Erratum: Future opportunities for the athlete biological passport
- 1Research and Expertise in Anti-Doping Sciences, Institute of Sport Sciences, University of Lausanne, Lausanne, Switzerland
- 2Laboratorio Antidoping, Federazione Medico Sportiva Italiana, Rome, Italy
The Athlete Biological Passport (ABP) was introduced to complement the direct anti-doping approach by indirectly outlining the possible use of prohibited substances or methods in sports. The ABP proved its effectiveness, at least through a deterrent effect, even though the matrices used for longitudinal monitoring (urine and blood) are subject to many intrinsic (e.g., genetic) and extrinsic (e.g., environmental conditions) confounding factors. In that context, new and more specific biomarkers are currently under development to enhance both the sensitivity and the specificity of the ABP. Multiple strategies are presently being explored to improve this longitudinal monitoring, with the development of the current modules, the investigation of new strategies, or the screening of new types of doping. Nevertheless, due to the variability induced by indirect biomarkers, the consideration of confounding factors should continuously support this research. Beyond tremendous advances in analytical sensitivity, machine learning-based approaches seem inevitable to facilitate an expert interpretation of numerous biological profiles and promote anti-doping efforts. This perspective article highlights the current innovations of the Athlete Biological Passport that seem the most promising. Through different research axes, this short manuscript provides an opportunity to bring together approaches that are more widely exploited (e.g., omics strategies) and others in the early stages of investigation (e.g., artificial intelligence) seeking to develop the ABP.
Introduction
The Athlete Biological Passport (ABP) was first introduced in 2009 to thwart doping practices in sports by indirectly pointing out the possible use of prohibited substances or methods. Through longitudinal, individual, and adaptive monitoring, the ABP was also developed to target athletes requiring special attention (1) for the subsequent direct detection of prohibited substances in their urine or blood. While laboratory detection techniques have improved massively (2), the short detection window for some substances remains the major limitation to direct detection (3). The first ABP module implemented includes hematological markers sensitive to different blood doping protocols, such as different types of erythropoiesis-stimulating agents (ESA) or autologous blood transfusions (4). A second module was developed to deal with doping by the so-called “pseudo-endogenous steroids” (that are, endogenous steroids when administered exogenously), with a screening of urinary biomarkers (1). Based on priors using a Bayesian network (5), the threshold values for each athlete are individualized as measurement points are recorded (4). With more than 180 Anti-Doping Rule Violations (ADRV) since its implementation, the ABP proved its effectiveness, at least through a deterrent effect outlined by a putative reduced amplitude of doping practices (6).
The confounding factors impacting the current variables of the ABP remain one of the main limitations of the indirect approach. For the hematological module, the impact of several factors on plasma volume (e.g., physical exercise) and erythropoiesis (e.g., altitude training) have been identified (7). Further, the influence of exogenous (e.g., alcohol) and endogenous factors (e.g., menstrual cycle) were shown to alter variables of the steroid module (8). We have performed a comparative analysis of studies published in peer-reviewed journals, and reporting ABP profiles from the Anti-Doping Administration and Management System (ADAMS). Very interestingly, despite a higher occurrence of Atypical Passport Findings (ATPF) in protocols involving prohibited substances, atypical profiles were also found in connection with several physiological confounders (Figure 1). Combined with a decrease in sensitivity when micro-dosing treatment is implemented (9), the development of new specific biomarkers is required. In this context, this perspective article aims to highlight promising approaches for the future development of the ABP.
