- 1Faculty of Physical Education and Sport, Charles University, Prague, Czech Republic
- 2Department of Sport Science, University of Innsbruck, Innsbruck, Austria
- 3Faculty of Education, Arts and Sports, Western Norway University of Applied Sciences, Sogndal, Norway
Introduction: It is acknowledged that training during recovery periods after injury involves reducing both volume and intensity, often resulting in losses of sport-specific fitness. Therefore, this study aimed to compare the effects of high-intensity training (HIT) and low-intensity training with blood flow restriction (LIT + BFR) of the finger flexors in order to preserve climbing-specific strength and endurance.
Methods: In a crossover design, thirteen intermediate climbers completed two 5-week periods of isometric finger flexors training on a hangboard. The trainings consisted of ten LIT + BFR (30% of max) or HIT sessions (60% of max without BFR) and were undertaken in a randomized order. The training session consisted of 6 unilateral sets of 1 min intermittent hanging at a 7:3 work relief ratio for both hands. Maximal voluntary contraction (MVC), force impulse from the 4 min all out test (W), critical force (CF) and force impulse above the critical force (W') of the finger flexors were assessed before, after the first, and after the second training period, using a climbing-specific dynamometer. Forearm muscle oxidative capacity was estimated from an occlusion test using near-infrared spectroscopy at the same time points.
Results: Both training methods led to maintaining strength and endurance indicators, however, no interaction (P > 0.05) was found between the training methods for any strength or endurance variable. A significant increase (P = 0.002) was found for W, primarily driven by the HIT group (pretest—25078 ± 7584 N.s, post-test—27327 ± 8051 N.s, P = 0.012, Cohen's d = 0.29). There were no significant (P > 0.05) pre- post-test changes for MVC (HIT: Cohen's d = 0.13; LIT + BFR: Cohen's d = −0.10), CF (HIT: Cohen's d = 0.36; LIT + BFR = 0.05), W` (HIT: Cohen's d = −0.03, LIT + BFR = 0.12), and forearm muscle oxidative capacity (HIT: Cohen's d = −0.23; LIT + BFR: Cohen's d = −0.07).
Conclusions: Low volume of BFR and HIT led to similar results, maintaining climbing-specific strength and endurance in lower grade and intermediate climbers. It appears that using BFR training may be an alternative approach after finger injury as low mechanical impact occurs during training.
Introduction
Sport climbers heavily rely on finger flexor contractions, making finger flexor strength and endurance crucial predictors of climbing performance (1, 2). Previous research has extensively investigated the physiological adaptations induced by high-intensity training (HIT) on finger strength and endurance (3, 4). For example, specific maximal strength and hypertrophy training designed for climbers have demonstrated significant increases in finger flexor strength and endurance after 5–10 weeks of training (5–8). However, HIT of the finger flexors may increase the risk of injuries in the fingers, hands, elbows, or shoulders, with chronic injuries being the most common among sport climbers (9, 10). Moreover, when recovering from injuries such as pulley ruptures or strains it is recommended to gradually increase training loads (11). Consequently, recovery periods require climbers to train with decreased intensity, often resulting in losses of sport-specific fitness.
An alternative approach to HIT for improving or maintaining finger strength and muscle hypertrophy is training at low intensities (typically 20%–40% of maximum strength) with blood flow restriction (LIT + BFR), achieved by applying external pressure to the limb proximal to the working muscle (12). LIT + BFR exercise creates a localized hypoxic environment and promotes recruitment of both types I and II muscle fibres, leading to enhanced muscle strength and power (13–15). Furthermore, changes in key markers of protein synthesis, such as mTOR and HIF-1, support the observed adaptations in the muscle following LIT + BFR training (16, 17). Accordingly, LIT + BFR triggers an upregulation of protein synthesis, facilitating muscle growth and strength gains despite the use of lower training loads (decreased mechanical stress). This suggests that the metabolic stress induced by LIT + BFR exercise can stimulate muscle protein synthesis to a comparable extent as high-intensity exercise (18, 19). To date there are no studies comparing HIT and LIT + BFR in climbing-specific hangboard resistance training. However, based on the existing literature, it is reasonable to hypothesize that LIT + BFR and HIT may yield comparable effects in finger flexors training in climbers.
Previous research has shown that increasing strength can be achieved with low volume of HIT per week (20, 21). However, it remains unknown whether the same training volume of LIT + BFR would yield similar effects. Most studies investigating blood flow restriction (BFR) interventions have primarily focused on designs maximizing their effectiveness for increasing muscle strength and hypertrophy (22, 23). However, during the recovery period following an injury, the primary objective of training is to maintain strength and endurance levels using minimal load and training volume (20). Low-intensity training (LIT) with BFR training has been proposed and utilized as a method of recovery after various types of injuries in lower limbs such as knee osteoarthritis (24) or arthroplasty (25), however, to authors best knowledge, there is not any literature available on this topic on the upper extremities related to the climbing.
Therefore, the objective of this study was to investigate the effects of low volume of LIT + BFR training and HIT on maintaining climbing-specific strength and endurance. We hypothesised that HIT and LIT + BFR will be equally effective in preserving sport specific strength and endurance in intermediate climbers.
