, Padua D. Development of a test battery to enhance safe return to sports after anterior cruciate ligament reconstruction. Knee Surg Sports Traumatol Arthrosc. (2017) 25(1):192–9. doi: 10.1007/s00167-016-4246-3
23. Kotsifaki R, Sideris V, King E, Bahr R, Whiteley R. Performance and symmetry measures during vertical jump testing at return to sport after ACL reconstruction. Br J Sports Med. (2023) 57(20):1304–10. doi: 10.1136/bjsports-2022-106588
24. Bishop C, Turner A, Jordan M, Harry J, Loturco I, Lake J, et al. A framework to guide practitioners for selecting metrics during the countermovement and drop jump tests. Strength Cond J. (2022) 44(4):95–103. doi: 10.1519/SSC.0000000000000677
25. McMahon JJ, Suchomel TJ, Lake JP, Comfort P. Understanding the key phases of the countermovement jump force-time curve. Strength Cond J. (2018) 40(4):96–106. doi: 10.1519/SSC.0000000000000375
26. Flagg KY, Karavatas SG, Thompson S Jr, Bennett C. Current criteria for return to play after anterior cruciate ligament reconstruction: an evidence-based literature review. Ann Transl Med. (2019) 7(S7):S252. doi: 10.21037/atm.2019.08.23
27. ŞiMşek D, Kirkaya İ, Onarici Güngör E, Soylu AR. Relationships among vertical jumping performance. EMG Activation, and Knee Extensor and Flexor Muscle Strength in Turkish Elite Male Volleyball Players. Turk Klin J Sports Sci. (2016) 8(2):46–56.
28. Wellsandt E, Failla MJ, Snyder-Mackler L. Limb symmetry indexes can overestimate knee function after anterior cruciate ligament injury. J Orthop Sports Phys Ther. (2017) 47(5):334–8. doi: 10.2519/jospt.2017.7285
29. Moran TE, Ignozzi AJ, Burnett Z, Bodkin S, Hart JM, Werner BC. Deficits in contralateral limb strength can overestimate limb symmetry Index after anterior cruciate ligament reconstruction. Arthrosc Sports Med Rehabil. (2022) 4(5):e1713–9. doi: 10.1016/j.asmr.2022.06.018
30. Wilk KE, Thomas ZM, Mangine RE, Fuller P, Davies GJ. Neurocognitive and reactive return to play testing protocol in overhead athletes following upper extremity injury. Int J Sports Phys Ther. (2023) 18(6):1364–75.38050554
31. Powell C, Jensen J, Johnson S. Functional performance measures used for return-to-sport criteria in youth following lower-extremity injury. J Sport Rehabil. (2018) 27(6):581–90. doi: 10.1123/jsr.2017-0061
32. Kotsifaki R, Korakakis V, King E, Barbosa O, Maree D, Pantouveris M, et al. Aspetar clinical practice guideline on rehabilitation after anterior cruciate ligament reconstruction. Br J Sports Med. (2023) 57(9):500–14. doi: 10.1136/bjsports-2022-106158
33. Buckthorpe M, Della Villa F, Della Villa S, Roi GS. On-field rehabilitation part 1: 4 pillars of high-quality on-field rehabilitation are restoring movement quality, physical conditioning, restoring sport-specific skills, and progressively developing chronic training load. J Orthop Sports Phys Ther. (2019) 49(8):565–9. doi: 10.2519/jospt.2019.8954
34. Buckthorpe M, Della Villa F, Della Villa S, Roi GS. On-field rehabilitation part 2: a 5-stage program for the soccer player focused on linear movements, multidirectional movements, soccer-specific skills, soccer-specific movements, and modified practice. J Orthop Sports Phys Ther. (2019) 49(8):570–5. doi: 10.2519/jospt.2019.8952
35. Duchateau J, Semmler JG, Enoka RM. Training adaptations in the behavior of human motor units. J Appl Physiol. (2006) 101(6):1766–75. doi: 10.1152/japplphysiol.00543.2006
36. Forelli F, Riera J, Marine P, Gaspar M, Memain G, Miraglia N, et al. Implementing velocity-based training to optimize return to sprint after anterior cruciate ligament reconstruction in soccer players: a clinical commentary. Int J Sports Phys Ther. (2024) 19(3):355–65. doi: 10.26603/001c.92704
37. Taberner M, Van Dyk N, Allen T, Jain N, Richter C, Drust B, et al. Physical preparation and return to performance of an elite female football player following ACL reconstruction: a journey to the FIFA women’s world cup. BMJ Open Sport Exerc Med. (2020) 6(1):e000843.34422284
38. Opar DA, Williams MD, Timmins RG, Hickey J, Duhig SJ, Shield AJ. Eccentric hamstring strength and hamstring injury risk in Australian footballers. Med Sci Sports Exerc. (2015) 47(4):857–65. doi: 10.1249/MSS.0000000000000465
39. Poitras I, Bielmann M, Campeau-Lecours A, Mercier C, Bouyer LJ, Roy JS. Validity of wearable sensors at the shoulder joint: combining wireless electromyography sensors and inertial measurement units to perform physical workplace assessments. Sensors. (2019) 19(8):1885. doi: 10.3390/s19081885
40. Hermens H. The State of the art on Sensors and Sensor Placement Procedures for Surface Electromyography: A Proposal for Sensor Placement Procedures. Enschede: Roessingh Research and Development (1997).
