- 1Department of Nutritional Sciences, University of Connecticut, Storrs, CT, United States
- 2Patriot Performance Laboratory, Frank Pettrone Center for Sports Performance, George Mason University, Fairfax, VA, United States
- 3Sport, Recreation, and Tourism Management, George Mason University, Fairfax, VA, United States
- 4Sports Medicine Department, Mayo Clinic Health System, La Crosse, WI, United States
- 5Exercise Science and Exercise Physiology, Kent State University, Kent, OH, United States
- 6Exercise Science and Athletic Training, Springfield College, Springfield, MA, United States
Introduction: The purpose was to examine the prevalence of low energy availability (LEA), explore dietary behaviors in men collegiate gymnasts (n = 14), and investigate the relationships between energy availability (EA), body composition, and plyometric performance.
Methods: Body composition was measured using air displacement plethysmography. Lower- and upper-body peak power (PWRpeak) and modified reactive strength index (RSImod) were calculated from countermovement jump (CMJ) and plyometric push-up (PP) assessments. Energy expenditure was tracked over 3 days, while daily energy and macronutrient intake were recorded. EA was calculated and used to categorize athletes into LEA and non-LEA groups. Pearson correlation coefficients were used to examine relationships between EA, body composition, and performance metrics.
Results: 85.7% of athletes (n = 12) exhibited LEA (20.98 ± 5.2 kcals/kg FFM), with non-LEA athletes (n = 2) marginally surpassing the <30 kcal/kg of fat-free mass (FFM) threshold (30.58 ± 0.2 kcals/kg FFM). The cohort (n = 14) consumed insufficient energy (30.5 ± 4.5 kcal/kg/day) and carbohydrates (3.7 ± 1.1 g/kg/day), resulting in LEA (22.36 ± 5.9 kcal/kg/FFM). EA was not correlated with body composition or performance metrics.
Discussion: A high prevalence of LEA may exist in men gymnasts, largely due to a low relative energy and carbohydrate intake.
1 Introduction
Gymnastics is a physically demanding sport that requires the combination of strength, muscular endurance, flexibility, coordination, and technical skill to execute intricate routines with precision and control (1). The sport of men's gymnastics includes six events: floor exercise, pommel horse, rings, vault, parallel bars, and high bar (2). Many routines involve a combination of twists, jumps, inversions, and other high-velocity movements resulting in a unique variety of physiological demands and concomitant adaptations (3). Durations of routines vary substantially with event, ranging from approximately 5 s in the vault to 70–90 s on the floor exercise, further highlighting the sport's diverse bioenergetic demands (1). To develop physiological capabilities, gymnasts adhere to rigorous training schedules from a young age, maintaining weekly training loads ranging from 21 to 37 h year-round, which presents long-term challenges to weight management practices (4, 5).
Low body fat to high fat free mass ratios are thought to enhance performance by improving movement efficiency, aesthetic presentation, strength-to-mass ratio, and providing various biomechanical advantages (3, 6). Specifically, leanness is considered a critical body composition characteristic for success, with elite female and male gymnasts maintaining body fat levels ranging from 13%–16% to 8%–12%, respectively (3, 7). Consequently, athletes engaged in aesthetic sports like gymnastics have been found to be at a higher risk of developing behaviors of disordered eating and excessive activity levels in an attempt to minimize fat mass (6). While research on disordered eating in men's gymnastics is limited, reports indicate that nearly 30% of male athletes engaged in sports emphasizing leanness exhibit harmful weight control behaviors (8, 9), marked by restricted energy and carbohydrate intake (10). Additionally, evidence suggests a curvilinear relationship exists between body mass index (BMI) and gymnastics performance, suggesting that excessively low BMI may adversely affect both performance and health (3, 11). Therefore, gymnasts’ pursuit of a lean physique, beyond the point of performance optimization, increases the risk of disordered eating patterns, potentially leading to relative energy deficiencies (5).
Low energy availability (LEA) is a metric used to quantify the residual energy available to support the body's physiological functions after accounting for the energy expenditure from exercise (12–14). LEA is used to identify athletes at risk of Relative Energy Deficiency in Sport (RED-S), which represents a multifactorial physiological dysfunction resulting from chronic energy deficiency, stemming from insufficient energy intake, excessive energy expenditure from training, or a combination of both (15, 16). Calculated energy availability (EA) values for males are: <30 kcal/kg FFM/day, clinical LEA; 30–45 kcal/kg FFM/day, subclinical LEA; ≥40 kcal/kg FFM/day, optimal EA (17). The majority of studies investigating the consequences of LEA have focused predominantly on female athletes (9). Research within women's gymnastics has highlighted the increased risk of LEA among pre-teenage (5), teenage (5), and adult (18, 19) gymnasts. Emerging evidence suggests that male athletes, particularly those in aesthetic-based competitions (18) or weight-category sports (20), are vulnerable to the detrimental effects of LEA (9). Indeed, the 2014 International Olympic Committee's (IOC) consensus statement (21), along with subsequent updates in 2018 (22) and 2023 (23), on RED-S, has broadened the understanding of LEA to include male athletes. While LEA is frequently used to identify risk factors or diagnose RED-S, there is a lack of research investigating the prevalence of LEA among men collegiate gymnasts. Moreover, uncertainties exist regarding the potential impact of LEA on various indices of physical performance, particularly in terms of plyometric performance.