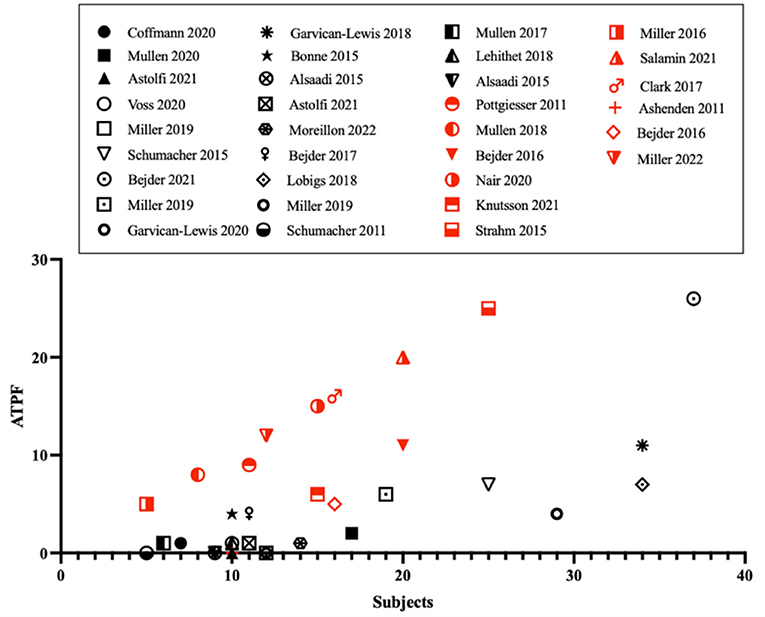
Figure 1. Occurrences of atypical passport findings (ATPF) as function of the number of subjects in the studies that used the official Anti-Doping Administration and Management System (ADAMS) training software. Publications involving doping protocols are presented with red symbols, publications investigating confounding factors in black figures.
Development of current modules
Variables analyzed by flow cytometry (with the Sysmex XN instruments generation since 2019) are now used for the measurement of the ABP hematological variables (10). Using a slightly modified technology for reticulocytes measurement compared to the previous XE and XT series (11), this latest generation of devices provides the quantification of many blood parameters currently not yet exploited by the ABP. For instance, reticulocyte hemoglobin equivalent (Ret-He) or immature reticulocyte fraction (IRF) could be potential candidates for the development of the ABP hematological module (12). These latter variables are indeed responsive to erythropoiesis alteration and independent of acute PV shift (13). Considered as a potential marker of iron deficiency (14), Ret-He could besides contribute to the existing work related to iron metabolism in the ABP framework (15–17). In addition, microvolumetric capillary whole blood collections have recently been suggested as an alternative to venous blood sampling (18). Demonstrating excellent agreement when generating individual ABP profiles, this collection method could become particularly useful in sample collection and need to be further explored.
Research seeking to identify new biomarkers to discriminate doping practices is the most investigated approach. However, the development of markers to discriminate the effects of confounding factors is a further alternative. In this way, the development of corrected individual limits by combining multiple markers sensitive to plasma volume (PV) variations has been suggested (19). This model was further validated in elite athletes, allowing them to discern hemoglobin concentration ([Hb]) changes caused by an alteration of erythropoiesis from those resulting from a transient hemodilution caused by physical exercise (20) or altitude exposure (21). The validity of these latter serum biomarkers in assessing PV variations was additionally shown in women monitored over 8 weeks (22). The model, therefore, seems particularly promising in encompassing all forms of natural variations in PV over time and could be considered useful in the toolbox for the interpretation of individual ABP profiles.
To complement the current steroid module, the development of a “blood steroid profile” is currently being developed (23, 24). Through ultra-high performance liquid chromatography-high-resolution mass spectrometry (UHPLC-HRMS) analysis, a method to quantify several endogenous steroids in serum has notably been implemented (23). Subsequently, a longitudinal evaluation of multiple serum biomarkers following the administration of transdermal testosterone (T) in women was performed, where the T/androstenedione ratio demonstrated the higher sensitivity during treatment (24). While a recent study reports an urgent need to develop biomarkers specific to women (25), serum monitoring has already demonstrated the potential of serum T measurement by liquid chromatography-mass spectrometry (LC-MS) to detect T doping in female athletes (26).
The isotope ratio mass spectrometry (IRMS) test is currently performed in case of suspicious variations of a steroid profile to confirm the non-endogenous origin of steroids (27). However, detecting abnormal isotopic values in steroid profile not identified as suspicious by the current criteria of the steroidal module of the ABP, longitudinal IRMS has shown a better sensitivity (28) and a lower individual variability (29, 30) in comparison to the traditional approach, based on the concentrations and concentration ratios of the steroid markers. Moreover, as the confirmation procedure is usually costly and time-consuming, a fast IRMS analysis has demonstrated adequate selectivity providing the examination of a larger number of samples (31). Longitudinal monitoring of 13C values of the target steroids and of the endogenous reference compounds would for instance reduce the number of false-negative results due to the intake of exogenous pseudo-endogenous steroids with an isotopic signature close to the endogenous ones. However, using IRMS in an indirect longitudinal screening procedure would represent a change of paradigm and current thresholds may result in a higher risk of false positive testing from a statistical standpoint. Nevertheless, although this paradigm change complicates its implementation, the application of an IRMS approach as an additional screening tool through the development of an “isotopic module” could be a particularly promising complement to the detection of steroidal doping (28).