Methods
Participants
Thirteen lower grade to intermediate climbers [6 male, 7 female participants: males—age, 24.3 ± 2.0 yrs; climbing ability level 13 ± 4 IRCRA (International Rock Climbing Research Association) grade; females—age, 32.6 ± 12.5 yrs; climbing ability 9 ± 2 IRCRA grade] volunteered to take part in the study. Participants self-reported their climbing ability using French/Sport grade which was transformed to the IRCRA difficulty scale ranging from 1 to 32 (26). At the beginning, all participants completed written informed consent forms and medical health questionnaires. Exclusion criteria included venous thrombosis, cardiovascular diseases (including high blood pressure and diabetes), unexplained chest pain, heart pathologies, and fainting during physical activities. Additionally, participants with carpal tunnel syndrome, acute upper limb injuries, tendosynovitis, or tendon injuries in the upper limb, pregnancy, or in the injury recovery phase were also excluded.
Participants were instructed to abstain from engaging in any strenuous exercise, consuming caffeine, and consuming alcohol within 24 h before each experimental testing session. Furthermore, participants were not allowed to maintain normal training routine or engage in any finger flexor strength and endurance training. This was achieved partially by the ongoing COVID lockdown when sport facilities were closed. Additionally, participants were asked to continue their regular dietary and supplement habits. The study was approved by Ethics Committee of Charles University, Faculty of Physical Education and Sport. The participants provided their written informed consent to participate in this study.
Experimental protocol
The 13 weeks long experimental protocol is depicted at Figure 1. All participants completed two 5 weeks periods of finger flexors training in a cross-over randomized order with a 1-week long washout period. The two training interventions consisted of either isometric HIT or LIT + BFR on a hangboard. Testing climbing specific strength and endurance was applied before and after each period of training (Figure 1).
To eliminate interference between individual tests, the participants underwent two separate testing sessions during the testing week. In the first session, the muscle oxidative capacity and the maximal voluntary contraction (MVC) were assessed. The second testing session involved performing a 4-min all-out test after the measurement of blood pressure to determine the level of occlusion.
Upon their first visit, participants were randomly assigned into two groups based on the training intervention. They were also familiarized with the laboratory setup. Additionally, they completed a questionnaire and signed the medical consent form. In the questionnaire, participants reported their climbing ability as proposed by Draper et al. (26).
Warm-up
All subjects completed a standardized self-directed warm-up prior to the assessment and training protocol. The warm-up consisted of three minutes of pulse-raising activity, such as jogging or cycling, followed by three minutes of climbing, which is considered a sport-specific activity. In addition, the warm-up included a series of 5:5 s work-to-rest ratio hangs on the testing edge in a half-crimp position at ∼50% of the perceived maximum force (27).
Training interventions
Both training interventions consisted of 10 training sessions (2 sessions per week during each 5-week period). The LIT + BFR and HIT participants previously scheduled a time of the day for the individual sessions of hangboard strength exercises. The intensity for each training type was based on the MVC tested prior to each intervention. The training was performed on the same wooden rung as for testing MVC and all-out test (see below) in standing position with arms ∼180° flexed in shoulder, and slightly flexed in elbows. Participants applied the target force on the rung by hanging (bending the knees). The online feedback of applied force was visible on the screen of the testing/training device (1D-SAC, Spacelab, Sofia, Bulgaria).
Blood flow restriction training
To implement BFR, we utilized a cuff provided by Occlude ApS (Aarhaus, Denmark). Prior to each training session, the cuff was inflated to 60% of the complete arterial occlusion pressure (21, 28) on training arm, which caused decrease in the blood flow in the downstream vascular system by 47%–48% (29). In each session both arms performed 6 sets over two blocks (one block consisted of three consecutive sets) unilaterally for each arm, and each set comprising 6 repetitions performed at 30% of MVC, with a work-to-rest ratio of 7 to 3 s. Following the completion of set 3 (60 s rest in between) for one arm, the cuff was deflated and participants immediately continued with the other arm for next three sets. In total, 36 isometric contractions for each arm were completed (Figure 2). The cuff pressure was monitored and controlled during the rest periods between sets.
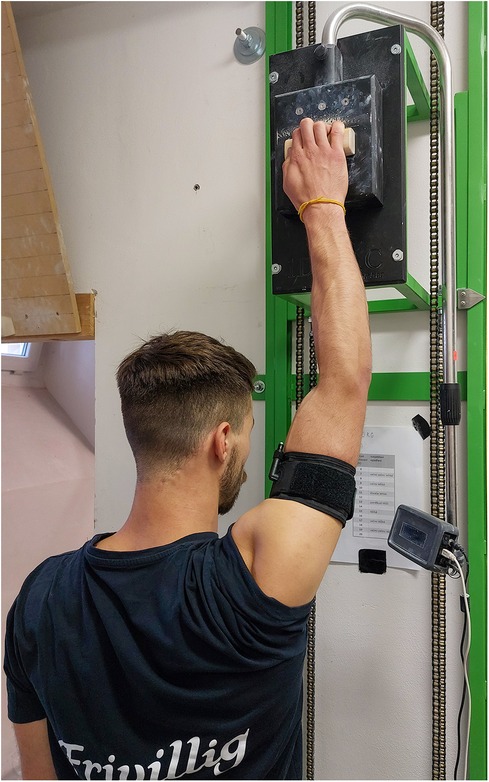
Figure 2. Position of participant during the Low-intensity training with the blood flow restriction.
High-Intensity training
Participants performed HIT sessions at 60% of their MVC. The same volume of training as for LIT + BFR was applied. Each training session consisted of 12 working sets (i.e., 6 sets of each arm divided into two blocks with 5 min rest in between), with each set comprising 6 repetitions and a work-to-rest ratio of 7 to 3 s. Following the completion of the third set, participants were given a 5 min recovery period while the other arm was exercising.