The rapid muscle contractions required during gymnastics routines on apparatuses such as the vault, rings, and floor exercise demand explosive lower- and upper-body power (24). These contractions are driven by the stretch-shortening cycle (SSC), marked by a swift transition between the initial eccentric “stretch” and the subsequent concentric “recoil” (25). The modified reactive strength index (RSImod), which is calculated from jump height, ground contact time, and body mass, serves as a useful indicator of successful technical execution (24), especially for movements requiring muscle re-contraction after landing. While RSImod focuses on the ability to produce force during the SSC, the measurement of peak power (PWRpeak) provides distinct yet related information. PWRpeak represents the maximum instantaneous power and is crucial for generating the height, rotation, and momentum necessary for movements such as vaulting, tumbling, and dismounting from apparatuses (24). Previous research has examined aspects of upper- and lower-body power in gymnasts including girls (26), women collegiate (27), and boys (28). However, research among men collegiate gymnasts, the metrics of RSImod and PWRpeak, and their relationship with EA and body composition remain understudied.
Therefore, the purpose of the current study was to examine the prevalence of LEA and explore dietary behaviors among a convenience sample of National Collegiate Athletic Association (NCAA) Division III collegiate men's gymnastics athletes. A secondary aim was to investigate the relationships between EA, body composition, and upper- and lower-body plyometric performance metrics.
2 Materials and methods
2.1 Experimental design
This observational study began in August prior to the academic term and gymnastics season. Athletes underwent assessments of body composition, reactive strength index (RSI), and power output during one day of testing. Additionally, a 3-day monitoring period was conducted to evaluate EA. Athletes recorded their dietary intake and were provided with team-based heart rate monitoring systems equipped with inertial sensors to measure activity energy expenditure during practices.
2.2 Participants
NCAA Division III men gymnastics athletes (n = 14) participated in the current study (age: 21.0 ± 1.2 years; height: 1.66 ± 4.68 m; body mass: 67.6 ± 5.1 kg). Players were medically cleared for intercollegiate athletic participation, had the risks and benefits explained beforehand, signed an institutionally approved written consent form, and completed a medical history form.
2.3 Procedures
2.3.1 Body composition
Athletes were instructed to refrain from exercise, eating, and drinking for ≥2 h before testing. Testing was conducted in the early morning after an overnight fast. Upon arrival to the laboratory, height and body mass were recorded to the nearest 0.01 cm and 0.02 kg, respectively, using a stadiometer (Detecto, Webb City, MO, USA) and digital scale (BOD POD model 2000A; BOD POD; Cosmed USA, Concord, CA, USA), with each subject barefoot. Body composition variables (i.e., percent body fat [BF%], fat-free mass [FFM], and fat mass ) were assessed using air displacement plethysmography (BOD POD model 2000A; BOD POD; Cosmed USA, Concord, CA, USA) according to standard operating procedures. Fat-free mass index (FFMI) was calculated using the following equation (29): FFM (kg)/Height2 (m2).
Before each testing session, calibration procedures were completed according to the manufacturer guidelines using an empty chamber and a calibrating cylinder of a standard volume (49.55 L). Once all tests passed, researchers proceeded with testing. A trained technician performed Bod Pod testing. Participants were instructed to enter the Bod Pod and sit in an erect position with their hands folded in their laps to obtain body volume. Athletes were instructed to wear unpadded compression shorts or spandex and, when applicable, a swim cap with the hair tucked in. They were asked to remove all jewelry to reduce air displacement. Athletes entered the Bod Pod and sat in an upright position with hands folded on their laps. Lung gas volume was estimated using manufacturer guidelines.Two tests were performed to ensure reliability of the assessment. If the tests results were not within 150 ml of each other, 2 more tests were executed to achieve reliable data.
2.3.2 Plyometric performance variables
Lower- and upper-body peak power (PWRpeak, w) and modified RSI (RSImod, AU) were calculated from countermovement jump (CMJ) and plyometric push-up (PP) assessments, using a commercially available force plate (AccuPower Force Platform, AMTI: Watertown, MA, USA). Athletes were instructed to keep their hands on their hips during the jump and were instructed to maintain straight legs during the flight, before landing on both feet with flexion of the hips, knees, and ankles. For the PP, athletes were positioned with their hands right below their shoulders and their feet shoulder-width apart. When instructed, athletes lowered their elbows to the height of their shoulders and pushed up as fast and as forcefully as they could. A trial was discarded and repeated when a participant did not lower their elbows to their shoulder height as visually inspected by the same trained researchers. Three attempts of CMJ and PP were conducted per assessment, with a minimum of 2-min rest between each trials to minimize fatigue effects.
PWRpeak was automatically yielded from AMTI's data acquisition software (NetForce, Watertown, MA, USA). RSImod was subsequently computed by normalizing force plate-derived jump height and ground contact time with respect to each athlete's body mass:
2.3.3 Energy availability
Athletes were asked to record dietary intake using an online commercially available nutrition analysis program (MyFitnessPal, Under Armour, Baltimore, MD, USA). Prior to this period, participants attended an informational session where they were educated on methods to estimate portion sizes accurately. This session included detailed descriptions and demonstrations of portion size estimatation techniques. Additionally, they were provided with informational packets and instructional videos to reinforce accurate self-reporting. Daily energy and macronutrient intakes were averaged across the 3-day monitoring period. Absolute energy and macronutrient intakes (kcal/day or g/day) were recorded and were expressed relative to body weight (kcal/kg/day or g/kg/day) to enable comparison between individuals. During the same 3-day period, activity energy expenditure was monitored via wearable monitoring devices (Polar H9, Polar Electro, Kempele, Finland) and calculated from proprietary algorithms. These devices have been used in previous studies to measure activity energy expenditure in athletic populations (30). EA was then calculated by subtracting the activity energy expenditure from energy intake and expressed as kcals per kilogram of FFM. A threshold of <30 kcal/kg of FFM was used to classify athletes as having LEA.
