- 1Department of Neuroscience, Rehabilitation, Ophthalmology, Genetics and Maternal Child Health, Università Degli Studi di Genova, Genoa, Italy
- 2Centro Polifunzionale di Scienze Motorie, Università Degli Studi di Genova, Genoa, Italy
- 3Department of Experimental Medicine, Section of Human Physiology, Università Degli Studi di Genova, Genoa, Italy
- 4IRCCS Policlinico San Martino, Genoa, Italy
The aim of our study was to develop a methodology that uses the metronome to constrain the swimmers' stroke rate with the aim to monitor changes in stroke length (SL) during two different periods of the season. Thirteen young trained swimmers (15.7 ± 1.7 y) performed three 50 m front crawl time trials during pre-season (PRE) and after 2 months, during the in-season period (IN). They were asked: (I) to swim at their maximum intensity (NO-MET condition); (II) to synchronize their stroke with a metronome beat set to their preferred intra-stroke-interval (ISI) (100% condition, corresponding to 48 ± 0.7 cycles/min); (III) to synchronize their stroke with a metronome beat set at 5% higher than their preferred ISI (95% condition, corresponding to 51 ± 0.8 cycles/min). The outcome parameters used to evaluate the performance were ISI, SL and total time of 50 m (TT). In NO-MET condition, results showed that TT in IN improved with respect to PRE, but no changes in ISI and SL. In 100% condition, no differences were obtained between the imposed and the performed ISI, whilst in 95% condition, the performed ISI was lower than the metronome ISI, and lower than that in 100% condition. At last, when using the metronome, SL was higher during IN compared to PRE and SL was lower in the 95% condition compared to the 100% condition. Results indicate that the use of the metronome successfully allowed monitoring changes in SL during different periods of the season. This methodology provides valuable information to coaches and athletes to enhance their performance throughout the season.
1. Introduction
In the last years, swimmers and coaches adopted a scientific approach both to plan the competitive season and monitor the training sessions. In this context, scientific research has investigated different methodologies to monitor workload to evaluate physical effort and to collect data on the athlete's response to a specific training program (1). In swimming competition, the objective is to cover a given distance as fast as possible (2). Therefore, average swimming speed, defined by Craig et al. (3) as the product of the stroke length and the stroke rate, is considered one of the most representative measure to improve swimming performance (4). A number of studies showed that an inverse relationship exists between these two parameters namely an increase in stroke rate corresponds to a decrease in SL (3–8). Moreover, stroke rate and SL have been demonstrated to play a key role in monitoring the performance in front crawl competitions (8–11).
The search for the best performance and training has led to a rapid development of technology as support during athletic preparation. Thanks to specific devices, coaches are able to measure, evaluate and control the physiological and technical parameters essential to achieve the sport targets (12–16). Inertial sensors are an example of reliable tool used to monitor stroke rate and SL (14, 17, 18) as well as motion capture system based on video acquisition (19, 20) and infrared technology (21, 22). However, these systems are expensive and require specific expertise both during the acquisition phase and in data analysis. A recent survey of 298 coaches' swimmers (245 males, 53 females) from the United States identified ease of use, accessibility and easy of understanding data as the main characteristics for a monitoring tool while reported the lack of finances, time restrictions and accessibility to suitable testing equipment as the main limitations (23, 24). A device that includes all the above-mentioned requirements is the metronome which provides an instant audio feedback through which athletes modulate their stroke rate (7, 25, 26). Indeed, the metronome is an electronic training device that beeps to help to develop a consistent pace. The device is a cheap, small, waterproof, floatable, and easy wearable tool that can be positioned under the swimming cap. Furthermore, it can be used by athletes and coaches also in open water where, due to the environment, it is more difficult to keep pace compared to the swimming pool (27). Since, as suggested by the scientific literature (23, 28), coaches' perception about the training and evaluation methodology is crucial, this method might answer coaches’ needs and will allow them to efficiently plan the training season. At last, human tendency to synchronize with an acoustic stimulation could explain the potential benefits of using acoustic rhythmical cues during physical practice and sport due to the existence of a central pattern generator in the nervous system, which may serve to regulate temporal functioning and govern rhythm response (29–31).
In the last few years, although some criticisms related to the higher physiological stress and distraction have been evidenced in using the metronome during swimming (25, 26), an increasing number of studies used it during training to optimize stroke rate with the aim to customize the best race strategy (25). Some of them have used metronome to pace the swimming speed and the device emits a sound at designated time intervals, equivalent to the end of each lap (32–35). Other studies have used metronome to evaluate inter-limb coordination (26, 36–38). Another way in which the metronome can be used is as a tool to constrain stroke rate by imposing an intra-stroke-interval (ISI), as already proposed by Alberty and colleagues (26) and Silva and colleagues (7).
The aim of the study was to test the possibility to use the metronome to constrain the inter-stroke-interval to measure changes in stroke length during two different periods of the training season. We hypothesize that, only when using the metronome, changes in SL will be evident, whilst when swimming without metronome this will be not possible.