Investigation of new anti-doping strategies
In the search for new biomarkers, omics strategies have also been considered (32). Designed to investigate biomarkers at a cellular level, this approach was suggested in an anti-doping approach. If transcriptomic and proteomic investigations have shown their interest (33), a metabolomic approach has recently demonstrated an interesting perspective (34). In this context, a recent study identified a panel of metabolites following autologous blood transfusion (35). Also applied for the screening of growth hormone (36) and testosterone misuse (37), individualized reference ranges seem to be the most promising approach (34). Nevertheless, the difficulty of setting reference values due to differences between sports disciplines (38) or various confounding factors such as intense cardiovascular effort (39) or nutritional supplements (40) constitute important challenges for field implementation. Therefore, despite some limitations, an explorative metabolomics approach looking for longitudinal profiling should be further investigated (41).
Through a microscopic approach, the morphology of red blood cells (RBC) could also be an innovative perspective in the future of blood doping detection, especially for blood transfusion. If the homologous blood transfusions (HBT) are detectable by flow cytofluorimetry-based method (42, 43), autologous blood transfusions are currently only trackable through indirect biomarkers (33). It is known that some properties of RBCs will be altered during storage (44), especially to membrane modification inducing a reduction of the deformability (45). Based on a recent study, it seems besides that deformability alteration is observed generally but also in the different subpopulations of RBC maturation (46). In this way, changes in the expression of CD 55 and CD 59 surface RBC proteins and variation in cell size have been observed (47), demonstrating the relevance of biomarkers related to blood aging and storage. More recently, a recent study investigated circulating RBC extracellular vesicles after transfusion, showing an increase of this biomarker in the hours following reinfusion, thus providing additional evidence in case of suspicious hematological profile (48). Therefore, a morphological approach to RBC membrane alterations could complement the ABP in the ABT identification and needs to be investigated.
Screening new forms of doping
Longitudinal biomarkers sensitive to other types of doping may also be another axis of research in the development of the ABP. Being under development for more than a decade by the World Anti-Doping Agency, an endocrine module will be introduced to tackle doping and particularly to address the use of growth hormones in an ABP approach (49). Several biomarkers have hence been investigated in the screening of different growth hormones (GH), in particular procollagen type III N-terminal peptide (P-III-NP), insulin growth factor-I (IGF-I), and the GH-2000 age discriminant score resulting from these two biomarkers (50–52). The stability of these markers confirms their relevance in a longitudinal approach (52), although a large intra-individual variability seems to be observed in women (53), complicating longitudinal follow-up. Showing very encouraging results when applied to the ABP model despite short detection windows (49), these outcomes strengthen the hypothesis for the future implementation of the endocrine module to complete the ABP screening spectrum. A similar approach seems to be the only option to detect also doping by other growth factors, that are presently not detectable by other analytical approaches.
Application of artificial intelligence
Key in today's data processing, the application of artificial intelligence (AI) in anti-doping has been investigated for several years and should be the subject of further research. By developing software gathering various types of information (e.g., physical performance or hematological data), an innovative strategy was initially developed to improve the target testing by emphasizing abnormal patterns (54). Since then, several approaches seeking to investigate multiple machine learning algorithms to identify general (55) or specific doping practices (56) have been investigated. These projects have confirmed the large potential of machine learning in anti-doping, leading the way for more elaborate design (56). Following a similar approach, performance monitoring has been suggested as an alternative to biological matrices (57). Using performance data such as competitive results (58, 59) or on critical power (60), an “athlete performance passport” has been suggested for building individualized career performance trajectories (61). Therefore, these models could contribute to trace abnormal performances and completing biological investigations in a field where AI will undoubtedly be part of future development.
