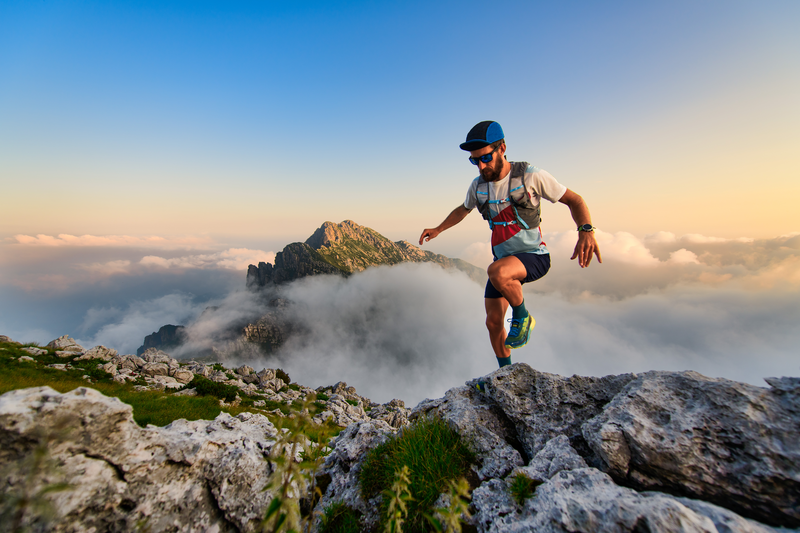
95% of researchers rate our articles as excellent or good
Learn more about the work of our research integrity team to safeguard the quality of each article we publish.
Find out more
REVIEW article
Front. Sports Act. Living , 11 December 2024
Sec. Exercise Physiology
Volume 6 - 2024 | https://doi.org/10.3389/fspor.2024.1423308
This article is part of the Research Topic Innovative Approaches to Exercise Assessment and Prescription in Non-Communicable Diseases View all 7 articles
Objectives: To conduct a systematic review to determine the acute and chronic effects of inspiratory muscle training (IMT) in type 2 diabetes mellitus (T2DM) patients on cardiac autonomic function, glucose variability, inspiratory muscle strength and endurance, hemodynamic variables, and exercise capacity.
Methods: A search was carried out according to a specific search strategy, following the PRISMA statement, and three independent reviewers have undertaken the article selection process. Searches were carried out in June 2023, on the following electronic databases: EMBASE, MEDLINE (PubMed), SCOPUS (Elsevier), and Web of Science. The methodological quality of the studies was assessed using the PEDro scale. The search was limited to English-language, randomized controlled trials (RCTs), involving T2DM patients (>18 years old, with or without autonomic neuropathy, and/or inspiratory muscle weakness) following an acute or chronic intervention protocol based on IMT. Exclusion criteria were reviews, clinical trials, case studies, theses, dissertations, scientific conference abstracts, subjects with other chronic respiratory/neurological/cardiovascular diseases, and studies addressing other breathing exercises.
Results: The search strategy identified 1,352 studies, of which eight (two involving acute and six involving chronic IMT effects) were included. A total of 214 adults aged 52–63 years (51/49 male/female ratio), with BMI ranging from 27 to 36.8 kg/m², were included. The results demonstrated that after IMT, acute effects were reported, such as reduced glucose levels and an increase in the parasympathetic pathway, but also chronic effects including improved inspiratory muscle strength, endurance, and exercise capacity.
Conclusion: Although some methodological differences among the studies were found, IMT may have beneficial effects on cardiac autonomic function, glucose level control, inspiratory muscle strength/endurance as well as exercise capacity. However, further studies are necessary to confirm these benefits.
Type 2 diabetes mellitus (T2DM) is a chronic metabolic disease characterized by hyperglycemia caused by insulin resistance. This resistance results from a gradual decline in sufficient β-cell insulin secretion, potentially linked to environmental or genetic factors (1–3). The International Diabetes Federation projected that the diabetes prevalence, will increase from 10.5% in 2021, to 11.3% by 2030 and 12.2% by 2040 (4). It is known that impaired muscle metabolism associated with phrenic nerve neuropathy in T2DM patients, contributes to decreased respiratory muscle strength, leading to respiratory muscle dysfunction. Although the physiological mechanisms are not fully understood, T2DM patients have shown reduced endurance in their respiratory muscles, and this decline is linked to the level of metabolic control, as measured by HbA1c levels (2, 5–8). In addition, chronic hyperglycemia leads to chronic complications such as autonomic cardiovascular neuropathy, which can affect the cardiac autonomic nervous system (1, 9, 10). A prior meta-analysis revealed diminished sympathetic and parasympathetic modulation in T2DM patients, likely due attributed to the deleterious metabolic consequences of altered glucose metabolism on cardiac autonomic regulation (9).
Contemporary literature recommends lifestyle modification, including physical activity and adherence to a well-balanced diet, as beneficial strategies for managing T2DM (11). For this population, both moderate-intensity continuous training and high-intensity interval training are recommended, with no evidence of superiority between them, and aerobic and resistance exercises are often advised (2, 12, 13). Several studies suggest that physical exercise positively influences various physiological and psychological factors in people with T2DM, including insulin sensitivity, skeletal muscle oxidative capacity, inflammation, blood glucose levels, stress, anxiety, depression, pain, mood, self-efficacy, cognitive function, cardiovascular health, and circadian rhythms (2, 14, 15). These findings indicate that physical exercise may benefit patients with T2DM by potentially enhancing their overall well-being and quality of life (15). Physical activities and dietary interventions are generally not associated with serious adverse effects. However, physical activity can sometimes lead to orthopedic injuries, and individuals with low physical fitness may face cardiovascular-related adverse effects during exercise, such as sudden increases in cardiovascular variables and, in rare cases, death. Notably, due to the low incidence of serious adverse effects, this aspect has been minimally explored in scientific studies (16).
Inspiratory muscle training (IMT) has been proposed as a complementary therapy for the management of T2DM. This type of ventilatory training has shown to decrease blood glucose levels, decrease insulin resistance and diaphragmatic breathing capacity, and increase respiratory muscle strength. It can also be used as an initial strategy prior to initiation of physical activity (17, 18). IMT has demonstrated positive effects on cardiac autonomic function, which is frequently impaired in T2DM patients, thereby increasing cardiovascular risk. IMT promotes a reduction of cardiac sympathetic modulation coupled with an elevation in cardiac parasympathetic modulation at rest (19, 20).
A recent systematic review assessed the chronic effects of IMT in T2DM, with inconclusive findings on functional capacity, glycemic control, and cardiac autonomic regulation (21). Our study, however, investigates the acute effects of IMT, focusing on immediate physiological responses that were not addressed in prior research. Additionally, we investigate previously unexplored outcomes, such as hemodynamic variables including heart rate and blood pressure. By examining these acute effects, our study aims to provide new insights into the potential mechanisms of action of IMT and its immediate impact on type 2 diabetes management.
These insights may enhance our understanding of the intervention's overall effectiveness and safety both during and immediately following its application. Therefore, this systematic review aims to assess the acute and chronic effects of IMT in T2DM focusing on cardiac autonomic function, glucose levels, inspiratory muscle strength and endurance, exercise capacity, functional capacity, and hemodynamic variables. This knowledge will deepen our understanding of IMT adaptations and adjustments in individuals with T2DM, providing valuable guidance to rehabilitation professionals. It will support the development of more effective IMT prescriptions in clinical settings, tailored to both short- and long-term treatment objectives.
This study was conducted following the Preferred Items for Systematic Reviews and Meta-analysis (PRISMA) 2020 guidelines (22), and was registered on the International Prospective Register of Systematic Reviews (PROSPERO) under the identification number: CRD42023481340.