2. Material and methods
2.1. Participants
An a-priori power analysis was conducted using G*Power (version 3.1.9.7) (39) to determine the minimum sample size required to test the study hypothesis. The effect size was set at 0.25 considered to be medium using Cohen's criteria (40). A F-test considering one group assessing the interaction between PERIOD (n = 2) and CONDITION (n = 2) was applied with a significance criterion of α = 0.05 and power = 0.8. The minimum sample size needed was N = 11 for detecting differences in discrimination sensitivity among measurements. Thirteen swimmers from the same team (7 males and 6 females; mean ± standard deviation age 15.7 ± 1.7 years; body mass 58.9 ± 6.9 kg; height 171.1 ± 7.6 cm) volunteered to participate in this study. According to McKay et al. (41) these athletes can be classified as trained/developmental level. They had a competitive swim experience of 7.4 ± 1.6 years, a swimming training frequency of 5 ± 1 session/wk with a total of 10.0 ± 2 h, with a swimming training volume of 30,000 ± 6,000 m/wk during the current season. The training planning includes both high-intensity and high-volume sessions for all participants. Personal-best time in the 50 m freestyle event was 27.33 ± 1.22 s, which corresponds to 536.8 ± 64.0 FINA Points, performance level 4 (42). The study was carried out in accordance with the code of ethics of the World Medical Association (Declaration of Helsinki 2014) for experiments involving humans. The project was approved by the local ethics committee (Comitato Etico per la Ricerca di Ateneo, University of Genova, Italy. N. 2020/21) and written consent was obtained from participants' parents.
2.2. Experimental design
The experimental protocol was conducted during pre-season (PRE) and 2 months later, i.e., the in-season period (IN), in a 50 m indoor swimming pool with water temperature at 27 °C. During the 2 months between PRE and IN, swimmers followed their regular training. In both periods, swimmers performed three 50 m front crawl trials with a push-off start and 6 min of rest between trials to attempt total recovery (43). Before each test, swimmers performed a moderate intensity 1,000 m swim warm-up (44).
In the first trial, participants were asked to swim at their maximum intensity, namely as fast as possible, without the use of metronome (NO-MET condition). The intra-stroke-interval (ISI) was considered as the time between the head emerged from the water (thus excluding the underwater phase) until the end of the lap, divided by the number of strokes. Since ISI quantifies the duration of a single stroke, it also indirectly gives a measure for stroke rate. ISI values of each subject were used as individual reference value to set the interval between two consecutive metronome beats in two conditions: in 100% condition the metronome was set at the mean ISI value corresponding to MET100, whilst in 95% condition the metronome was set at 95% of ISI (MET95), thus imposing a faster stroke rate. In the second trial, participants were asked to swim in synchronous with the audio feedback provided by a waterproof metronome (Tempo Trainer Pro, Finis) positioned under their swimming cap and set at MET100. In the text we will use the acronyms PRE100 when referring to swimmers' data acquired in pre-season, and IN100 when referring to those acquired in in-season. In the third trial, the metronome was set at MET95. In the text we will use the acronyms PRE95 when referring to data acquired in pre-season, and IN95 when referring to IN. In IN, MET100 and MET95 were the same as those imposed in in-season (Figure 1).
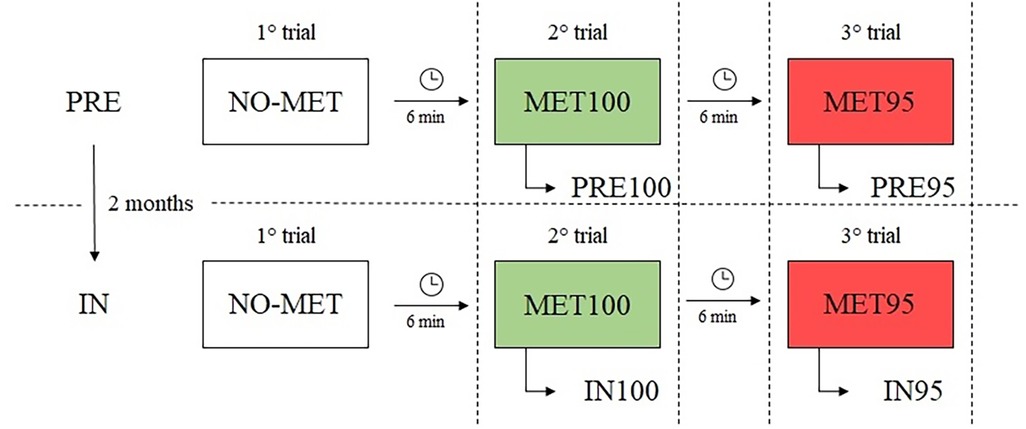
Figure 1. Experimental protocol. Athletes swim 3 times 50 m front crawl during pre-season (PRE) and after 2 months, during in-season (IN). During the first trial swimming was performed without using the metronome (NO-MET, white boxes). The second trial was performed with the metronome set to the individual mean intra-stroke-interval measured in NO-MET (MET100, green boxes). The third trial was performed with metronome set to 95% of the individual intra-stroke-interval (MET95, red box). PRE100 and PRE95 correspond to data collected during PRE at MET100 and MET95, respectively. IN100 and IN95 correspond to data collected during IN at MET100 and MET95, respectively.