Testing climbing specific strength and endurance
Maximal strength
The maximal strength of the finger flexors was determined using a custom-made dynamometer (1D-SAC, Spacelab, Sofia, Bulgaria). The participant was instructed to maintain a 5 s long half-crimp position while “hanging” on the wooden rung. The rung depth was 23 mm with a 10 mm radius to maximize the activation of the flexor digitorum profundus (FDP) and flexor digitorum superficialis (FDS) (30). Two attempts were performed separated by a two-minute rest in between. Participants were instructed to progressively transfer as much of their weight as possible onto the wooden rung with their dominant arm. The highest peak value from the two trials was considered as the MVC of finger flexors, and this value was used to determine relative workloads for the following training intervention.
All-out test
To assess the critical force (CF), force impulse from all contractions (W), and impulse above the critical force (W'), the 4-min all-out test was performed (31). This test involved 24 isometric maximal voluntary contractions on the same rung as for maximal strength (1D-SAC, Spacelab, Sofia, Bulgaria) in a half crimp position with a 7:3 s work to rest ratio.
During the “rest” phase, participants were instructed to maintain the anatomical position with upper-limb over the head level and were not allowed to shake their forearms or hands, as shaking is known to aid recovery (32). However, participants could dry their fingers using the chalk. Loud verbal encouragements were given to all participants to reach their maximum force during every contraction. Force and time data were continuously recorded throughout the test. For the visual representation see Figure 3.
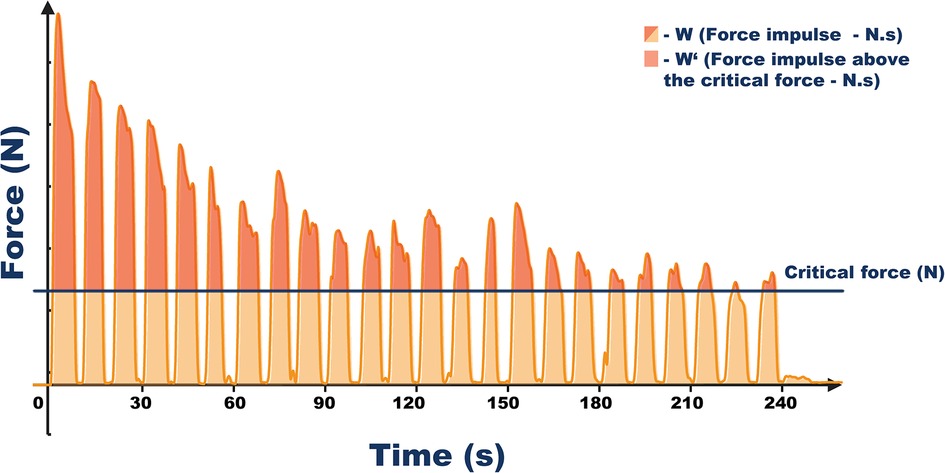
Figure 3. Vizualization of data acquired by the all-out test for the finger flexors. Critical force was calculated as the average force from the last three contractions. The duration of the all-out test was 240 s with 7:3 work to rest ratio. Force impulse from all contractions was calculated as the area under the force-time curve and represents total isometric muscle work during the test (W). Impulse above the critical force represents energy store component (W’).
For each contraction in all tests, the length (in seconds), peak and mean force (in kilograms), and the impulse were determined. The CF was defined as the mean force from the last three contractions of the test.
Muscle oxidative capacity
To assess the muscle oxidative capacity, near-infrared spectroscopy (NIRS) (Portamon, Artinis Medical Systems BV, The Netherlands) was employed to monitor changes in tissue oxygenation levels of the FDP. A chartered physiotherapist located the FDP using the technique recommended by Schweizer and Hudek (30), where the thumb and first finger were squeezed together, and the middle of the muscle belly was palpated (30). The NIRS device sampling frequency was set to 10 Hz and data were processed using the Oxysoft software (Artinis Medical System, BV, The Netherlands). Path length factor was set to 4. Muscle oxidative capacity was estimated by calculating half-time to recovery of the tissue oxygen saturation (O2HTR) after arterial occlusion (33).
Participants were instructed to rest in a supine position with their arm elevated above heart level for 20 min after fitting the artery tourniquet. Following the initial measurement of the baseline, the tourniquet was inflated to a supramaximal pressure of 250 mmHg for 5 min. After that, the cuff was rapidly released, and recovery muscle tissue oxygen saturation (StO2) values were recorded for 3 min. Half-time of StO2 recovery was calculated, which represents a valid estimate of oxidative capacity (33).
Statistical analysis
Statistical analyses were performed using IBM SPSS for Windows (IBM Corp. Released 2020. IBM SPSS Statistics for Windows, Version 27.0. Armonk, NY: IBM Corp). Descriptive statistics (mean ± standard deviation) were used to characterize strength and endurance indicators during pretest and post-test. The analysis of variance (ANOVA) 2 × 2 with repeated measures was conducted to examine the main effects of time (pretest vs. post-test) and training method (LIT + BFR vs. HIT), as well as their interaction effect. The significance level was set at P < 0.05. Post hoc analysis using Bonferroni correction was performed to compare specific pairs of interventions in terms of their effects on the pretest and post-test measures. Effect sizes of 0.3, 0.5, and 0.8 were interpreted as small, medium, and large effects, respectively (34). Utilizing the Shapiro-Wilk test, all data were determined to be normal and met the criteria of Mauchly's test of sphericity.