41. Halaki M, Gi K. Normalization of EMG signals: to normalize or not to normalize and what to normalize to? In: Naik GR, éditeur. Computational Intelligence in Electromyography Analysis—a Perspective on Current Applications and Future Challenges. Chapter 7. Rijeka: InTech (2012). p. 175–94.
42. Krosshaug T, Steffen K, Kristianslund E, Nilstad A, Mok KM, Myklebust G, et al. The vertical drop jump is a poor screening test for ACL injuries in female elite soccer and handball players: a prospective cohort study of 710 athletes. Am J Sports Med. (2016) 44(4):874–83. doi: 10.1177/0363546515625048
43. Bates NA, Schilaty ND, Ueno R, Hewett TE. Timing of strain response of the ACL and MCL relative to impulse delivery during simulated landings leading up to ACL failure. J Appl Biomech. (2020) 36(3):148–55. doi: 10.1123/jab.2019-0308
44. Hewett TE, Ford KR, Xu YY, Khoury J, Myer GD. Effectiveness of neuromuscular training based on the neuromuscular risk profile. Am J Sports Med. (2017) 45(9):2142–7. doi: 10.1177/0363546517700128
45. Letafatkar A, Rajabi R, Minoonejad H, Rabiei P. Efficacy of perturbation-enhanced neuromuscular training on hamstring and quadriceps onset time, activation and knee flexion during a tuck-jump task. Int J Sports Phys Ther. (2019) 14(2):214–27. doi: 10.26603/ijspt20190214
46. Maffiuletti NA, Aagaard P, Blazevich AJ, Folland J, Tillin N, Duchateau J. Rate of force development: physiological and methodological considerations. Eur J Appl Physiol. (2016) 116(6):1091–116. doi: 10.1007/s00421-016-3346-6
47. Stasinaki AN, Zaras N, Methenitis S, Bogdanis G, Terzis G. Rate of force development and muscle architecture after fast and slow velocity eccentric training. Sports. (2019) 7(2):41. doi: 10.3390/sports7020041
48. Louder T, Thompson BJ, Bressel E. Association and agreement between reactive strength Index and reactive strength Index-modified scores. Sports. (2021) 9(7):97. doi: 10.3390/sports9070097
49. Pleša J, Kozinc Ž, Smajla D, Šarabon N. The association between reactive strength index and reactive strength index modified with approach jump performance. PLoS One. (2022) 17(2):e0264144.