2.3. Data analysis
Outcome measures used to evaluate swimming performance were: the mean ISI value (s), and the 50 m total time (TT, s; manually recorded by a stopwatch 3X300M Stopwatch, Finis) (45). Furthermore, the meters covered during underwater phase (m), defined as the distance between the wall and the point where the head breaks the surface, evaluated by means of a video camera—GoProR HERO5- at 120 fps and with a resolution of 720 pixels, located at the starting side of pool was used (46) data were analysed with Kinovea, were assessed. At last, the stroke count (SC) were used to compute the stroke length (SL, m) using the following equation: [lap distance (50 m)—underwater phase (m)] / SC. We referred to the work of Girold and colleagues, who used the number of stroke cycles to compute the stroke length (47).
2.4. Statistical analysis
Normality was checked by means of the Shapiro-Wilk test. All variables were normally-distributed. Normally distributed data are reported as mean values ± standard error (SE). In NO-MET condition, TT, SL and ISI acquired in PRE and IN periods were compared by means of paired t-test. With the aim to test if participants swam synchronously with the metronome in the two periods of the season and at the two conditions, we applied on ISI values recorded in 100% condition an ANOVA comparing MET100 (namely, the period imposed by the metronome) with PRE100 and IN100 (namely, swimmers' ISI values recorded during the trials), and a second ANOVA on ISI values acquired at 95% condition comparing MET95 with PRE95 and IN95. With the aim to compare ISI and SL when using the metronome in the two periods of the season, an ANOVA with CONDITION (2 levels, 100% and 95%) and PERIOD (2 levels, PRE and IN) as within subject factors was applied. Newman–Keuls post hoc tests were applied in case of significant interaction. Hedges' g and partial η2 values were used to measure the effect size when applying t-test and ANOVA, respectively. Concerning Hedges' g, 0.2, 0.5 and 0.8 represent small, medium and large effect, respectively. Concerning partial η2, 0.01, 0.06, and 0.14 indicated small, medium, or large effects, respectively (48). Linear association between SL and ISI were tested by means of Pearson's correlation coefficient (r) analysis. Data from 100% and 95% condition were pooled together. The level of statistical significance was set at p ≤ .05. Statistical analyses were performed with SPSS20.
3. Results
3.1. NO-MET condition
The statistical analyses showed an improvement in TT during IN (30.57 ± 0.49 s) with respect to PRE (31.04 ± 0.44 s) [t(12)= 2.40, p = 0.03, g = 0.28]. No changes were observed in ISI [PRE: 0.62 ± 0.01 s; IN: 0.61 ± 0.01 s; t(12) = 0.93, p = 0.37, g = 0.22] and SL [PRE: 1.00 ± 0.02 m; IN: 1.00 ± 0.02 m; t(12) = 0.51, p = 0.62, g = 0.11].
3.2. Comparison between ISI imposed by the metronome and participants’ ISI
ANOVA on ISI recorded in 100% condition showed no main effect [F(2,24)= 2.47, p = 0.11, η2 = 0.17]. Differently, a main effect on ISI in 95% condition was found [F(2,24) = 8.79 p = 0.001, η2 = 0.42]. Post hoc analysis showed that PRE95 (0.61 ± 0.01 s, p = 0.002) and IN95 (0.61 ± 0.01 s, p = 0.004) were higher with respect MET95 (0.59 ± 0.01 s) (Figure 2).
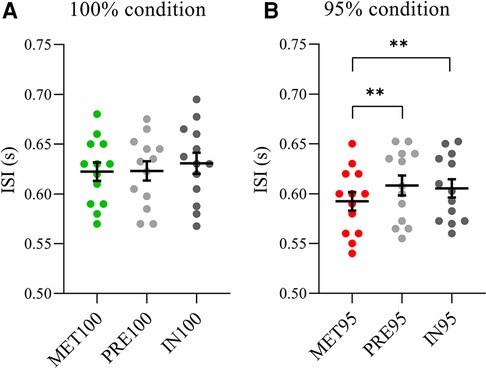
Figure 2. Comparison between ISI imposed by the metronome and participants’ ISI. Intra-stroke-interval (ISI, s) when the metronome was set to the individual time between strokes (100% condition) (A) and to its 95% (95% condition) (B) Comparison between the metronome values (MET100, green circles or MET95, red circles) and ISI acquired in pre-season (PRE100 during 100% condition; PRE95 during 95% condition, light grey circles) and in-season (IN100 during 100% condition; IN95 during 95% condition, dark grey circles). Horizontal bars represent the mean values and error bars indicate the standard error of the mean. ** p < 0.01.
3.3. Statistical assessment of ISI and Sl in presence of metronome
ANOVA on ISI highlighted a main effect of CONDITION [F(1,12) = 41.61, p < 0.001, η2 = 0.78] and indicated a lower ISI value in 95% (0.61 ± 0.01 s) with respect to 100% (0.63 ± 0.01 s). No main effect of PERIOD was observed [F(1,12) = 0.55, p = 0.47, η2 = 0.04]. ANOVA on SL showed a main effect of CONDITION [F(1,12) = 12.50 p = 0.004, η2 = 0.51] that indicated a lower SL value in 95% (0.96 ± 0.07 m) with respect to 100% (0.99 ± 0.06 m). A main effect of PERIOD [F(1,12) = 7.71, p = 0.017, η2 = 0.39], with SL larger in IN (0.99 ± 0.06 m) than in PRE (0.96 ± 0.06 m) was observed (Figure 3). Positive linear relationships between ISI and SL were found in PRE (r = 0.56, p = 0.003) and IN (r = 0.44, p = 0.025) (Figure 4).
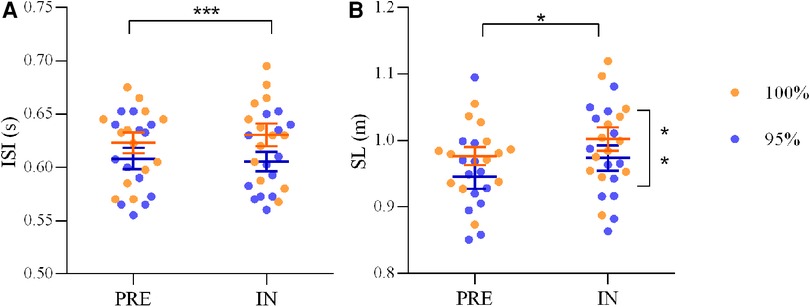
Figure 3. Statistical assessment of ISI and SL in presence of metronome. Comparison between intra-stroke-interval (ISI, s) values (A) and stroke length (SL, m) (B) in the two period of the season (PRE and IN) during 100% condition (orange circles) and 95% condition (blues circles). * p < 0.05, ** p < 0.01, *** p < 0.001.
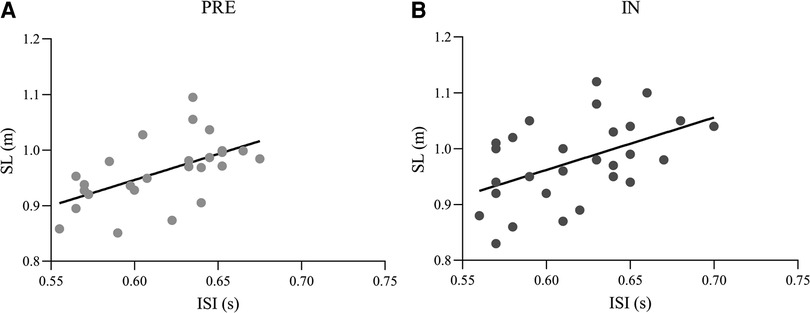
Figure 4. Linear relationship between intra-stroke-interval (ISI) and stroke length (SL) in pre-season (PRE) (A) and in-season (iN) (B).
4. Discussion
Results of this study showed that, in NO-MET condition, TT improved during the IN period with respect to PRE period with no changes in SL and ISI. When the metronome was imposed, participants were able to swim in synchronous with the time set by the device only during the 100% condition (imposed stroke rate 48 ± 0.7 cycles/min). Although during 95% condition (imposed stroke rate 51 ± 0.8 cycles/min) ISI was lower than in 100% condition, swimmers were not able to follow the metronome. SL was higher during IN than PRE period and lower during the 95% with respect to the 100% condition. Finally, a linear relationship between ISI and SL in both PRE and IN periods was found.
The beneficial effects of the auditory information on movement control and timing, as well as movement execution, have been shown by several studies (49). Clinical researchers highlighted the use of the metronome to facilitate rehabilitation of intrinsically rhythmical movements including an improvement in gait velocity, step cadence and stride length reducing gait variability (50–55). On the other hand, the use of a specific tool that provides sounds has been shown to favour the optimization of both the movement control and its execution in sports disciplines such as rowing (56), cycling (57) and swimming (58). In swimming research, the metronome was used during training to constrain the stroke rate (6, 7, 26, 59) and the training pacing (32, 34, 35, 60).
In the present study, we proposed a methodology that used the metronome to constrain swimmers' stroke rate and measure the changes in SL in two different periods of the season (PRE and IN) and with two different values of ISI (100% and 95% conditions). Monitoring changes in stroke length is important when considering the results of the studies by Craig et al. (3) and Letzelter et al. (5), who reported that faster swimmers showed longer SL than slower swimmers. Considering the present results, when athletes were free to swim at their own pace, TT improved during IN compared to PRE period suggesting that the regular training performed by the swimmers was effective in improving their performance. However, going deeply into the reasons of this improvement, in NO-MET condition no changes of the parameters describing the performance (i.e., ISI or SL) were found. For this reason, it was not possible to understand what caused the TT improvement. On the other hand, when swimmers were asked to synchronize their strokes with the acoustic stimulus provided by the metronome, it was possible to quantify the changes in SL. The SL was found to be greater in IN period compared to PRE period. Therefore, one might conclude that this methodology has been effective in revealing a change of a parameter crucial for the swimming performance that is not possible to observe in free swimming, except when one uses expensive technologies, challenging to use and not at disposal of all the swimming teams. With respect to these tools, the metronome has the advantage to be a cheap and easy-to-use device, available to all coaches and athletes.
It is worth to note that SL was greater in 100% than in 95% condition. Indeed, when athletes were asked to deviate from their preferred stroke rate, decreasing the ISI value, the stroke efficiency decreased, thus detrimentally influencing the SL. This was also confirmed by the correlations between ISI and SL in both PRE and IN periods, in accordance with a previous study that reported that the improvement in TT was achieved by the combination of increases/decreases in stroke rate and SL (61).
Another point that needs to be discussed is athletes' ability to synchronize their strokes with the acoustic stimulus provided by the metronome. Results showed that they managed to follow the beat of the MET100, namely their preferred stroke rate. Instead, when they were asked to swim at a higher pace, following the beat of the MET95, swimmers tried to speed up the strokes, as demonstrated by the difference between the conditions, but they were unable to follow the metronome. This confirms what has been shown by previous studies where the stroke rate error (error between imposed and preferred stroke rate) was the lowest at the preferred stroke rate (6, 60). Thus, by using the metronome it was possible to unveil that athletes were not able to swim at a rate 5% higher than the own rate. Since previous studies showed a positive relationship between stroke rate and strength (9, 62), this result could be attributed to the need to increment muscle strength. Since the increase in stroke rate is one of the primary factors determining the swimming performance (62), it could be speculated that thanks to this methodology it is possible to quantify how much swimmers can increase the stroke rate, and therefore plan specific training characterized by low-volume and high-velocity/force intensity aimed at improving this performance parameter (63, 64).
Limitations
A possible limitation of this study is that the proposed methodology was tested only on athletes competing at trained/developmental level. Thus, we are not aware of its effectiveness when applied on athletes of other levels. Future studies to verify the reliability of this metronome-based methodology in other categories are needed. Another limitation is that we neither evaluated their level of maturation nor collected detailed information on the anthropometrics characteristics, except body mass and height. Indeed, both stroke rate and stroke length might be influenced by these aspects.
Conclusion
In conclusion, the use of the metronome allowed us to quantify the changes of one of the key performance parameters, namely the stroke length. Our findings suggest that coaches and young trained swimmers can monitor with this small, waterproof, easy to set up and economic tool, both the stroke length and the stroke rate during different periods of the training season and then customize their best race strategy. At last, since swimmers were not able to swim with a slower ISI (95% condition), it would be useful to introduce during the in-season training period, resistance exercises characterized by a low-volume and high-velocity/force intensity.
Data availability statement
The raw data supporting the conclusions of this article will be made available by the authors, without undue reservation.
Ethics statement
The studies involving humans were approved by Comitato Etico per la Ricerca di Ateneo, University of Genova, Italy. The studies were conducted in accordance with the local legislation and institutional requirements. Written informed consent for participation in this study was provided by the participants’ legal guardians/next of kin.
Author contributions
MF: Conceptualization, Data curation, Formal Analysis, Investigation, Writing – original draft. AB: Conceptualization, Formal Analysis, Supervision, Writing – original draft. LP: Methodology, Writing – review & editing. MB: Methodology, Writing – original draft. FT: Methodology, Writing – original draft. EF: Writing – review & editing. PR: Writing – review & editing. MB: Conceptualization, Formal Analysis, Supervision, Writing – original draft.
Funding
The author(s) declare that no financial support was received for the research, authorship, and/or publication of this article.
Conflict of interest
The authors declare that the research was conducted in the absence of any commercial or financial relationships that could be construed as a potential conflict of interest.
The author(s) declared that they were an editorial board member of Frontiers, at the time of submission. This had no impact on the peer review process and the final decision.
Publisher's note
All claims expressed in this article are solely those of the authors and do not necessarily represent those of their affiliated organizations, or those of the publisher, the editors and the reviewers. Any product that may be evaluated in this article, or claim that may be made by its manufacturer, is not guaranteed or endorsed by the publisher.
References
1. Mujika I. Quantification of training and competition loads in endurance sports: methods and applications. Int J Sports Physiol Perform. (2017) 12:9–17. doi: 10.1123/ijspp.2016-0403
2. McGibbon KE, Pyne DB, Shephard ME, Thompson KG. Pacing in swimming: a systematic review. Sports Med. (2018) 48:1621–33. doi: 10.1007/s40279-018-0901-9
3. Craig AB Jr, Skehan PL, Pawelczyk JA, Boomer WL. Velocity, stroke rate, and distance per stroke during elite swimming competition. Med Sci Sports Exerc. (1985) 17(6):625–34. doi: 10.1249/00005768-198512000-00001
4. Craig AB, Pendergast DR. Relationships of stroke rate, distance per stroke, and velocity in competitive swimming. Med Sci Sports. (1979) 11:278–83.522640
5. Letzelter H, Freitag W. Stroke length and stroke frequency variations in men’s and women’s 100-m freestyle swimming. Biomecha Med Swim. (1986) 14:315–22.
6. Simbaña-Escobar D, Hellard P, Seifert L. Influence of stroke rate on coordination and sprint performance in elite male and female swimmers. Scand J Med Sci Sports. (2020) 30:2078–91. doi: 10.1111/sms.13786
7. Silva AF, Figueiredo P, Morais S, Vilas-Boas JP, Fernandes RJ, Seifert L. Task constraints and coordination flexibility in young swimmers. Motor Control. (2019) 23(4):535–52. doi: 10.1123/mc.2018-0070
8. Morais JE, Barbosa TM, Bragada JA, Nevill AM, Marinho DA. Race analysis and determination of stroke frequency—stroke length combinations during the 50-M freestyle event. J Sports Sci Med. (2023) 22:156–65. doi: 10.52082/jssm.2023.156
9. Girold S, Maurin D, Dugué B, Chatard JC, Millet G. Effects of dry-land vs. resisted- and assisted-sprint exercises on swimming sprint performances. J Strength Cond Res. (2007) 21(2):599–605. doi: 10.1519/R-19695.1
10. Aspenes S, Kjendlie PL, Hoff J, Helgerud J. Combined strength and endurance training in competitive swimmers. J Sports Sci Med. (2009) 8(3):357–65. http://www.jssm.org.24149998
11. Kwok WY, So BCL, Tse DHT, Ng SSM. A systematic review and meta-analysis: biomechanical evaluation of the effectiveness of strength and conditioning training programs on front crawl swimming performance. J Sports Sci Med. (2021) 20:564–85. doi: 10.52082/jssm.2021.564
12. Faelli E, Strassera L, Ottobrini S, Ferrando V, Bisio A, Puce L, et al. Not breathing during the approach phase ameliorates freestyle turn performance in prepubertal swimmers. Front Sports Act Living. (2021) 3:731953. doi: 10.3389/fspor.2021.731953
13. Barbosa TM, Barbosa AC, Simbaña Escobar D, Mullen GJ, Cossor JM, Hodierne R, et al. The role of the biomechanics analyst in swimming training and competition analysis. Sports Biomechan. (2021) 1–18. doi: 10.1080/14763141.2021.1960417 [Epub ahead of print].
14. Mooney R, Corley G, Godfrey A, Quinlan LR, ÓLaighin G. Inertial sensor technology for elite swimming performance analysis: a systematic review. Sensors (Switzerland). (2015) 16:18. doi: 10.3390/s16010018
15. Mooney R, Corley G, Godfrey A, Osborough C, Quinlan L, Laighin G. Application of video-based methods for competitive swimming analysis: a systematic review. Sports Exerc Med. (2015) 1(5):133–50. doi: 10.17140/SEMOJ-1-121
16. Santos CC, Marinho DA, Neiva HP, Costa MJ. Propulsive forces in human competitive swimming: a systematic review on direct assessment methods. Sports Biomech. (2021):1–21. doi: 10.1080/14763141.2021.1953574 [Epub ahead of print].
17. Magalhaes FA, Vannozzi G, Gatta G, Fantozzi S. Wearable inertial sensors in swimming motion analysis: a systematic review. J Sports Sci. (2015) 33:732–45. doi: 10.1080/02640414.2014.962574
18. Félix ER, da Silva HP, Olstad BH, Cabri J, Correia PL. Swimbit: a novel approach to stroke analysis during swim training based on attitude and heading reference system (ahrs). Sports (Basel). (2019) 7:238. doi: 10.3390/sports7110238
19. Millet G, Chollet D, Chalies S, Chatard J. Coordination in front crawl in elite triathletes and elite swimmers. Int J Sports Med. (2002) 23:99–104. doi: 10.1055/s-2002-20126
20. Figueiredo P, Sanders R, Gorski T, Vilas-Boas JP, Fernandes RJ. Kinematic and electromyographic changes during 200 m front crawl at race pace. Int J Sports Med. (2013) 34(1):49–55. doi: 10.1055/s-0032-1321889
21. Olstad BH, Vaz JR, Zinner C, Cabri JMH, Kjendlie PL. Muscle coordination, activation and kinematics of world-class and elite breaststroke swimmers during submaximal and maximal efforts. J Sports Sci. (2017) 35:1107–17. doi: 10.1080/02640414.2016.1211306
22. Washino S, Mayfield DL, Lichtwark GA, Mankyu H, Yoshitake Y. Swimming performance is reduced by reflective markers intended for the analysis of swimming kinematics. J Biomech. (2019) 91:109–13. doi: 10.1016/j.jbiomech.2019.05.017
23. Mooney R, Corley G, Godfrey A, Osborough C, Newell J, Quinlan LR, et al. Analysis of swimming performance: perceptions and practices of US-based swimming coaches. J Sports Sci. (2016) 34(11):997–1005. doi: 10.1080/02640414.2015.1085074
24. Callaway AJ, Cobb JE, Jones I. A comparison of video and accelerometer based approaches applied to performance monitoring in swimming. Int J Sports Sci Coach. (2002) 4:139–53. doi: 10.1260/1747-9541.4.1.139
25. Buchheit M, Laursen PB. High-intensity interval training, solutions to the programming puzzle: Part I: cardiopulmonary emphasis. Sports Med. (2013) 43(5):313–38. doi: 10.1007/s40279-013-0029-x
26. Alberty M, Potdevin F, Dekerle J, Pelayo P, Gorce P, Sidney M. Changes in swimming technique during time to exhaustion at freely chosen and controlled stroke rates. J Sports Sci. (2008) 26(11):1191–200. doi: 10.1080/02640410801974984
27. Zacca R, Neves V, da Silva Oliveira T, Soares S, Rama LMPL, de Souza Castro FA, et al. 5 km front crawl in pool and open water swimming: breath-by-breath energy expenditure and kinematic analysis. Eur J Appl Physiol. (2020) 120(9):2005–18. doi: 10.1007/s00421-020-04420-7
28. Costa MJ, Marinho DA, Santos CC, Quinta-Nova L, Costa AM, Silva AJ, et al. The coaches’ perceptions and experience implementing a long-term athletic development model in competitive swimming. Front Psychol. (2021) 12:685584. doi: 10.3389/fpsyg.2021.685584
29. Schneider S, Askew CD, Abel T, Strüder HK. Exercise, music, and the brain: is there a central pattern generator? J Sports Sci. (2010) 28:1337–43. doi: 10.1080/02640414.2010.507252
30. Terry PC, Curran ML, Karageorghis CI, Martin OV, Parsons-Smith R. Supplemental material for effects of music in exercise and sport: a meta-analytic review. Psychol Bull. (2020) 146:91. doi: 10.1037/bul0000216.supp
31. Bove M, Strassera L, Faelli E, Biggio M, Bisio A, Avanzino L, et al. Sensorimotor skills impact on temporal expectation: evidence from swimmers. Front Psychol. (2017) 8:1714. doi: 10.3389/fpsyg.2017.01714
32. Martin L, Whyte GP. Comparison of critical swimming velocity and velocity at lactate threshold in elite triathletes. Int J Sports Med. (2000) 21:366–8. doi: 10.1055/s-2000-3786
33. Martin L, Thompson K. Reproducibility of diurnal variation in sub-maximal swimming. Int J Sports Med. (2000) 21:387–92. doi: 10.1055/s-2000-3829
34. Turner AP, Smith T, Coleman SGS. Use of an audio-paced incremental swimming test in young national-level swimmers. Int J Sports Physiol Perform. (2008) 3:68–79. doi: 10.1123/ijspp.3.1.68
35. Thompson KG, MacLaren DPM, Lees A, Atkinson G. Accuracy of pacing during breaststroke swimming using a novel pacing device, the aquapacer™. J Sports Sci. (2002) 20:537–46. doi: 10.1080/026404102760000044
36. McLean SP, Palmer D, Ice G, Truijens M, Smith JC. Oxygen uptake response to stroke rate manipulation in freestyle swimming. Med Sci Sports Exerc. (2010) 42:1909–13. doi: 10.1249/MSS.0b013e3181d9ee87
37. Van Houwelingen J, Roerdink M, Huibers AV, Evers LLW, Beek PJ. Pacing the phasing of leg and arm movements in breaststroke swimming to minimize intra-cyclic velocity fluctuations. PLoS One. (2017) 12:e0186160. doi: 10.1371/journal.pone.0186160
38. Yamakawa KK, Shimojo H, Takagi H, Tsubakimoto S, Sengoku Y. Kinematic and EMG data during underwater dolphin kick change while synchronizing with or without synchronization of kick frequency with the beat of a metronome. Data Brief. (2017) 14:28–31. doi: 10.1016/j.dib.2017.07.027
39. Faul F, Erdfelder E, Lang A-G, Buchner A. G*power 3: a flexible statistical power analysis program for the social, behavioral, and biomedical sciences. Behav Res Methods. (2007) 39:175–91. doi: 10.3758/BF03193146
40. Cohen J. Statistical power analysis. Curr Dir Psychol Sci. (1992) 1:98–101. doi: 10.1111/1467-8721.ep10768783
41. McKay AK, Stellingwerff T, Smith ES, Martin DT, Mujika I, Goosey-Tolfrey VL, et al. Defining training and performance caliber: a participant classification framework. Int J Sports Physiol Perform. (2021) 17(2):317–31. doi: 10.1123/ijspp.2021-0451
42. Ruiz-Navarro JJ, López-Belmonte Ó, Gay A, Cuenca-Fernández F, Arellano R. A new model of performance classification to standardize the research results in swimming. Eur J Sport Sci. (2023) 23:478–88. doi: 10.1080/17461391.2022.2046174
43. Hancock AP, Sparks KE, Kullman EL. Postactivation potentiation enhances swim performance in collegiate swimmers. J Strength Cond Res. (2015) 29:912–7. doi: 10.1519/JSC.0000000000000744
44. Neiva HP, Marques MC, Barbosa TM, Izquierdo M, Marinho DA. Warm-up and performance in competitive swimming. Sports Med. (2014) 44:319–30. doi: 10.1007/s40279-013-0117-y
45. Gonjo T, McCabe C, Coleman S, Soares S, Fernandes RJ, Vilas-Boas JP, et al. Do swimmers conform to criterion speed during pace-controlled swimming in a 25-m pool using a visual light pacer? Sports Biomech. (2021) 20(6):651–64. doi: 10.1080/14763141.2019.1572781
46. Qiu X, Fuente BD, Lorenzo A, Veiga S. Comparison of starts and turns between individual and relay swimming races. Int J Environ Res Public Health. (2021) 18:4740. doi: 10.3390/ijerph18094740
47. Girold S, Jalab C, Bernard O, Carette P, Kemoun G, Dugué B. Dry-land strength training vs. electrical stimulation in sprint swimming performance. J Strength Cond Res. (2012) 26(2):497–505. doi: 10.1519/JSC.0b013e318220e6e4
48. Cohen J. Statistical power analysis for the behavioral sciences. 2nd edition. Erlbaum, Hillsdale, NJ: Lawrence Erlbaum Associates (1988).
49. Schaffert N, Janzen TB, Mattes K, Thaut MHA. Review on the relationship between sound and movement in sports and rehabilitation. Front Psychol. (2019) 10:244. doi: 10.3389/fpsyg.2019.00244
50. Thaut MH, McIntosh GC, Rice RR, Miller RA, Rathbun J, Brault JM. Rhythmic auditory stimulation in gait training for Parkinson’s disease patients. Movement Disorders: Official Journal of the Movement Disorder Society. (1996) 11(2):193–200. doi: 10.1002/mds.870110213
51. Arias P, Cudeiro J. Effects of rhythmic sensory stimulation (auditory, visual) on gait in Parkinson’s disease patients. Exp Brain Res. (2008) 186:589–601. doi: 10.1007/s00221-007-1263-y
52. Arias P, Cudeiro J. Effect of rhythmic auditory stimulation on gait in Parkinsonian patients with and without freezing of gait. PLoS One. (2010) 5(3):e9675. doi: 10.1371/journal.pone.0009675
53. Song J-H, Zhou P-Y, Song J-H, Zhou P-Y. Rhythmic auditory stimulation with visual stimuli on motor and balance function of patients with Parkinson’s disease. Eur Rev Med Pharmacological Sci. (2015) 19:2001–7.
54. Hove MJ, Suzuki K, Uchitomi H, Orimo S, Miyake Y. Interactive rhythmic auditory stimulation reinstates natural 1/f timing in gait of Parkinson’s patients. PLoS One. (2012) 7:e32600. doi: 10.1371/journal.pone.0032600
55. Pau M, Corona F, Pili R, Casula C, Sors F, Agostini T, et al. Effects of physical rehabilitation integrated with rhythmic auditory stimulation on spatio-temporal and kinematic parameters of gait in Parkinson’s disease. Front Neurol. (2016) 7:126. doi: 10.3389/fneur.2016.00126
56. Schaffert N, Mattes K, Effenberg AO. Listen to the boat motion: acoustic information for elite rowers. In Human interaction with auditory displays-proceedings of the interactive sonification workshop. (2010). p. 31–8.
57. Sigrist R, Fox S, Riener R, Wolf P. Benefits of crank moment sonification in cycling. Procedia Eng. (2016) 147:513–8. (Elsevier Ltd). doi: 10.1016/j.proeng.2016.06.230
58. Chollet D, Madani M, Micallef JP. Effects of two types of biomechanical bio-feedback on crawl performance. Biomech Med Swim. (1992) Swimming Science VI: 57–62.
59. Potdevin F, Bril B, Sidney M, Pelayo P. Stroke frequency and arm coordination in front crawl swimming. Int J Sports Med. (2006) 27:193–8. doi: 10.1055/s-2005-837545
60. Seifert L, Komar J, Crettenand F, Dadashi F, Aminian K, Millet GP. Inter-limb coordination and energy cost in swimming. J Sci Med Sport. (2014) 17(4):439–44. doi: 10.1016/j.jsams.2013.07.003
61. Wakayoshi K, Yoshida T, Ikuta Y, Mutoh Y, Miyashita M. Adaptations to six months of aerobic swim training. Changes in velocity, stroke rate, stroke length and blood lactate. Int J Sports Med. (1993) 14(7):368–72. doi: 10.1055/s-2007-1021194
62. Girold S, Calmels P, Maurin D, Milhau N, Chatard J-C. Assisted and resisted sprint training in swimming. J Strength Cond Res. (2006) 20:547–54. doi: 10.1519/R-16754.1
63. Crowley E, Harrison AJ, Lyons M. The impact of resistance training on swimming performance: a systematic review. Sports Med. (2017) 47:2285–307. doi: 10.1007/s40279-017-0730-2
Keywords: test, training, swimming performance, pacing, timing
Citation: Fassone M, Bisio A, Puce L, Biggio M, Tassara F, Faelli E, Ruggeri P and Bove M (2023) The metronome-based methodology to monitor the stroke length changes in trained swimmers. Front. Sports Act. Living 5:1268146. doi: 10.3389/fspor.2023.1268146
Received: 2 August 2023; Accepted: 6 October 2023;
Published: 17 October 2023.
Edited by:
Mário J. Costa, University of Porto, PortugalReviewed by:
Catarina C. Santos, Research Centre in Sports Sciences, Health Sciences and Human Development (CIDESD), PortugalAna Gay, University of Granada, Spain
© 2023 Fassone, Bisio, Puce, Biggio, Tassara, Faelli, Ruggeri and Bove. This is an open-access article distributed under the terms of the Creative Commons Attribution License (CC BY). The use, distribution or reproduction in other forums is permitted, provided the original author(s) and the copyright owner(s) are credited and that the original publication in this journal is cited, in accordance with accepted academic practice. No use, distribution or reproduction is permitted which does not comply with these terms.
*Correspondence: Ambra Bisio ambra.bisio@unige.it Marco Bove marco.bove@unige.it