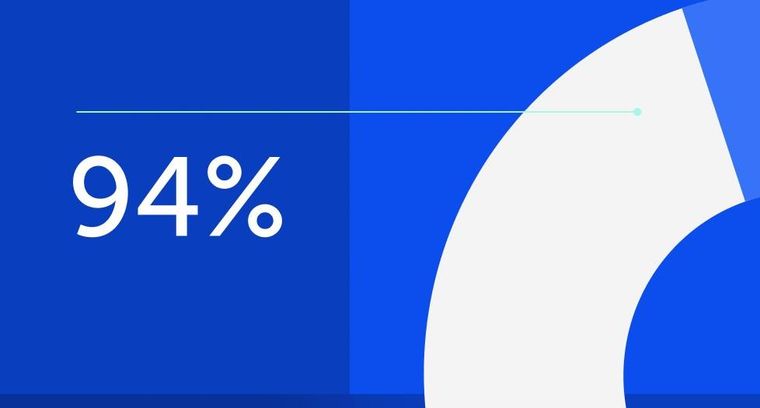
94% of researchers rate our articles as excellent or good
Learn more about the work of our research integrity team to safeguard the quality of each article we publish.
Find out more
BRIEF RESEARCH REPORT article
Front. Sports Act. Living, 06 February 2024
Sec. Biomechanics and Control of Human Movement
Volume 6 - 2024 | https://doi.org/10.3389/fspor.2024.1352725
Studies previously conducted on high jump have yielded important information regarding successful performance. However, analyses in competitive scenarios have often disregarded athletes’ unsuccessful attempts. This study aimed to investigate the biomechanical differences between successful and unsuccessful jumps during competition. High-speed video footage (200 Hz) was obtained from 11 athletes during the 2018 Men's World Athletics Indoor Championship Final. From each athlete, one successful (SU) and one unsuccessful (UN) jump at the same bar height were included in the analysis, leaving seven athletes in total. Following whole-body 3D manual digitization, several temporal and kinematic variables were calculated for the run-up, take-off, and flight phases of each jump. During SU jumps, athletes raised the center of mass to a greater extent (p < 0.01) from take-off. Touchdown in SU jumps was characterized by a faster anteroposterior velocity (p < 0.05), lower backward lean (p < 0.05), and changes in joint angles for the stance and trail limbs (p < 0.05). Athletes also shortened the final contact time during SU jumps (p < 0.01) after producing a longer flight time in the final step of the run-up (p < 0.05). Elite-level high jumpers undertake a series of adjustments to successfully clear the bar after UN jumps. These adjustments reinforce the importance of the run-up in setting the foundations for take-off and bar clearance. Furthermore, the findings demonstrate the need for coaches to be mindful of the adjustments required in stance and trail limbs when looking to optimize feedback to athletes during training and competition.
The main objective of the high jump event is for the jumper to raise their center of mass (CM) to a maximum height while crossing the bar. As the high jump event has been contested since the first modern Olympiad, it is not surprising that a great number of techniques have been adopted in order to achieve this (1). Although almost all modern high jumpers utilize the Fosbury Flop technique (2), this technique still allows a wide range of technical variations compared to other jumps. There are a wealth of biomechanical data available for the high jump, which describes the run-up run, take-off (TO), and flight phases (3–7); this forms the basis of current technical models used by coaches to develop strategies to achieve maximum technical efficiency.
Analyses of the high jump have mainly been conducted during international competitions to describe the performances of the best athletes. The advantage of conducting analyses during official competitions is the increased ecological validity, both in terms of the physical environment as well as the motivation of athletes and pressure of competitive situations. Biomechanical analyses of the high jump have highlighted key performance determinants that provide key reference points for coaches to build their technical models and can be used by athletic federations to build their monitoring and talent identification programs. Several authors have stated that the take-off is the most important phase of the high jump (5, 8, 9) with the peak height of the CM during flight being heavily dependent on the height and vertical velocity of the CM at take-off. Although a great deal of attention has rightly been focused on the critical determinants of these two take-off parameters (10–12), a successful bar clearance based on an optimal supine layout position at the peak of the jump is a function of several run-up, take-off, and flight characteristics. Indeed, it has recently been demonstrated (13) that modern athletes make use of hip–shoulder rotations during the take-off phase to generate long axis rotation and enable the athlete to move from a forward-facing take-off position to a supine bar clearance position. The characteristics of the high jump undoubtedly necessitate high levels of reactive strength, lower-body stiffness, and flexibility (14, 15), but it is the technical complexity of the event which dictates that successful jumps offer a limited window for errors in execution.
Athletes in jump events are known to make small modifications in their movement patterns as they adjust their technique in response to external feedback to ensure a consistent take-off point (16). These adjustments might provide useful compensatory changes ensuring a consistent performance outcome but can also introduce a level of dysfunctional variability that may lead to unsuccessful jumps (17). Analyses of unsuccessful high jumps could yield important information about the factors contributing to suboptimal performance. While successful (SU) and unsuccessful (UN) jump comparisons have been undertaken for other events (18), key performance parameters in the high jump have mainly been identified by comparing SU jumps of different heights (6, 9, 19). As such, limited information exists on the magnitude and type of changes made by athletes to bring about successful bar clearance following an unsuccessful jump. Considering that modern, world-class high jumpers display great technical variation in jump styles (6, 7), some degree of inter-individual variability in the adjustments made following UN jumps may be expected (3, 20). Nevertheless, it would be useful to understand the extent to which modern, world-class high jumpers display common and significant differences between SU and UN jumps. In particular, information on the changes that occur in the temporal and kinematic characteristics of the run-up, take-off, and flight would be useful to inform coaches in the design of corrective strategies relating to bar clearance.
At present, there is limited information that compares the differences between SU and UN high jumps during competition, particularly in world-class performers. This information is needed to better understand the adjustments made by world-class jumpers to achieve bar clearance after UN jumps. There is limited research across all standards of high jump athletes; however, research into the highest level of performers can be used by coaches as models of excellence to inform their technical and conditioning strategies. Thus, the aim of this study was to investigate the kinematic differences between SU and UN jumps in the men's high jump final of the 2018 World Indoor Athletics Championships. The purpose of the study was to provide coaches with information on the most important factors determining successful bar clearance, which can be used to optimize feedback to athletes between jumps and thus inform corrective strategies in technique.
Data were collected as part of the Birmingham 2018 IAAF World Indoor Championships Biomechanics Projects. The collection and use of the data was approved by World Athletics (the owner and controller of the data) and locally approved through institutional research ethics procedures (Leeds Beckett University Ethics Sub-committee; application reference: 61250). Jumps from seven male world-class high jumpers were analyzed (body mass: 76.8 ± 7.2 kg; body height: 1.94 ± 0.04 m; personal best: 2.32 ± 0.03 m). To address the aim of the study, only athletes (seven of the 11 finalists) performing an SU and a UN attempt at the same bar height were analyzed. This height (2.25 ± 0.06 m) was not the same for all athletes as it was dictated by individual performances.
Four high-speed cameras (Sony PXW-FS5) operating at 200 Hz (shutter speed: 1/1,250 s; ISO: 2,000–4,000; FHD: 1,920 × 1,080 pixels; progressive scan) were used to record the high jump action during the men's final competition commencing three steps before take-off and ending when the athlete had landed. A standardized calibration procedure was conducted before and after the competition using a rigid cuboid calibration frame measuring 3.044 m3 and comprising 24 reference points (7). The recorded video files were imported into a 3D movement analysis software (SIMI Motion version 9.2.2, Simi Reality Motion Systems GmbH, Germany) with one SU attempt and one UN attempt for each athlete then being manually digitized by a single experienced operator. An event synchronization technique (synchronization of four critical instants) was applied through SIMI Motion to synchronize the two-dimensional coordinates from each camera involved in the recording. The digitizing involved a continuous whole-body analysis throughout the take-off and flight phases of each jump. A 17-point whole-body model was digitized beginning three steps from the final take-off position and ending following complete bar clearance by each athlete. In accordance with de Leva (21), the 17 digitized points were the center of the head and bilaterally shoulder, elbow, wrist, metacarpophalangeal, hip, knee, ankle, and metatarsophalangeal (MTP) joint center. Each file was first digitized frame by frame, and upon completion, adjustments were made as necessary using the points over frame method (22). The reliability of the digitizing process showed minimal total errors (intraclass correlation coefficient >0.97) when it was repeated for specific variables for five randomly selected athletes with an intervening period of 48 h. The Direct Linear Transformation (DLT) algorithm (23) was used to reconstruct the real-world 3D coordinates from individual camera's x and y image coordinates. de Leva’s (21) body segment parameter models were used to obtain data for the whole-body CM and for key body segments. A recursive second-order, low-pass Butterworth digital filter (zero phase lag) was employed to filter the outcome variable with a 7–15 Hz cut-off frequency range determined through residual analysis (Winter, 2009).
Following data processing, a number of key (6, 7, 13) kinematic variables were computed to characterize the run-up, take-off, and flight phases of each athlete's jump (Table 1). The contact and flight phases of the final three steps of the run-up were categorized in an incremental order with 1 and 2 being the take-off and penultimate contacts, respectively. Athlete's body heights were obtained (24) and used to scale a number of linear displacement (horizontal and vertical) variables to account for differences in stature. In line with previous analyses (13), shoulder–hip separation angles were calculated using a custom-written Matlab script (version R2021b, MathWorks, Inc., Natick, MA, USA).
Results are reported as means ± standard deviations (SD). All statistical analyses were carried out using SPSS Statistics 26 (IBM SPSS, Inc., Chicago, IL, USA) and distribution parameters were used to check the appropriateness of parametric tests. Paired samples t-tests were then used to quantify the differences between SU and UN jumps; significance was set at p < 0.05 (25). Cohen's d (26) was used as an effect size to determine the magnitude of differences between successful and unsuccessful jumps with interpretation thresholds of 0.2 (small), 0.5 (medium), 0.8 (large), 1.2 (very large), and 2.0 (huge).
Concerning the velocity-related variables (Table 2), a significant difference was observed in anteroposterior CM velocity at touchdown (TD) (p < 0.05, d = 0.015) with the SU jumps demonstrating a 6.3 ± 5.1% faster velocity than the UN jumps. No other differences were observed for any of the velocity-related variables. For the spatiotemporal variables (Table 2), a significant difference was observed in ground contact time (CT) at take-off (p < 0.01, d = 0.835) and at contact 4 (p < 0.05, d = 0.847) during the run-up. Specifically, the UN attempts were characterized by a 6.2 ± 3.2% and 5.1 ± 3.3% longer contact time than the SU attempts at contact 4 (initiation of the third step before take-off) during the run-up and at take-off, respectively. The longer contact time at take-off in the UN attempts was preceded by a significantly shorter flight time (FT) (18.8 ± 15.4%, p < 0.05, d = 0.847) as well as a significantly lower FT:CT ratio in the final (take-off) step (22.5 ± 16.1%, p < 0.05, d = 0.917). The longer contact time at take-off was also characterized by a longer duration of knee extension (19.4 ± 29.2%, d = 0.826) during UN attempts, although this large effect did not reach statistical significance.
Table 2. Kinematic and spatiotemporal characteristics of the run-up and take-off for SU and UN attempts.
Regarding the postural characteristics of the take-off phase for the SU and UN attempts (Table 3), a significantly longer CM–foot distance at TD was observed for the UN attempts (4.2 ± 4.2%, p < 0.05, d = 0.803). A number of significant differences were also observed for the trail leg at TD, with the knee joint being more extended (11.2 ± 4.1%, p < 0.01, d = 1.232) and the hip joint more flexed (3.9 ± 3.8%, p < 0.05, d = 0.918) in the UN attempts. The UN attempts also demonstrated a significantly greater peak vertical thigh velocity for the trail leg at TD (9.3 ± 7.0%, p < 0.05, d = 1.099; Table 3). Differences in the take-off leg were not significant, although there was a tendency toward a difference in ankle joint angle at TD (p = 0.07, 4.6 ± 5.5%, d = 1.028). Specifically, despite not significant, the UN attempts were characterized by a seemingly more plantar-flexed ankle joint at TD but a higher degree of dorsiflexion during the take-off phase (Table 3). There were some very large individual changes in shoulder–hip separation for some athletes; however, the variables were characterized by notable individual variation in execution between athletes.
With respect to the CM and pelvis positioning during the take-off and flight phases (Table 4), no significant differences in peak CM height were detected between the attempts. From the perspective of the run-up, no differences in CM height were observed at TD but the UN attempts displayed a significantly greater CM height at TO (p < 0.05, d = 0.326). In relation to the subsequent flight phase, a significant difference in CM raise height was then observed (p < 0.01, d = 0.716) with the SU attempts raising the CM a greater vertical distance from the take-off position.
Table 4. Center of mass and pelvis positioning during the take-off and flight phases for SU and UN attempts.
The purpose of this study was to compare the biomechanical characteristics of SU and UN attempts during a major international competition in elite male high jumpers. Athletes displayed significant biomechanical differences in temporal and kinematic variables between SU and UN jumps identified in the run-up as well as during take-off and flight phases. During SU jumps, athletes raised the CM to a greater extent from take-off. This could be related to a reduction in backward lean at the final touchdown and changes in positioning and angular kinematics of the stance and trail limbs as well as to changes in the temporal characteristics of the run-up. For some biomechanical variables, individual variability across the athletes was displayed in the comparisons between SU and UN; this likely reflects the differences in the technical models adopted by the world-class high jumpers and the high number of degrees of freedom in a complex movement like high jump.
Given that the objective of the high jump is for the jumper to raise their CM to a maximal height to facilitate clearance of the bar, it was not surprising that differences between SU and UN jumps were apparent in the flight phase of the jumps. Although the peak height of the CM was not significantly higher in the SU jumps, the jumpers displayed a lower CM height at TO during SU jumps (1.35 vs. 1.37 m, p < 0.05) and subsequently managed to raise the CM a greater vertical distance up to the apex of the jump (0.97 vs. 0.93 m, p < 0.01, d = 0.716). This suggests that athletes were more able to utilize a maximal vertical propulsion during the take-off phase or that the take-off actions (including swing actions by the trail leg and arms) created more efficient rotations around the CM.
The interdependency of biomechanical variables in the high jump is well known (13) with the run-up and take-off phases laying down the foundations for the flight phase. Research has stated that the take-off is the most important phase (8) with a number of “key” variables often being the focus of attention (e.g., horizontal velocity, knee angle, CM height). Indeed, vertical velocity at take-off is known to be a major determinant for maximizing vertical CM displacement during flight (8) and a linear relationship has previously been reported (11) between run-up speed and jump height when analyzing the best jump of each athlete. The comparison of UN and SU jumps in the present study showed some large individual differences in velocity (at take-off and touchdown) but also considerable individual variability between athletes with SU jumps not always being characterized by faster run-up speeds or higher vertical take-off velocities. A notable finding, however, was the significantly higher anteroposterior velocity at TD in the SU jumps (5.31 vs. 4.98 m/s, p < 0.05), which was not apparent when the anteroposterior and mediolateral velocities were combined as resultant horizontal velocity. A degree of caution is required when comparing SU and UN jumps is that the observed differences may reflect the correction of “errors” that were made during UN jumps or simply that the athlete correctly performed the appropriate movement pattern during SU jumps. Whichever the scenario, it is clear that athletes ran up in the SU trials in a manner where they were traveling at a faster velocity in the direction of the landing mat at the start of the take-off phase. The faster anteroposterior velocity at TD is likely linked to the significantly shorter CM–foot distance at TD (0.77 vs. 0.80 m, p < 0.05, d = 0.803) in the SU jumps, which demonstrates a reduced backward lean, placing the athlete in a favorable position to generate lower braking impulses during the initial stages of the take-off phase. Indeed, it is known that inward and backward leaning body positions are pre-planned strategies used by jumpers to counterbalance the forward pull of inertia, control twisting/somersaulting rotation, and to increase the time spent applying force to the ground (27). It becomes clear that athletes subsequently underwent modifications in their take-off technique during SU jump, which likely resulted from their more upright body position at TD. This was apparent in a number of kinematic and temporal variables with the athletes significantly shortening their (final) ground contact time (0.169 s vs. 0.178 s, p < 0.01, d = 0.835) during SU trials, tending to land with a more dorsiflexed ankle (120.96° vs. 126.48°, p = 0.070, d = 1.028) and tending to require less time to undertake knee extension during the take-off phase (0.068 vs. 0.080 s, p = 0.120, d = 0.826).
While there has often been a focus on the positioning and action of the take-off leg within studies that have analyzed only successful jumps (9, 19), the present findings highlight that SU jumps are also characterized by significant and large changes in the trail leg during the take-off phase. Specifically, the trail leg was shown to be significantly less extended at the knee (100.00° vs. 111.19°, p < 0.01, d = 1.232) and significantly less flexed at the hip (166.90° vs. 160.39°, p < 0.05, d = 0.918) at TD in the SU attempts with the trail leg thigh segment also displaying a significantly lower peak vertical velocity during SU attempts (5.83 vs. 6.37 m/s, p < 0.05, d = 1.099). The large effect sizes demonstrate the importance of the actions performed by the trail leg, which functions as a swing element during take-off sequentially synchronized with the swinging action of the arms (28). One explanation for the need of higher vertical velocities of the trail leg thigh during take-off could be the possibly larger braking impulses in UN jumps, which would require the jumper to rely on creating higher vertical impulses with the swing elements during the take-off. The present findings, therefore, highlight that world-class high jumpers undertake simultaneous adjustments in both the take-off as well as in the trail leg to achieve bar clearance after UN jumps and that their timely coherent work is clearly of high importance for an optimal take-off execution. Given that most of the differences between SU and UN during the take-off phase were observed at TD and not TO, it seems logical that some of these differences may have been compensatory changes in response to external feedback designed to maintain consistent take-off conditions (e.g., velocity, angle). While the take-off conditions maintained a level of consistency, there were common and significant differences between SU and UN jumps, which may have represented a level of dysfunctional variability that led to a change in performance outcome (17). Although it is clear that the differences between SU and UN jumps were greatest during the take-off phase, the influence of the run-up also requires attention since its purpose is to set appropriate conditions for landing, flight, and bar clearance (29). While the athletes displayed a consistent path of run-up during SU and UN jumps, some athletes displayed larger changes in take-off distance (up to 16 cm), but these were not consistent across all athletes. The movement execution required following an UN jump is likely dependent on the technical approach adopted, and the large variation in some biomechanical variables without doubt reflects the great variation that modern world-class high jumpers display in their approach to the event (6, 7).
In terms of the common differences observable in the run-up, athletes displayed significant differences in the pattern of the final four run-up steps with the SU attempts displaying a shorter fourth (p < 0.05, d = 0.847) and final (i.e., take-off) ground contact (p < 0.01, d = 0.835). Despite the shorter final ground contact time (take-off time), the SU jumps showed a tendency for athletes to lengthen their last step prior to take-off, which resulted in a significantly longer flight time (p < 0.05, d = 0.847) and larger flight time:contact time ratio (0.24 vs. 0.31, p < 0.05, d = 0.917). These observations together with the higher anteroposterior velocity and differences in joint kinematics for both take-off and trail leg at TD as well as slightly slower longer contact time at second contact (contact prior take-off) could indicate a poorer execution of the step prior to the take-off in the UN jumps. Despite not reaching statistical significance, the ankle and knee on the take-off leg demonstrated a lower minimal angle for the UN jumps (Table 3) which alongside the longer CM–foot distance at TD may indicate larger braking impulses, which were not effectively counteracted/overcome. The differences observed in the final stages of the run-up likely influenced the joint loading at the start of take-off and the subsequent work required by the stance and trail limb in optimizing vertical propulsion. The athletes, therefore, were able to position themselves in a more beneficial body configuration for executing the take-off in the SU jump and to lift their CM to a greater vertical distance up to the apex of the jump. Thus, coaches and athletes should be mindful that differences between SU and UN jumps in world-class high jumpers manifest in the run-up and that modifications in the execution of final strides can allow the desired take-off conditions and performance outcomes. This knowledge can be used to inform analysis and corrective strategies and may be used when designing technical models that facilitate reproducible performance outcomes.
The main strength of the current study is that the data are of world-class high jump athletes (30) competing in World Championship finals; therefore, the research has high ecological validity and the results can be used by coaches as a model of excellence. One limitation is the limited sample size and the homogenous but largely individually variable nature of the sample (i.e., elite male jumpers), which may have influenced the differences that were observed. Moreover, the study is limited by the analysis of only one SU and UN trial per athlete, which is a challenge of in-competition analyses. Future data collection involving a larger, more heterogenous sample and several trials per athlete across multiple heights may help explain the factors influencing high jump success further.
The present findings characterize the differences between successful and unsuccessful jumps in elite-level male high jumpers. The elite athletes displayed notable individual variability between successful and unsuccessful jumps, which was likely dependent on the individual's technical approach and the implementation of this. Despite the individual variability, the athletes demonstrated several common and significant biomechanical differences between successful and unsuccessful jumps, which started in the run-up and continued into the take-off and flight phases. In converting unsuccessful trials to successful bar clearances, athletes particularly demonstrated modifications in run-up velocity, backward lean, take-off phase duration, and in positioning of the trail leg. These kinematic variables should be the focus of future studies that compare successful and unsuccessful high jump performances. Furthermore, coaches can utilize these findings to optimize feedback during training and competition when considering corrective strategies.
The raw data supporting the conclusions of this article will be made available by the authors, without undue reservation.
The studies involving human participants were reviewed and approved by Carnegie School of Sport Research Ethics Committee, Leeds Beckett University, The patients/participants provided their written informed consent to participate in the study.
GN: Conceptualization, Data curation, Formal Analysis, Investigation, Methodology, Software, Writing – original draft, Writing – review & editing. GE: Conceptualization, Writing – original draft, Writing – review & editing. SM: Conceptualization, Funding acquisition, Investigation, Project administration, Writing – original draft, Writing – review & editing. JW: Conceptualization, Data curation, Investigation, Methodology, Software, Writing – original draft, Writing – review & editing. AB: Conceptualization, Data curation, Funding acquisition, Investigation, Methodology, Project administration, Software, Writing – original draft, Writing – review & editing.
The authors declare financial support was received for the research, authorship, and/or publication of this article.
The data collection and initial data analysis were supported by funding provided by the IAAF/World Athletics as part of a wider development/education project; however, the nature of the data is purely descriptive and not associated with any governing body, commercial sector, or product. The results of the present study do not constitute endorsement by World Athletics.
The authors declare that the research was conducted in the absence of any commercial or financial relationships that could be construed as a potential conflict of interest.
All claims expressed in this article are solely those of the authors and do not necessarily represent those of their affiliated organizations, or those of the publisher, the editors and the reviewers. Any product that may be evaluated in this article, or claim that may be made by its manufacturer, is not guaranteed or endorsed by the publisher.
2. Dapena J. The evolution of high jumping technique: biomechanical analysis. ISBS-Conference Proceedings Archive; July 18–22; Tsukuba, Japan. International Society of Biomechanics in Sports (2002).
3. Ritzdorf W, Conrad A, Loch M. Intra-individual comparison of the jumps of Stefka Kostadinova at the II World Championships in athletics Rome 1987 and the games of the XXIV Olympiad Seoul 1988. N Stud Athel. (1989) 4(4):35–41.
4. Antekolovic L, Blazevic I, Mejovsek M, Coh M. Longitudinal follow-up of kinematic parameters in the high jump—a case study. N Stud Athel. (2006) 21(4):27–37.
5. Coh M, Supej M. Biomechanical model of the take-off action in the high jump—a case study. N Stud Athel. (2008) 23(4):63–73.
6. Nicholson G, Bissas A, Merlino S. Biomechanical report for the IAAF world championships 2017: high jump men’s. In: 2017 IAAF World Championships Biomechanics Research Project, July 2018, UK. International Association of Association of Athletics Federations (2018). Available online at: https://www.iaaf.org/about-iaaf/documents/research (accessed May 5, 2023).
7. Nicholson G, Bennett TD, Bissas A, Merlino S. Biomechanical Report for the IAAF World Indoor Championships 2018: High Jump Men. Birmingham: International Association of Athletics Federations (2019). Available online at: https://www.iaaf.org/about-iaaf/documents/research (accessed May 5, 2023).
8. Dapena J. Scientific Services Project—HIGH JUMP. Biomechanics Laboratory, Dept. of Kinesiology, Indiana University (2006).
9. Pavlović R. The differences of kinematic parameters high jump between male and female finalists world championship Daegu, 2011. Turk J Kinesiol. (2017) 3(4):60–9.
10. Alexander R. Optimal take-off techniques for high and long jumps. Philos Trans Biol Sci. (1990) 329(1252):3–10. doi: 10.1098/rstb.1990.0144
11. Dapena J, McDonald C, Cappaert J. A regression analysis of high jumping technique. Int J Sport Biomech. (1990) 6:246–61. doi: 10.1123/ijsb.6.3.246
12. Grieg MP, Yeadon MR. The influence of touchdown parameters on the performance of a high jumper. J Appl Biomech. (2000) 16(4):367–78. doi: 10.1123/jab.16.4.367
13. Nicholson G, Jongerius N, Tucker CB, Thomas A, Merlino S, Bissas A. The association between hip-shoulder separation angles and technique characteristics in world-class high jumpers. Front Sports Act Living. (2022) 4:873526. doi: 10.3389/fspor.2022.873526
14. Ridzdorf W. Approaches to technique and technical training in the high jump. N Stud Athel. (2009) 24(3):31–4.
15. Boden E, Braunstein B, Heinrich K, Sanno M, Stäudle B, Ritzdorf W, et al. Leg- and joint stiffness in male elite high jump: the influence of stiffness on sports performance. ISBS Proc Arch. (2017) 35(1):150.
16. Needham L, Bezodis I, Exell T, Irwin G. Pole-athlete interaction during the pole vault approach phase. ISBS Proc Arch. (2017) 35(1):781–4.
17. Theodorou Α, Panoutsakopoulos V, Exell T, Vujkov N. Comparison of step characteristic interaction and asymmetry between failed and successful attempts in pole vault. ISBS Proc Arch. (2017) 35(1):777–80.
18. Cassirame J, Sanchez H, Exell TA, Panoutsakopoulos V, Theodorou AS, Homo S, et al. Differences in approach run kinematics: successful vs. unsuccessful jumps in the pole vault. Int J Perform Anal Sport. (2019) 19(5):794–808. doi: 10.1080/24748668.2019.1657655
19. Ae M, Nagahara R, Ohshima Y, Koyama H, Takamoto M, Shibayama K. Biomechanical analysis of the top three male high jumpers at the 2007 world championships in athletics. N Stud Athel. (2008) 23(2):45–52.
20. Panoutsakopoulos V, Kollias I. 3-D biomechanical analysis of women’s high jump. N Stud Athel. (2012) 27(3):31–48.
21. de Leva P. Adjustments to Zatsiorsky–Seluyanov’s segment inertia parameters. J. Biomech. (1996) 29:1223–30. doi: 10.1016/0021-9290(95)00178-6
22. Bahamonde RE, Stevens RR. Comparison of Two Methods of Manual Digitization on Accuracy and Time of Completion. Salzburg, Austria: ISBS (2006).
23. Abdel-Aziz YI, Karara HM. Direct Linear Transformation from Comparator Coordinates into Object Space Coordinates in Close-Range Photogrammetry. Falls Church, VA: American Society of Photogrammetry (1971).
26. Cohen J. Statistical Power Analysis for the Behavioural Sciences. 2nd ed. Hillsdale, NJ: Lawrence Erlbaum (1988).
27. Dapena J. Contributions of angular momentum and catting to the twist rotation in high jumping. J Appl Biomech. (1997) 13:239–53. doi: 10.1123/jab.13.2.239
28. Lees A, Rojas J, Ceperos M, Soto V, Gutierrez M. How the free limbs are used by elite high jumpers in generating vertical velocity. Ergonomics. (2000) 43(10):1622–36. doi: 10.1080/001401300750004041
29. Dapena J, Chung CS. Vertical and radial motions of the body during the take-off phase of high jumping. Med Sci Sports Exerc. (1988) 20:290–302. doi: 10.1249/00005768-198806000-00014
Keywords: kinematics, biomechanics, videography, coaching, competition
Citation: Nicholson G, Epro G, Merlino S, Walker J and Bissas A (2024) Differences in run-up, take-off, and flight characteristics: successful vs. unsuccessful high jump attempts at the IAAF world championships. Front. Sports Act. Living 6:1352725. doi: 10.3389/fspor.2024.1352725
Received: 8 December 2023; Accepted: 18 January 2024;
Published: 6 February 2024.
Edited by:
Adamantios Arampatzis, Humboldt University of Berlin, GermanyReviewed by:
Lida Mademli, Aristotle University of Thessaloniki, Greece© 2024 Nicholson, Epro, Merlino, Walker and Bissas. This is an open-access article distributed under the terms of the Creative Commons Attribution License (CC BY). The use, distribution or reproduction in other forums is permitted, provided the original author(s) and the copyright owner(s) are credited and that the original publication in this journal is cited, in accordance with accepted academic practice. No use, distribution or reproduction is permitted which does not comply with these terms.
*Correspondence: Gareth Nicholson Zy5uaWNob2xzb25AbGVlZHNiZWNrZXR0LmFjLnVr
Disclaimer: All claims expressed in this article are solely those of the authors and do not necessarily represent those of their affiliated organizations, or those of the publisher, the editors and the reviewers. Any product that may be evaluated in this article or claim that may be made by its manufacturer is not guaranteed or endorsed by the publisher.
Research integrity at Frontiers
Learn more about the work of our research integrity team to safeguard the quality of each article we publish.