50. Flanagan EP, Comyns TM. The use of contact time and the reactive strength Index to optimize fast stretch-shortening cycle training. Strength Cond J. (2008) 30(5):32–8. doi: 10.1519/SSC.0b013e318187e25b
51. Hortobagyi T, Hill JP, Houmard JA, Fraser DD, Lambert NJ, Israel RG. Adaptive responses to muscle lengthening and shortening in humans. J Appl Physiol. (1996) 80(3):765–72. doi: 10.1152/jappl.1996.80.3.765
52. Nyland J, Kuzemchek S, Parks M, Caborn DNM. Femoral anteversion influences vastus medialis and gluteus medius EMG amplitude: composite hip abductor EMG amplitude ratios during isometric combined hip abduction-external rotation. J Electromyogr Kinesiol. (2004) 14(2):255–61. doi: 10.1016/S1050-6411(03)00078-6
53. Guilhem G, Cornu C, Guével A. Neuromuscular and muscle-tendon system adaptations to isotonic and isokinetic eccentric exercise. Ann Phys Rehabil Med. (2010) 53(5):319–41. doi: 10.1016/j.rehab.2010.04.003
54. Dolman B, Verrall G, Reid I. Physical principles demonstrate that the biceps femoris muscle relative to the other hamstring muscles exerts the most force: implications for hamstring muscle strain injuries. Muscles Ligaments Tendons J. (2014) 4(3):371–7.25506583
55. Donnelly CJ, Elliott BC, Doyle TLA, Finch CF, Dempsey AR, Lloyd DG. Changes in muscle activation following balance and technique training and a season of Australian football. J Sci Med Sport. (2015) 18(3):348–52. doi: 10.1016/j.jsams.2014.04.012
56. Hewett TE, Myer GD, Ford KR, Heidt RS, Colosimo AJ, McLean SG, et al. Biomechanical measures of neuromuscular control and Valgus loading of the knee predict anterior cruciate ligament injury risk in female athletes: a prospective study. Am J Sports Med. (2005) 33(4):492–501. doi: 10.1177/0363546504269591
57. Onate J, Herman D, Grooms D, Sutton Z, Wilkerson G. Neuroscience principles for ACL rehabilitation and reinjury risk reduction. In: Noyes FR, Barber-Westin S, éditeurs. Return to Sport After ACL Reconstruction and Other Knee Operations. Cham: Springer International Publishing (2019). p. 359–81.
58. King E, Richter C, Daniels KAJ, Franklyn-Miller A, Falvey E, Myer GD, et al. Can biomechanical testing after anterior cruciate ligament reconstruction identify athletes at risk for subsequent ACL injury to the contralateral uninjured limb? Am J Sports Med. (2021) 49(3):609–19. doi: 10.1177/0363546520985283
59. Alashram AR, D’Onofrio R, Sannicandro I, Manzi V, Padua E, Youssef T, et al. Return to training in soccer players after anterior cruciate ligament reconstruction: reflections and considerations. J Sports Med Phys Fitness. (2024) 64(6):578–87. doi: 10.23736/S0022-4707.23.15448-X
60. Draovitch P, Patel S, Marrone W, Grundstein MJ, Grant R, Virgile A, et al. The return-to-sport clearance Continuum is a novel approach toward return to sport and performance for the professional athlete. Arthrosc Sports Med Rehabil. (2022) 4(1):e93–101. doi: 10.1016/j.asmr.2021.10.026
61. Rodriguez-Merchan EC, Valentino LA. Return to sport activities and risk of reinjury after primary anterior cruciate ligament reconstruction. Arch Bone Jt Surg. (2022) 10(8):648–60. doi: 10.22038/ABJS.2021.50463.2504
62. Bisciotti GN, Volpi P, Alberti G, Aprato A, Artina M, Auci A, et al. Italian Consensus statement (2020) on return to play after lower limb muscle injury in football (soccer). BMJ Open Sport Exerc Med. (2019) 5(1):e000505. doi: 10.1136/bmjsem-2018-000505
63. Barber-Westin S, Noyes FR. One in 5 athletes sustain reinjury upon return to high-risk sports after ACL reconstruction: a systematic review in 1239 athletes younger than 20 years. Sports Health. (2020) 12(6):587–97. doi: 10.1177/1941738120912846
Keywords: neuromotor control, rehabilitation, elite soccer, CMJ, lower-limb injuries, LSI, norm values
Citation: Memain G, Carling C, Bouvet J, Maille P, Tamalet B, Fourcade P and Yiou E (2024) Evaluation of the impact of a 3-week specific-sport rehabilitation program on neuromotor control during single-leg countermovement-jump tests in professional soccer players with lower-limb injuries. Front. Sports Act. Living 6:1448401. doi: 10.3389/fspor.2024.1448401
Received: 13 June 2024; Accepted: 20 November 2024;
Published: 5 December 2024.
Edited by:
Robert C. Lynall, University of Georgia, United StatesReviewed by:
Italo Sannicandro, University of Foggia, ItalyKornelius Kraus, Self-employed, Aying, Germany
Copyright: © 2024 Memain, Carling, Bouvet, Maille, Tamalet, Fourcade and Yiou. This is an open-access article distributed under the terms of the Creative Commons Attribution License (CC BY). The use, distribution or reproduction in other forums is permitted, provided the original author(s) and the copyright owner(s) are credited and that the original publication in this journal is cited, in accordance with accepted academic practice. No use, distribution or reproduction is permitted which does not comply with these terms.
*Correspondence: Geoffrey Memain, Z21lbWFpbkBmZmYuZnI=