The search was performed in June 2023, in the following electronic databases: EMBASE, MEDLINE (PubMed), SCOPUS (Elsevier), and Web of Science. It was restricted to randomized clinical trials and the English language. The following search strategy incorporated Medical Subject Headings (MeSH) with keywords was applied: (breathing exercises OR exercise, breathing OR respiratory muscle training OR muscle training, respiratory OR training, respiratory muscle OR inspiratory muscle training) AND (exercise tolerance OR exercise capacity OR glycemic control OR control, glycemic OR blood glucose control OR control, blood glucose OR glucose control, blood OR blood glucose OR hyperglycemia OR hypoglycemia OR glycemic index OR heart rate OR heart rate OR blood pressure OR respiratory muscle strength OR inspiratory muscle strength) AND (diabetes mellitus, type 2 OR diabetes mellitus).
Articles from each database were retrieved and imported into the Rayyan platform (available in: https://www.rayyan.ai). The study selection process involved three independent reviewers. Initially, two independent reviewers (A.L. and Z.B.M.) screened the titles and abstracts of all records identified following our eligibility criteria. Subsequently, full texts were screened and reviewed in order to determine inclusion in this systematic review. If there were some disagreements in some of the steps between these two independent reviewers, a third independent reviewer (C.G.) was consulted. Additionally, the reference lists of the included articles were screened to identify other possible eligible trials.
To formulate the study question and set eligibility criteria, the Population, Intervention, Comparison, Outcome, Study Design (PICOS) system was used. Eligible studies included: adult T2DM patients (P); performed an acute (<4 weeks) or chronic (≥4 weeks) IMT program (I); compared with a control group either without intervention or with a sham intervention (C); assessed cardiac autonomic function, hemodynamic variables (i.e., blood pressure, heart rate), glucose measurements, inspiratory muscle strength and endurance, exercise capacity, and/or functional capacity (O); were randomized clinical trials (RCTs) (S) evaluating the impact of IMT on T2DM subjects. Eligibility of selected articles was based on assessment of one or more of the following outcomes measures: cardiovascular autonomic function, inspiratory muscle strength (MIP and S-Index), glucose measurements (HbA1c or CGMS), and exercise capacity (VO2peak, VO2max or VCO2max). Eligible IMT protocols involve the use of inspiratory muscle trainer devices and the inspiratory load during the protocol must be ≥30% of the maximal inspiratory pressure (MIP) in the intervention group, as previous studies have shown that intensities lower than 30% do not promote clinically significant benefits in patients with T2DM (21). Exclusion criteria were included studies employing different types of respiratory exercises (e.g., expiratory muscle training, slow breathing) or combining other interventions during IMT protocol as well as additional comorbidities (e.g., neurological, chronic respiratory, cardiovascular, or musculoskeletal diseases).
From each selected study, data were reported through a descriptive analysis and extracted independently by two reviewers (A.L. and Z.B.M.), including: (1) characteristics of the samples, participants, and groups (sample size, group number, sex, age, MIP, T2DM diagnosis, glucose level, glycated hemoglobin (HbA1c), medication and other comorbidities) summarized in Table 1; (2) characteristics of interventions [duration, devices used, frequency, intensity, duration, respiratory frequency, intervention details, supervision, and control group (CG) intervention] reported in Table 2; and (3) outcomes description (autonomic function, glucose measurements, inspiratory muscle strength/endurance, and exercise capacity) detailed in Table 3.
The PEDro quality scale for RCTs was used to assess the quality of the included studies. This scale comprises 11 items including eligibility criteria, randomized allocation, concealed allocation, comparable at baseline, blinded subjects, blinded therapists, blinded assessors, adequate follow-up, intention to treat analysis, between-group comparisons, point estimates and variability. The first item does not contribute to the score, resulting in a maximum achievable score of 10 points. Moreover, the methodological quality of articles was categorized as poor (<4 points), fair (4–5 points), good (6–8), or excellent (9–10), based on previous quality criteria (30). Quality assessment was conducted independently by two reviewers (A.L. and Z.B.M.) and in case of disagreements between them, a third reviewer (C.G.) was consulted.
A PRISMA flow diagram of the literature search and selection is presented in Figure 1. The initial search identified a total of 1,352 potential studies through the database search (EMBASE = 1,075, PubMed = 152, SCOPUS = 74, Web of Science = 49) and PubMed additional studies suggestions. A total of 342 duplicates were identified and removed. From the titles and abstract reading, 17 studies were selected for full-text reading. Out of 17 studies, 11 were excluded, six were included and two studies retrieved from PubMed suggestions were screened and accepted for inclusion (Figure 1). As a result, eight studies were included in this systematic review.
A total of 214 adults aged 52–63 years were included, with male (n = 104) and female (n = 100) proportions of 50.9% and 49.1%, respectively. One study did not report the sex of the participants (19). Seven studies included participants diagnosed with T2DM with more than five years of diagnosis (19, 24–29). According to the body mass index (BMI) classification, one study included Class I obese participants in the intervention group (23), and seven studies included overweight subjects (19, 24–29). Some participants were also presented with autonomic neuropathy or inspiratory muscle weakness, when their MIP were inferior to 70% of predicted value. Additionally, four studies have included some patients under beta-blockers (25, 26, 28, 29). More details of the study characteristics are reported in Table 1.
Different protocols including frequency, intensity, time, and type (FITT principles) were used to evaluate both acute (single session) and chronic effects (ranging from 8 to 12 weeks). The interventions ranged from 13 to 70 min, occurring 3–7 days per week. Two studies focused on acute effects, delivering IMT in a single session (25, 29), with an intensity of 60% of MIP in the intervention group (25, 29). Chronic IMT effects were investigated in six studies, with intervention durations ranging from 8 to 12 weeks (19, 23–25, 27, 28).
In these studies, the commonly applied training intensity ranged from 30% to 40% of MIP, as implemented in five of the included studies (19, 23, 24, 26, 28). Conversely, only one study applied a higher IMT intensity at 75% of MIP (27). The frequency of training ranged from 3 to 7 days a week (19, 23, 24, 26–28). Moreover, seven studies included at least one supervised session with a physiotherapist (23–29). Considering the IMT devices, seven studies utilized either a POWERbreathe® or a Threshold IMT device (19, 23–26, 28, 29), while one study used a TrainAir device with biofeedback (27).
Of the 8 studies included in this review, 7 of them compared the intervention group to the sham group, based on the same protocol but with a training intensity ranging from no resistance (0% of MIP) up to 15% of MIP. Finally, one study compared the IMT effects to the usual care, based on medication only (23). For more comprehensive details, please refer to Table 2.
The outcomes reported in this systematic review were summarized in Table 3, including: (1) heart rate (HR), heart rate variability (HRV), mean arterial pressure (MAP), blood pressure (BP), forearm vascular resistance (FVR), calf blood flow (CBF), and calf vascular resistance (CVR); (2) glucose measurement through plasma glucose and glycated hemoglobin (HbA1c); (3) inspiratory muscle strength determined by the MIP, the Sniff nasal inspiratory pressure (SNIP) and inspiratory muscle endurance represented by the ratio of the maximum threshold pressure (Pthmax) to MIP; (4) exercise capacity and/or functional capacity assessed either by cardiopulmonary exercise test (CPET), 6-minutes walking test (6MWT) or Timed Up and Go (TUG).