2.4 Statistical analysis
SPSS version 29.0 (IBM Corp., Armonk, NY, USA) was used for summary statistics. Athletes were categorized into LEA vs. non-LEA groups to observe differences in body composition, EA, and plyometric performance variables. Absolute and relative carbohydrate, protein, and fat intake were also analyzed. However, due to the small sample size of athletes in the non-LEA group (n = 2), inferential statistics were de-emphasized and a descriptive analysis (mean ± SD, range) was prioritized. Pearson correlation coefficients were used to examine relationships between EA, body mass, fat-free mass, fat mass, body fat percentage, CMJ-height, CMJ-RSImod, CMJ-PWRpeak, PP-height, PP-RSImod, and PP-PWRpeak. The following criteria were used for interpreting the correlation coefficients: very weak: <0.20; weak: 0.20–0.39; moderate: 0.40–0.59; strong: 0.60–0.79; and very strong: >0.80 (p < 0.05) (31).
3 Results
A summary of anthropometric, body composition, and demographic data stratified by energy status are presented as mean ± SD in Table 1. All athletes exhibited body fat levels below 10% (9.2 ± 3.5%; range: 5.7–19.1), with a body mass of 67.6 ± 5.1 kg (range: 57.5–76.0), FFM of 61.3 ± 5.7 kg (range: 52.1–69.9), FFMI of 22.12 ± 1.7 kg m2 (range: 18.93–24.76), and FM of 6.15 ± 2.2 kg (range: 3.5–12.3).
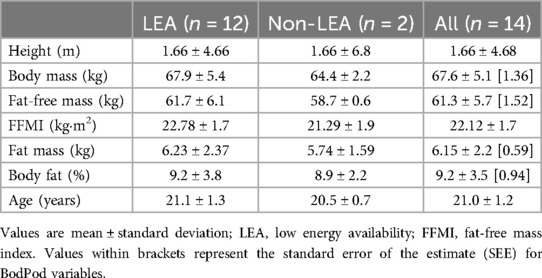
Table 1. Summary of subject anthropometric, body composition and demographic data, stratified by energy status (n = 14).
A summary of dietary intake (energy, carbohydrates, protein, fat), activity energy expenditure, and EA, stratified by LEA status is provided in Table 2. A total of 85.7% of athletes (n = 12) exhibited LEA (20.98 ± 5.2 kcals/kg FFM), with non-LEA athletes (n = 2) marginally surpassing the <30 kcal/kg of FFM threshold (30.58 ± 0.2 kcals/kg FFM), thus qualifying for subclinical LEA (30–40 kcal/kg/FFM) (9, 17). The entire cohort (n = 14) consumed insufficient energy (30.5 ± 4.5 kcal/kg/day; range: 23.5–39.0), resulting in LEA (22.36 ± 5.9 kcal/kg/FFM; range: 12.0–30.7). Carbohydrate intake was low (3.7 ± 1.1 g/kg/day; range: 2.6–7.0), likely contributing to low energy intake. Relative protein and fat intakes for the cohort were 1.6 ± 0.4 kg (range: 90.3–113.6) and 0.9 ± 0.3 kg (range: 53.0–88.3), respectively. Average activity energy expenditure for the 3-day monitoring period was 685.8 ± 170.4 kcal (range: 400.0–1,003.0).
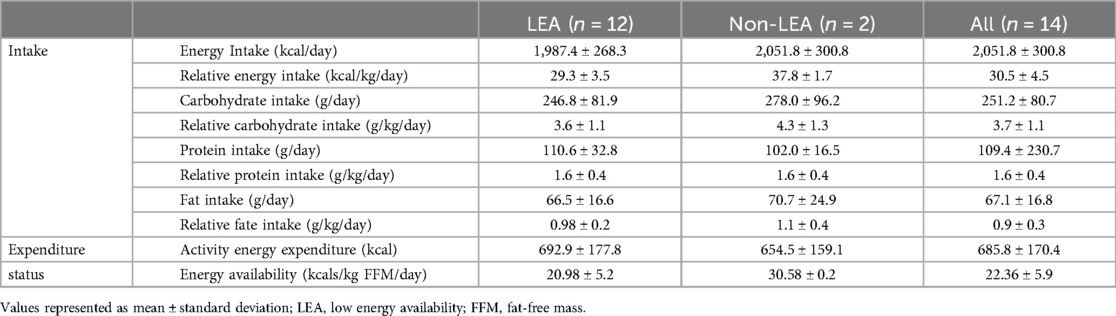
Table 2. A summary of average daily activity energy expenditure and macronutrient intake by energy status.
A summary of average movement height, RSImod, and PWRpeak for CMJ and PP, stratified by LEA status is included in Table 3. For lower body, athletes had a CMJ-height of 0.38 ± 0.05 m (range: 0.29–0.46), RSImod of 0.48 ± 0.10 AU/kg (range: 0.31–0.64), and a PWRpeak of 3,663.9 ± 563.6 w (range: 2,362.6–4,662.6). For upper body, athletes had a PP-height of 0.25 ± 0.09 m (range: 0.12–0.42), RSImod of 0.19 ± 0.06 AU/kg (range: 0.11–0.29), and PWRpeak of 1,678.9 ± 295.3 w (range: 1,233.8–2,301.9).
Relationships between EA, body composition metrics, and upper- and lower-body plyometric metrics are presented in Table 4. EA was not correlated with body composition metrics nor upper- or lower-body performance metrics. BF% was correlated with FFM (p = 0.025; 95% CI: −0.855, 0.093), FM [p < 0.001; 95% CI: (0.95, 0.995)], CMJ-height [p = 0.012; 95% CI: (−0.877, −0.177)], and CMJ-RSImod [p = 0.43; 95% CI: (−0.835, −0.024)]. FM was correlated with CMJ-height [p = 0.008; 95% CI: (−0.888, −0.226)] and CMJ-RSImod [p = 0.037; 95% CI: (−0.841, −0.043)]. CMJ-height was correlated with CMJ-RSImod [p < 0.01; 95% CI: (0.401, 0.923)] and CMJ-PWRpeak [p = 0.04; 95% CI: (0.031, 0.838)]. CMJ-RSImod was corelated with CMJ-PWRpeak [p = 0.025; 95% CI: (0.091, 0.855)]; PP-height was correlated with PP-PWRpeak [p < 0.001; 95% CI: (0.537, 0.945)].
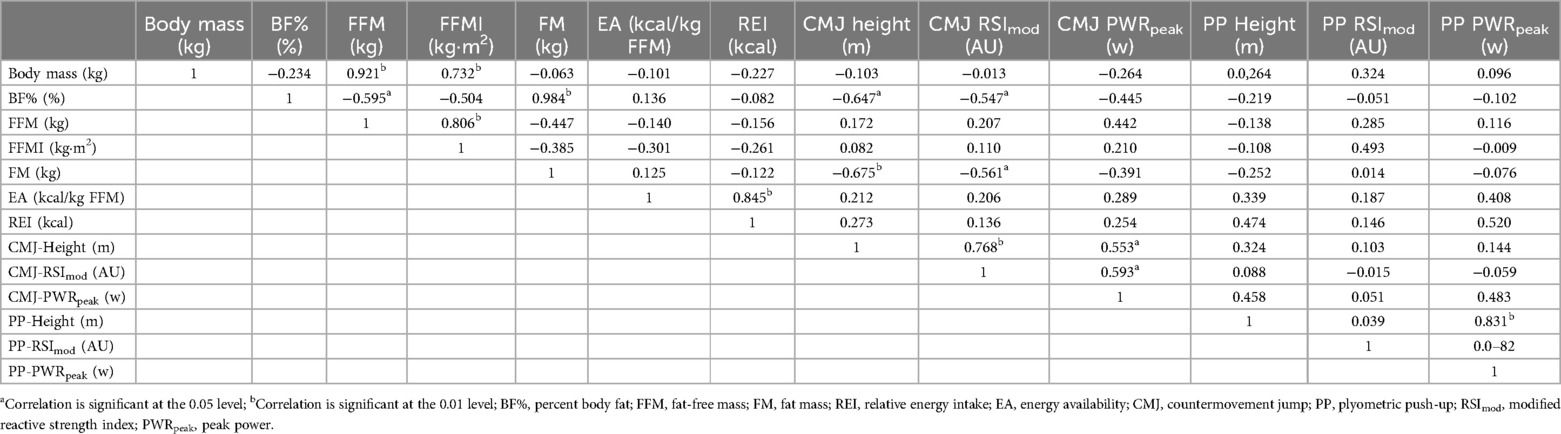
Table 4. Relationships between energy availability, body composition, and upper- and lower-body plyometric variables.
4 Discussion
The primary aim of the current study was to examine the prevalence of LEA and to explore dietary behaviors among a convenience sample of men collegiate gymnasts. Of note, 85.7% of the athletes within the cohort experienced LEA, as determined through direct assessment of dietary intake and activity energy expenditure. Additionally, the cohort consumed inadequate energy (30.5 ± 4.5 kcal/kg/day), largely influenced by a low carbohydrate intake (3.7 ± 1.1 g/kg/day), leading to the observed state of LEA (22.36 ± 5.9 kcals/kg FFM/day). No significant correlation existed between EA and either body composition or upper- and lower-body plyometric performance metrics. This study is novel as it marks the first instance of LEA prevalence reported in a sample of male gymnasts.
Previous reports in women's gymnastics indicate LEA prevalence rates range from 35%–56% for adult recreational and elite athletes (18), and 75% for teenage athletes (5), which are all lower than the prevalance of LEA observed in the current study. Moreover, the high prevalence of LEA observed in men gymnasts surpasses previous findings in men's dancing (29%) (32), a similar aesthetic sport emphasizing weight-control behavior. However, it is important to consider that LEA assessment in the study of men's dancing was conducted using the Dance-Specific Energy Availability Questionnaire (DEAQ), underscoring the challenge of making direct comparisons between studies due to differences in measurement techniques (9). LEA prevalence rates of 25% (33), 30% (34), 66% (35), and 70% (10), have been reported in male endurance athetes from non-aesthetic sports like cycling, distance running, and race walking. While LEA rates are notably lower than those observed in the current study, these studies had larger sample sizes (n = 21–53) and longer assessment durations (≥7 days). The absence of a standardized reference time frame for dietary or exercise assessments complicates absolute comparisons of LEA prevalence (36). Further, these studies involved well-trained, elite adult, or masters participants (age range: 18–50 years), potentially lacking the unique constraints experienced by the collegiate athletes in the current study, such as limited fueling opportunites due to demanding academic schedules, which could hinder access to optimal nutritional resources (37). It is important to note that the cross-sectional nature of these studies makes it unclear whether the causes of LEA are intentional, such as disordered eating behaviors, or inadvertent, stemming from factors like high-volume training or lack of education (9, 21, 38). With the exception of high-level endurance sports, which require high-volumes of training, low energy intake likely underpins the high prevalence of LEA for most aesthetic sport athletes. For example, the activity energy expenditure observed in the current study (692.9 ± 177.8 kcal) would not be considered an excessively high activity level. As such, it is apparent that nutrient deficiencies, primarily insufficient macronutrient energy consumption, plays a major role in influencing LEA prevalence (10).