Conclusion
The development of new biomarkers is required for the later development of the ABP. Several fields of investigation are currently being pursued, in which AI will certainly play an essential role. Nevertheless, to provide specific and robust analysis, these new markers need to be studied extensively to determine the natural variability, especially in a population of elite athletes with increased influencing factors. Overall, all these resources could grant a step forward in the target testing and support anti-doping authorities in obtaining the right sample, at the right time, from the right athlete.
Data availability statement
The original contributions presented in the study are included in the article/supplementary material, further inquiries can be directed to the corresponding author.
Author contributions
BK and RF conceptualized the article. BK drafted the manuscript. All authors contributed to revising critically the manuscript and approved the final submitted version.
Funding
Open access funding was provided by the University of Lausanne.
Conflict of interest
The authors declare that the research was conducted in the absence of any commercial or financial relationships that could be construed as a potential conflict of interest.
Publisher's note
All claims expressed in this article are solely those of the authors and do not necessarily represent those of their affiliated organizations, or those of the publisher, the editors and the reviewers. Any product that may be evaluated in this article, or claim that may be made by its manufacturer, is not guaranteed or endorsed by the publisher.
References
1. WADA. Athlete Biological Passport Operating Guidelines. Montreal, Canada: World Anti-Doping Agency (2021). Available online at: https://www.wada-ama.org/sites/default/files/resources/files/guidelines_abp_v8_final.pdf (accessed September 20, 2022).
2. Thevis M, Piper T, and Thomas A. Recent advances in identifying and utilizing metabolites of selected doping agents in human sports drug testing. J Pharm Biomed Anal. (2021) 205:114312. doi: 10.1016/j.jpba.2021.114312
3. Saugy M, and Leuenberger N. Antidoping: from health tests to the athlete biological passport. Drug Test Anal. (2020) 12:621–8. doi: 10.1002/dta.2773
4. Saugy M, Lundby C, and Robinson N. Monitoring of biological markers indicative of doping: the athlete biological passport. Br J Sports Med. (2014) 48:827–32. doi: 10.1136/bjsports-2014-093512
5. Sottas PE, Robinson N, Rabin O, and Saugy M. The athlete biological passport. Clin Chem. (2011) 57:969–76. doi: 10.1373/clinchem.2011.162271
6. Iljukov S, Kauppi JP, Uusitalo ALT, Peltonen JE, and Schumacher YO. Association between implementation of the athlete biological passport and female Elite Runners' performance. Int J Sports Physiol Perform. (2020) 15:1231–6. doi: 10.1123/ijspp.2019-0643
7. Krumm B, and Faiss R. Factors confounding the athlete biological passport: a systematic narrative review. Sports Med Open. (2021) 7:65. doi: 10.1186/s40798-021-00356-0
8. Kuuranne T, Saugy M, and Baume N. Confounding factors and genetic polymorphism in the evaluation of individual steroid profiling. Br J Sports Med. (2014) 48:848–55. doi: 10.1136/bjsports-2014-093510
9. Ashenden M, Gough CE, Garnham A, Gore CJ, and Sharpe K. Current markers of the Athlete Blood Passport do not flag microdose EPO doping. Eur J Appl Physiol. (2011) 111:2307–14. doi: 10.1007/s00421-011-1867-6
10. Equey T, Sletten C, Dehnes Y, D'Onofrio G, Brugnara C, Baume N, et al. Standardization of reticulocyte counts in the athlete biological passport: a practical update. Int J Lab Hematol. (2021) 44:112–7. doi: 10.1111/ijlh.13732
11. Naud JF, Giraud S, Robinson N, Desharnais P, Ericsson M, Saugy M, et al. Standardization of reticulocyte counts in the athlete biological passport. Int J Lab Hematol. (2019) 41:387–91. doi: 10.1111/ijlh.12988
12. Jeppesen JS, Breenfeldt Andersen A, Bonne TC, Thomassen M, Sørensen H, Nordsborg NB, et al. Immature reticulocytes are sensitive and specific to low-dose erythropoietin treatment at sea level and altitude. Drug Test Anal. (2021) 13:1331–40. doi: 10.1002/dta.3031
13. Banfi G, Tavana R, Freschi M, and Lundby C. Reticulocyte profile in top-level alpine skiers during four consecutive competitive seasons. Eur J Appl Physiol. (2010) 109:561–8. doi: 10.1007/s00421-010-1382-1
14. Toki Y, Ikuta K, Kawahara Y, Niizeki N, Kon M, Enomoto M, et al. Reticulocyte hemoglobin equivalent as a potential marker for diagnosis of iron deficiency. Int J Hematol. (2017) 106:116–25. doi: 10.1007/s12185-017-2212-6
15. Andersen AB, Bejder J, Bonne TC, Sørensen H, Sørensen H, Jung G, et al. Hepcidin and erythroferrone complement the athlete biological passport in the detection of autologous blood transfusion. Med Sci Sports Exerc. (2022) 54:1604–16. doi: 10.1249/MSS.0000000000002950
16. Leuenberger N, Bulla E, Salamin O, Nicoli R, Robinson N, Baume N, et al. Hepcidin as a potential biomarker for blood doping. Drug Test Anal. (2017) 9:1093–7. doi: 10.1002/dta.2122
17. Robach P, Gammella E, Recalcati S, Girelli D, Castagna A, Roustit M, et al. Induction of erythroferrone in healthy humans by micro-dose recombinant erythropoietin or high-altitude exposure. Haematologica. (2021) 106:384–90. doi: 10.3324/haematol.2019.233874
18. Goodrum JM, Lewis LA, Fedoruk MN, Eichner D, and Miller GD. Feasibility of microvolumetric capillary whole blood collections for usage in Athlete Biological Passport analysis. Drug Test Anal. (2022) 14:1291–9. doi: 10.1002/dta.3254
19. Lobigs LM, Sottas PE, Bourdon PC, Nikolovski Z, El-Gingo M, Varamenti E, et al. A step towards removing plasma volume variance from the Athlete's Biological Passport: the use of biomarkers to describe vascular volumes from a simple blood test. Drug Test Anal. (2018) 10:294–300. doi: 10.1002/dta.2219
20. Garvican-Lewis LA, Lobigs LM, Equey T, Goebel C, Agon V, McCowan A, et al. A multi-parametric approach to remove the influence of plasma volume on the athlete biological passport during a Union Cycliste Internationale cycling stage race. Drug Test Anal. (2020) 12:1252–63. doi: 10.1002/dta.2878
21. Lobigs LM, Garvican-Lewis LA, Vuong VL, Tee N, Gore CJ, Peeling P, et al. Validation of a blood marker for plasma volume in endurance athletes during a live-high train-low altitude training camp. Drug Test Anal. (2018) 10:1176–83. doi: 10.1002/dta.2370
22. Moreillon B, Equey T, Astolfi T, Salamin O, and Faiss R. Removal of the influence of plasma volume fluctuations for the Athlete Biological Passport and stability of haematological variables in active women taking oral contraception. Drug Test Anal. (2022) 14:1004–16. doi: 10.1002/dta.3218
23. Ponzetto F, Mehl F, Boccard J, Baume N, Rudaz S, Saugy M, et al. Longitudinal monitoring of endogenous steroids in human serum by UHPLC-MS/MS as a tool to detect testosterone abuse in sports. Anal Bioanal Chem. (2016) 408:705–19. doi: 10.1007/s00216-015-9185-1
24. Salamin O, Nicoli R, Langer T, Boccard J, Grundisch CS, Xu C, et al. Longitudinal evaluation of multiple biomarkers for the detection of testosterone gel administration in women with normal menstrual cycle. Drug Test Anal. (2021) 14:833–50. doi: 10.1002/dta.3040
25. Borjesson A, Lehtihet M, Andersson A, Dahl ML, Vicente V, Ericsson M, et al. Studies of athlete biological passport biomarkers and clinical parameters in male and female users of anabolic androgenic steroids and other doping agents. Drug Test Anal. (2020) 12:514–23. doi: 10.1002/dta.2763