Results
At baseline, no differences for were observed between the training methods for any of the variables (P > 0.05).
There was a significant main effect of time for impulse (delta W = + 1568 Ns; P = 0.002). However, there was no significant interaction of time and training method demonstrating no substantial differences between LIT + BFR and HIT (P = 0.057–0.855).
Pairwise comparisons showed significant increases of force impulse only for HIT method (Table 1, Figure 4). Otherwise, non-significant improvements with small or no effect size were found for all strength and endurance indicators and no significant decreases of climbing specific strength or endurance indicators were demonstrated (Table 1, Figure 4).
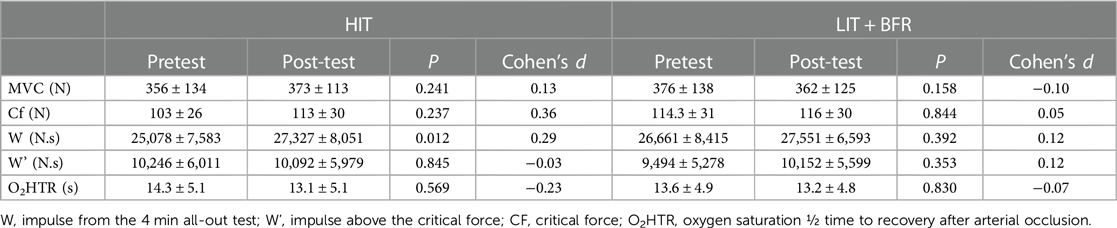
Table 1. Mean (± standard deviation) score of pretest and post-test measurements for high intensity training (HIT) and low intensity training with blood flow restrictions (LIT + BFR).
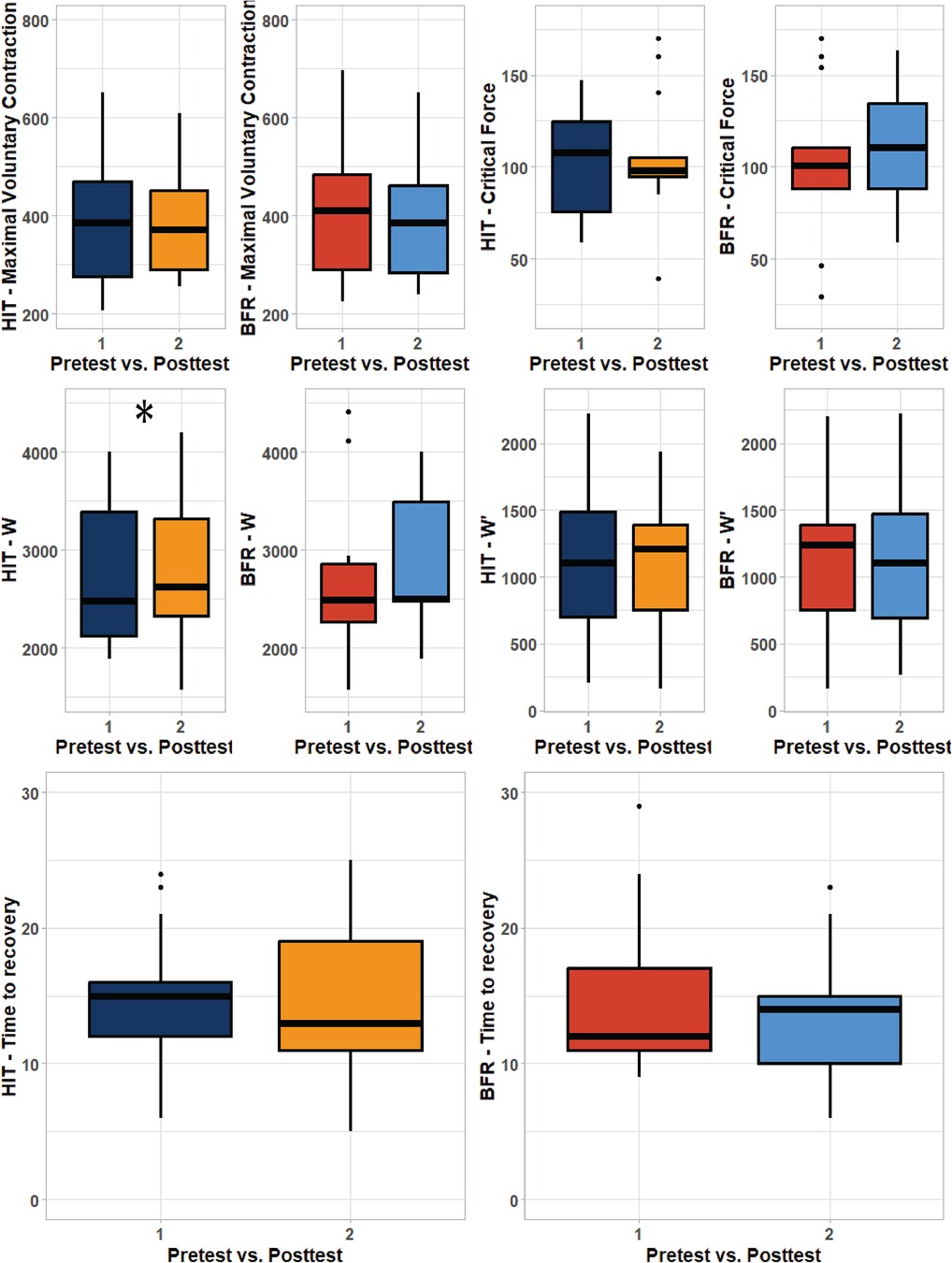
Figure 4. Boxplot visualization of pretest post-test results. Left panel represent high intensity training (HIT) while right panels represent low intensity training with blood flow restriction (LIT + BFR) The area of box shows quartile and whiskers represent 1.5 interquartile range between the first and third quartile. The line in the middle corresponds to the mean value. W—impulse, W’—impulse above the critical force, O2HTR—oxygen ½ time to recovery after occlusion. * represents significant improvements from pretest (P < 0.05).
Discussion
The main finding of the current study was that small volume of LIT + BFR was equally effective as HIT to maintain finger flexor strength and endurance in lower grade and intermediate climbers.
To evaluate maximum finger flexor strength, we employed an ecological setting with the arm positioned overhead without any fixation. This method has been demonstrated to be a valid and reliable measure of climbing-specific strength, with a standard error of measurement (SEM) of 35 N (35). Neither the HIT, nor LIT + BFR interventions resulted in significant changes in finger flexor strength. The observed pretest-post-test changes fell within the previously mentioned SEM range. It has been observed that strength decreases occur rapidly with a training interruption, becoming more pronounced after 8 days of inactivity (36) It is hypothesized that neural factors such as motor unit recruitment and synchronization, firing frequency, and intramuscular coordination are responsible for strength losses during the early stages of inactivity, while morphological factors contribute to greater strength decreases thereafter (37). Our study demonstrates that low volume of intermittent isometric HIT (60% MVC, with a total exercise time of 36 × 10:3 s work: relief cycles per session, two sessions per week) and an equivalent volume of low-intensity with BFR (30% MVC) were effective in maintaining the initial strength level for 5 weeks. All participants were able to sustain both training protocols without premature localized exhaustion. Therefore, it may be speculated that 2 sessions per week, with a total of 12 min of isometric non-exhaustive exercise per arm at low intensity and with BFR, counteracted the deteriorating changes that neural factors may have on maximal strength due to inactivity.
During high-intensity resistance training, a single set of 6–12 repetitions with loads ranging from approximately 70%–85% 1 repetition maximum 2–3 times per week reaching volitional or momentary failure for 8–12 weeks can produce suboptimal, yet significant increases in squat and bench press strength in resistance-trained men (20). Our non-exhaustive protocol with smaller muscle groups, slightly lower intensity, and similar volume did not result in significant improvements. It appears that exhaustive protocols are necessary to induce structural changes leading to strength increases (38, 39). However, a similar volume of non-exhaustive exercise may have benefits in maintaining the current level of strength.
LIT + BFR training does not only have impact on maximal strength improvements but may also, due to peripheric and central adaptations, have direct or indirect impact on endurance performance (40, 41). In our study, we estimated endurance of the finger flexors using several indicators: W, W', CF from 4 min all out test and O2HTR from arterial occlusion test. W is an indicator of total working capacity and represents an overall measure of finger strength and endurance. W’ is the capacity to release energy above the CF and is often related to strength-endurance capacity while the level of CF represents the amount of energy predominantly released by aerobic metabolism (42). O2HTR is a standardized NIRS derived functional index estimating muscle aerobic capacity. Faster recovery of FDP has been associated with increased climbing ability (43). Similar to maximal strength, no decreases in any endurance indicators were observed. On the contrary, after HIT, W was statistically higher, suggesting that low volume of HIT may lead to overall improvement in finger flexor working capacity in intermediate climbers as W represents both strength and endurance components. However, the effect size for improvement changes was low, and no differences between the two methods were found. The maintenance of all endurance indicators during 5-weeks LIT + BFR training is very promising as submaximal resistance to fatigue appears to be deteriorated to a greater extent from training interruption in comparison with maximal force and maximal power (37).
Endurance adaptations following LIT + BFR training have been associated with improvements in macro- and microvascular functions, muscle redox and ionic buffering, and mitochondrial respiratory capacity (40, 41). In our study, the aerobic capacity of the finger flexor muscles was estimated from the NIRS signal. It is important to note that the sensitivity of StO2 recovery as a training indicator in climbers is still unknown, and further experimental studies are needed to validate its use. Subsequent studies should also aim to investigate the pathways explaining forearm oxidative capacity and consider using NIRS technology to independently assess skeletal muscle oxygen diffusion capacity and mitochondrial respiratory capacity (44).
There are other strength and limitations to be stated. A strength of the study is that all participants refrained from engaging in any climbing-specific or upper-body strength activities during the 13-week experimental period, ensuring that any observed changes could be attributed to our experimental conditions. The intervention may be regarded as a simulation of a rehabilitation period. Participants were fit enough to train under controlled environment but could not train/climb in an uncontrolled environment due to lock-down restrictions. The crossover design allowed for a direct comparison between the two training modalities within the same group of participants, minimizing inter-individual variability (45). However, due to time requirements, a relatively short one-week washout period between the training interventions was applied. Of note, a control group was not included which might be useful of quantifying no strength training or the short washout period. Nevertheless, this does not seem to influence our results as no changes in any indicator were observed after the HIT or LIT + BFR intervention. The small group size in this study may limit the generalizability of the findings and the ability to detect small differences between the training modalities. Moreover, using BFR with more advanced climbers may have provided different results. MVC was assessed only once before each training intervention to set the training load. In other words, the climbers trained at the same relative intensity throughout the whole period. This may also explain the lack of changes during the different periods. If MVC was tested every week, there may had been a progression in the training which ultimately may have led to an increase in (some of) the variables. On the other hand, during recovery periods from an injury, regular testing of MVC would increase stress on injured tissues and may slow the recovery process.
Our findings support the hypothesis that both approaches, with and without BFR, were equally effective in preserving the studied parameters during the minimal training period. However, it is important to note that physiology of these adaptations may differ during exercise at 30% of MVC compared to higher intensity exercise (23, 46, 47). Therefore, BFR training at a lower intensity (30% of MVC) appears to be a viable substitute for HIT during recovery periods and may offer advantages, particularly for climbers recovering from injuries, although it is more discomforting and less enjoyable compared to HIT (48).
In conclusion, this study demonstrates that low volume of non-exhaustive BFR training at a lower intensity can be as effective as HIT in preserving sport-specific strength and endurance. These findings suggest that LIT + BFR training may be a viable alternative for climbers recovering from injuries.
Data availability statement
The original contributions presented in the study are included in the article/Supplementary Material, further inquiries can be directed to the corresponding author.
Ethics statement
The studies involving humans were approved by Ethics Committee of Charles University, Faculty of Physical Education and Sport. The studies were conducted in accordance with the local legislation and institutional requirements. The participants provided their written informed consent to participate in this study. Written informed consent was obtained from the individual(s) for the publication of any potentially identifiable images or data included in this article.
Author contributions
TJ: Writing – original draft, Conceptualization, Investigation, Data curation. AS: Writing – review & editing, Methodology. VA: Methodology, Writing – review & editing. JB: Conceptualization, Methodology, Supervision, Writing – review & editing.
Funding
The author(s) declare financial support was received for the research, authorship, and/or publication of this article.
The study was supported by the grant of Charles University, Cooperatio Program, research area Sport Sciences—Biomedical and Rehabilitation Medicine. As a university-grant, the funding did not influence the design, results, or interpretation of this study.
Conflict of interest
The authors declare that the research was conducted in the absence of any commercial or financial relationships that could be construed as a potential conflict of interest.
Publisher's note
All claims expressed in this article are solely those of the authors and do not necessarily represent those of their affiliated organizations, or those of the publisher, the editors and the reviewers. Any product that may be evaluated in this article, or claim that may be made by its manufacturer, is not guaranteed or endorsed by the publisher.
Supplementary material
The Supplementary Material for this article can be found online at: https://www.frontiersin.org/articles/10.3389/fspor.2023.1256136/full#supplementary-material
References
1. Maciejczyk M, Michailov ML, Wiecek M, Szymura J, Rokowski R, Szygula Z, et al. Climbing-Specific exercise tests: energy system contributions and relationships with sport performance. Front Physiol. (2022) 12:787902. doi: 10.3389/fphys.2021.787902
2. Philippe M, Wegst D, Müller T, Raschner C, Burtscher M. Climbing-specific finger flexor performance and forearm muscle oxygenation in elite male and female sport climbers. Eur J Appl Physiol. (2012) 112(8):2839–47. doi: 10.1007/s00421-011-2260-1
3. Langer K, Simon C, Wiemeyer J. Strength training in climbing: a systematic review. J Strength Cond Res. (2023) 37(3):751–67. doi: 10.1519/JSC.0000000000004286
4. Thompson EB, Farrow L, Hunt JE, Lewis MP, Ferguson RA. Brachial artery characteristics and micro-vascular filtration capacity in rock climbers. Eur J Sport Sci. (2015) 15(4):296–304. doi: 10.1080/17461391.2014.940560
5. Hermans E, Saeterbakken AH, Vereide V, Nord ISO, Stien N, Andersen V. The effects of 10 weeks hangboard training on climbing specific maximal strength, explosive strength, and finger endurance. Front Sports Act Living. (2022) 4:888158. doi: 10.3389/fspor.2022.888158
6. Levernier G, Laffaye G. Four weeks of finger grip training increases the rate of force development and the maximal force in elite and top world-ranking climbers. J Strength Cond Res. (2019) 33(9):2471–80. doi: 10.1519/JSC.0000000000002230
7. López-Rivera E, González-Badillo JJ. Comparison of the effects of three hangboard strength and endurance training programs on grip endurance in sport climbers. J Hum Kinet. (2019) 66:183–95. doi: 10.2478/hukin-2018-0057
8. Medernach JP, Kleinöder H, Lötzerich HH. Fingerboard in competitive bouldering: training effects on grip strength and endurance. J Strength Cond Res. (2015) 29(8):2286–95. doi: 10.1519/JSC.0000000000000873
9. Barrile AM, Feng S, Nesiama J, Huang C. Injury rates, patterns, mechanisms, and risk factors among competitive youth climbers in the United States. Wilderness Environ Med. (2022) 33(1):25–32. doi: 10.1016/j.wem.2021.09.005
