- 1College of Sports Science and Technology, Mahidol University, Nakhon Pathom, Thailand
- 2Cardiff School of Sport and Health Sciences, Cardiff Metropolitan University, Cardiff, United Kingdom
Master runners maintain a similar running economy to young runners, despite displaying biomechanical characteristics that are associated with a worse running economy. This apparent paradox may be explained by a greater physiological effort—i.e., percentage of maximal oxygen uptake (VO2-max)—that master runners perform at a given speed. Moreover, age-related responses to non-exhaustive sustained running are yet underexplored. The aims of this study were, therefore, to examine if biomechanical adjustments in master runners are physiological-effort dependent, and to explore the age-related biomechanical changes during a non-exhaustive sustained run. Young (23.9 ± 6; n = 12) and master (47.3 ± 6.9; n = 12) runners performed a sustained 30-minute treadmill run matched for relative physiological effort (70% VO2-max), while spatiotemporal and lower-limb kinematic characteristics were collected during the 1st and 30th minute. Group differences were observed in step/stride length, knee touch-down angle, and knee stiffness. However, both groups of runners had a similar step frequency, vertical center of mass oscillation, and knee range of motion. Age-related adjustment in these latter characteristics may thus not be an inevitable result of the aging process but rather a strategy to maintain running economy. The relative physiological effort of runners should, therefore, be considered when examining age-related adjustments in running biomechanics.
Introduction
The benefits of distance running for attenuating age-related deterioration of musculoskeletal and cardiovascular health are increasingly recognized (1–5). Running participation at older ages can thus contribute to improved quality of life and help reduce the economic burden on healthcare systems. Accordingly, it is encouraging that the participation of master runners (i.e., > 35 years old) in long-distance running events has increased substantially over the last ∼40 years (6–8). Important motivational factors for running participation among master runners are competition and personal achievement (9, 10). Hence, understanding how aging affects running performance is essential for helping older individuals maintain running motivation.
The ability to sustain a high running speed for an extended period of time is fundamental for running performance, especially in long-distance events (races >5 km). It is well documented that long-distance running performance is closely connected to running economy (11–13)—i.e., the amount of oxygen consumed (VO2) at a given sub-maximal speed. The primary determinants of running economy are physiological and biomechanical in nature (14, 15). Physiological factors that can enhance running economy include a higher maximal oxygen uptake (VO2-max), an increased percentage of slow-twitch muscle fibers, and a higher stroke volume (15). From a biomechanical perspective, Moore (16) has pointed out several parameters that can positively affect running economy, such as running at a preferred stride length and frequency (i.e., spatiotemporal factors), less vertical oscillation, increased leg stiffness, and reduced lower-limb joint extension at take-off (i.e., lower-limb kinematic factors). Running performance, and changes thereof, are thus challenging to assess by considering physiological or biomechanical factors in isolation.
Declines in running performance appear to be an inevitable result of the aging process (17, 18). Age-related performance loss has been linked to several distinct physiological and biomechanical characteristics in master runners (19). Physiologically, aging introduces declines in, e.g., peak heart rate and VO2-max (20). Biomechanically, spatiotemporal and kinematic alterations, such as increased stride frequencies (21), and reduced lower-limb joint range of motion (22), have been shown to occur with increasing age. It is interesting to note that many biomechanical characteristics that are distinctive of master runners are also linked to a worse running economy (16), which in turn is an important determinant of distance running performance (11–13). Biomechanical alterations with age thus likely contribute to reductions in running economy and subsequently contribute to performance loss in master runners. Age-related changes in running kinematics and performance have indeed been linked previously in short- (23) and middle-distance (24) runners. However, trained master runners have also been shown to maintain a similar running economy at sub-maximal speeds compared to young runners, despite having distinct biomechanical characteristics (21, 25). Although running economy and performance are influenced by numerous factors, it is yet unclear how this apparent paradox can be explained.
Most studies that have investigated the biomechanical differences between master and young runners have matched the running speed for both groups. However, at a given sub-maximal running speed, master runners perform at a higher percentage of their VO2-max compared to young runners, despite having a comparable running economy (21, 26)—i.e., master runners perform a relatively greater physiological effort to maintain the same speed. It could, therefore, be that master runners adjust their running biomechanics in response to this greater physiological effort, which can contribute to reductions in performance despite maintaining a similar running economy. However, it is yet unclear if differences in running biomechanics are also present in master runners if the relative physiological effort, rather than the exact running speed, remains the same. Furthermore, changes in joint kinematics are known to occur after typical non-exhaustive running sessions—i.e., a duration of around 30–45 min, running at sub-maximal efforts such as 70%–85% of maximal heart rate or oxygen uptake (27, 28). Although there are indications that biomechanical adjustments after a bout of sustained running are comparable between master and young runners, evidence is still limited (29). The aim of this study was, therefore, twofold. First, to examine if differences in spatiotemporal and lower-limb kinematic characteristics are present in master runners if the overall physiological effort is matched with young runners. Second, to examine if changes in spatiotemporal and lower-limb kinematic characteristics during a typical non-exhaustive sustained run are similar between master and younger runners. We hypothesized (1) that age-related biomechanical adjustments depend on the relative physiological effort during running, and (2) that biomechanical responses to a sustained run are independent of age.
Methods
Participants
In total, 24 healthy recreational long-distance runners (i.e., competing in races >5 km) volunteered for this study (16 males and eight females). Participants were included if they met the age criteria for one of two age groups (Table 1)—either young runners (<35 years; n = 12) or master runners (>40 years; n = 12) (6). Only runners within the defined age brackets were included to ensure distinct age groups, and both groups were intentionally balanced for the number of male and female runners (Table 1). The sample size was determined based on a two-sided independent t-test (80% power, α = 0.05), considering a detectable difference of three degrees in knee range of motion, with a small to medium effect size (using G*Power 3.1, Universität Düsseldorf, Düsseldorf, Germany). All runners recruited for this study ran at least 25 kilometers per week in training and had completed at least one minimarathon race (10.55 km) in the last twelve months. Participants were excluded from this study if they had any current lower-limb injuries or previous surgery of the lower limbs in the past six months. All participants provided informed consent before participating in this study, in line with the ethical procedures, which were approved by the Mahidol University Central Institutional Review Board (reference number MU-CIRB 2019/132.0808).