26. Handelsman DJ, and Bermon S. Detection of testosterone doping in female athletes. Drug Test Anal. (2019) 11:1566–71. doi: 10.1002/dta.2689
27. Piper T, Geyer H, Haenelt N, Huelsemann F, Schaenzer W, Thevis M, et al. Current insights into the steroidal module of the athlete biological passport. Int J Sports Med. (2021) 42:863–78. doi: 10.1055/a-1481-8683
28. Jardines D, Botrè F, Colamonici C, Curcio D, Procida G, and de la Torre X. Longitudinal evaluation of the isotope ratio mass spectrometric data: towards the 'isotopic module' of the athlete biological passport? Drug Test Anal. (2016) 8:1212–21. doi: 10.1002/dta.2118
29. de la Torre X, Jardines D, Curcio D, Colamonici C, and Botre F. Isotope ratio mass spectrometry in antidoping analysis: The use of endogenous reference compounds. Rapid Commun Mass Spectrom. (2019) 33:579–86. doi: 10.1002/rcm.8377
30. de la Torre X, Jardines D, and Botrè F. Evaluation of longitudinal (13) C IRMS data in antidoping analysis. Drug Test Anal. (2022) 1–14. doi: 10.1002/dta.3339
31. de la Torre X, Colamonici C, Curcio D, and Botrè F. Fast IRMS screening of pseudoendogenous steroids in doping analyses. Drug Test Anal. (2017) 9:1804–12. doi: 10.1002/dta.2321
32. Reichel C. OMICS-strategies and methods in the fight against doping. Forensic Sci Int. (2011) 213:20–34. doi: 10.1016/j.forsciint.2011.07.031
33. Salamin O, De Angelis S, Tissot JD, Saugy M, and Leuenberger N. Autologous blood transfusion in sports: emerging biomarkers. Transfus Med Rev. (2016) 30:109–15. doi: 10.1016/j.tmrv.2016.05.007
34. Botrè F, Georgakopoulos C, and Elrayess MA. Metabolomics and doping analysis- promises and pitfalls. Bioanalysis. (2020) 12:719–22. doi: 10.4155/bio-2020-0137
35. Al-Nesf A, Mohamed-Ali N, Acquaah V, Al-Jaber M, Al-Nesf M, Yassin MA, et al. Untargeted metabolomics identifies a novel panel of markers for autologous blood transfusion. Metabolites. (2022) 12:425. doi: 10.3390/metabo12050425
36. Narduzzi L, Dervilly G, Marchand A, Audran M, Le Bizec B, Buisson C, et al. Applying metabolomics to detect growth hormone administration in athletes: proof of concept. Drug Test Anal. (2020) 12:887–99. doi: 10.1002/dta.2798
37. Raro M, Ibáñez M, Gil R, Fabregat A, Tudela E, Deventer K, et al. Untargeted metabolomics in doping control: detection of new markers of testosterone misuse by ultrahigh performance liquid chromatography coupled to high-resolution mass spectrometry. Anal Chem. (2015) 87:8373–80. doi: 10.1021/acs.analchem.5b02254
38. Al-Khelaifi F, Diboun I, Donati F, Botrè F, Alsayrafi M, Georgakopoulos C, et al. A pilot study comparing the metabolic profiles of elite-level athletes from different sporting disciplines. Sports Med Open. (2018) 4:2. doi: 10.1186/s40798-017-0114-z
39. Al-Khelaifi F, Donati F, Botrè F, Latiff A, Abraham D, Hingorani A, et al. Metabolic profiling of elite athletes with different cardiovascular demand. Scand J Med Sci Sports. (2019) 29:933–43. doi: 10.1111/sms.13425
40. Al-Khelaifi F, Diboun I, Donati F, Botrè F, Alsayrafi M, Georgakopoulos C, et al. Metabolomics profiling of xenobiotics in elite athletes: relevance to supplement consumption. J Int Soc Sports Nutr. (2018) 15:48. doi: 10.1186/s12970-018-0254-7
41. Narduzzi L, Dervilly G, Audran M, Le Bizec B, and Buisson C. A role for metabolomics in the antidoping toolbox? Drug Test Anal. (2020) 12:677–90. doi: 10.1002/dta.2788
42. Donati F, de la Torre X, Pagliarosi S, Pirri D, Prevete G, and Botrè F. Detection of homologous blood transfusion in sport doping by flow cytofluorimetry: state of the art and new approaches to reduce the risk of false-negative results. Front Sports Active Living. (2022) 4:808449. doi: 10.3389/fspor.2022.808449
43. Giraud S, Robinson N, Mangin P, and Saugy M. Scientific and forensic standards for homologous blood transfusion anti-doping analyses. Forensic Sci Int. (2008) 179:23–33. doi: 10.1016/j.forsciint.2008.04.007
44. Islamzada E, Matthews K, Lamoureux E, Duffy SP, Scott MD, Ma H, et al. Blood unit segments accurately represent the biophysical properties of red blood cells in blood bags but not hemolysis. Transfusion. (2021) 62:448–56. doi: 10.1111/trf.16757
45. Yoshida T, Prudent M, and D'Alessandro A. Red blood cell storage lesion: causes and potential clinical consequences. Blood Transfus. (2019) 17:27–52. doi: 10.2450/2019.0217-18
46. Grau M, Zollmann E, Bros J, Seeger B, Dietz T, Noriega Ureña JA, et al. Autologous blood doping induced changes in red blood cell rheologic parameters, RBC age distribution, and performance. Biology. (2022) 11:647. doi: 10.3390/biology11050647
47. Donati F, Acciarini R, De Benedittis I, de la Torre X, Pirri D, Prete M, et al. Detecting autologous blood transfusion in doping control: biomarkers of blood aging and storage measured by flow cytofluorimetry. Curr Pharm Biotechnol. (2018) 19:124–35. doi: 10.2174/1389201019666180405165118
48. Voss SC, Yassin M, Grivel JC, Al Hmissi S, Allahverdi N, Nashwan A, et al. Red blood cell derived extracellular vesicles during the process of autologous blood doping. Drug Test Anal. (2021) 1–11. doi: 10.1002/dta.3157
49. Equey T, Pastor A, de la Torre Fornell R, Thomas A, Giraud S, Thevis M, et al. Application of the athlete biological passport approach to the detection of growth hormone doping. J Clin Endocrinol Metab. (2022) 107:649–59. doi: 10.1210/clinem/dgab799
50. Lehtihet M, Bhuiyan H, Dalby A, Ericsson M, and Ekström L. Longitudinally monitoring of P-III-NP, IGF-I, and GH-2000 score increases the probability of detecting two weeks' administration of low-dose recombinant growth hormone compared to GH-2000 decision limit and GH isoform test and micro RNA markers. Drug Test Anal. (2019) 11:411–21. doi: 10.1002/dta.2506
51. Marchand A, Martin JA, Collot D, Hoang O, Roulland I, Semence F, et al. Combined administration of microdoses of growth hormone and erythropoietin: Effects on performance and evaluation of GH detection capability using anti-doping methods. Drug Test Anal. (2019) 11:1698–713. doi: 10.1002/dta.2674
52. Erotokritou-Mulligan I, Eryl Bassett E, Cowan DA, Bartlett C, Milward P, Sartorio A, et al. The use of growth hormone (GH)-dependent markers in the detection of GH abuse in sport: physiological intra-individual variation of IGF-I, type 3 pro-collagen (P-III-P) and the GH-2000 detection score. Clin Endocrinol. (2010) 72:520–6. doi: 10.1111/j.1365-2265.2009.03668.x
53. Ericsson M, Bhuiyan H, Yousif B, Lehtihet M, and Ekström L. The intra-individual stability of GH biomarkers IGF-I and P-III-NP in relation to GHRH administration, menstrual cycle, and hematological parameters. Drug Test Anal. (2020) 12:1620–8. doi: 10.1002/dta.2953
54. Manfredini AF, Malagoni AM, Litmanen H, Zhukovskaja L, Jeannier P, Dal Follo D, et al. Performance and blood monitoring in sports: the artificial intelligence evoking target testing in antidoping (AR.I.E.T.T.A.) project. J Sports Med Phys Fitness. (2011) 51:153–9.
55. Kelly T, Beharry A, and Fedoruk M. Applying machine learning techniques to advance anti-doping. Eur J Sports Exercise Sci. (2019) 7:1–9.
56. Rahman MR, Bejder J, Bonne TC, Breenfeldt Andersen A, Rodriguez Huertas J, Aikin R, et al. AI-based approach for improving the detection of blood doping in sports. arXiv e-prints. (2022).
57. Hopker J, Schumacher YO, Fedoruk M, Morkeberg J, Bermon S, Iljukov S, et al. Athlete performance monitoring in anti-doping. Front Physiol. (2018) 9:232. doi: 10.3389/fphys.2018.00232
58. Montagna S, and Hopker J. A Bayesian approach for the use of athlete performance data within anti-doping. Front Physiol. (2018) 9:884. doi: 10.3389/fphys.2018.00884
59. