- 1Department of Nursing, Faculty of Health Science, Ariel University, Ariel, Israel
- 2Department of Pediatric Rehabilitation, The Edmond and Lily Safra Children’s Hospital, The Chaim Sheba Medical Center, Tel Hashomer, Ramat-Gan, Israel
- 3Sackler Faculty of Medicine, Tel Aviv University, Tel-Aviv, Israel
Introduction: Acquired brain injury (ABI) is a prevalent diagnosis in pediatric rehabilitation. Gross motor skills are often affected by ABI and limit the ability to participate in various physical activities. However, as ABI injury location is diverse, children and adolescents (youth) with localized ABI, such as ABI in the posterior fossa (ABI-PF) may present unique and different motor disabilities than youth with ABI on account of traumatic brain injury (TBI).
Aims: The aims of the study were: (1) to compare gross motor deficits in youth with TBI vs. ABI-PF; and (2) to compare two methods on scoring BOT2 to determine which is better for identifying motor deficits.
Methods: Participated in this study youth with TBI (N = 50) and ABI-PF (N = 30). Participants were tested on Bruininks-Oseretsky Test of Motor Proficiency-2nd Edition (BOT2) Upper-Limb Coordination, Balance, Strength, Running Speed and Agility, and Bilateral-Coordination subtests. Motor performance deficits were established using two-standard deviations (2SD) and age-equivalent methods. Between-group differences were assessed via independent t-tests and receiver operating characteristic curves (ROC).
Results: According to the 2SD method, motor deficits in the ABI-PF group ranged from 20% to 66.66%, whereas in the TBI group 8%–16%. According to the age-equivalent method, in the TBI and ABI-PF groups 40%–66.0% and 46.66%–76.66% of the youth presented motor deficits, respectively. Moreover, ROC analysis showed that motor performance deficits of both groups in all sub-scales except for Bilateral Coordination differed enough to result in medium area under the curve.
Conclusions: Motor deficits post-pediatric ABI are prevalent. In comparison to the TBI group, deficits are greater in the ABI-PF group. Moreover, compared to the 2SD method, the extent of motor deficiency is greater in the age-equivalent method. Therefore, using the later might provide a more valid classification of deficits in gross motor proficiency for youth post-ABI.
Introduction
Acquired brain injury (ABI) is a prevalent diagnosis in pediatric rehabilitation (1) with an incidence of 661–1,035 per 100,000 (2). ABI can be classified into traumatic and non-traumatic (1). Traumatic brain injury (TBI) occurs when a sudden trauma results in damage to the brain (e.g., car accidents, falls) (3). Non-TBI cases include, for example, stroke, hypoxic/ischemic brain damage, infectious diseases or toxicity, and brain tumors. Brain tumors are the most common disease group of solid tumors in childhood (4). Moreover, tumors are the second most common form of cancer in childhood and almost half of all pediatric brain tumors arise in the posterior fossa (PF) (5).
In ABI, motor disabilities are often considered a less pervasive problem than psychosocial and cognitive deficits (6). Accordingly, it has been reported that 56%–72% of children and adolescents (youth) with ABI were able to ambulate independently at the time of discharge from the hospital (7). However, advanced gross motor skills (e.g., balance, static and dynamic postural control, speed and agility, coordination, and strength) which are important for high-level gross motor activities such as hopping, running and jumping are often affected by ABI. These problems remain long-lasting deficits that affect the higher motor performance of youth for years after ABI (8) and limit their ability to participate in various physical activities (3). However, as the ABI injury location is diverse, youth with localized ABI, such as ABI in the PF (ABI-PF) may present unique and different motor disabilities than youth with TBI. More specifically, damage because of PF tumors is usually localized to the cerebellar area, and the cerebellum is connected to many cerebral areas and its input regulates the excitability of cerebral motor control areas. Therefore, lesions to the cerebellum will affect the execution of various movements, specifically rapid, timed, and spatial dependent (9). Damage to the cerebellum may also result in balance and associative motor learning difficulties (10). In contrast, in TBI, this type of specific cerebellar damage is much less common, and the pathology commonly involves white matter tract damage because of diffuse axonal injury (11) as well as localized damage to specific cortical areas, most commonly to the frontal lobes. Accordingly, in comparison to ABI-PF, in TBI, it is a common finding that youth will have a higher level of motor function. Differences in motor function between the two groups may be enhanced by high-grade malignant tumor-related chemotherapy in youth with tumor-related ABI (12).
Despite the increased survival of youth with brain tumors, studies investigating objective motor functioning in this population are scarce (13). For example, Piscione et al. (14) study revealed significant differences between youth with brain tumors and normative population data for body coordination and strength and agility. Varedi et al. (15) observed balance impairments in 48% of adult survivors of pediatric central nervous system tumors. Specifically, within the pediatric PF tumor survivors, Piscione et al. (14) reported vermis infiltration of the tumor as a risk factor for lower body coordination scores and chemo and radiotherapy for lower strength and agility scores. In a more recent study by Decock et al., among youth with PF tumors (N = 56), motor performance deficits at the beginning of rehabilitation ranged from 5.35% (upper limb mobility) to 26.78% (balance). At the end of the rehabilitation, the prevalence of motor performance deficits ranged from zero percent (upper limb mobility) to 8.92% (balance) (16).
Several outcome measures are used to assess advanced motor skills performance in youth post-ABI (1). One of the most used outcome measures is the Bruininks-Oseretsky Test of Motor Proficiency 2 (BOT2) (17). The BOT2 is an evaluative eight-subtest standardized measure tool that assesses gross and fine motor proficiency (i.e., Fine Motor Precision, Fine Motor Integration, Manual Dexterity, Bilateral Coordination, Balance, Running Speed and Agility, Upper-Limb Coordination, and Strength) in youth aged four to 21 years old. The test was acknowledged as a supplementary measure to the core outcome measures for the evaluation of youth who sustained ABI (18). BOT2 can be used in two different ways to detect motor deficits. The first method utilizes the various subtests' individual point scores. A cutoff point of two standard deviations below the mean is commonly used to establish the presence of motor performance deficits (19). The second method utilizes the different subtests' age equivalents calculations. According to this method, youth can be grouped according to the gap between their chronological age and their motor performance age equivalent. The two different evaluation methods may provide different results concerning the motor proficiency level of youth with ABI. However, to the best of our knowledge, no previous study compared motor proficiency tests, including BOT2, different scoring systems effect on the prevalence of motor proficiency problems. There is however much debate in the topic regrading which type of scoring system is more appropriate in other populations and assessment domains. For example, when assessing writing skills, it has been suggested that the reliability for age-equivalent scores is much poorer for advanced test-takers (20). Accordingly, many assessments do not report age or grade equivalents beyond a specified age or grade level. For example, in the Oral and Language Written Scales (21), age-equivalent scores are not reported after age 12 and grade-equivalent scores are reported only up to grade six. The acquisition of writing skills occurs most rapidly during the early years because writing mechanics are taught in the primary grades. The degree of discrimination among examinees with advanced writing skills is demonstrated by smaller changes in score points (22).
Considering the knowledge on different scoring systems in other domains, a better understanding of the motor proficiency of youth with ABI and the impact of different scoring systems on it is important. Clinicians and researchers can use the BOT2 to screen for motor impairment, determine the need for further assessment/intervention, develop and evaluate motor training programs and make placement decisions regarding physical education programs (3). This study was therefore undertaken to: (1) compare gross motor deficits in youth with TBI vs. ABI-PF, and (2) compare two methods on scoring BOT2 to determine which is better for identifying motor deficits.
Materials and methods
Participants
Included in this cross-sectional study were: (1) youth (males and females) diagnosed with moderate-to-severe ABI. Diagnosis of ABI was conducted by medical doctors. Different tests and measures were conducted to diagnose and map ABI condition. Among these tests were image tests, namely, computerized tomography and/or magnetic resonance imaging. In addition, thorough medical history was obtained from the patient and/or family members. Specifically, for TBI, injury severity level was ranked using the Glasgow Comma Scale upon admission to the emergency room. Included in the study only youth with Glasgow Comma Scale of 3–12); (2) age range: 5–18 years old; (3) youth in the chronic phase of recovery (i.e., at least six months post-injury); and (4) youth who are able to follow simple three-step directions and commands (specifically: raising the arms, getting up from a chair and stopping an activity).
Conversely, participants were excluded from the study if they met any of the subsequent criteria: (1) inability to independently traverse a distance of at least 10 meters, either with or without orthotic assistance, (2) presence of pre-existing conditions that could impede motor performance, directly or indirectly related to the trauma, and (3) sustaining fractures that hindered the appropriate administration of theBOT-2.
All methodologies and procedures executed in the course of this study adhered strictly to pertinent guidelines and regulations. The study itself obtained ethical approval, including a waiver from the obligation of securing informed consent, from the ethics committee of Chaim Sheba Medical Center (Approval Code: 6504-19-SMC).
Measures
BOT2 is a reliable and valid instrument of motor-skills performance, used in individuals ages four through 21 (17). The complete battery of BOT2 consists of 53 items classified into eight subtests: Fine Motor Precision, Fine Motor Integration, Manual Dexterity, Upper Limb Coordination, Bilateral Coordination, Balance, Running Speed and Agility and Strength (17, 23). This study focused on gross motor function, therefore, only the following BOT2 subtests were examined: Upper Limb Coordination, Bilateral Coordination, Balance, Running Speed and Agility and Strength.
Procedure
This study is a retrospective cross-sectional analysis focusing on youth who were hospitalized in a pediatric rehabilitation due to moderate-to-severe ABI. All individuals admitted to the department underwent a thorough assessment following the department's standardized protocol. Subsequently, all pertinent data were documented in the patients' medical records. Upon obtaining ethical approval from the institution to carry out this study, a detailed review of the medical records of youth diagnosed with moderate-to-severe ABI was conducted. This review aimed to identify those who met the specific inclusion criteria for the study. Individuals meeting the inclusion criteria had their relevant demographic, clinical, and BOT2 data extracted and analyzed.
The BOT2 is administered routinely in the pediatric department by the department's physical and occupational therapists as part of a motor performance battery to every youth having sustained an ABI. The current study focuses only on the gross motor domains which are all evaluated by the department's physical therapists. All physical therapists involved in BOT2 assessment (N = 3) had at least five years of seniority in pediatric rehabilitation and a minimum of two years of experience in administrating the BOT2. In addition, all of them partook in BOT2 administration training, and inter-rater agreement was examined. As the gross motor section of the BOT2 may require a lot of effort on behalf of the evaluated youth, the evaluation was commonly conducted during the first evaluation section of the day in a quiet room. The test was conducted according to the BOT2 manual using the long form. Testing was conducted in one session lasting approximately 60 min. Per the BOT2 protocol, item raw scores were calculated for each item (e.g., the number of correct responses or the duration of an activity sustained). Following this, the total point score is calculated for each subtest. Subsequently, using tables provided in the manual, each total point score is converted to a scale score (mean = 15; standard deviation = 5). Finally, age-equivalent scores were also calculated (17).
Data analysis
To evaluate for demographic and clinical characteristics bias, differences between the TBI and the ABI-PF groups in age, time post-injury, and gender were evaluated using independent t-tests and chi-square tests.
Gross motor performance deficits in youth with TBI and ABI-PF
The presence of motor performance deficits was established using the 2SD method. Following is a description of the 2SD method calculation. First, each item of BOT2 received a raw score. The raw scores' units in the various sub-domains assessed are as followed: Upper Limb Coordination—number of catches, throws and dribbles; Bilateral Coordination—number of jumps, touches, pivoting thumbs and feet and fingers taps; Balance—number of seconds or number of steps; Running speed and agility—number of seconds; and Strength—number of repetitions in a specified time unit and distance. Following, each raw score was converted into point score which ranges from 0 to 10, depending on the sub-scale. Finally, the point scores are converted to scaled scores which are based on norms of age and sex. Scaled scores mean score is 15 and the standard deviation is 5. A cutoff point of two standard deviations below the mean was used to establish the presence of apparent motor performance deficits (19). Accordingly, participants were grouped into those performing within and less than two standard deviations. This information was added to the data analysis section (19). In addition, age equivalents were obtained from the manual for each subject's performance on each subtest evaluated. For this study, three groups of performance deficits below chronological age were obtained: (1) up to 24 months (24), (2) 25–35 months, and (3) ≥36 months. First, the prevalence of motor performance deficits in the two assessment methods in the entire group (TBI + ABI-PF) was calculated. Next, deficits prevalence in each etiology group was calculated separately and compared using a chi-square test.
To better understand BOT2 ability to identify youth with motor proficiency on account of TBI vs. ABI-PF issues, receiver operating characteristics (ROC) curves were constructed. In ROC curves, the rate of true positive sensitivity was plotted on the y-axes, and the rate of false positives (1-specificity) was plotted on the x-axes (25). The area under the ROC curve (AUC) indicates the power of the instrument as it denotes the probability of the assessment to rank the child into the correct group. AUCs of 0.5–0.7, 0.7–0.9, and 0.9–1.0, represent low, medium, and high accuracy, respectively (26).
All data analyses were done using Statistical Package for Social Science (SPSS), version 29. ROC analysis was conducted using MedCalc statistical software, version 14.10.2.
Post-hoc power analysis
Post-hoc power analysis for the main (first) research question, pertaining to the differences between youth with TBI and ABI-PF in gross motor ability was conducted using G*Power (version 3.1.9.4). More specifically, the test evaluated the power of the study to detect significant between-group differences in motor ability deficits using each of the evaluation methods (age equivalent and two-standard deviation method). For this purpose, the test family used was “exact” with proportion inequality statistical test for two independent groups (Fisher's exact test), given an alpha, n, and effect sizes.
Results
A total of N = 80 youth with ABI participated in the study (mean age = 11.34 ± 3.55 years; age range: 5.7–17.2 years); age range: 5.6–19; 60% boys). Most participants had TBI (n = 50). The mean Glasgow Comma Scale upon admission to the emergency room of youth with TBI was six and ranged from three-to-nine (moderate-to-severe injury). For information regarding group differences and additional information, refer to Table 1.
Gross motor performance deficits in youth with TBI in comparison to ABI-PF
The prevalence of motor performance deficits in the two standard deviation method in the entire group (TBI + ABI-PF) ranged from 16.25% (Bilateral coordination) to 35.00% (Balance). Motor performance deficits were greater when using the age equivalent method and ranged from 75.00% (Balance) to 92.50% (Bilateral coordination). Using the age-equivalent method the prevalence of total motor performance deficits was statistically significantly greater in the ABI-PF group vs. the TBI group only in the Balance sub-scale (Chi-squared = 8.2 (p = 0.04). When using the two-standard deviation method the prevalence of deficits was greater in the ABI-PF vs. the TBI group in the following sub-domains: Upper Limb Coordination (Chi-squared = 24.7, p < 0.01), Balance (Chi-squared = 20.4, p < 0.01), Running Speed and Agility (Chi-squared = 6.9 (p = 0.008), and Strength (Chi-squared = 13.6 (p < 0.01). For additional information, refer to Table 2.
When looking separately at the TBI group, according to the two-standard deviation method, motor deficits ranged from 8.0% (Strength) to 16.0% (Balance and Running Speed and Agility). Motor deficit prevalence in the ABI-PF was higher and ranged from 20.0% (Bilateral Coordination) to 66.66% (Balance). In contrast, the age-equivalent method revealed higher rates of motor deficits. In the TBI group, 40% (Upper Limb Coordination) to 66.0 (Bilateral Coordination) of the youth presented motor function that is ≥36 months below their chronological age. In the ABI-PF group, 46.66% (Bilateral Coordination) to 76.66% (Balance) of the youth presented such motor deficits (see Table 2).
ROC analysis showed that all BOT2 sub-scales, except for Bilateral Coordination, demonstrated medium accuracy in differentiating between youth with PF and non-PF injuries (AUC = 0.7–0.9). Bilateral Coordination accuracy is considered low (AUC < 0.7) (Figures 1A–B, 2A–C). Meaning, motor performance deficits of both groups in all sub-scales except for Bilateral Coordination differed enough to result in medium AUC.
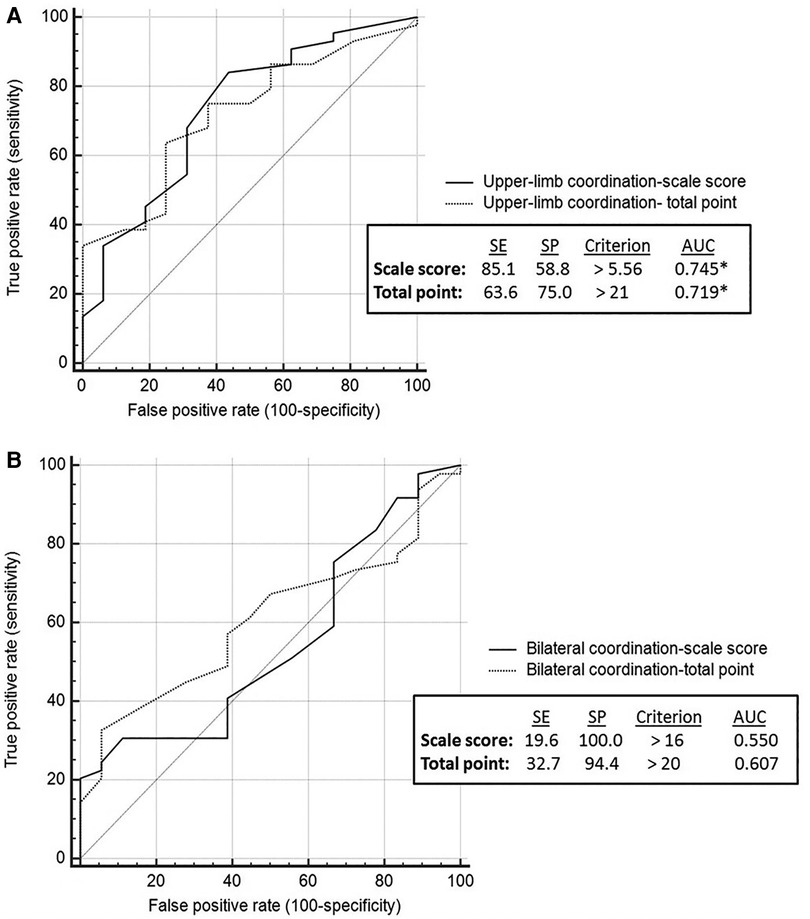
Figure 1. Coordination sub-scales: discriminative ability by brain injury etiology. Discriminative validity of upper limb coordination sub-scale (A). Discriminative validity of bilateral coordination sub-scale (B). AUC, area under the curve; SE, sensitivity; SP, specificity.
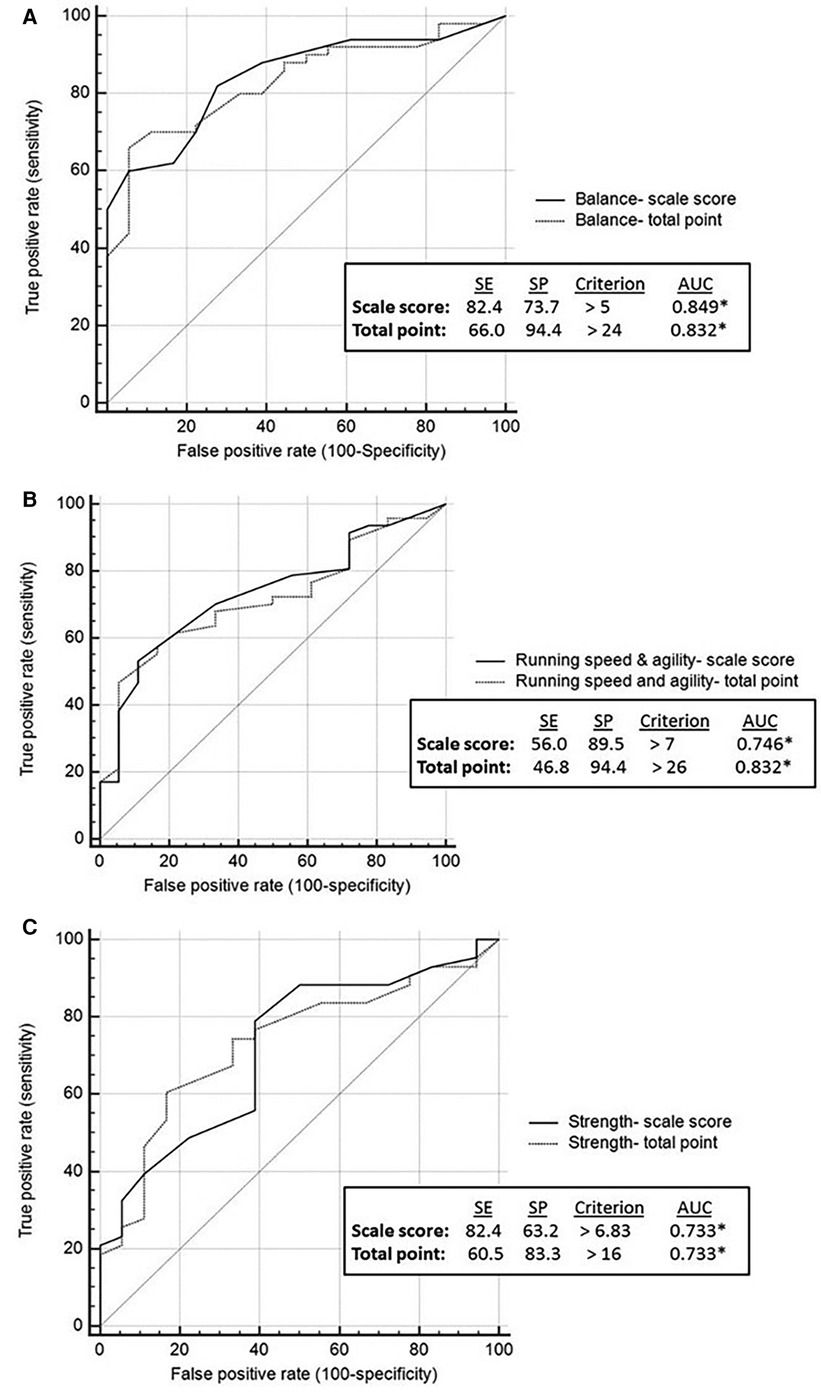
Figure 2. Balance, running speed and agility and strength: discriminative ability by brain injury etiology. Discriminative validity of balance sub-scale (A). Discriminative validity of running speed and agility sub-scale (B). Discriminative validity of strength sub-scale (C). AUC, area under the curve; SE, sensitivity; SP, specificity.
Post-hoc power analysis
Post-hoc power analysis for this section revealed that when using the age equivalent method, in which both study groups presented high prevalence of motor performance deficits, the mean power of the study was not sufficient (power = 0.40). However, for the two-standard deviation method, the power was good and equals to 0.85.
Discussion
The main aims of the study were to increase knowledge on gross motor performance deficits in youth with ABI and specifically in youth post TBI vs. ABI-PF and examine the influence of motor assessment methods on deficits prevalence. In addition, BOT2's ability to discriminate between youth with different ABI etiologies was examined. Understanding motor performance post-pediatric ABI is of special importance as motor deficits may result in difficulties in making and keeping personal relationships, in difficulties in taking part in social activities and in limitations to participating in recreational or leisure activities. In the following section, we discuss the results of each of the aims.
Gross motor performance deficits after ABI
The data showed that deficits in motor performance are present in the chronic phase in youth with moderate-to-severe ABI. According to the two-standard deviation method, the prevalence of deficits in the different BOT2 sub-domains ranged between 20.0% and 66.66% in the ABI-PF group and 8.0%–16.0% in the TBI group. The prevalence of motor deficits in both study groups were considerably higher in the age equivalent method (>90% and >64% of youth in the ABI-PF and TBI groups, respectively). The differences between the two methods regarding the prevalence of motor deficits post-ABI is important as according to Deitz et al. (23) the two-standard deviations method might be one of the criteria for receiving therapy services in certain programs. Therefore, using the two-standard deviations method to identify motor prevalence would not be accurate enough as a child with a score that falls between 1 and 2 standard deviations below the mean, will not be qualified for therapeutic services while he/she might still present significant motor deficits in comparison to his/her peers—preventing him/her from participating in common or mutual activities. Moreover, as among the TBI group, most children score within two standard deviations from the norm's mean in all domains assessed (Table 2). Therefore, it may be appropriate to use a more challenging motor assessment in this sub-group of children with ABI. For instance, Wong and colleagues (27) developed a 20 items scale (the Acquired Brain Injury Challenge Assessment) to assess the advanced motor skills of children with ABI. The authors reported that the new assessment tool displayed excellent reliability and initial evidence of validity. Another assessment of interest in children and adolescents with TBI is the High-level Mobility Assessment Tool (HiMAT). The HiMAT demonstrated excellent inter-rater reliability, re-test reliability and responsiveness to change among children and adolescents with TBI (N = 52) (28).
The age-equivalent method also possesses several limitations. For example, the use of age curve fails to consider the variation in the curve. Consequently, a child may achieve an age-equivalent score at a level lower or higher than their chronological age, however, this level of performance may be within the normal bounds of performance for a child of that age (29). Despite the disadvantages associated with the age-equivalent method, age-equivalent scores can still be more useful because they provide an estimate of the child's absolute level of performance (30).
Sub-domains with most significant deficits
Balance—The sub-domain with the highest prevalence of deficits in both study groups is balance. More specifically, in the ABI-PF group 66.66% (two standard deviations method) to 93.32% (age equivalent method) of the group had deficits in balance. In the TBI group, the prevalence ranged from 16% to 64% (two standard deviation and age equivalent method, respectively). Other authors also reported balance deficits post-ABI (24). Long-lasting balance problems post-ABI is not surprising. In the ABI-PF group, damage to the cerebellum can cause balance deficits. More specifically, evidence indicates that certain areas of cerebellar cortex and nuclei (e.g., cerebellar vermis and fastigial nucleus) appear to be engaged in numerous functions, including, balance/vestibular behaviors (31, 32). In the TBI group, balance problems may be explained by white matter lesions. More specifically, in a study of N = 507 youth with TBI, widespread disruption in white matter organization was observed following complicated mild to severe TBI. These alterations appear to persist and encompass a larger number of white matter regions with time post-injury. The corpus callosum appears to be particularly vulnerable to injury, an effect that persists years post-TBI (33). Such white matter changes may disrupt important cortical-subcortical connections that assist in motor control and balance (34).
Slowness—slowness as evaluated in the Running Speed and Agility is another domain in which youth with ABI-PF and TBI exhibited significant difficulties. Accordingly, cognitive response speed deficits and cognitive motor speeded performance deficits (i.e., such as speeded hand function tests and finger tapping) (19) had previously been reported as a problem and a prominent characteristic post-ABI (35–37). Slowness also commonly manifests in gait. For instance, Schaaf et al. (1997) (7) reported that in repeated gait analysis, ambulatory youth with ABI demonstrate significant reductions in velocity and cadence and in other motor activities which require speed. Slowness in the TBI group can be explained by the diffuse axonal injury, damage to the frontal cortex (e.g., premotor cortex, supplementary motor area), and basal ganglia. In the ABI-PF group, slowness may be caused by damage to cerebellar controlling rhythmic movements areas (38). As with the deficits seen in balance, slowness may hinder youth's post-ABI ability to reengage in school and community activity (3), specifically, the ability to engage in physical activities in the same pace with their peers.
Interventions and physical activity for improving motor ability
Considering the impact of motor performance deficits in real life, it is also important to explore the extent to which interventions improve motor function. For example, based on a systematic review of the impact of physical therapy intervention on balance post-TBI (N = 259), the evidence about the effects of the physical therapy interventions in improving the balance ability was limited (39). In another systematic review on the effectiveness of interventions on gross motor outcomes of youth with an ABI, the authors concluded that although the included studies demonstrated preliminary evidence for a positive effect on gross motor outcomes following the interventions, low study methodological quality indicates that care is needed when interpreting and generalizing results to youth with an ABI (40, 41)
Regarding physical activity, the results of this study show that both groups of youth, and especially the ABI-PF group, have low level of motor ability. Hence it is important to adapt activities in therapy and community-based activities to enable the child to partake in it (42). There are four main aspects that can be changed to adapt the activity: (1) Teaching or coaching style—pertains to how the instructor delivers the activity. For example, using appropriate physical assistance; (2) Rules changing (e.g., allowing for more bounces of ball); (3) Changing the environment (e.g., making changes to the activity space); and (4) Allow the use of equipment (e.g., changing the devices used to play the game) (42–44).
Our study has several limitations to generalization. First, study participants consisted of youth during the chronic phase of ABI recovery (≥6 months post-injury). Therefore, our findings may not be generalized to individuals during the acute and sub-acute phases of recovery. Second, in the current study, we have a relatively heterogenic group in terms of age. Therefore, for better generalizability, future studies with larger sample sizes are warranted. Finally, future studies should aim at exploring factors associated motor performance deficits in the chronic phase of TBI and ABI-PF recovery.
Conclusion
Motor deficits years post-ABI in youth are prevalent. However, in comparison to the TBI group, deficits are more severe and more prevalent in the ABI-PF group. Moreover, in comparison to the two-standard deviations method, the extent of motor deficiency is greater when using the age-equivalent method in both study groups. Therefore, using age-equivalent method might provide a more valid classification of deficits in gross motor proficiency for youth post-ABI. The results also suggest that motor performance deficits of both groups in all sub-scales except for Bilateral Coordination differed enough to result in medium AUC. Consequently, BOT2 is a valid measure of physical performance for youth in the chronic phase of ABI. It is important to consider the child's post-ABI motor deficits as they might have cardinal implications on their ability to reenter school and partake in activities with their peers, beyond their deficits in cognitive and academic functions. Study's results may contribute to clinicians and therapeutic sports experts, as knowledge on motor abilities of youth post-ABI is important when choosing intervention/activity type.
Data availability statement
The data analyzed in this study is subject to the following licenses/restrictions: Data is available upon request. Requests to access these datasets should be directed to Sharon Barak:c2hhcm9uaS5iYXJha2lAZ21haWwuY29t.
Ethics statement
The study involving humans was approved by Chaim Sheba Medical Center (Approval Code: 6504-19-SMC). The study was conducted in accordance with the local legislation and institutional requirements. The ethics committee/institutional review board waived the requirement of written informed consent for participation from the participants or the participants’ legal guardians/next of kin because it was a retrospective study on data in the medical records.
Author contributions
SB: Conceptualization, Formal Analysis, Investigation, Methodology, Software, Writing – original draft, Writing – review & editing. AB: Conceptualization, Methodology, Supervision, Writing – review & editing. TY: Data curation, Investigation, Project administration, Supervision, Writing – review & editing. EE: Conceptualization, Data curation, Investigation, Methodology, Supervision, Writing – original draft, Writing – review & editing. SA: Data curation, Investigation, Methodology, Supervision, Writing – original draft. JL: Conceptualization, Methodology, Writing – review & editing.
Funding
The author(s) declare that no financial support was received for the research, authorship, and/or publication of this article.
Conflict of interest
The authors declare that the research was conducted in the absence of any commercial or financial relationships that could be construed as a potential conflict of interest.
Publisher's note
All claims expressed in this article are solely those of the authors and do not necessarily represent those of their affiliated organizations, or those of the publisher, the editors and the reviewers. Any product that may be evaluated in this article, or claim that may be made by its manufacturer, is not guaranteed or endorsed by the publisher.
References
1. Dumas HM, Haley SM, Ludlow LH. Achieving a minimally important difference in physical function during pediatric inpatient rehabilitation. Int J Rehabil Res. (2008) 31(3):257–60. doi: 10.1097/MRR.0b013e3282fb7d03
2. Centers for Disease Control and Prevention. Department of Health and Human Services, National Center for Injury Prevention and Control. 2006. Traumatic brain injury in the United States: emergency department visits, hospitalizations, and deaths.
3. Ibey RJ, Chung R, Benjamin N, Littlejohn S, Sarginson A, Salbach NM, et al. Development of a challenge assessment tool for high-functioning children with an acquired brain injury. Pediatr Phys Ther. (2010) 22(3):268–76. doi: 10.1097/PEP.0b013e3181ea8ff6
4. Kaatsch P, Rickert CH, Kuand¨hl J, Schuand¨z J, Michaelis J. Population-based epidemiologic data on brain tumors in German children. Cancer. (2001) 92(12):3155–64. doi: 10.1002/1097-0142(20011215)92:12%3C3155::AID-CNCR10158%3E3.0.CO;2-C
5. Koustenis E, Hernáiz Driever P, De Sonneville L, Rueckriegel SM. Executive function deficits in pediatric cerebellar tumor survivors. Eur J Paediatr Neurol. (2016) 20(1):25–37. doi: 10.1016/j.ejpn.2015.11.001
6. Emanuelson I, Von Wendt L, Beckung E, Hagberg I. Late outcome after severe traumatic brain injury in children and adolescents. Pediatr Rehabil. (1998) 2(2):65–70. doi: 10.3109/17518429809068157
7. Vander Schaaf PJ, Kriel RL, Krach LE, Luxenberg MG. Late improvements in mobility after acquired brain injuries in children. Pediatr Neurol. (1997) 16(4):306–10. doi: 10.1016/S0887-8994(97)00025-8
8. Kuhtz-Buschbeck JP, Hoppe B, Gölge M, Dreesmann M, Damm-Stünitz U, Ritz A. Sensorimotor recovery in children after traumatic brain injury: analyses of gait, gross motor, and fine motor skills. Dev Med Child Neurol. (2003) 45(12):821–8. doi: 10.1111/j.1469-8749.2003.tb00897.x
9. Manto M, Bower JM, Conforto AB, Delgado-García JM, Da Guarda SNF, Gerwig M, et al. Consensus paper: roles of the cerebellum in motor control-the diversity of ideas on cerebellar involvement in movement. Cerebellum. (2012) 11(2):457–87. doi: 10.1007/s12311-011-0331-9
10. Raybaud C, Ramaswamy V, Taylor MD, Laughlin S. Posterior fossa tumors in children: developmental anatomy and diagnostic imaging. Child’s Nervous System. (2015) 31:1661–76. doi: 10.1007/s00381-015-2834-z
11. Povlishock JT, Katz DI. Update of neuropathology and neurological recovery after traumatic brain injury. J Head Trauma Rehabil. (2005) 20:76–94. doi: 10.1097/00001199-200501000-00008
12. Bjornard KL, Gilchrist LS, Inaba H, Diouf B, Hockenberry MJ, Kadan-Lottick NS, et al. Peripheral neuropathy in children and adolescents treated for cancer. Lancet Child Adolesc Health. (2018) 2(10):744–54. doi: 10.1016/S2352-4642(18)30236-0
13. Gielis M, Dirix V, Vanderhenst E, Uyttebroeck A, Feys H, Sleurs C, et al. Better detection of reduced motor functioning in brain tumor survivors based on objective motor assessments: an incentive for improved standardized follow-up. Eur J Pediatr. (2022) 181(7):2731–40. doi: 10.1007/s00431-022-04472-1
14. Piscione PJ, Bouffet E, Mabbott DJ, Shams I, Kulkarni A V. Physical functioning in pediatric survivors of childhood posterior fossa brain tumors. Neuro Oncol. (2014) 16(1):147–55. doi: 10.1093/neuonc/not138
15. Varedi M, Lu L, Phillips NS, Partin RE, Brinkman TM, Armstrong GT, et al. Balance impairment in survivors of pediatric brain cancers: risk factors and associated physical limitations. J Cancer Surviv. (2021) 15(2):311–24. doi: 10.1007/s11764-020-00932-5
16. Decock M, De Wilde R, Van der Looven R, Vander Linden C. Motor functioning and intelligence quotient in paediatric survivors of a Fossa posterior tumor following a multidisciplinary rehabilitation program. Int J Environ Res Public Health. (2022) 19(12):7083. doi: 10.3390/ijerph19127083
17. Bruininks R, Bruininks B. BOT2 Bruininks-Oseretsky Test of Motor Proficiency second edition Manual. Crowley, TX: AGS Publishing (2005).
18. McCauley SR, Wilde EA, Anderson VA, Bedell G, Beers SR, Campbell TF, et al. Recommendations for the use of common outcome measures in pediatric traumatic brain injury research. J Neurotrauma. (2012) 29(4):678–705. doi: 10.1089/neu.2011.1838
19. Gagnon I, Forget R, Sullivan SJ, Friedman D. Motor performance following a mild traumatic brain injury in children: an exploratory study. Brain Inj. (1998) 12(10):843–53. doi: 10.1080/026990598122070
20. McCauley RJ, Swisher L. Use and misuse of norm-referenced test in clinical assessment. Journal of Speech and Hearing Disorders. (1984) 49(4):338–48. doi: 10.1044/jshd.4904.338
21. Goldblatt J, Friedman F. Oral and written language scales (OWLS). Diagnostique. (1999) 24(1–4):197–209. doi: 10.1177/153450849902401-417
23. Deitz JC, Kartin D, Kopp K. Review of the bruininks-oseretsky test of motor proficiency, second edition (BOT-2). Phys Occup Ther Pediatr. (2007) 27(4):87–102. doi: 10.1080/J006v27n04_06
24. O’Flaherty SJ, Chivers A, Hannan TJ, Kendrick LM, McCartney LC, Wallen MA, et al. The westmead pediatric TBI multidisciplinary outcome study: use of functional outcomes data to determine resource prioritization. Arch Phys Med Rehabil. (2000) 81(6):723–9. doi: 10.1016/s0003-9993(00)90100-x
25. Muschelli J. ROC And AUC with a binary predictor: a potentially misleading metric. J Classif. (2020) 37(3):696–708. doi: 10.1007/s00357-019-09345-1
26. Swets JA. Measuring the accuracy of diagnostic systems. Science. (1988) 240(4857):1285–93. doi: 10.1126/science.32876
27. Wong RKY, McEwan J, Finlayson D, Chung S, Wan L, Salbach NM, et al. Reliability and validity of the acquired brain injury challenge assessment (ABI-CA) in children. Brain Inj. 2014;28(13–14):1734–43. doi: 10.3109/02699052.2014.947620
28. Kissane AL, Eldridge BJ, Kelly S, Vidmar S, Galea MP, Williams GP. High-level mobility skills in children and adolescents with traumatic brain injury. Brain Inj. 2015;29(13–14):1711–6. doi: 10.3109/02699052.2015.1075174
29. Maloney ES, Larrivee LS. Limitations of age-equivalent scores in reporting the results of norm-referenced tests. Contemp Issues Commun Sci Disord. (2007) 34(Fall):86–93. doi: 10.1044/cicsd_34_F_86
30. Ryan J, Lopez S, Sumerall S. Understanding test construction. In: Dorfman W, Hersen M, editors. Perspectives on individual differences: Understanding Psychological Assessment. Washington: Library of Congress Cataloging in Publication Data (2000). p. 1–19.
31. Koziol LF, Budding D, Andreasen N, D’Arrigo S, Bulgheroni S, Imamizu H, et al. Consensus paper: the cerebellum’s role in movement and cognition. The Cerebellum. (2014) 13(1):151–77. doi: 10.1007/s12311-013-0511-x
32. Bonaventura RE, Giustino V, Chiaramonte G, Giustiniani A, Smirni D, Battaglia G, et al. Investigating prismatic adaptation effects in handgrip strength and in plantar pressure in healthy subjects. Gait Posture. (2020) 76:264–9. doi: 10.1016/j.gaitpost.2019.12.022
33. Dennis EL. White matter disruption in pediatric traumatic brain injury: results from ENIGMA pediatric msTBI. bioRxiv. (2020) 97(3):1–32. doi: 10.1212/WNL.0000000000012222
34. Shen D, Wu S, Shi Y, Wang S, Zhang Y, Wang C. The correlation between white matter hyperintensity and balance disorder and fall risk: an observational, prospective cohort study. Chronic Dis Transl Med. (2016) 2(3):173–80. doi: 10.1016/j.cdtm.2016.11.008
35. Prigatano GP, Gray JA. Parental concerns and distress after paediatric traumatic brain injury: a qualitative study. Brain Inj. (2007) 21(7):721–9. doi: 10.1080/02699050701481605
36. Moon D. Disorders of movement due to acquired and traumatic brain injury. Curr Phys Med Rehabil Rep. (2022) 10(4):311–23. doi: 10.1007/s40141-022-00368-1
37. Crasta JE, Sibel J, Slomine BS, Mahone EM, Mostofsky SH, Suskauer SJ. Subtle motor signs in children with chronic traumatic brain injury. Am J Phys Med Rehabil. (2019) 98(9):737–44. doi: 10.1097/PHM.0000000000001110
38. Konoike N, Nakamura K. Cerebral substrates for controlling rhythmic movements. Brain Sci. (2020) 10(8):514. doi: 10.3390/brainsci10080514
39. Alashram AR, Annino G, Raju M, Padua E. Effects of physical therapy interventions on balance ability in people with traumatic brain injury: a systematic review. NeuroRehabilitation. (2020) 46(4):455–66. doi: 10.3233/NRE-203047
40. Baque E, Sakzewski L, Barber L, Boyd RN. Systematic review of physiotherapy interventions to improve gross motor capacity and performance in children and adolescents with an acquired brain injury. Brain Inj. (2016) 30(8):948–59. doi: 10.3109/02699052.2016.1147079
41. Molteni E, Ranzini MBM, Beretta E, Modat M, Strazzer S. Individualized prognostic prediction of the long-term functional trajectory in pediatric acquired brain injury. J Pers Med. (2021) 11(7):675. doi: 10.3390/jpm11070675
42. Woods M. Inclusive Sport Design. How to adapt and modify your sport activities to include all. (2017). p. 1–4.
43. Ewing-Cobbs L, Barnes MA, Fletcher JM. Early brain injury in children: development and reorganization of cognitive function. Dev Neuropsychol. (2003) 24(2–3):669–704. doi: 10.1080/87565641.2003.9651915
Keywords: BOT2, pediatric, brain injury, traumatic brain injury, motor performance, validity
Citation: Barak S, Brezner A, Yissar T, Eisenstein E, Ackerman-Laufer S and Landa J (2024) Gross motor proficiency deficits among children and adolescents post posterior fossa brain tumor removal vs. traumatic brain injury in the chronic phase of recovery: a cross-sectional study. Front. Sports Act. Living 6:1284421. doi: 10.3389/fspor.2024.1284421
Received: 6 September 2023; Accepted: 2 January 2024;
Published: 22 January 2024.
Edited by:
Jaap Van Dieen, VU Amsterdam, NetherlandsReviewed by:
Chi-Wen Chien, Hong Kong Polytechnic University, Hong Kong SAR, ChinaValerio Giustino, University of Palermo, Italy
© 2024 Barak, Brezner, Yissar, Eisenstein, Ackerman-Laufer and Landa. This is an open-access article distributed under the terms of the Creative Commons Attribution License (CC BY). The use, distribution or reproduction in other forums is permitted, provided the original author(s) and the copyright owner(s) are credited and that the original publication in this journal is cited, in accordance with accepted academic practice. No use, distribution or reproduction is permitted which does not comply with these terms.
*Correspondence: Sharon Barak c2hhcm9uaS5iYXJha2lAZ21haWwuY29t