Cardiac autonomic modulation was evaluated in four studies (19, 25, 26, 29). One study concluded that IMT had no significant effect on HRV in time and frequency domains (26). A study reported a decrease in the power spectrum of the high-frequency band in normalized units (HFnu) (29). Another study proposed that IMT contributed to the reduction in sympathetic cardiac modulation by decreasing the normalized unit of the low-frequency (LFnu) power component of HRV (19). Regarding HR, three studies underwent analysis, each yielding conflicting data (25, 27, 29). One study observed lower HR at the end of the protocol in the T2DM and cardiovascular autonomic neuropathy (T2DM-CAN) group compared to the T2DM and control groups (25). One study showed a non-significant decrease in HR in the intervention group compared to the control group (27). One study revealed that the HR was higher in the experimental load compared to the sham load during the first, second, and last minute of intervention (29). Two studies showed an increase in MAP during the training session (25, 29). Two studies evaluated the BP, one highlighted a non-significant reduction in systolic and diastolic BP at rest (27), and another study showed an increase in BP during the protocol (29). Only one study investigated CBF and CVR, exposing a reduction in CBF and an increase in CVR in the T2DM and T2DM-CAN during the intervention in experimental groups compared with the control group (25).
Four studies evaluated glucose levels (23, 25, 28, 29). Two studies evaluated fasting plasma glucose levels (23, 28). In one study, there was a 106% reduction after the protocol (28), while the second study, although lacking statistically significant outcomes, revealed glucose values below 130 mg/dl, suggesting clinical significance (23). One study found no changes in HbA1c levels (28). One study showed a reduction in glucose levels over time without changes in glucose variability (29). One study illustrated a reduction in glucose levels evaluated by a continuous glucose monitoring system (CGMS) (25).
The assessment of inspiratory muscle strength was carried out in five distinct studies (19, 24, 26–28). Four studies demonstrated an improvement in inspiratory muscle strength (19, 24, 26, 27). One study reported no changes in inspiratory muscle strength (28). Additionally, two studies appraised muscle endurance, revealing an increase in endurance time and in the pressure time-index (PTI) characterizing maximal threshold pressure divided by MIP (26, 28).
Four studies evaluated exercise capacity (19, 24, 26, 27). One study employed the 6MWT, TUG, and HGS, demonstrating improvement in all three functional tests within both groups from baseline to the end of the protocol. The experimental group showed greater improvement than the sham group (24). Three studies used CPET with either a treadmill (26, 27) or a cycle ergometer (19). One study identified improvement in aerobic capacity between groups, with increased VO2max to IMT (27). Two studies reported no significant changes in exercise capacity (19, 26).
The items, total score of the PEDro scale and the quality evidence were described in Table 4. The highly variable scores (between 4 and 9) highlighted the heterogeneous methodological quality of the studies, with an average score of 5.5 points, which is considered “fair”. Five studies were classified as fair (19, 23, 25, 27, 29), two studies as good (24, 26) and one study as excellent (28) according to the Cashin & McAuley classification (30). The main items not reported in the included studies were unconcealed group allocation and blinding of subjects, therapists, and assessors. Although it would be important to consider these factors in future studies, it is possible that blinding process may have been limited by the study design and type of intervention adopted.
This systematic review aimed to determine the acute and chronic effects of IMT on different aspects of patients with T2DM. After careful analysis of the eight RCTs included in our study, with a total sample size of 214 participants, IMT demonstrated beneficial effects both in the short and long term. Of these studies, two focused on acute effects and assessed the cardiac autonomic modulation and glucose levels (25, 29), while six assessed chronic effects by evaluating inspiratory muscle endurance and strength, and exercise capacity. It is known that T2DM affects the whole body, including the cardiovascular, muscular, and/or respiratory systems (2, 5, 6, 8). Several studies have demonstrated increased insulin resistance, neuromuscular alterations and poor blood perfusion (1, 24) causing inspiratory muscle weakness (19), reduced exercise capacity (24), and alterations of autonomous function. IMT may delay or counter inspiratory muscle weakness and improve inspiratory muscle strength (24). Similarly to obese patients, IMT led to an improvement in inspiratory muscle strength and exercise capacity (31). According to the results reported in this systematic review, an increased hemodynamic response was observed during IMT however these changes were not sustained after session. IMT may promote acute decease in the resting cardiac sympathetic modulation and a reduction in glucose levels although only one study assessed these effects. In the long-term effects, inspiratory muscle strength and endurance were increased, also resulting in increased exercise capacity. Consequently, the use of IMT appears to be a non-conventional training option for T2DM patients. Furthermore, IMT may serve as a supplementary approach, particularly beneficial for individuals who are not compliant with traditional rehabilitation methods (32–34).
Acutely, IMT at intensities of 60% of MIP caused exaggerated peripheral vasoconstriction in T2DM, suggesting hyperactivation of the inspiratory metaboreflex. This activation can lead to higher HR and MAP, as well as reduced parasympathetic cardiac activity, as observed during the experimental session at 60% of MIP, but not with the sham load (25, 29). It is well known that inspiratory metaboreflex activation during strenuous exercise primarily increases MAP by enhancing cardiac output. However, these changes on hemodynamic variables were not sustained after the exercise ceased. Additionally, glucose levels significantly decreased during inspiratory muscle metaboreflex activity and remained low for at least 30 min after the acute session. The abrupt decrease in glucose levels observed during inspiratory loading was of a similar magnitude to the acute reduction in glucose levels observed after acute aerobic exercise in a similar population. This is consistent with subsequent findings of increased GLUT4 protein content in the sheep diaphragm induced by chronic inspiratory resistive flow. Controversially, although a reduction in glucose was observed 5 min and 120 min after the IMT session, no differences were found between the inspiratory loads (i.e., 60% of MIP vs. sham group). One of hypothesis is that controlled ventilation, applied before both experimental loads (2% and 60% of PImax), would be the determinant of glucose reduction (29). Controlled ventilation induces vagal stimulation, resulting in insulin secretion and glucose reduction. This is consistent with studies indicating that parasympathetic activation decreases hepatic glucose release and enhances insulin secretion in hyperglycemic conditions (35). Additionally, afferent vagal nerve stimulation leads to a sustained increase in glucose levels, partly mediated by the suppression of pancreatic insulin secretion. Conversely, efferent vagal nerve stimulation prompts pancreatic glucagon secretion, which seems to be counteracted by insulin secretion during selective efferent vagal nerve stimulation (36, 37).
It is known that inspiratory muscle weakness (IMW) and cardiovascular autonomic neuropathy (CAN) induced by T2DM can lead to resting sympathetic hyperactivity (20) related to an impairment of the cardiovascular autonomous control, as result an increased resting HR are often observed in these patients (38). When non-invasively assessed by HRV, Corrêa et al. (26) reported no significant difference in HRV despite employing a comparable protocol to Kaminski et al. (19), who found a positive reduction in sympathetic modulation indicated by decreased resting LFnu indexes after IMT, provides valuable insights into the effects of IMT. Although the mechanisms influencing HRV after IMT are conflicting in the literature (20), IMT may provide beneficial effects on the autonomic nervous system by increasing the resting parasympathetic activity and/or reducing the sympathetic activation, which is considered a cardioprotective response (20, 39–41). The physiological mechanisms involved in these changes might be related to improvements on baroreflex and metaboreflex sensitivity (42).
It is known that slow breathing and diaphragmatic breathing have been associated with increased baroreflex sensitivity in healthy and pathological conditions which might contribute for an improved cardiovascular autonomic regulation (20, 43, 44), however, greater effects on baroreflex have been observed when an inspiratory load is applied (44, 45). Furthermore, an increase in inspiratory muscle strength following loaded IMT is associated with a delay in the metaboreflex threshold, a reflex mediated by peripheral sympathetic activity. Both baroreflex and metaboreflex are physiological reflexes that respond to metabolic changes. The arterial baroreflex plays an essential role in BP adjustments given the baroreceptors in the carotid and aortic arteries (46). Alterations in BP due to changes in intrathoracic pressures during IMT, might lead to the stretching of these baroreceptors due to changes in the venous return, improving the baroreceptors responses to afferent neuronal firing driven by respiration (46). Consequently, this improved baroreflex sensitivity after chronic IMT, might promote a better autonomic balance, increasing the parasympathetic nerve activity and decreasing the sympathetic nerve activity at rest (46). While the intake of beta-blockers predominantly affects the autonomic nervous system by antagonizing beta-adrenergic receptors, which are pivotal in sympathetic nervous system function, it is noteworthy that only a minority of patients in the sample were taking beta-blocker medications.
Numerous studies have demonstrated the efficacy of IMT in lowering BP, achieved through the inhibition of sympathetic and the stimulation of parasympathetic modulation (43). Slow breathing and diaphragmatic breathing have proven to be a key contributor to BP reduction (43, 44). Furthermore, the application of resistance as during IMT has been proved to intensify negative intrathoracic pressures during the training, enhancing the impact on baroreflex sensitivity due to changes on venous return, and amplifying the reduction in BP (47). In one study, an elevation in BP, HR, and reduction of the HFnu component was noted, however, it is essential to contextualize that in this study, the outcome measures were assessed during the intervention (29). This finding aligns with expectations, as the heightened cardiovascular demands and increased oxygen requirements in active muscles during exercise typically increase sympathetic activity, leading to elevated HR and BP (48). Also, during exercise, the parasympathetic modulation is expected to decrease explaining the HFnu reduction (49). However, contrasting findings suggested that IMT did not impact the baroreflex in non-clinical populations and younger individuals (42, 50). Suggesting that more research is needed on the effects of IMT on baroreflex in subjects with T2DM.
Regarding glucose assessment, post IMT, patients exhibited reductions in both overall glucose levels and fasting blood glucose (25, 28). The improved inspiratory muscle strength after training, which is strongly related to and delays the activation of inspiratory metaboreflex (51, 52), can decrease dyspnea levels during exercise and enhance exercise tolerance, enabling individuals with T2DM to adopt a more physically active lifestyle. We hypothesize that an increased physical activity levels after IMT is crucial for elevating skeletal muscles blood glucose consumption, given that skeletal muscles in healthy and diabetic populations eliminate approximately 85% of blood glucose (53, 54). This phenomenon is attributed to the increase in glucose clearance facilitated by insulin stimulation and muscle contraction during exercise, which results in the redistribution and increase of the glucose transporter 4 translocation (GLUT4) at the cell surface, thus improving glucose utilization (54). In four studies assessing glucose levels, only two identified effects on glucose reduction at intensities of 60% and 30% of the 1RM in acute and chronic forms, respectively (25, 28).
Moreover, a reduction in glucose levels may also be dependent on intensity levels and protocol duration. While positive benefits have been noted in cardiovascular disease patients at intensities equivalent to 60% of MIP, future researches should investigate the potential impacts of high-intensity programs (i.e., >60% of MIP), as well as prolonged protocol durations specifically in patients with T2DM (55). Higher intensities combined with longer durations of IMT may yield more effective results in reducing blood glucose levels, fasting blood glucose as well as HbA1c (56–58). However, further studies are needed to identify optimal intensities and protocol durations.
Another hypothesis that could explain this drop in glucose is the intake of medication and the level of insulin secreted during a meal. Schein et al. (29) hypothesized that the elevation of insulin levels is induced by the meal, as well as by the medication, leading to a reduction in glucose levels. Although participants in seven studies were on medication, only one study reported a change in dosage due to medical recommendation (28). The lack of data makes it impossible to conclude whether IMT is solely responsible for the change in anti-diabetic drug dosage. Further studies are also needed to understand these changes. The hypothesis of controlled ventilation would also be decisive in glucose reduction. According to Tanida et al. (35), controlled ventilation would enable vagal stimulation, leading to insulin secretion, and thus glucose reduction.
Furthermore, obstructive sleep apnea is common condition present in patients with T2DM and can impair glucose tolerance through mechanisms such as intermittent hypoxia and increased sympathetic activity, potentially worsening glucose control (59). Given that IMT can enhance respiratory muscle strength and reduce obstructive sleep apnea severity, it is plausible that IMT could offer additional benefits by improving sleep apnea, which may subsequently lead to better glucose control (60). However, only one study included in this review reported on the occurrence of obstructive sleep apnea in T2DM patients or its improvement following IMT interventions, which should be further investigated (27).
Recent systematic reviews with meta-analyses suggest that aerobic exercise (61), combined exercise (62), resistance training (63), and high-intensity interval training (64) are beneficial for reducing glycemic levels in the diabetic population. Additionally, recent topic reviews evaluating other exercise modalities, such as Pilates and Yoga, in populations with obesity/overweight, also indicate improvements in parameters such as glucose metabolism (65, 66). Therefore, the findings of this systematic review suggest that IMT could serve as an additional and complementary strategy for enhancing glucose metabolism, as it offers glycemic benefits comparable to those reported in the literature for other forms of exercise as previously mentioned.
Enhancements in inspiratory muscle strength reported in four studies involving long-term protocols may be explained by the presence of IMW among patients with T2DM (19, 24, 26, 27). Subjects with IMW presenting a pre-training MIP of 56 ± 13 cmH2O, improving to 121 ± 22 cmH2O post-training (26, 27), while subjects without IMW presented a pre-training MIP of 88 ± 26 cmH2O and a post-training MIP of 137 ± 27 cmH2O (19). Dall'Ago et al. (67) stated that IMT significantly improved inspiratory muscle strength in patients with chronic heart failure accompanied by IMW, and Basso-Vanelli et al. (68) reported that patients with respiratory muscle weakness (RMW) were more likely to improve their strength and endurance with IMT than those without RMW. The reduction of respiratory muscle strength in T2DM patients is mainly due to impaired respiratory neuromuscular function caused by polyneuropathy (69). However, the mechanisms behind the development of IMT in T2DM patients are still poorly understood; therefore, more studies should be conducted to understand the role of IMW development in this specific population (26). IMT may offer more pronounced benefits for people with IMW than for those without, especially as studies agree that IMT increases diaphragmatic strength, thereby delaying diaphragmatic fatigue and activation of accessory inspiratory muscles (24). As well as the patient's physical condition, the intensity of the MIP may also explain the contradictory findings within the studies analyzed in this systematic review. The MIP intensity was set at 30%, a level that might be considered insufficient, especially considering that optimal enhancements in muscle strength have been observed at 60% of the MIP for both heart failure patients, with or without IMW (55). Regarding inspiratory muscle endurance, a low intensity (30% of MIP) seems to be sufficient to achieve significant improvement, such intensity may stimulate slow-twitch fibers which are characterized by their ability to sustain contractions for extended period (26, 28). Cho et al. (70) and Britto et al. (71) confirm this hypothesis, reporting that 30% of MIP intensity showed improvement in respiratory muscle endurance in patients with stroke.
Two studies showed a significant improvement in exercise capacity, using an intensity superior or equal to 40% of MIP, while the other two studies found no improvement, using an intensity of 30% of MIP. One hypothesis is that engaging in moderate to high-intensity IMT confers greater advantages in enhancing inspiratory muscle strength and, subsequently, exercise capacity. This enhancement is facilitated by the delayed onset of the inspiratory metaboreflex during physical exertion (72). This activation pattern reduces blood flow to the inspiratory muscles during exercise while enhancing blood perfusion in peripheral muscles (24). Notably, in healthy individuals, IMT delays the inspiratory metaboreflex, leading to reduced sympathetic activation and diminished peripheral vasoconstriction, factors that can otherwise limit exercise capacity (73). Consequently, enhancing diaphragmatic strength through IMT has been shown to alleviate the severity of dyspnea and boost both exercise and functional capacity across diverse populations (74, 75).
Although a limited number of studies report data on the adverse effects of IMT, it has been shown to be safe and well-tolerated in patients at risk of prolonged hospitalization, asthmatic subjects and children suffering from neuromuscular diseases (76–78). Some minor adverse effects such as muscle soreness has been reported (79). After IMT, Pinto et al. (28) reported hyperglycemia and/or hypoglycemia, fatigue, dyspnea, nausea, dizziness, and headaches, which may be uncorrelated with the application of IMT. Instead, hyperglycemia or hypoglycemia may result from dietary habits taken by the patient (80). Elevated blood glucose levels can also be explained by increased inspiratory load, as high-intensity exercise can prevent blood sugar from dropping by increasing counter-regulatory hormones, which can lead to an increase in blood glucose levels (81). The observation of increased GLUT4 protein content in the sheep diaphragm points in the same direction (82). Presence of dyspnea, for its part, may result from the patient's sedentary lifestyle but also from the respiratory muscular atrophy caused by diabetes (83).
The present systematic review was limited by (1) the heterogeneity of intervention protocols regarding duration, frequency, device, supervision, (2) the intensity of MIP used (varying from low-intensity to high-intensity), (3) different patient characteristics such as the pathologies included (inspiratory muscle weakness or autonomic neuropathy), lifestyle (sedentary or active), gender, drug treatments, different ranges of age, and/or diet (4) the small sample size not being representative of or significant to the study population, (5) the lack of information concerning the sample calculation of certain studies representing potential attrition biases, as well as the lack of information on some dropouts. In addition, two of the included studies with a short-term protocol mainly assessed autonomic function and glucose measurement (25, 29), whereas the other six studies with a long-term protocol focused mainly on inspiratory muscle endurance and strength, and exercise capacity (19, 23, 24, 26–28). Additionally, the studies did not assess the same outcome measures for the same duration of intervention, which makes it difficult to compare the findings among them.
This systematic review identified eight studies assessing the acute and chronic effect of IMT in patients with T2DM. Respiratory training has demonstrated acute positive effects on autonomic function and glucose levels depending on the intensity of MIP chosen. Chronic effects such as increased strength and endurance of the inspiratory muscles, and improved exercise capacity can also be observed. There is only one study that mention the positive chronic effects on autonomic function. IMT can be presented as a complementary tool to conventional training in T2DM patients. However, the results must be interpreted with caution, as some of the included studies presented biases (e.g., selection, attrition, and intervention biases). Further studies are needed to evaluate all outcome measures (cardiac autonomic modulation, blood glucose levels, inspiratory muscle strength/endurance and exercise capacity), including a standardized short- and long-term intervention protocol and a homogeneous sample, to obtain more accurate and meaningful results.
ZB-M: Data curation, Formal Analysis, Investigation, Methodology, Visualization, Writing – original draft, Writing – review & editing. CG: Data curation, Formal Analysis, Investigation, Methodology, Visualization, Writing – original draft, Writing – review & editing. AL: Data curation, Formal Analysis, Investigation, Methodology, Visualization, Writing – original draft, Writing – review & editing. PF: Methodology, Writing – original draft, Writing – review & editing. IM: Methodology, Writing – original draft, Writing – review & editing. PR-S: Methodology, Writing – original draft, Writing – review & editing. JM-M: Methodology, Writing – original draft, Writing – review & editing. RA: Conceptualization, Data curation, Formal Analysis, Investigation, Methodology, Project administration, Resources, Supervision, Validation, Visualization, Writing – original draft, Writing – review & editing.
The author(s) declare that no financial support was received for the research, authorship, and/or publication of this article.
The authors declare that the research was conducted in the absence of any commercial or financial relationships that could be construed as a potential conflict of interest.
All claims expressed in this article are solely those of the authors and do not necessarily represent those of their affiliated organizations, or those of the publisher, the editors and the reviewers. Any product that may be evaluated in this article, or claim that may be made by its manufacturer, is not guaranteed or endorsed by the publisher.
6MWT, 6-minute walk test; BMI, body mass index; BP, blood pressure; CAN, cardiovascular autonomic neuropathy; CBF, calf blood flow; CG, control group; CGMS, continuous glucose monitoring system; CPET, cardiopulmonary exercise test; CVR, calf vascular resistance; DM, diabetes mellitus; DM-CAN, diabetes mellitus with cardiovascular autonomic neuropathy; GLUT-4, glucose transporter type 4; HbA1c, glycated hemoglobin; HFnu, normalized units of the high-frequency power; HGS, hand grip strength; HR, heart rate; HRV, heart rate variability; IG, intervention group; IMS, inspiratory muscle strength; IMT, inspiratory muscle training; IMW, inspiratory muscle weakness; LFn, low-frequency power; LFnu, normalized unit of the low-frequency power; MAP, mean arterial pressure; MIP, maximal inspiratory pressure; PEDro, physiotherapy evidence database; Pthmax, inspiratory muscle endurance; PTI, pressure time index; RCT, randomized controlled trial; RMW, respiratory muscle weakness; SD, standard deviation; SNIP, sniff nasal inspiratory pressure; T2DM, type 2 diabetes mellitus; TUG, timed up and go; VO2max, maximal oxygen consumption.
1. Stanford KI, Goodyear LJ. Exercise and type 2 diabetes: molecular mechanisms regulating glucose uptake in skeletal muscle. Adv Physiol Educ. (2014) 38(4):308–14. doi: 10.1152/advan.00080.2014
2. Colberg SR, Sigal RJ, Yardley JE, Riddell MC, Dunstan DW, Dempsey PC, et al. Physical activity/exercise and diabetes: a position statement of the American Diabetes Association. Diabetes Care. (2016) 39:2065–79. doi: 10.2337/dc16-1728
3. American Diabetes Association Professional Practice Committee. Classification and diagnosis of diabetes: standards of medical care in diabetes—2022. Diabetes Care. (2022) 45(Supplement_1):S17–38. doi: 10.2337/dc22-S002
4. Magliano DJ, Boyko EJ, committee IDFD Ates. IDF Diabetes Atlas. IDF Diabetes Atlas Vol. 2021. Brussels: International Diabetes Federation © International Diabetes Federation (2021).
5. Brannagan TH, Promisloff RA, McCluskey LF, Mitz KA. Proximal diabetic neuropathy presenting with respiratory weakness. J Neurol Neurosurg Psychiatry. (1999) 67(4):539–41. doi: 10.1136/jnnp.67.4.539
6. Davis WA, Knuiman M, Kendall P, Grange V, Davis TM. Glycemic exposure is associated with reduced pulmonary function in type 2 diabetes: the fremantle diabetes study. Diabetes Care. (2004) 27:752–7. doi: 10.2337/diacare.27.3.752
7. Kolahian S, Leiss V, Nürnberg B. Diabetic lung disease: fact or fiction? Rev Endocr Metab Disord. (2019) 20(3):303–19. doi: 10.1007/s11154-019-09516-w
8. Bassi-Dibai D, Santos-de-Araújo AD, Dibai-Filho AV, de Azevedo LFS, Goulart CDL, Luz GCP, et al. Rehabilitation of individuals with diabetes mellitus: focus on diabetic myopathy. Front Endocrinol. (2022) 13:869921. doi: 10.3389/fendo.2022.869921
9. Benichou T, Pereira B, Mermillod M, Tauveron I, Pfabigan D, Maqdasy S, et al. Heart rate variability in type 2 diabetes mellitus: a systematic review and meta-analysis. PLoS One. (2018) 13:e0195166. doi: 10.1371/journal.pone.0195166
10. Nesti L, Pugliese NR, Sciuto P, Natali A. Type 2 diabetes and reduced exercise tolerance: a review of the literature through an integrated physiology approach. Cardiovasc Diabetol. (2020) 19:134. doi: 10.1186/s12933-020-01109-1
11. Zheng Y, Ley SH, Hu FB. Global aetiology and epidemiology of type 2 diabetes mellitus and its complications. Nat Rev Endocrinol. (2018) 14(2):88–98. doi: 10.1038/nrendo.2017.151
12. Newsome ANM, Reed R, Sansone J, Batrakoulis A, McAvoy C, Parrott MW. ACSM worldwide fitness trends: future directions of the health and fitness industry. ACSM’s Health Fit J. (2024) 28(1):14–26. doi: 10.1249/FIT.0000000000000933
13. Opazo-Díaz E, Montes-de-Oca-García A, Galán-Mercant A, Molina-García J, Hernández-Mocholí MA, Martínez-Rodríguez A, et al. Characteristics of high-intensity interval training influence anthropometrics, glycemic control, and cardiorespiratory fitness in type 2 diabetes mellitus: a systematic review and meta-analysis of randomized controlled trials. Sports Med. (2024) 54(12):3127–49. doi: 10.1007/s40279-024-02114-0
14. Batrakoulis A, Jamurtas AZ, Fatouros IG. High-intensity interval training in metabolic diseases: physiological adaptations. ACSM’s Health Fit J. (2021) 25(5):54–9. doi: 10.1249/FIT.0000000000000703
15. Rias YA, Apriliyasari RW, Gautama MSN, Hasan F, Teli M, Chiu HY, et al. Effects of physical and mind-body exercise on sleep quality in individuals with diabetes mellitus: a systematic review and meta-analysis. J Prev Med Public Health. (2024) 24(4):354. doi: 10.3961/jpmph.24.354
16. Hemmingsen B, Gimenez-Perez G, Mauricio D, Figuls MR, Metzendorf MI, Richter B. Diet, physical activity or both for prevention or delay of type 2 diabetes mellitus and its associated complications in people at increased risk of developing type 2 diabetes mellitus. Cochrane Database Syst Rev. (2017) 12. doi: 10.1002/14651858.CD003054.pub4
17. Silva MDS, Motriz GM, Pereira DAG, Pinto ACC, Machado FL, Borja AL, et al. Influence of inspiratory muscle training on changes in fasting hyperglycemia in the older adult: the Epidoso project. J Diabetes Sci Technol. (2015) 9(6):1352–3. doi: 10.1177/1932296815599006
18. Edwards AM, Wells C, Jones AM, Rodriguez B, Harrison K, Heathcote S, et al. Efficacy of inspiratory muscle training as a practical and minimally intrusive technique to aid functional fitness among adults with obesity. Respir Physiol Neurobiol. (2016) 234:85–8. doi: 10.1016/j.resp.2016.09.007
19. Kaminski DM, Schaan BD, da Silva AM, Soares PP, Dal Lago P, Bassi N, et al. Inspiratory muscle training in patients with diabetic autonomic neuropathy: a randomized clinical trial. Clin Auton Res. (2015) 25(4):263–6. doi: 10.1007/s10286-015-0291-0
20. Abreu RM, Rehder-Santos P, Minatel V, Dos Santos GL, Catai AM. Effects of inspiratory muscle training on cardiovascular autonomic control: a systematic review. Auton Neurosci Basic Clin. (2017) 208:29–35. doi: 10.1016/j.autneu.2017.09.002
21. Arruda NSD, Peixoto NDM, Callegaro CC, Trevisan ME, Jaenisch RB. Effects of inspiratory muscle training in type 2 diabetes: a systematic review. Braz J Cardiovasc Surg. (2023) 38:e20220366. doi: 10.21470/1678-9741-2022-0366
22. Page MJ, McKenzie JE, Bossuyt PM, Boutron I, Hoffmann TC, Mulrow CD, et al. The PRISMA 2020 statement: an updated guideline for reporting systematic reviews. Br Med J. (2021) 372:n71. doi: 10.1136/bmj.n71
23. Ahmad AM, Ali HM. Low-intensity inspiratory loaded exercises improve physical health perception in women with type 2 diabetes. Physiother Quart. (2020) 28(2):9–14. doi: 10.5114/pq.2020.92472
24. Albarrati A, Taher M, Nazer R. Effect of inspiratory muscle training on respiratory muscle strength and functional capacity in patients with type 2 diabetes mellitus: a randomized clinical trial. J Diabetes. (2021) 13(4):292–8. doi: 10.1111/1753-0407.13106
25. dos Santos Corrêa AP, Antunes CF, Figueira FR, de Castro MA, Ribeiro JP, Schaan BD. Effect of acute inspiratory muscle exercise on blood flow of resting and exercising limbs and glucose levels in type 2 diabetes. PLoS One. (2015) 10(3):121384. doi: 10.1371/journal.pone.0121384
26. Corrêa AP, Ribeiro JP, Balzan FM, Mundstock L, Ferlin EL, Moraes RS. Inspiratory muscle training in type 2 diabetes with inspiratory muscle weakness. Med Sci Sports Exerc. (2011) 43(7):1135–41. doi: 10.1249/MSS.0b013e31820a7c12
27. Moawd SA, Azab AR, Alrawaili SM, Abdelbasset WK. Inspiratory muscle training in obstructive sleep apnea associating diabetic peripheral neuropathy: a randomized control study. BioMed Res Int. (2020) 2020:5036585. doi: 10.1155/2020/5036585
28. Pinto MB, Bock PM, Schein ASO, Portes J, Monteiro RB, Schaan BD, et al. Inspiratory muscle training on glucose control in diabetes: a randomized clinical trial. Int J Sport Nutr Exerc Metab. (2021) 31(1):21–31. doi: 10.1123/ijsnem.2020-0175
29. Schein AS, Corrêa AP, Macedo AC, Dartora DR, da Silveira AD, Severo MD, et al. Acute inspiratory muscle exercise effect on glucose levels, glucose variability and autonomic control in patients with type 2 diabetes: a crossover randomized trial. Auton Neurosci. (2020) 226:102669. doi: 10.1016/j.autneu.2020.102669
30. Cashin AG, McAuley JH. Clinimetrics: physiotherapy evidence database (PEDro) scale. J Physiother. (2020) 66(1):59. doi: 10.1016/j.jphys.2019.08.005
31. Caicedo-Trujillo S, Torres-Castro R, Vasconcello-Castillo L, Solis-Navarro L, Sanchez-Ramirez D, Núñez-Cortés R, et al. Inspiratory muscle training in patients with obesity: a systematic review and meta-analysis. Front Med. (2023) 10:1284689. doi: 10.3389/fmed.2023.1284689
32. Pan B, Ge L, Xun YQ, Chen YJ, Gao CY, Han X, et al. Exercise training modalities in patients with type 2 diabetes mellitus: a systematic review and network meta-analysis. Int J Behav Nutr Phys Act. (2018) 15:1–14. doi: 10.1186/s12966-018-0703-3
33. Azambuja ACM, Oliveira LZ, Sbruzzi G. Inspiratory muscle training in patients with heart failure: what is new? Systematic review and meta-analysis. Phys Ther. (2020) 100(12):2099–109. doi: 10.1093/ptj/pzaa171
34. Beaujolin A, Mané J, Presse C, Barbosa-Silva J, Bernini M, Corbellini C, et al. Inspiratory muscle training intensity in patients living with cardiovascular diseases: a systematic review. Hearts. (2024) 5(1):75–90. doi: 10.3390/hearts5010006
35. Tanida M, Yamamoto N, Morgan DA, Kurata Y, Shibamoto T, Rahmouni K. Leptin receptor signaling in the hypothalamus regulates hepatic autonomic nerve activity via phosphatidylinositol 3-kinase and AMP-activated protein kinase. J Neurosci. (2015) 35(2):474–84. doi: 10.1523/JNEUROSCI.1828-14.2015
36. Verberne AJM, Sabetghadam A, Korim WS. Neural pathways that control the glucose counterregulatory response. Front Neurosci. (2014) 8:38. doi: 10.3389/fnins.2014.00038
37. Meyers EE, Kronemberger A, Lira V, Rahmouni K, Stauss HM. Contrasting effects of afferent and efferent vagal nerve stimulation on insulin secretion and blood glucose regulation. Physiol Rep. (2016) 4(4):e12718. doi: 10.14814/phy2.12718
38. Verrotti A, Prezioso G, Scattoni R, Chiarelli F. Autonomic neuropathy in diabetes mellitus. Front Endocrinol. (2014) 5:205. doi: 10.3389/fendo.2014.00205
39. Ferreira JB, Plentz RD, Stein C, Casali KR, Arena R, Dal Lago P. Inspiratory muscle training reduces blood pressure and sympathetic activity in hypertensive patients: a randomized controlled trial. Int J Cardiol. (2013) 166(1):61–7. doi: 10.1016/j.ijcard.2011.09.069
40. Martins de Abreu R, Porta A, Rehder-Santos P, Cairo B, da Silva CD, Signini ÉF, et al. Effects of inspiratory muscle-training intensity on cardiovascular control in amateur cyclists. Am J Physiol Regul Integr Comp Physiol. (2019) 317:891–R902. doi: 10.1152/ajpregu.00167.2019
41. Mello PR, Guerra GM, Borile S, Rondon MU, Alves MJ, Negrão CE, et al. Inspiratory muscle training reduces sympathetic nervous activity and improves inspiratory muscle weakness and quality of life in patients with chronic heart failure: a clinical trial. J Cardiopulm Rehabil Prev. (2012) 32(5):255–61. doi: 10.1097/HCR.0b013e31825828da
42. De Abreu R, Porta A, Rehder-Santos P, Cairo B, Donisete da Silva C, De Favari Signini É, et al. Effects of inspiratory muscle-training intensity on cardiovascular control in amateur cyclists. Am J Phys Regul Integr Comp Physiol. (2019) 317(6):R891–902. doi: 10.1152/ajpregu.00167.2019
43. Jones CU, Sangthong B, Pachirat O. An inspiratory load enhances the antihypertensive effects of home-based training with slow deep breathing: a randomised trial.
44. da Silva CD, de Abreu RM, Rehder-Santos P, De Noronha M, Catai AM. Can respiratory muscle training change the blood pressure levels in hypertension? A systematic review with meta-analysis. Scand J Med Sci Sports. (2021) 31(7):1384–94. doi: 10.1111/sms.13943
45. Dick TE, Mims JR, Hsieh YH, Morris KF, Wehrwein EA. Increased cardio-respiratory coupling evoked by slow deep breathing can persist in normal humans. Respir Physiol Neurobiol. (2014) 204:99–111. doi: 10.1016/j.resp.2014.09.013
46. Fisher JP, Young CN, Fadel PJ. Comprehensive physiology. Compr Physiol. (2015) 5(2):475–512. doi: 10.1002/cphy.c140022
47. James JEA. The effects of changes of extramural, “intrathoracic”, pressure on aortic arch baroreceptors. J Physiol (Lond). (1971) 214(1):89. doi: 10.1113/jphysiol.1971.sp009420
48. Mohammed LL, Dhavale M, Abdelaal MK, Alam AN, Blazin T, Prajapati D, et al. Exercise-induced hypertension in healthy individuals and athletes: is it an alarming sign? Cureus. (2020) 12(12):e11988. doi: 10.7759/cureus.11988
49. Michael S, Graham KS, Davis GM. Cardiac autonomic responses during exercise and post-exercise recovery using heart rate variability and systolic time intervals—a review. Front Physiol. (2017) 8. doi: 10.3389/fphys.2017.00301
50. DeLucia CM, De Asis RM, Bailey EF. Daily inspiratory muscle training lowers blood pressure and vascular resistance in healthy men and women. Exp Physiol. (2018) 103:201–11. doi: 10.1113/EP086641
51. Laoutaris ID. Exercise intolerance and skeletal muscle metaboreflex activity in chronic heart failure: do we need to recruit more muscle in exercise training? Eur J Prev Cardiol. (2020) 27(17):1858–61. doi: 10.1177/2047487320912623
52. Chan JS, Mann LM, Doherty CJ, Angus SA, Thompson BP, Devries MC, et al. The effect of inspiratory muscle training and detraining on the respiratory metaboreflex. Exp Physiol. (2023) 108(4):636–49. doi: 10.1113/EP090779
53. O’Gorman DJ, Karlsson HKR, McQuaid S, Yousif O, Rahman Y, Gasparro D, et al. Exercise training increases insulin-stimulated glucose disposal and GLUT4 (SLC2A4) protein content in patients with type 2 diabetes. Diabetologia. (2006) 49:2983–92. doi: 10.1007/s00125-006-0457-3
54. Wendt C, Espelage L, Eickelschulte S, Springer C, Toska L, Scheel A, et al. Contraction-mediated glucose transport in skeletal muscle is regulated by a framework of AMPK, TBC1D1/4, and Rac1. Diabetes. (2021) 70:2796–809. doi: 10.2337/db21-0587
55. Sadek Z, Salami A, Joumaa WH, Awada C, Ahmaidi S, Ramadan W. Best mode of inspiratory muscle training in heart failure patients: a systematic review and meta-analysis. Eur J Prev Cardiol. (2018) 25(16):1691–701. doi: 10.1177/2047487318792315
56. Umpierre D, Stein R, Vieira PJC, Ribeiro JP. Blunted vascular responses but preserved endothelial vasodilation after submaximal exercise in chronic heart failure. Eur J Cardiovasc Prev Rehabil. (2009) 16:53–9. doi: 10.1097/HJR.0b013e32831c8489
57. Boniol M, Dragomir M, Autier P, Boyle P. Physical activity and change in fasting glucose and HbA1c: a quantitative meta-analysis of randomized trials. Acta Diabetol. (2017) 54(11):983–91. doi: 10.1007/s00592-017-1037-3
58. Lee J, Kim D, Kim C. Resistance training for glycemic control, muscular strength, and lean body mass in old type 2 diabetic patients: a meta-analysis. Diabetes Ther. (2017) 8. doi: 10.1007/s13300-017-0258-3
59. Reutrakul S, Mokhlesi B. Obstructive sleep apnea and diabetes: a state of the art review. Chest. (2017) 152(5):1070–86. doi: 10.1016/j.chest.2017.05.009
60. Dar JA, Mujaddadi A, Moiz JA. Effects of inspiratory muscle training in patients with obstructive sleep apnoea syndrome: a systematic review and meta-analysis. Sleep Sci. (2022) 15(4):480–9. doi: 10.5935/1984-0063.20220081
61. Miranda-Tueros M, Ramirez-Peña J, Cabanillas-Lazo M, Paz-Ibarra JL, Pinedo-Torres I. Effects of aerobic exercise on components of the metabolic syndrome in older adults with type 2 diabetes mellitus: systematic review and meta-analysis. Rev Peru Med Exp Salud Publica. (2024) 41(2):146–55. doi: 10.17843/rpmesp.2024.412.12751
62. Al-Mhanna SB, Rocha-Rodrigues S, Mohamed M, Batrakoulis A, Aldhahi MI, Afolabi HA, et al. Effects of combined aerobic exercise and diet on cardiometabolic health in patients with obesity and type 2 diabetes: a systematic review and meta-analysis. BMC Sports Sci Med Rehabil. (2023) 15(1):165. doi: 10.1186/s13102-023-00766-5
63. Wan Y, Su Z. The impact of resistance exercise training on glycemic control among adults with type 2 diabetes: a systematic review and meta-analysis of randomized controlled trials. Biol Res Nurs. (2024) 26(4):597–623. doi: 10.1177/10998004241246272
64. Feng J, Zhang Q, Chen B, Chen J, Wang W, Hu Y, et al. Effects of high-intensity intermittent exercise on glucose and lipid metabolism in type 2 diabetes patients: a systematic review and meta-analysis. Front Endocrinol (Lausanne). (2024) 15:1360998. doi: 10.3389/fendo.2024.1360998
65. Batrakoulis A. Psychophysiological adaptations to yoga practice in overweight and obese individuals: a topical review. Diseases. (2022) 10(4):107. doi: 10.3390/diseases10040107
66. Batrakoulis A. Psychophysiological adaptations to pilates training in overweight and obese individuals: a topical review. Diseases. (2022) 10(4):71. doi: 10.3390/diseases10040071
67. Dall'Ago P, Chiappa GR, Guths H, Stein R, Ribeiro JP. Inspiratory muscle training in patients with heart failure and inspiratory muscle weakness. J Am Coll Cardiol. (2006) 47:757–63. doi: 10.1016/j.jacc.2005.09.052
68. Basso-Vanelli RP, Di Lorenzo VAP, Labadessa IG, Regueiro EM, Jamami M, Gomes EL, et al. Effects of inspiratory muscle training and calisthenics-and-breathing exercises in COPD with and without respiratory muscle weakness. Respir Care. (2016) 61(1):50–60. doi: 10.4187/respcare.03947
69. Kabitz H-J, Sonntag F, Walker D, Schwoerer A, Walterspacher S, Kaufmann S, et al. Diabetic polyneuropathy is associated with respiratory muscle impairment in type 2 diabetes. Diabetologia. (2007) 51:191–7. doi: 10.1007/s00125-007-0856-0
70. Cho JE, Lee HJ, Kim MK, Lee WH. The improvement in respiratory function by inspiratory muscle training is due to structural muscle changes in patients with stroke: a randomized controlled pilot trial. Top Stroke Rehabil. (2018) 25(1):37–43. doi: 10.1080/10749357.2017.1383681
71. Britto RR, Rezende NR, Marinho KC, Torres JL, Parreira VF, Teixeira-Salmela LF. Inspiratory muscular training in chronic stroke survivors: a randomized controlled trial. Arch Phys Med Rehabil. (2011) 92(2):184–90. doi: 10.1016/j.apmr.2010.09.029
72. Ribeiro JP, Chiappa GR, Callegaro CC. The contribution of inspiratory muscles function to exercise limitation in heart failure: pathophysiological mechanisms Contribuição da musculatura inspiratória na limitação ao exercício na insuficiência cardíaca: mecanismos fisiopatológicos. Rev Bras Fisioter. (2012) 16(4):261–8. doi: 10.1590/S1413-35552012005000034
73. Callegaro CC, Ribeiro JP, Tan CO, Taylor JA. Attenuated inspiratory muscle metaboreflex in endurance-trained individuals. Respir Physiol Neurobiol. (2011) 177(1):24–9. doi: 10.1016/j.resp.2011.03.001
74. Lötters F, Van Tol B, Kwakkel G, Gosselink R. Effects of controlled inspiratory muscle training in patients with COPD: a meta-analysis. Eur Respir J. (2002) 20(3):570–7. doi: 10.1183/09031936.02.00237402
75. Pehlivan E, Mutluay F, Balcı A, Kılıç L. The effects of inspiratory muscle training on exercise capacity, dyspnea and respiratory functions in lung transplantation candidates: a randomized controlled trial. Clin Rehabil. (2018) 32(10):1328–39. doi: 10.1177/0269215518777560
76. Human A, Corten L, Jelsma J, Morrow B. Inspiratory muscle training for children and adolescents with neuromuscular diseases: a systematic review. Neuromusc Disord. (2017) 27(6):503–17. doi: 10.1016/j.nmd.2017.03.009
77. Nepomuceno BRV, Barreto MDS, Almeida NC, Guerreiro CF, Xavier-Souza E, Neto MG. Safety and efficacy of inspiratory muscle training for preventing adverse outcomes in patients at risk of prolonged hospitalisation. Trials. (2017) 18:626. doi: 10.1186/s13063-017-2372-y
78. Lista-Paz A, Cousillas LB, Jacome C, Fregonezi G, Labata-Lezaun N, Llurda-Almuzara L, et al. Effect of respiratory muscle training in asthma: a systematic review and meta-analysis. Ann Phys Rehabil Med. (2023) 66:101691. doi: 10.1016/j.rehab.2022.101691
79. Luo Z, Qian H, Zhang X, Wang Y, Wang J, Yu P. Effectiveness and safety of inspiratory muscle training in patients with pulmonary hypertension: a systematic review and meta-analysis. Front Cardiovasc Med. (2022) 9:999422. doi: 10.3389/fcvm.2022.999422
80. Freeman J. Management of hypoglycemia in older adults with type 2 diabetes. Postgrad Med. (2019) 131(4):241–50. doi: 10.1080/00325481.2019.1578590
81. Kjaer M, Hollenbeck CB, Frey-Hewitt B, Galbo H, Haskell W, Reaven GM. Glucoregulation and hormonal responses to maximal exercise in non-insulin-dependent diabetes. (1990) 68(5):2067–74. doi: 10.1152/JAPPL.1990.68.5.2067
82. Bhandari ANIT, Xia YING, Cortright RONALD, Dohm GL, Bazzy AR. Effect of respiratory muscle training on GLUT-4 in the sheep diaphragm. Med Sci Sports Exerc. (2000) 32(8):1406–11. doi: 10.1097/00005768-200008000-00008
Keywords: glucose, respiratory muscle training, autonomic nervous system, exercise capacity, hemodynamic
Citation: Breuil-Marsal Z, Godek C, Lotti A, Feiereisen P, Marçal IR, Rehder-Santos P, Milan-Mattos JC and Abreu RMd (2024) Acute and chronic effects of inspiratory muscle training in patients with type 2 diabetes mellitus: a systematic review of randomized controlled trials. Front. Sports Act. Living 6:1423308. doi: 10.3389/fspor.2024.1423308
Received: 2 August 2024; Accepted: 15 November 2024;
Published: 11 December 2024.
Edited by:
Hassane Zouhal, University of Rennes 2—Upper Brittany, FranceReviewed by:
Bruce Rogers, University of Central Florida, United StatesCopyright: © 2024 Breuil-Marsal, Godek, Lotti, Feiereisen, Marçal, Rehder-Santos, Milan-Mattos and Abreu. This is an open-access article distributed under the terms of the Creative Commons Attribution License (CC BY). The use, distribution or reproduction in other forums is permitted, provided the original author(s) and the copyright owner(s) are credited and that the original publication in this journal is cited, in accordance with accepted academic practice. No use, distribution or reproduction is permitted which does not comply with these terms.
*Correspondence: Raphael Martins de Abreu, cmFwaGFlbG1hcnRpbnMuYWJyZXVAZ21haWwuY29t
Disclaimer: All claims expressed in this article are solely those of the authors and do not necessarily represent those of their affiliated organizations, or those of the publisher, the editors and the reviewers. Any product that may be evaluated in this article or claim that may be made by its manufacturer is not guaranteed or endorsed by the publisher.
Research integrity at Frontiers
Learn more about the work of our research integrity team to safeguard the quality of each article we publish.