10. Grønhaug G. Self-reported chronic injuries in climbing: who gets injured when ? Open Sport Exerc Med. (2018) 4(1):1–6. doi: 10.1136/bmjsem-2018-000406
11. Lum ZC, Park L. Rock climbing injuries and time to return to sport in the recreational climber. J Orthop. (2019) 16(4):361–3. doi: 10.1016/j.jor.2019.04.001
12. Patterson SD, Hughes L, Warmington S, Burr J, Scott BR, Owens J, et al. Blood flow restriction exercise: considerations of methodology, application, and safety. Front Physiol. (2019) 10:533. doi: 10.3389/fphys.2019.00533
13. BjØrnsen T, Wernbom M, Kirketeig A, Paulsen G, SamnØy L, BÆkken L, et al. Type 1 muscle fiber hypertrophy after blood flow-restricted training in powerlifters. Med Sci Sports Exerc. (2019) 51(2):288–98. doi: 10.1249/MSS.0000000000001775
14. Reece TM, Godwin JS, Strube MJ, Ciccone AB, Stout KW, Pearson JR, et al. Myofiber hypertrophy adaptations following 6 weeks of low-load resistance training with blood flow restriction in untrained males and females. J Appl Physiol. (2023) 134(5):1240–55. doi: 10.1152/japplphysiol.00704.2022
15. Wang J, Mogensen AG, Thybo F, Brandbyge M, Brorson J, van Hall G, et al. Low-load blood flow-restricted resistance exercise produces fiber type-independent hypertrophy and improves muscle functional capacity in older individuals. J Appl Physiol. (2023) 134(4):1047–62. doi: 10.1152/japplphysiol.00789.2022
16. Fujita S, Abe T, Drummond MJ, Cadenas JG, Dreyer HC, Sato Y, et al. Blood flow restriction during low-intensity resistance exercise increases S6K1 phosphorylation and muscle protein synthesis. J Appl Physiol. (2007) 103(3):903–10. doi: 10.1152/japplphysiol.00195.2007
17. Panzhinskiy E, Culver B, Ren J, Bagchi D, Nair S. Chapter 22 - Role of mammalian target of rapamycin (mTOR) in muscle growth. In: Bagchi D, Nair S, Sen CK, editors. Nutrition and enhanced sports performance. San Diego: Academic Press (2013). p. 217–27. doi: 10.1016/B978-0-12-396454-0.00022-9
18. Barbieri E, Sestili P. Reactive oxygen Species in skeletal muscle signaling. J Signal Transduct. (2012) 2012:1–17. doi: 10.1155/2012/982794
19. Steinbacher P, Eckl P. Impact of oxidative stress on exercising skeletal muscle. Biomolecules. (2015) 5(2):356–77. doi: 10.3390/biom5020356
20. Androulakis P, James K, James PF. The Minimum effective training dose required to increase 1RM strength in resistance - trained men: a systematic review and meta - analysis. Sport Med. (2020) 50(4):751–65. doi: 10.1007/s40279-019-01236-0
21. Das A, Paton B. Is there a minimum effective dose for vascular occlusion during blood flow restriction training? Front Physiol. (2022) 13:838115. doi: 10.3389/fphys.2022.838115
22. Abe T, Fujita S, Nakajima T, Sakamaki M, Ozaki H, Oga-sawara R, et al. Effects of low-intensity cycle training with restricted leg blood flow on thigh muscle volume and VO2max in young men. J Sport Sci Med. (2010) 9(April):452–8.
23. Abe T, Kearns CF, Sato Y. Muscle size and strength are increased following walk training with restricted venous blood flow from the leg muscle, Kaatsu-walk training. J Appl Physiol. (2006) 100(5):1460–6. doi: 10.1152/japplphysiol.01267.2005
24. Ferraz RB, Gualano B, Rodrigues R, Kurimori CO, Fuller R, Lima FR, et al. Benefits of resistance training with blood flow restriction in knee osteoarthritis. Med Sci Sport Exerc. (2018) 50(5):897–905. doi: 10.1249/MSS.0000000000001530
25. Franz A, Ji S, Bittersohl B, Zilkens C, Behringer M. Impact of a six-week prehabilitation with blood-flow restriction training on pre- and postoperative skeletal muscle mass and strength in patients receiving primary total knee arthroplasty. Front Physiol. (2022) 13:881484. doi: 10.3389/fphys.2022.881484
26. Draper N, Giles D, Schöffl V, Fuss FK, Watts PB, Wolf P, et al. Comparative grading scales, statistical analyses, climber descriptors and ability grouping: international rock climbing research association position statement. Sports Technol. (2016) 8(3-4):88–94. doi: 10.1080/19346182.2015.1107081
27. Baláš J, Kodejška J, Krupková D, Hannsmann J, Fryer S. Reliability of near-infrared spectroscopy for measuring intermittent handgrip contractions in sport climbers. J Strength Cond Res. (2018) 32(2):494–501. doi: 10.1519/JSC.0000000000002341
28. Sieljacks P, Matzon A, Wernbom M, Ringgaard S, Vissing K, Overgaard K. Muscle damage and repeated bout effect following blood flow restricted exercise. Eur J Appl Physiol. (2016) 116(3):513–25. doi: 10.1007/s00421-015-3304-8
29. Singer TJ, Stavres J, Elmer SJ, Kilgas MA, Pollock BS, Kearney SG, et al. Knee extension with blood flow restriction : impact of cuff pressure on hemodynamics. Eur J Appl Physiol. (2020) 120(1):79–90. doi: 10.1007/s00421-019-04250-2
30. Schweizer A, Hudek R. Kinetics of crimp and slope grip in rock climbing. J Appl Biomech. (2011) 27(2):116–21. doi: 10.1123/jab.27.2.116
31. Giles D, Hartley C, Maslen H, Hadley J, Taylor N, Torr O, et al. An all-out test to determine finger flexor critical force in rock climbers. Int J Sports Physiol Perform. (2021) 16(7):942–9. doi: 10.1123/ijspp.2020-0637