The mean EA value observed in the current study was 22.36 ± 5.9 kcals/kg FFM/day, falling below the threshold of <30 kcals/kg FFM/day used to classify individuals with LEA (17). Surprisingly, it is lower than previously reported values in pre-teenage (60.04 kcal/kg FFM/day), teenage (29.7 kcal/kg FFM/day), and adult (31.5 kcals/kg FFM/day) female gymnasts (5, 19). Differences in sample size, measurement techniques, and assessment durations may partially explain the disparities in EA. However, the notably lower EA observed in the current study suggests potential issues with energy intake among men collegiate gymnasts that warrant further investigation. In part, lower EA values may be explained by the greater amounts of FFM in male gymnasts compared to females. It is plausible that men and women gymnasts may have comparable levels of energy expenditure; thus if the men neglected to compensate by increasing energy intake, lower EA values will result. The mean EA for athletes in the current study is comparable to previous season average values reported for male cyclists (20 kcals/kg FFM/day) (10), but appears lower than previously reported preseason values for male distance runners and racewalkers (27–36 kcals/kg FFM/day) (39, 40). Further, the mean FFM (61.3 ± 5.7 kg) for the gymnasts is comparable to that previously reported for male cyclists (63.8 ± 3.8 kg). These findings contribute to the growing body of evidence suggesting that male athletes are vulnerable to the adverse effects of LEA, particularly those engaged in high-volume training (e.g., distance runners), weight-category sports (e.g., wrestlers), and aesthetic-based competitions (e.g., gymnasts, dancers) (9) due to their higher energy requirements and greater amounts of FFM.
The primary contributor of LEA appears to stem from insufficient energy intake, namely from a low carbohydrate intake. Scientific societies have provided recommendations regarding the optimal carbohydrate intake for athletes based on their specific sports disciplines (41, 42). For men's and women's gymnastics, a relative carbohydrate intake of 5–7 g/kg/day is suggested (42). However, while previous reports indicated an average carbohydrate intake of 5.1 ± 2.3 g/kg/day for adult female gymnasts without LEA (19), gymnasts in the current study consumed 3.7 ± 1.1 g/kg/day, a level similar to that reported for teenage female gymnasts with LEA (3.7 ± 1.3 g/kg/day) (5). Given the limited evidence indicating substantial differences in carbohydrate utilization between adolescents and adults (43), it seems that inadequate carbohydrate intake is the most probable contributor to energy deficiency in male and female gymnastics athletes, irrespective of age (5, 43). In support, protein (1.6 ± 0.4 kcal/kg/day) and fat (0.9 ± 0.3 kcal/kg/day) intake were within appropriate ranges (41, 42) considering the type and amount of physical activity performed by the men gymnasts. However, the focus on leanness in gymnastics often results in weight-control measures, including carbohydrate restriction, a trend also noted in competitive male cyclists (10). Research highlights the prevalence of fad diets advocating carbohydrate restriction in sports emphasizing leanness (10), which stresses the importance of sport nutrition education, practical dietary skills development, and behavior change interventions, which may be necessary to effectively address nutritional deficiencies among athletes (16).
The current investigation is the first to report PWRpeak and RSImod values for a sample of collegiate men gymnasts. Notably, the CMJ-PWRpeak (3,663.9 ± 563.6 w) exceeds previous reports for NCAA DI women gymnasts (32,100 ± 350 w) (27) and substantially surpasses findings for male youth gymnasts (1,400 ± 543 w) (28). While average RSImod has not been documented in other gymnastics populations (e.g., women, youth), the observed average RSImod in current study (0.48 ± 0.10 w) surpasses values reported for men's baseball (0.41 ± 0.08 w), men's tennis (0.30 ± 0.07 w), and men's soccer (0.44 ± 0.05 w) athletes (44). The notably higher RSImod observed in men gymnasts compared to athletes in field or court sports underscores the unique demands of gymnastics, where athletes heavily rely on the SSC during plyometric activities, as they maneuver their bodies acrobatically on various apparatuses. Over time, this likely leads to sport-specific adaptations, which help develop lower-body power and explosiveness. Currently, PP-PWRpeak (1,678.9 ± 295.3 w) or PP-RSImod (0.19 ± 0.06 w) values have not been reported for gymnastics or other aesthetic sport athletes.
A secondary aim of the current study was to investigate the relationships between EA, body composition metrics, and upper- and lower-body plyometric performance metrics, namely RSImod and PWRpeak, in a sample of collegiate men's gymnastics athletes. While no correlations were found, this finding contrasts with a recent study conducted by Jurov et al., which observed decreases in CMJ height following 14 days of induced LEA with a 50% reduction in EA in trained male endurance athletes (45). In a subsequent study, the same research group observed decreases in PWRpeak, relative power, and CMJ-height following 14 days of induced LEA with a 25% reduction in EA (46). In a final study, where EA was reduced in progressive stages of 25%, 50%, and 75%, Jurov et al. discovered CMJ height decreased proportionately with increased EA reductions, and most of the negative effects occurred in the range of 9–25 kcals/kg FFM/day (47). Differences between the aforementioned studies and the current one in subject populations, sport demands, and study design, may account for the disparate results between EA and plyometric performance. However, it is noteworthy that the EA observed in the current study (22.36 ± 5.9 kcals/kg FFM/day) falls within the upper range associated with the most pronounced negative effects on performance as reported by Jurov et al. (47). Further, reports suggesting that suboptimal EA detrimentally affects explosive power even prior to hormonal changes indicate the potential utility of monitoring changes in plyometric performance as early indications of the adverse effects of LEA (46). However, more research is needed to elucidate the specific impact of LEA on plyometric performance in men's gymnastics. Additionally, the current study reported significant negative associations (r = −0.547) between CMJ-RSImod and BF%, emphasizing the importance of maintaining low BF%. This is critical for not only enhancing movement efficiency and promoting aesthetic presentation but also for optimizing strength-to-mass ratio and gaining various biomechanical advantages during set routines (3). Lastly, our findings revealed no significant correlation between EA and body composition variables, contrasting with reports from studies on athletes in other sports (48). This may be due to the lack of standardized protocols for calculating EA, potential errors in self-reported dietary intake and activity expenditure measurements, and individual variability in physiological responses (49). The complex nature of body composition changes in response to EA further complicates the detection of clear correlations (49). Future research with larger sample sizes, accurate measurement techniques, and standardized protocols is necessary to elucidate this relationship.
While the current study has provided valuable insights into dietary behaviors and plyometric performance for a sample of collegiate male gymnasts, a few limitations should be considered. First, the study's small sample size (n = 14) may limit the generalizability of the findings. Additionally, the reliance on a single time point, heart rate-derived activity energy expenditure measures, and self-reported dietary intake might not fully capture the dynamic nature of athletes’ energy balance. It should also be noted that the Polar H9 heart rate monitors, used in this study to estimate activity energy expenditure, have not been validated for measuring exercise energy expenditure in activities like gymnastics that often involve substantial anaerobic components. Further, under-reporting is a common issue in dietary self-reporting, and there is the potential for subjects to alter their dietary intake during the recording period, which might not reflect their long-term intake patterns (49). Lastly, the lack of information on whether certain athletes were undergoing intentional weight-cutting during data collection is a notable limitation, as such phases could influence energy intake and expenditure, potentially skewing the results. Future research with larger cohorts and longitudinal designs is warranted to clarify these relationships in greater detail.
5 Conclusion
The current research is novel in that it provides the first report of LEA prevalence and upper- and lower-body plyometric performance metrics in a sample of men collegiate gymnasts. Results indicate there may be a high prevalence of LEA, underscoring the need for attention to nutritional practices within this population. Findings suggest that athletes are underfueling, particularly in terms of carbohydrates, which is critical for meeting the energy demands of intense gymnastics training and competition. Failure to address LEA may have implications for performance outcomes among athletes participating in weight-control sports. While the relationship between EA and plyometric performance has been documented in sports such as distance running, it has yet to be determined in men's gymnastics, underscoring the need for further research. Additionally, prolonged LEA can compromise various aspects of athlete health, including bone metabolism, hormonal function, and immune response, thereby elevating the risk of injuries and illness. Interventions aimed at optimizing EA and carbohydrate intake are important for safeguarding the well-being, and potentially physical performance, of men collegiate gymnasts.
Data availability statement
The raw data supporting the conclusions of this article will be made available by the authors, without undue reservation.
Ethics statement
The studies involving humans were approved by Springfield College Institutional Review Board. The studies were conducted in accordance with the local legislation and institutional requirements. The participants provided their written informed consent to participate in this study.
Author contributions
NK: Writing – original draft, Writing – review & editing, Data curation, Formal Analysis. MJ: Writing – original draft, Writing – review & editing, Conceptualization. AJ: Conceptualization, Visualization, Writing – original draft, Writing – review & editing. MM: Data curation, Formal Analysis, Writing – review & editing. LW: Data curation, Project administration, Visualization, Writing – original draft, Writing – review & editing. JF: Methodology, Project administration, Supervision, Writing – original draft, Writing – review & editing.
Funding
The author(s) declare that no financial support was received for the research, authorship, and/or publication of this article.
Acknowledgments
The authors would like to thank all the men's gymnastics athletes and coaching staff who participated in this project and supported the study procedures.
Conflict of interest
The authors declare that the research was conducted in the absence of any commercial or financial relationships that could be construed as a potential conflict of interest.
Publisher's note
All claims expressed in this article are solely those of the authors and do not necessarily represent those of their affiliated organizations, or those of the publisher, the editors and the reviewers. Any product that may be evaluated in this article, or claim that may be made by its manufacturer, is not guaranteed or endorsed by the publisher.
References
1. Kaufmann S, Ziegler M, Werner J, Noe C, Latzel R, Witzany S, et al. Energetics of floor gymnastics: aerobic and anaerobic share in male and female sub-elite gymnasts. Sports Med Open. (2022) 8(3):1–10. doi: 10.1186/s40798-021-00396-6
2. Sleeper MD, Kenyon LK, Elliott JM, Cheng MS. Measuring sport-specific physical abilities in male gymnasts: the men’s gymnastics functional measurement tool. Int J Sports Phys Ther. (2016) 11:1082–100.27999723
3. Trexler ET, Smith-Ryan AE, Roelofs EJ, Hirsch KR. Body composition, muscle quality and scoliosis in female collegiate gymnasts: a pilot study. Int J Sports Med. (2015) 36:1087–92. doi: 10.1055/s-0035-1555781
4. Edouard P, Steffen K, Junge A, Leglise M, Soligard T, Engebretsen L. Gymnastics injury incidence during the 2008, 2012 and 2016 Olympic games: analysis of prospectively collected surveillance data from 963 registered gymnasts during Olympic games. Br J Sports Med. (2018) 52:475–81. doi: 10.1136/bjsports-2017-097972
5. Villa M, Villa-Vicente JG, Seco-Calvo J, Mielgo-Ayuso J, Collado PS. Body composition, dietary intake and the risk of low energy availability in elite-level competitive rhythmic gymnasts. Nutrients. (2021) 13:1–15. doi: 10.3390/nu13062083
6. Currie A. Sport and eating disorders—understanding and managing the risks. Asian J Sports Med. (2010) 1:63–8. doi: 10.5812/asjsm.34864
7. Bale P, Goodway J. Performance variables associated with the competitive gymnast. Sports Med. (1990) 10:139–45. doi: 10.2165/00007256-199010030-00001
8. Sundgot-Borgen J, Torstveit MK. Prevalence of eating disorders in elite athletes is higher than in the general population. Clin J Sport Med. (2004) 14:25–32. doi: 10.1097/00042752-200401000-00005
9. McGuire A, Warrington G, Doyle L. Low energy availability in male athletes: a systematic review of incidence, associations, and effects. Transl Sports Med. (2020) 3:173–87. doi: 10.1002/tsm2.140
10. Viner RT, Harris M, Berning JR, Meyer NL. Energy availability and dietary patterns of adult male and female competitive cyclists with lower than expected bone mineral density. Int J Sport Nutr Exerc Metab. (2015) 25:594–602. doi: 10.1123/ijsnem.2015-0073
11. Sherman RT, Thompson RA, Rose JS. Body mass index and athletic performance in elite female gymnasts. J Sport Behav. (1996) 19.
12. Loucks AB, Kiens B, Wright HH. Energy availability in athletes. J Sports Sci. (2011) 29(Suppl 1):S7–15. doi: 10.1080/02640414.2011.588958
13. Williams NI, Koltun KJ, Strock NCA, De Souza MJ. Female athlete triad and relative energy deficiency in sport: a focus on scientific rigor. Exerc Sport Sci Rev. (2019) 47:197–205. doi: 10.1249/JES.0000000000000200
14. Logue DM, Madigan SM, Melin A, Delahunt E, Heinen M, Donnell S-JM, et al. Low energy availability in athletes 2020: an updated narrative review of prevalence, risk, within-day energy balance, knowledge, and impact on sports performance. Nutrients. (2020) 12:1–19. doi: 10.3390/nu12030835
15. Jagim AR, Fields J, Magee MK, Kerksick CM, Jones MT. Contributing factors to low energy availability in female athletes: a narrative review of energy availability, training demands, nutrition barriers, body image, and disordered eating. Nutrients. (2022) 14:1–26. doi: 10.3390/nu14050986
16. Magee MK, Lockard BL, Zabriskie HA, Schaefer AQ, Luedke JA, Erickson JL, et al. Prevalence of low energy availability in collegiate women soccer athletes. J Funct Morphol Kinesiol. (2020) 5:1–9. doi: 10.3390/jfmk5040096
17. Melin AK, Heikura IA, Tenforde A, Mountjoy M. Energy availability in athletics: health, performance, and physique. Int J Sport Nutr Exerc Metab. (2019) 29:152–64. doi: 10.1123/ijsnem.2018-0201
18. Meng K, Qiu J, Benardot D, Carr A, Yi L, Wang J, et al. The risk of low energy availability in Chinese elite and recreational female aesthetic sports athletes. J Int Soc Sports Nutr. (2020) 17(13):1–7. doi: 10.1186/s12970-020-00344-x
19. Silva MRG, Paiva T. Low energy availability and low body fat of female gymnasts before an international competition. Eur J Sport Sci. (2015) 15:591–9. doi: 10.1080/17461391.2014.969323
20. Dolan E, Crabtree N, McGoldrick A, Ashley DT, McCaffrey N, Warrington GD. Weight regulation and bone mass: a comparison between professional jockeys, elite amateur boxers, and age, gender and BMI matched controls. J Bone Miner Metab. (2012) 30:164–70. doi: 10.1007/s00774-011-0297-1
21. Mountjoy M, Sundgot-Borgen J, Burke L, Carter S, Constantini N, Lebrun C, et al. The IOC consensus statement: beyond the female athlete triad–relative energy deficiency in sport (RED-S). Br J Sports Med. (2014) 48:491–7. doi: 10.1136/bjsports-2014-093502
22. Mountjoy M, Sundgot-Borgen JK, Burke LM, Ackerman KE, Blauwet C, Constantini N, et al. IOC consensus statement on relative energy deficiency in sport (RED-S): 2018 update. Br J Sports Med. (2018) 52:687–97. doi: 10.1136/bjsports-2018-099193
23. Mountjoy M, Ackerman KE, Bailey DM, Burke LM, Constantini N, Hackney AC, et al. 2023 International Olympic Committee’s (IOC) consensus statement on relative energy deficiency in sport (REDs). Br J Sports Med (2023) 57:1073–97. doi: 10.1136/bjsports-2023-106994
24. Dallas G, Dallas C, Pappas P, Paradisis G. Acute effect of bounce drop jump and countermovement drop jump with and without additional load on jump performance parameters and reactive strength index on young gymnasts. Hum Mov. (2023) 24:95–105. doi: 10.5114/hm.2023.125931
25. Flanagan EP, Comyns TM. The use of contact time and the reactive strength Index to optimize fast stretch-shortening cycle training. Strength Cond J. (2008) 30:32–8. doi: 10.1519/SSC.0b013e318187e25b
26. Hall E, Bishop DC, Gee TI. Effect of plyometric training on handspring vault performance and functional power in youth female gymnasts. PLoS One. (2016) 11(e0148790):1–10. doi: 10.1371/journal.pone.0148790
27. French DN, Gómez AL, Volek JS, Rubin MR, Ratamess NA, Sharman MJ, et al. Longitudinal tracking of muscular power changes of NCAA division I collegiate women gymnasts. J Strength Cond Res (2004) 18:101–7. doi: 10.1519/1533-4287(2004)018%3C0101:ltompc%3E2.0.co;2
28. Kochanowicz A, Niespodzinski B, Mieszkowski J, Kochanowicz K, Zasada M. Vertical jump peak power estimation in young male gymnasts. Baltic J Health Phys Act. (2016) 8:25–31. doi: 10.29359/BJHPA.08.1.03
29. VanItallie TB, Yang MU, Heymsfield SB, Funk RC, Boileau RA. Height-normalized indices of the body’s fat-free mass and fat mass: potentially useful indicators of nutritional status. Am J Clin Nutr. (1990) 52:953–9. doi: 10.1093/ajcn/52.6.953
30. Rayner SE, Pellerine LP, Wu Y, Shivgulam ME, Petterson JL, Kimmerly DS, et al. Faster stepping cadence partially explains the higher metabolic cost of walking among females versus males. J Appl Physiol. (2024) 136:1238–44. doi: 10.1152/japplphysiol.00904.2023
31. Warner RM. Applied Statistics II: Multivariable and Multivariate Techniques. 3rd ed. London, UK: SAGE Publications Inc. (2021).
32. Keay N, Overseas A, Francis G. Indicators and correlates of low energy availability in male and female dancers. BMJ Open Sport Exerc Med. (2020) 6(e000906):1–7. doi: 10.1136/bmjsem-2020-000906
33. Heikura IA, Uusitalo ALT, Stellingwerff T, Bergland D, Mero AA, Burke LM. Low energy availability is difficult to assess but outcomes have large impact on bone injury rates in elite distance athletes. Int J Sport Nutr Exerc Metab. (2018) 28:403–11. doi: 10.1123/ijsnem.2017-0313
34. Torstveit MK, Fahrenholtz I, Stenqvist TB, Sylta Ø, Melin A. Within-day energy deficiency and metabolic perturbation in male endurance athletes. Int J Sport Nutr Exerc Metab. (2018) 28:419–27. doi: 10.1123/ijsnem.2017-0337
35. Jurov I, Keay N, Hadžić V, Spudić D, Rauter S. Relationship between energy availability, energy conservation and cognitive restraint with performance measures in male endurance athletes. J Int Soc Sports Nutr. (2021) 18(24):1–10. doi: 10.1186/s12970-021-00419-3
36. Koehler K, Achtzehn S, Braun H, Mester J, Schaenzer W. Comparison of self-reported energy availability and metabolic hormones to assess adequacy of dietary energy intake in young elite athletes. Appl Physiol Nutr Metab. (2013) 38:725–33. doi: 10.1139/apnm-2012-0373
37. Heaney S, O’Connor H, Naughton G, Gifford J. Towards an understanding of the barriers to good nutrition for elite athletes. Int J Sports Sci Coach. (2008) 3:391–401. doi: 10.1260/174795408786238542
38. Nattiv A, Loucks AB, Manore MM, Sanborn CF, Sundgot-Borgen J, Warren MP, et al. American college of sports medicine position stand. The female athlete triad. Med Sci Sports Exerc. (2007) 39:1867–82. doi: 10.1249/mss.0b013e318149f111
39. Hooper DR, Kraemer WJ, Saenz C, Schill KE, Focht BC, Volek JS, et al. The presence of symptoms of testosterone deficiency in the exercise-hypogonadal male condition and the role of nutrition. Eur J Appl Physiol. (2017) 117:1349–57. doi: 10.1007/s00421-017-3623-z
40. Heikura IA, Burke LM, Bergland D, Uusitalo ALT, Mero AA, Stellingwerff T. Impact of energy availability, health, and sex on hemoglobin-mass responses following live-high-train-high altitude training in elite female and male distance athletes. Int J Sports Physiol Perform. (2018) 13:1090–6. doi: 10.1123/ijspp.2017-0547
41. Potgieter S. Sport nutrition: a review of the latest guidelines for exercise and sport nutrition from the American college of sport nutrition, the International Olympic Committee and the International Society for Sports Nutrition. South Afr J Clin Nutr. (2013) 26:6–16. doi: 10.1080/16070658.2013.11734434
42. Thomas DT, Erdman KA, Burke LM. American college of sports medicine joint position statement. Nutrition and athletic performance. Med Sci Sports Exerc. (2016) 48:543–68. doi: 10.1249/MSS.0000000000000852
43. Parnell JA, Wiens KP, Erdman KA. Dietary intakes and supplement use in pre-adolescent and adolescent Canadian athletes. Nutrients. (2016) 8. doi: 10.3390/nu8090526
44. Suchomel TJ, Sole CJ, Bailey CA, Grazer JL, Beckham GK. A comparison of reactive strength index-modified between six U. S. collegiate athletic teams. J Strength Cond Res. (2015) 29:1310–6. doi: 10.1519/JSC.0000000000000761
45. Jurov I, Keay N, Rauter S. Severe reduction of energy availability in controlled conditions causes poor endurance performance, impairs explosive power and affects hormonal status in trained male endurance athletes. Appl Sci (2021) 11:8618. doi: 10.3390/app11188618
46. Jurov I, Keay N, Spudić D, Rauter S. Inducing low energy availability in trained endurance male athletes results in poorer explosive power. Eur J Appl Physiol. (2022) 122:503–13. doi: 10.1007/s00421-021-04857-4
47. Jurov I, Keay N, Rauter S. Reducing energy availability in male endurance athletes: a randomized trial with a three-step energy reduction. J Int Soc Sports Nutr. (2022) 19:179–95. doi: 10.1080/15502783.2022.2065111
48. Bartlett JD, Hatfield M, Parker BB, Roberts LA, Minahan C, Morton JP, et al. DXA-derived estimates of energy balance and its relationship with changes in body composition across a season in team sport athletes. Eur J Sport Sci. (2020) 20:859–67. doi: 10.1080/17461391.2019.1669718
Keywords: low energy availability, body composition, reactive strength index, power output, gymnastics
Citation: Kuhlman NM, Jones MT, Jagim AR, Magee MK, Wilcox L and Fields JB (2024) Dietary intake, energy availability, and power in men collegiate gymnasts. Front. Sports Act. Living 6: 1448197. doi: 10.3389/fspor.2024.1448197
Received: 12 June 2024; Accepted: 6 September 2024;
Published: 18 September 2024.
Edited by:
Baoming Tian, Zhejiang University of Technology, ChinaReviewed by:
Karen Dolins, Columbia University, United StatesNemanja Lakicevic, University of Palermo, Italy
Copyright: © 2024 Kuhlman, Jones, Jagim, Magee, Wilcox and Fields. This is an open-access article distributed under the terms of the Creative Commons Attribution License (CC BY). The use, distribution or reproduction in other forums is permitted, provided the original author(s) and the copyright owner(s) are credited and that the original publication in this journal is cited, in accordance with accepted academic practice. No use, distribution or reproduction is permitted which does not comply with these terms.
*Correspondence: Margaret T. Jones, mjones15@gmu.edu