Testing procedure and data analysis
The data collection consisted of two visits to Mahidol University's College of Sports Science and Technology biomechanics laboratory. During the first visit, participants performed a ten-minute familiarization and warmup run on the treadmill (Valiant 2 cpet, Lode, The Netherlands) at a self-selected running speed before performing a protocol to determine maximal oxygen uptake (VO2-max) (30–32). The VO2-max protocol consisted of an incremental run until exhaustion while oxygen uptake (VO2) was measured with a portable gas analyzer (Oxycon mobile, Jaeger, Germany). Running speed started at eight kilometers per hour and was increased by two kilometers per hour every 2 min. If a speed of 16 km per hour was reached, the incline of the treadmill was increased by 2% every 2 min. There were three termination criteria for the VO2-max protocol (33): (1) oxygen consumption during running reached a plateau with a plateau defined as two VO2 values over a 30-s period where the second value did not exceed the first value; (2) participants reached a respiratory exchange ratio of 1.15; (3) participants could not continue the protocol and asked to stop the test. Based on the results of the VO2-max protocol, each participant's running speed for the sustained run during the second laboratory visit was determined. A regression line was fitted to the VO2 measurements across all running speeds. From this regression, each runner's speed at 70% of their VO2-max was determined.
During the second visit, all runners performed a sustained treadmill run during which their spatiotemporal and kinematic characteristics were assessed. Participants were asked to avoid high-intensity exercise and caffeine consumption in preparation for the second visit and wore their own running shoes in which they were most comfortable running. After a 10-minute warmup and familiarization on the treadmill, participants were instructed to perform a 30-minute sustained run on the treadmill (Walkerview performance 3.0, TecnoBody, Italy) at 70% of their VO2-max (27, 28). The combination of this duration and intensity was deemed non-exhaustive and reflective of a typical training run.
Three-dimensional kinematics were recorded using a motion capture system consisting of eight optoelectronic cameras (OptiTrack, NaturalPoint, USA), sampling at 100 Hz, and 44 retro-reflective markers (16 mm diameter) were attached to the participant's body following the lower limb and trunk model (34). Prior to each 30-min treadmill run, a static trial was captured. Throughout the run, kinematic data for five strides were collected during the 1st and 30th minute. Visual 3D (version 6.0, C-motion, Germantown, USA) was then used to process the marker trajectories and analyze kinematics. Marker trajectories were gap-filled and filtered at 9 Hz using a fourth order Butterworth lowpass filter. An eight-segment musculoskeletal model was then built using the static trial, from which segment and joint kinematics during running were derived. Inverse kinematic constraints were applied to each of the lower limb joints. Several discrete kinematic variables were then determined to assess running kinematics for each runner.
Spatiotemporal and kinematic characteristics
For each of the five strides that were collected at both time points (i.e., 1st and 30th minute), the moments of touch-down and take-off were identified using the minimal vertical velocity of the pelvis center of mass (35) and peak knee extension (36) respectively. Touch-down and take-off were used to determine spatiotemporal running characteristics—i.e., stride and step length, and step frequency. Kinematic characteristics included the joint angles of the hip, knee, and ankle, and the whole-body center of mass (CoM) position (which was normalized to each participant's height) at touch-down and take-off. In addition, the range of motion of each joint and the CoM during the stance phase was determined. Since bilateral kinematic asymmetries are common, can be substantial, and can affect group comparisons (37–40), leg-specific comparisons were made between the master and young runners.
Stiffness of the knee joint was calculated using a previously described and validated kinematics-based method (Figure 1) (28, 41–43). Briefly, knee stiffness values Kknee were determined for the initial contact phase (from touch-down to the peak knee angular velocity) and the weight acceptance phase (from peak knee angular velocity to maximal knee flexion) according to:
in which I is the participant's mass multiplied by the length of the thigh squared (), is the knee angular velocity in rad⋅s−1, is the knee angle in radians, and ROM is the range of motion of the knee in degrees, for either the initial contact or weight acceptance phase of landing. The thigh length was measured as the distance between the greater trochanter and lateral femoral epicondyle (Table 1). was determined by up-sampling the knee angle data between touch-down and maximal knee flexion to 500 Hz and fitting a line to the data points between 20%–80% of each phase. Further details and examples can be found in Dutto and Braun (28), Verheul et al. (42), and Zhang and Lake (43).
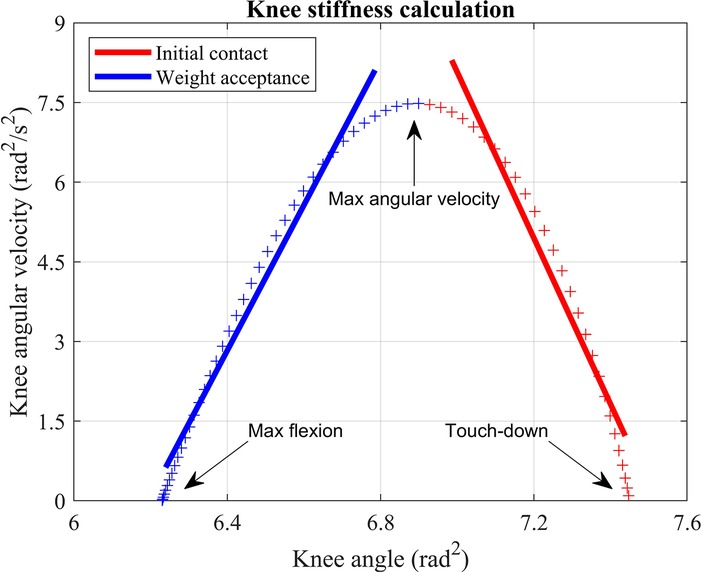
Figure 1. A representative example trial of the kinematics-based calculation of the knee stiffness during the initial contact (red) and weight acceptance (blue) phase of landing.
Statistical analysis
Since bilateral kinematic asymmetries are common and can be substantial (37, 38, 40), we performed leg-specific statistical analyses for each kinematic variable. SPSS (version 18.0, IBM, Chicago, IL, USA) was used to perform statistical analyses. A Shapiro–Wilk test was used to determine the normal distribution of discrete data. Group characteristics (Table 1) were compared using t-tests, and effect sizes were assessed using Cohen's d as small (d = 0.2), medium (d = 0.5), or large (d = 0.8). A two-way repeated measures ANOVA was used to evaluate the effect of age (i.e., master vs. young runners) and running time (1st and 30th minutes). Holm Post-hoc tests were used to determine the locations of significant effects. All data are reported as mean ± standard deviation (SD). The level of statistical significance was set at p < 0.05. To enhance result comparisons across studies (44), effect sizes were determined by calculating the partial eta squared (). Effect sizes were evaluated as either small ( = 0.01), medium ( = 0.06), or large ( = 0.14) effects (45).
Results
Young runners had a higher (p = 0.07, d = 0.8) VO2-max compared to the master runners (Table 1). Accordingly, the running speed at 70% of VO2-max was reduced (p = 0.07, d = 0.8) in the master group, and the young runners completed a greater distance (3.96 ± 1.2 km) during the 30-minute treadmill run than the master runners (3.19 ± 0.74 km). Although these differences were not significant, the effect sizes were large.
Spatiotemporal characteristics
Step lengths were significantly longer by 16% (p < 0.001) for the young compared to the master group, for both legs with a large effect size (right = 0.16; left = 0.15) (Figure 2). Moreover, in both groups the step lengths significantly increased (p < 0.001; = 0.08–0.1) after the 30-minute sustained run. Consequently, stride lengths were also longer for the young runners (16%; p < 0.001; = 0.16) and increased by 3% over time (p < 0.001; = 0.12) (Figure 2). Step frequency, however, was similar for both groups and significantly decreased (p < 0.001; = 0.21) after 30 min of running (Figure 3), with a small interaction between age and running time (p < 0.05; = 0.04).
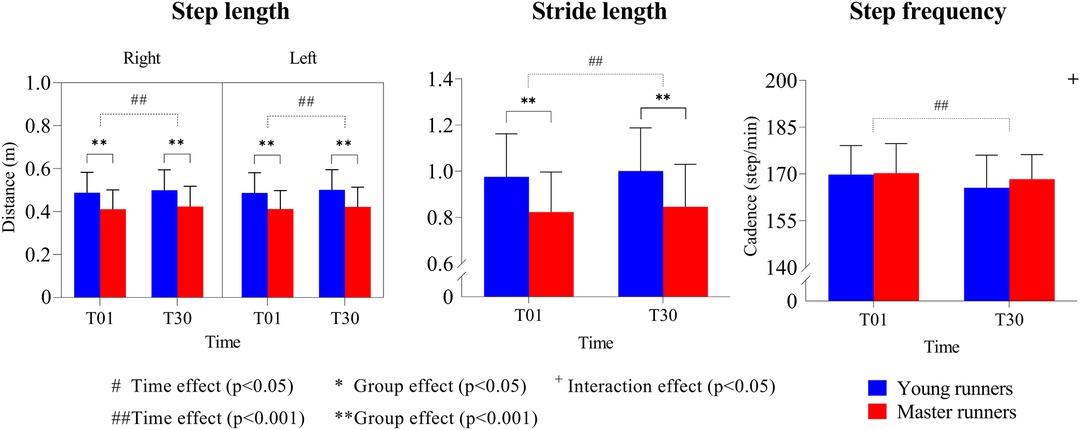
Figure 2. Means and standard deviations for step length (left and right), stride length, and step frequency during running. T01 = 1st minute; T30 = 30th minute.
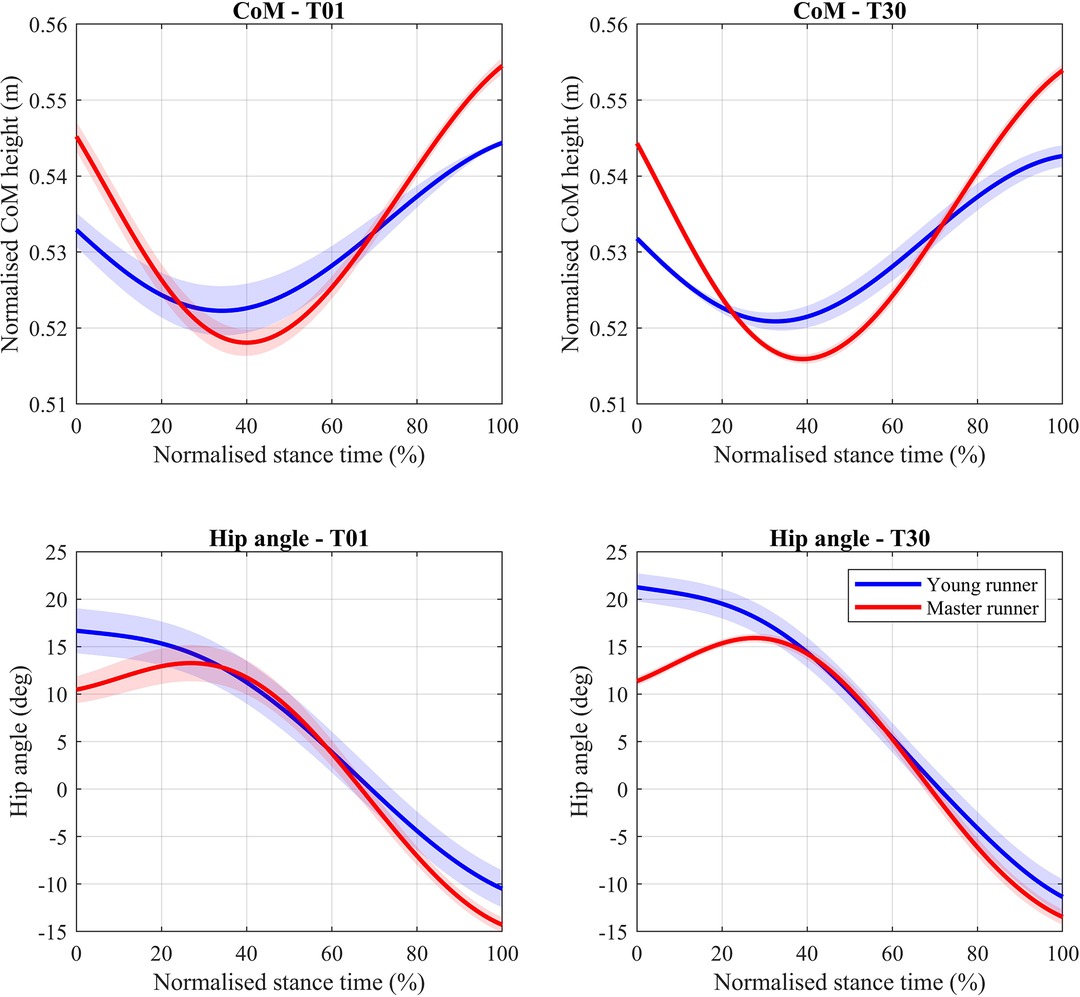
Figure 3. Centre of mass (CoM; top row) and hip angle (bottom row) trajectories during the stance phase of running for one representative young (blue) and master (red) runner. Curves represent means and standard deviations (shaded areas) over five ground contacts. Data are shown for the start (T01) and end (T30) of the sustained 30-minute run.
Kinematic characteristics
The normalized vertical position of the CoM was significantly higher at touch-down in the master runners compared to the young group (1%; p < 0.001; = 0.18), whereas running time significantly lowered the position of the CoM at touch-down in both groups (1%; p < 0.001; = 0.32) (Figure 4—top row). At take-off, however, the vertical CoM position was significantly higher in the master runners compared to the young runners (1%; p < 0.001; = 0.16), but the sustained run did not affect this. Furthermore, age did not affect the range of motion of the CoM, but running time did by 5% (p < 0.001; = 0.15).
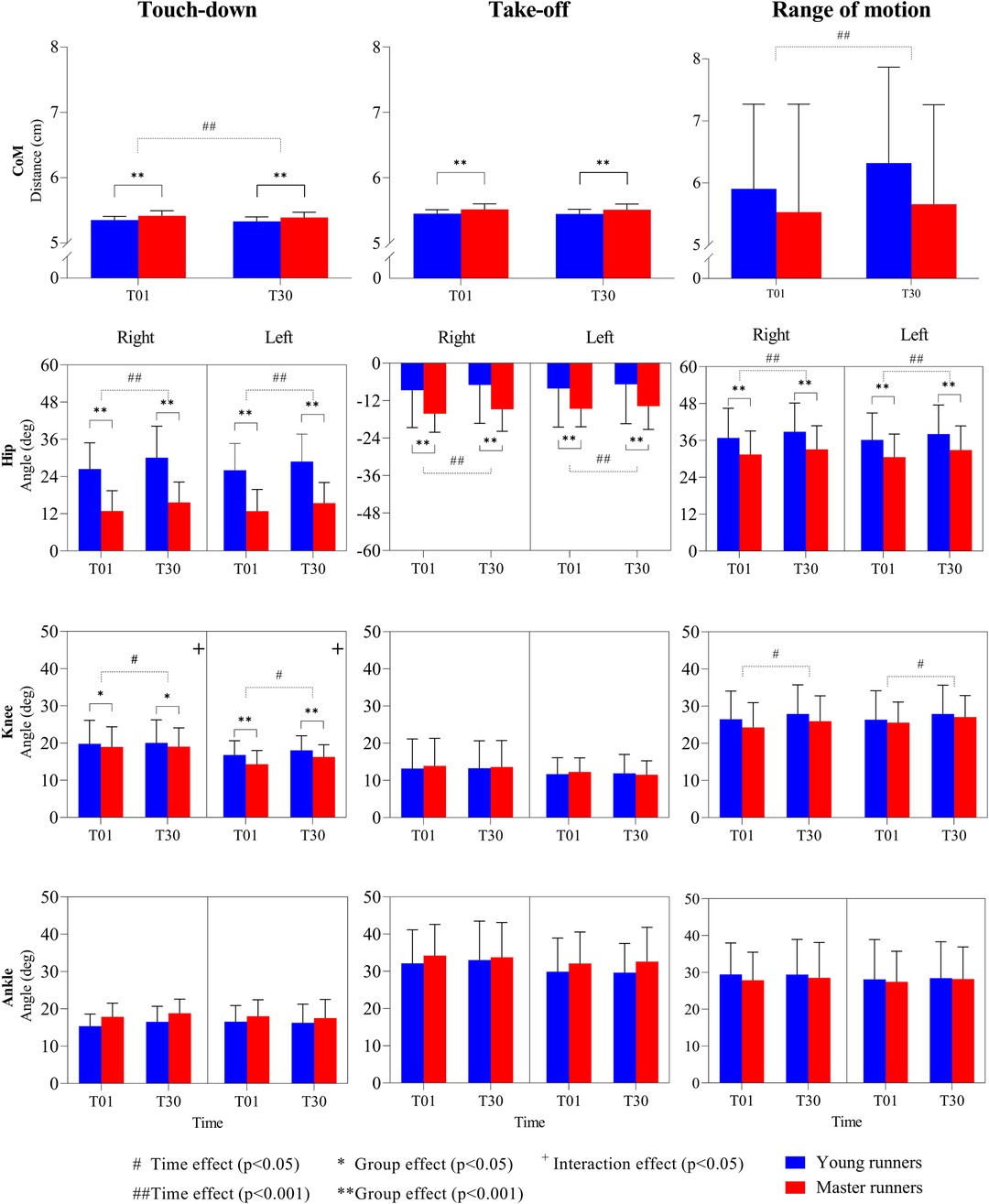
Figure 4. Means and standard deviations of the center of mass position (CoM) and hip, knee, and ankle joint angles (left and right), at touch-down and take-off, and the range of motion during the stance phase of running. Positive-negative angles indicate flexion-extension (hip and knee), and plantarflexion- dorsiflexion (ankle). T01 = 1st minute; T30 = 30th minute.
The angles of the hip and knee joints at touch-down were significantly larger (48%–50% and 11%–13% respectively; p < 0.05) for the young runners compared to the master group, both for the right (hip = 0.45; knee = 0.06) and left (hip = 0.44; knee = 0.16) leg (Figure 4—left column). The sustained run caused significant increases in the hip (14%–17%; p < 0.001; = 0.41–0.45) and knee (1%–4%; p < 0.05; = 0.08–0.09) joint angles for both legs at touch-down with medium to large effect sizes. There was also small to medium interaction between age and running time for knee touch-down angle for both legs (p < 0.05; = 0.04–0.07). The ankle joint angle at touch-down, however, was neither affected by age nor running time.
Age and 30 min of sustained running both significantly (p < 0.001) affected the hip joint angle at take-off (Figure 4—middle column). For both legs, there were medium to large 90%–98% increases in hip angle with age ( = 0.11–0.14), but a large 9%–12% decrease with running time ( = 0.14–0.2). However, the knee and ankle joint angles at take-off were unaffected by age or running time.
The range of motion of the hip during the stance phase was significantly greater in the young runners compared to the master runners (15%; p < 0.001; = 0.1) for both the right and left leg (Figure 4—right column). Likewise, sustained running had a large increasing effect (5%–6%; p < 0.001; = 0.23–0.35) on the hip range of motion in both age groups. Although age did not affect the range of motion of the knee or ankle joints, the knee range of motion was significantly increased after the sustained run in both legs (3%–6%; p < 0.05; = 0.06–0.12).
Knee joint stiffness
A medium significant effect of age (p < 0.02; = 0.05–0.09) on knee stiffness (Figure 5) was found. In both legs, a 9%–15% higher knee stiffness was found for the young runners compared to the master runners during both the initial impact and weight acceptance phases of landing. However, knee stiffness was not affected by running time.
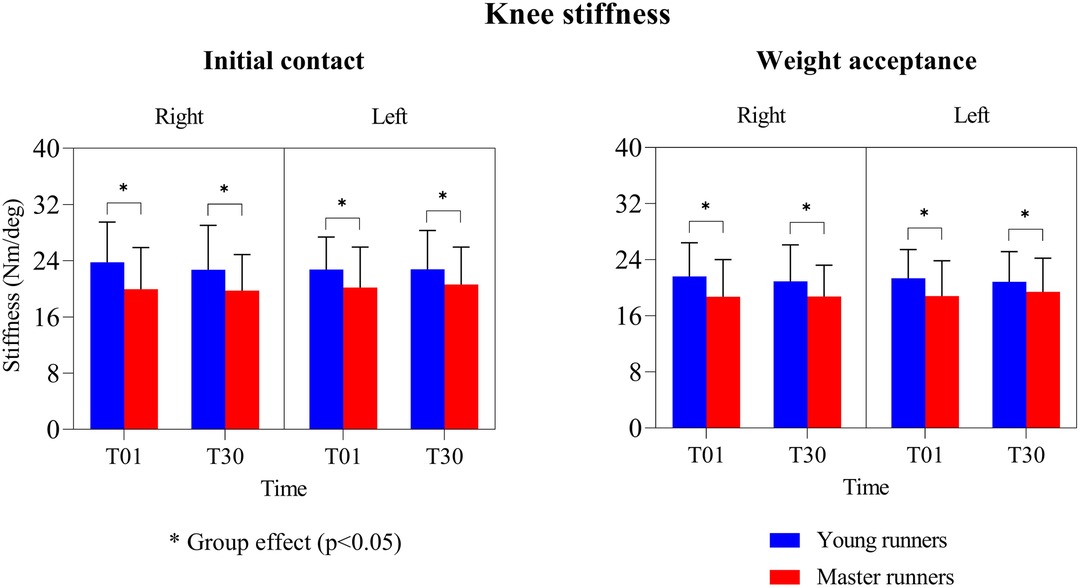
Figure 5. Means and standard deviations of left and right knee stiffness during the initial impact and weight acceptance phase. T01 = 1st minute; T30 = 30th minute.
Discussion
Well-trained master runners have been found to have a similar running economy compared to young runners, despite showing altered biomechanics that are related to a worse running economy. The results of this study show that when physiological effort is matched, master runners run with a different step length, knee touch-down angle, and knee stiffness, compared to younger runners. However, step frequency, vertical center of mass oscillation, and knee range of motion were similar between both groups. These findings indicate that age-related adjustment in step frequency, vertical center of mass oscillation, and knee range of motion may not be an inevitable result of the aging process but rather a strategy to maintain running economy.
An essential component of running performance is speed, which is determined by the combination of step length and frequency. Runners are known to self-optimize these parameters to get close to their economical optimum for a given speed (46). We found that both the step and stride length were significantly reduced in the master runners compared to the young runners, while step frequency was the same in both groups. Several others have reported similar reductions in step and stride length for master runners, but these were always accompanied by increases in step frequency to maintain speed (21, 22, 47, 48). In this study, however, each runner's speed was matched to be at 70% of their VO2-max and hence, running speeds varied across runners (Table 1). Our results thus show that master runners who run at the same physiological effort adjust (i.e., lower) their step length but maintain the same step frequency, which leads to the observed reductions in speed. In other words, running performance loss in master runners is primarily associated with changes in step length but not frequency. In line with our first hypothesis, step frequency adjustments are thus likely to be dependent on physiological effort and not age.
An increased range of motion of the body's CoM during the stance phase of running (or vertical oscillation) is associated with a worse running economy (16, 49). We did not find any differences in vertical oscillation between both groups of runners. In contrast, Karamanidis and Arampatzis (48) found that master runners run with less vertical oscillation than young runners, which should thus contribute to a better running economy. Since that study used the same speed for all runners, and the master group ran at a greater (perceived) physiological effort (i.e., higher percentage of VO2-max), it is possible that the master runners adjusted their vertical oscillation to a more economical pattern to maintain running economy. Since we matched each runner's running speed to be at the same physiological effort, together, these results provide evidence that master runners adjust their vertical oscillation depending on the relative physiological effort, which supports our first hypothesis.
Running with more knee flexion at touch-down and a larger knee joint range of motion has been linked to a higher metabolic demand—i.e., a worse running economy (50–52). Previous studies have found older runners to run with a more flexed knee at touch-down and a smaller knee joint range of motion (22, 25, 47), which would respectively contribute to a worse and better running economy, and thus a negligible net change. In our study, however, master runners only ran with a less flexed knee at touch-down but had a similar knee range of motion to young runners, which together would contribute to a better running economy. Hence, master runners adjust multiple knee characteristics (i.e., touch-down angle and range of motion) when the relative physiological effort to perform the same running speed increases with age, to help maintain a similar running economy (21). Since only the knee touch-down angle was different from the young runners in our study, adjustments of the touch-down angle of the knee joint are likely to be independent of effort and primarily age-related. Adjustments of the knee range of motion, however, are likely to be physiological-effort dependent, which further supports our first hypothesis.
Good evidence exists for a positive correlation between running economy and the amount of flexion of the lower-limb joints at take-off (53, 54). Although we did not observe any group differences for the take-off angle of the ankle and knee joints, master runners ran with a significantly more extended hip joint at take-off (together with a lower hip range of motions and less hip flexion at touch-down). This can be a contributing factor to a less economic running style and performance loss. Interestingly, not many previous studies have investigated the kinematics of the hip joint during running in older populations. Future work should, therefore, further examine the relationship between hip flexion at take-off and running economy, and possibly consequent performance loss, in master runners.
An increased stiffness of the knee joint is related to better running economy and performance (55, 56). We found the knee to be 7%–19% stiffer in young compared to master runners, both during the initial contact phase and the weight acceptance phase, regardless of the effort-matched design in this study. This decrease in knee stiffness with age is in line with previous findings of ∼10%–20% stiffness declines in the knee and ankle joints (57), and leg (21) in older runners. Stiffness reductions have primarily been linked to the loss of muscle strength and neuromuscular function due to aging, both of which may be slowed down by running training (21, 58, 59). It has indeed been shown that (young) runners with a higher training volume can more efficiently coordinate the thigh muscles to regulate knee stiffness during landing (42), whereas older runners with a consistently high training volume can retain their stiffness regulation capacity (58, 60). These findings support the notion that sufficiently high running volume might positively contribute to the maintenance of appropriate leg and joint stiffness in master runners and can help attenuate economy and performance loss with aging.
Changes in joint kinematics have been shown to occur over the course of a sustained run (27, 29, 61). Our findings further support the notion that non-exhaustive sustained running affects lower-limb kinematics. We found that 30 min of running at 70% of VO2-max increased the step and stride length, decreased the step frequency, increased the hip and knee joint angles at touch-down, lowered the CoM position at touch-down, decreased the hip angle at take-off, and increased the range of motion of CoM, hip, and knee. However, only for the step frequency and the touch-down angle of the knee did we observe a significant interaction between age and running time, but the effect sizes were small to medium. Together, these findings are largely in line with a previous work (29) that did not find sustained running to affect the running biomechanics of master and young runners differently and supports our second hypothesis that biomechanical responses to a sustained run are largely independent of age.
Bilateral kinematic asymmetries are common and can be substantial, although clear evidence of the detrimental effects of asymmetries on running economy and performance is lacking (37, 38, 40). However, given the high asymmetry prevalence, either focusing on a single leg or combining both legs together in the same analyses can introduce biases in kinematic comparisons between groups. Therefore, we performed leg-specific comparisons between the master and young runners. We observed that all the significant group differences were present in both legs, which further confirms that the observed kinematic changes are indeed associated with age and/or sustained running and are not the result of, e.g., limb dominance.
Several limitations to this study should be considered. First, only lower-limb kinematics in the sagittal plane were examined. However, other planes of lower-limb kinematics may also contribute to running economy and performance and can be considered in future investigations of the effect of aging on running biomechanics. Second, kinetic data were not collected and examined in this study, while external kinetics (i.e., ground reaction forces) and joint kinetics (i.e., joint moments) are known to be affected by aging (21, 22, 62, 63) and to be important considerations for running economy and performance (64). Third, a non-exhaustive 30-minute running protocol was used in this study to examine the effect of a typical training run on running biomechanics. Similar protocols have previously been shown to induce alterations in running kinematics (27, 28). Since the runners included in this study were all well-trained, less-trained runners may display more prominent changes in kinematics and further highlight age-related differences in biomechanical responses to sustained running. Fourth, confounding factors, such as physiological and anthropometric differences between male and female runners, were not considered but can influence the observed differences or similarities between age groups. Since this study was a first step to examine the role that the physiological effort at which runners perform plays in biomechanical differences that have been observed between younger and master runners, we encourage future studies to delve into the interactions of other confounding factors (including sex, anthropometrics, etc). Finally, VO2 was not measured and monitored during the sustained 30-min run. The assumption that runners maintained a relative physiological effort of 70% of their VO2-max could thus not be verified.
Conclusion
We show that master runners do not have a different step frequency, vertical oscillation of the CoM, or knee range of motion when relative physiological effort was matched with young runners. Speed-matched studies have previously found age-related differences in these characteristics. Those adjustments in master runners may, therefore, not be an inevitable result of aging but rather a strategy to maintain running economy (and consequent performance). However, differences in step/stride length, knee angle at touch-down, and knee stiffness were observed between groups even when the relative physiological effort was matched in all runners. Adjustments in these characteristics are thus more likely to be the result of the aging process. The relative physiological effort at which runners perform should, therefore, be considered when examining age-related adjustments in running biomechanics.
Data availability statement
The raw data supporting the conclusions of this article will be made available by the authors, without undue reservation.
Ethics statement
The studies involving humans were approved by Mahidol University Central Institutional Review Board (reference number MU-CIRB 2019/132.0808). The studies were conducted in accordance with the local legislation and institutional requirements. The participants provided their written informed consent to participate in this study.
Author contributions
PJ: Conceptualization, Data curation, Formal Analysis, Investigation, Methodology, Project administration, Resources, Supervision, Validation, Writing – original draft, Writing – review & editing, Funding acquisition. SS: Conceptualization, Data curation, Formal Analysis, Investigation, Methodology, Validation, Writing – review & editing, Software, Visualization. WL: Conceptualization, Writing – review & editing, Funding acquisition, Resources, Supervision. JV: Conceptualization, Resources, Supervision, Data curation, Formal Analysis, Investigation, Methodology, Project administration, Software, Validation, Visualization, Writing – original draft, Writing – review & editing.
Acknowledgments
The authors would like to acknowledge the Zenas Technology Company (Thailand) for providing the equipment for the data collection and Mahidol University for funding this study. Zenas Technology was not involved in the study design, analysis, interpretation of data, the writing of this article or the decision to submit it for publication.
Conflict of interest
The authors declare that the research was conducted in the absence of any commercial or financial relationships that could be construed as a potential conflict of interest.
Publisher's note
All claims expressed in this article are solely those of the authors and do not necessarily represent those of their affiliated organizations, or those of the publisher, the editors and the reviewers. Any product that may be evaluated in this article, or claim that may be made by its manufacturer, is not guaranteed or endorsed by the publisher.
References
1. Fries JF, Singh G, Morfeld D, O’Driscoll P, Hubert H. Relationship of running to musculoskeletal pain with age. A six-year longitudinal study. Arthritis Rheum. (1996) 39(1):64–72. doi: 10.1002/art.1780390109
2. Lane NE, Bloch DA, Wood PD, Fries JF. Aging, long-distance running, and the development of musculoskeletal disability: a controlled study. Am J Med. (1987) 82(4):772–80. doi: 10.1016/0002-9343(87)90014-3
3. Lavie CJ, Lee D, Sui X, Arena R, O’Keefe JH, Church TS, et al. Effects of running on chronic diseases and cardiovascular and all-cause mortality. Mayo Clin Proc. (2015) 90(11):1541–52. doi: 10.1016/j.mayocp.2015.08.001
4. Ortega JD, Beck ON, Roby JM, Turney AL, Kram R. Running for exercise mitigates age-related deterioration of walking economy. PLOS ONE. (2014) 9(11):e113471. doi: 10.1371/journal.pone.0113471
5. Stenroth L, Cronin NJ, Peltonen J, Korhonen MT, Sipilä S, Finni T. Triceps surae muscle-tendon properties in older endurance- and sprint-trained athletes. J Appl Physiol. (2016) 120(1):63–9. doi: 10.1152/japplphysiol.00511.2015
6. Lepers R, Cattagni T. Do older athletes reach limits in their performance during marathon running? Age (Omaha). (2012) 34(3):773–81. doi: 10.1007/s11357-011-9271-z
7. Reusser M., Sousa C. V., Villiger E., Alvero Cruz J. R., Hill L., Rosemann T., Nikolaidis P. T., Knechtle B. (2021). Increased participation and decreased performance in recreational master athletes in “Berlin marathon” 1974–2019. Front Physiol. 12:631237. doi: 10.3389/fphys.2021.631237
8. Scheer V. Participation trends of ultra endurance events. Sports Med Arthrosc. (2019) 27(1):3. doi: 10.1097/JSA.0000000000000198
9. León-Guereño P, Galindo-Domínguez H, Balerdi-Eizmendi E, Rozmiarek M, Malchrowicz-Mośko E. Motivation behind running among older adult runners. BMC Sports Sci Med Rehabil. (2021) 13(1):138. doi: 10.1186/s13102-021-00366-1
10. Ruiz Juan F., Zarauz Sancho A. (2012). Predictor variables of motivation on Spanish master athletes. J Hum Sport Exerc. (2012) 7(3):617–28. doi: 10.4100/jhse.2012.73.02
11. Conley DL, Krahenbuhl GS. Running economy and distance running performance of highly trained athletes. Med Sci Sports Exercise. (1980) 12(5):357–60. doi: 10.1249/00005768-198025000-00010
12. Di Prampero PE, Capelli C, Pagliaro P, Antonutto G, Girardis M, Zamparo P, et al. Energetics of best performances in middle-distance running. J Appl Physiol. (1993) 74(5):2318–24. doi: 10.1152/jappl.1993.74.5.2318
13. Pollock ML. Submaximal and maximal working capacity of elite distance runners. Part I: cardiorespiratory aspects*. Ann N Y Acad Sci. (1977) 301(1):310–22. doi: 10.1111/j.1749-6632.1977.tb38209.x
14. Morgan D, Martin P, Krahenbuhl G. Factors affecting running economy. Sports Medicine. (1989) 7:310–30. doi: 10.2165/00007256-198907050-00003
15. Saunders PU, Pyne DB, Telford RD, Hawley Ja. Factors affecting running economy in trained distance runners. Sports Medicine. (2004) 34(7):465–85. doi: 10.2165/00007256-200434070-00005
16. Moore IS. Is there an economical running technique? A review of modifiable biomechanical factors affecting running economy. Sports Medicine. (2016) 0:0. doi: 10.1007/s40279-016-0474-4
17. Reaburn P, Dascombe B. Endurance performance in masters athletes. Eur Rev Aging Phys Act. (2008) 5(1):31–42. Article 1. doi: 10.1007/s11556-008-0029-2
18. Trappe S. Marathon runners—how do they age? Sports Medicine. (2007) 37(4):302–5. doi: 10.2165/00007256-200737040-00008
19. Willy R, Paquette M. The physiology and biomechanics of the master runner. Sports Med Arthrosc. (2019) 27:15–21. doi: 10.1097/JSA.0000000000000212
20. Tanaka H, Seals DR. Endurance exercise performance in masters athletes: age-associated changes and underlying physiological mechanisms. J Physiol (Lond). (2008) 586(1):55–63. doi: 10.1113/jphysiol.2007.141879
21. Beck ON, Kipp S, Roby JM, Grabowski AM, Kram R, Ortega JD. Older runners retain youthful running economy despite biomechanical differences. Med Sci Sports Exercise. (2016) 48(4):697–704. doi: 10.1249/MSS.0000000000000820
22. Bus SA. Ground reaction forces and kinematics in distance running in older-aged men. Med Sci Sports Exerc. (2003) 35(7):1167. doi: 10.1249/01.MSS.0000074441.55707.D1
23. Dahl J, Degens H, Hildebrand F, Ganse B. Age-related changes of sprint kinematics. Front Physiol. (2019) 10:613. https://www.frontiersin.org/articles/10.3389/fphys.2019.00613 doi: 10.3389/fphys.2019.00613
24. Dahl J, Degens H, Hildebrand F, Ganse B. Do changes in middle-distance running kinematics contribute to the age-related decline in performance? J Musculoskelet Neuronal Interact. (2020) 20(1):94–100.32131373
25. Hahn H, Vincent KR, Herman DC, Chen C, Zdziarski LA, Morgan C, et al. Biomechanical, metabolic and cardiopulmonary responses of masters recreational runners during running at different speeds. Research in Sports Medicine. (2017) 25(2):118–31. doi: 10.1080/15438627.2017.1282359
26. Quinn TJ, Manley MJ, Aziz J, Padham JL, MacKenzie AM. Aging and factors related to running economy. The Journal of Strength & Conditioning Research. (2011) 25(11):2971. doi: 10.1519/JSC.0b013e318212dd0e
27. Dierks TA, Davis IS, Hamill J. The effects of running in an exerted state on lower extremity kinematics and joint timing. J Biomech. (2010) 43(15):2993–8. doi: 10.1016/j.jbiomech.2010.07.001
28. Dutto DJ, Braun WA. DOMS-Associated Changes in ankle and knee joint dynamics during running. Med Sci Sports Exercise. (2004) 36(4):560–6. doi: 10.1249/01.MSS.0000121957.83226.CC
29. Borgia B, Dufek JS, Silvernail JF, Radzak KN. The effect of fatigue on running mechanics in older and younger runners. Gait Posture. (2022) 97:86–93. doi: 10.1016/j.gaitpost.2022.07.249
30. Ferguson MA, Alderson NL, Trost SG, Essig DA, Burke JR, Durstine JL. Effects of four different single exercise sessions on lipids, lipoproteins, and lipoprotein lipase. J Appl Physiol. (1998) 85(3):1169–74. doi: 10.1152/jappl.1998.85.3.1169
31. Mooses M, Tippi B, Mooses K, Durussel J, Mäestu J. Better economy in field running than on the treadmill: evidence from high-level distance runners. Biol Sport. (2015) 32(2):155–9. doi: 10.5604/20831862.1144418
32. Riboli A, Cè E, Rampichini S, Venturelli M, Alberti G, Limonta E, et al. Comparison between continuous and discontinuous incremental treadmill test to assess velocity at v˙O2max. J Sports Med Phys Fitness. (2017) 57(9):1119–25. doi: 10.23736/S0022-4707.16.06393-3
33. Edvardsen E, Hem E, Anderssen SA. End criteria for reaching maximal oxygen uptake must be strict and adjusted to sex and age: a cross-sectional study. PLoS One. (2014) 9(1):e85276. doi: 10.1371/journal.pone.0085276
34. Vanrenterghem J, Gormley D, Robinson M, Lees A. Solutions for representing the whole-body centre of mass in side cutting manoeuvres based on data that is typically available for lower limb kinematics. Gait and Posture. (2010) 31(4):517–21. doi: 10.1016/j.gaitpost.2010.02.014
35. Milner CE, Paquette MR. A kinematic method to detect foot contact during running for all foot strike patterns. J Biomech. (2015) 48(12):3502–5. doi: 10.1016/j.jbiomech.2015.07.036
36. Fellin RE, Rose WC, Royer TD, Davis IS. Comparison of methods for kinematic identification of footstrike and toe-off during overground and treadmill running. J Sci Med Sport. (2010) 13(6):646–50. doi: 10.1016/j.jsams.2010.03.006
37. Afonso J, Peña J, Sá M, Virgile A, García-de-Alcaraz A, Bishop C. Why sports should embrace bilateral asymmetry: a narrative review. Symmetry (Basel). (2022) 14 (10):1993. doi: 10.3390/sym14101993
38. Bishop C, Turner A, Read P. Effects of inter-limb asymmetries on physical and sports performance: a systematic review. J Sports Sci. (2018) 36(10):1135–44. doi: 10.1080/02640414.2017.1361894
39. Cavanagh PR, Lafortune MA. Ground reaction forces in distance running. J Biomech. (1980) 13(5):397–406. doi: 10.1016/0021-9290(80)90033-0
40. Maloney SJ. The relationship between asymmetry and athletic performance: a critical review. J Strength Cond Res. (2019) 33(9):2579. doi: 10.1519/JSC.0000000000002608
41. Li L, Heiderscheit BC, Caldwell GE, Hamill J. Knee stiffness measurement during the stance phase of level running. J Orthop Sports Phys Ther. (1998) 27(1):99.
42. Verheul J, Clansey AC, Lake MJ. Adjustments with running speed reveal neuromuscular adaptations during landing associated with high mileage running training. J Appl Physiol. (2017) 122(3):653–65. doi: 10.1152/japplphysiol.00801.2016
43. Zhang Z, Lake M. A Re-examination of the measurement of foot strike mechanics during running: the immediate effect of footwear midsole thickness. Frontiers in Sports and Active Living. (2022) 4:824183. doi: 10.3389/fspor.2022.824183
44. Knudson D. Significant and meaningful effects in sports biomechanics research. Sports Biomechanics. (2009) 8(1):96–104. doi: 10.1080/14763140802629966
46. Moore IS, Ashford KJ, Cross C, Hope J, Jones HSR, McCarthy-Ryan M. Humans optimize ground contact time and leg stiffness to minimize the metabolic cost of running. Frontiers in Sports and Active Living. (2019) 1:53. doi: 10.3389/fspor.2019.00053
47. Fukuchi RK, Duarte M. Comparison of three-dimensional lower extremity running kinematics of young adult and elderly runners. J Sports Sci. (2008) 26(13):1447–54. doi: 10.1080/02640410802209018
48. Karamanidis K, Arampatzis A. Mechanical and morphological properties of different muscle-tendon units in the lower extremity and running mechanics: effect of aging and physical activity. J Exp Biol. (2005) 208:3907–23. doi: 10.1242/jeb.01830
49. Tseh W, Caputo JL, Morgan DW. Influence of gait manipulation on running economy in female distance runners. J Sports Sci Med. (2008) 7(1):91–5.24150139
50. Kyrolainen H, Komi PV, Belli A. Changes in muscle activity patterns and kinetics with increasing running speed. The Journal of Strength and Conditioning Research. (1999) 13(4):400–6. doi: 10.1519/1533-4287(1999)013-0400:CIMAPA%3E2.0.CO;2
51. Peikriszwili Tartaruga M, Brisswalter J, Peyré-Tartaruga L, Avila A, Alberton C, Coertjens M, et al. The relationship between running economy and biomechanical variables in distance runners. Res Q Exerc Sport. (2012) 83:367–75. doi: 10.5641/027013612802573012
52. Sinclair J, Taylor PJ, Edmundson CJ, Brooks D, Hobbs SJ. The influence of footwear kinetic, kinematic and electromyographical parameters on the energy requirements of steady state running. Mov Sport Sci - Sci Mot. (2013) 80(80):39–49. doi: 10.1051/sm/2012025
53. Moore IS, Jones AM, Dixon SJ. Mechanisms for improved running economy in beginner runners. Med Sci Sports Exercise. (2012) 44(9):1756–63. doi: 10.1249/MSS.0b013e318255a727
54. Williams KR, Cavanagh PR. Relationship between distance running mechanics, running economy, and performance. J Appl Physiol. (1987) 63(3):1236–45. doi: 10.1152/jappl.1987.63.3.1236
55. Arampatzis A, Brüggemann G-P, Metzler V. The effect of speed on leg stiffness and joint kinetics in human running. J Biomech. (1999) 32:1349–53. doi: 10.1016/S0021-9290(99)00133-5
56. Butler RJ, Crowell HP, McClay Davis I. Lower extremity stiffness: implications for performance and injury. Clin Biomech. (2003) 18:511–7. doi: 10.1016/S0268-0033(03)00071-8
57. Powell DW, Williams DSB. Changes in vertical and joint stiffness in runners with advancing age. The Journal of Strength & Conditioning Research. (2018) 32(12):3416. doi: 10.1519/JSC.0000000000001869
58. Pantoja PD, Morin JB, Peyré-Tartaruga LA, Brisswalter J. Running energy cost and spring-mass behavior in young versus older trained athletes. Med Sci Sports Exerc. (2016) 48(9):1779–86. doi: 10.1249/MSS.0000000000000959
59. Tarpenning KM, Hamilton-Wessler M, Wiswell RA, Hawkins SA. Endurance training delays age of decline in leg strength and muscle morphology. Med Sci Sports Exerc. (2004) 36(1):74. doi: 10.1249/01.MSS.0000106179.73735.A6
60. Diss CE, Greene A, Demmel IM. High levels of endurance training mitigate age-related changes in running biomechanics—a longitudinal study (2022).
61. Derrick TR, Dereu D, Mclean SP. Impacts and kinematic adjustments during an exhaustive run. Med Sci Sports Exerc. (2002) 34(6):998–1002. doi: 10.1097/00005768-200206000-00015
62. Devita P, Fellin RE, Seay JF, Ip E, Stavro N, Messier SP. The relationships between age and running biomechanics. Med Sci Sports Exerc. (2016) 48(1):98. doi: 10.1249/MSS.0000000000000744
63. Freedman Silvernail J, Boyer K, Rohr E, Brüggemann G-P, & Hamill J. Running mechanics and variability with aging. Med Sci Sports Exerc. (2015) 47(10):2175. doi: 10.1249/MSS.0000000000000633
Keywords: aging, running biomechanics, performance, lower-Limb kinematics, spatiotemporal analysis
Citation: Jamkrajang P, Suwanmana S, Limroongreungrat W and Verheul J (2023) Spatiotemporal and kinematic adjustments in master runners may be associated with the relative physiological effort during running. Front. Sports Act. Living 5:1271502. doi: 10.3389/fspor.2023.1271502
Received: 2 August 2023; Accepted: 15 September 2023;
Published: 10 October 2023.
Edited by:
Adamantios Arampatzis, Humboldt University of Berlin, GermanyReviewed by:
Chamnan Chinnasee, Thaksin University, ThailandKatya N. Mileva, London South Bank University, United Kingdom
© 2023 Jamkrajang, Suwanmana, Limroongreungrat and Verheul. This is an open-access article distributed under the terms of the Creative Commons Attribution License (CC BY). The use, distribution or reproduction in other forums is permitted, provided the original author(s) and the copyright owner(s) are credited and that the original publication in this journal is cited, in accordance with accepted academic practice. No use, distribution or reproduction is permitted which does not comply with these terms.
*Correspondence: Weerawat Limroongreungrat d2VlcmF3YXQubGltQG1haGlkb2wuYWMudGg= Jasper Verheul anB2ZXJoZXVsQGNhcmRpZmZtZXQuYWMudWs=