32. Baláš J, Michailov M, Giles D, Kodejška J, Panáčková M, Fryer S, et al. Active recovery of the finger flexors enhances intermittent handgrip performance in rock climbers performance in rock climbers. Eur J Sport Sci. (2016) 16(7):764–72. doi: 10.1080/17461391.2015.1119198
33. Fryer S, Stoner L, Giles KSD, Sveen J, Garrido I. Forearm muscle oxidative capacity index predicts sport rock - climbing performance. Eur J Appl Physiol. (2016) 116(8):1479–84. doi: 10.1007/s00421-016-3403-1
34. Lakens D. Calculating and reporting effect sizes to facilitate cumulative science: a practical primer for t-tests and ANOVAs. Front Psychol. (2013) 4:863. doi: 10.3389/fpsyg.2013.00863
35. Michailov ML, Baláš J, Tanev SK, Stoyanov H, Kodejška J, Brown L. Reliability and validity of finger strength and endurance measurements in rock climbing reliability and validity of finger strength and endurance measurements in rock. Res Q Exerc Sport. (2018) 89(2):246–54. doi: 10.1080/02701367.2018.1441484
36. Wortman RJ, Brown SM, Savage-elliott I, Finley ZJ, Mulcahey MK. Blood flow restriction training for athletes: a systematic review. Am J Sports Med. (2021) 49(7):1938–44. doi: 10.1177/0363546520964454
37. Bosquet L, Berryman N, Dupuy O, Mekary S, Arvisais D, Bherer L, et al. Effect of training cessation on muscular performance: a meta-analysis. Scand J Med Sci Sports. (2013) 23(3):e140–9. doi: 10.1111/sms.12047
38. Hermans E, Andersen V, Saeterbakken AH. The effects of high resistance-few repetitions and low resistance-high repetitions resistance training on climbing performance. Eur J Sport Sci. (2017) 17(4):378–85. doi: 10.1080/17461391.2016.1248499
39. Schoenfeld BJ, Wilson JM, Lowery RP, Krieger JW. Muscular adaptations in low-versus high-load resistance training: a meta-analysis. Eur J Sport Sci. (2016) 16(1):1–10. doi: 10.1080/17461391.2014.989922
40. Horiuchi M, Stoner L, Poles J. The effect of four weeks blood flow restricted resistance training on macro - and micro - vascular function in healthy, young men. Eur J Appl Physiol. (2023) 123:2179–89. doi: 10.1007/s00421-023-05230-3
41. Pignanelli C, Petrick HL, Keyvani F, Heigenhauser GJF, Quadrilatero J, Holloway GP, et al. Low-load resistance training to task failure with and without blood flow restriction: muscular functional and structural adaptations. Am J Physiol Regul Integr Comp Physiol. (2020) 318(2):R284–95. doi: 10.1152/ajpregu.00243.2019
42. Giles D, Chidley JB, Taylor N, Torr O, Hadley J, Randall T, et al. The determination of finger-flexor critical force in rock climbers. Int J Sports Physiol Perform. (2019) 14(7):972–9. doi: 10.1123/ijspp.2018-0809
43. Fryer S, Stone KJ, Sveen J, Dickson T, Giles D, Baláš J, et al. Differences in forearm strength, endurance, and hemodynamic kinetics between male boulderers and lead rock climbers. Eur J Sport Sci. (2017) 17(9):1177–83. doi: 10.1080/17461391.2017.1353135
44. Pilotto AM, Adami A, Mazzolari R, Brocca L, Crea E, Zuccarelli L, et al. Near-infrared spectroscopy estimation of combined skeletal muscle oxidative capacity and O2 diffusion capacity in humans. J Physiol. (2022) 18:4153–68. doi: 10.1113/JP283267
45. Nolan SJ, Hambleton I, Dwan K. The use and reporting of the cross-over study design in clinical trials and systematic reviews: a systematic assessment. Plos One. (2016) 69:1–14. doi: 10.1371/journal.pone.0159014
46. Cook SB, Scott BR, Hayes KL, Murphy BG. Neuromuscular adaptations to low-load blood flow restricted resistance training. J Sports Sci Med. (2018) 17(1):66–73.29535579
47. Ratamess NA, Brent A, Evetoch TK, Housh TJ. Progression models in resistance training for healthy adults. Med Sci Sport Exerc. (2009) 41(3):687–708. doi: 10.1249/MSS.0b013e3181915670
Keywords: injury, hypertrophy, hypoxia, ischemia, intermittent exercise, isometric contraction, strength, oxidative capacity
Citation: Javorský T, Saeterbakken AH, Andersen V and Baláš J (2023) Comparing low volume of blood flow restricted to high-intensity resistance training of the finger flexors to maintain climbing-specific strength and endurance: a crossover study. Front. Sports Act. Living 5:1256136. doi: 10.3389/fspor.2023.1256136
Received: 10 July 2023; Accepted: 18 September 2023;
Published: 29 September 2023.
Edited by:
Emiliano Cè, University of Milan, ItalyReviewed by:
Shannon Siegel, University of San Francisco, United StatesChristian Doria, University of Milan, Italy
© 2023 Javorský, Saeterbakken, Andersen and Baláš. This is an open-access article distributed under the terms of the Creative Commons Attribution License (CC BY). The use, distribution or reproduction in other forums is permitted, provided the original author(s) and the copyright owner(s) are credited and that the original publication in this journal is cited, in accordance with accepted academic practice. No use, distribution or reproduction is permitted which does not comply with these terms.
*Correspondence: Jiří Baláš YmFsYXNAZnR2cy5jdW5pLmN6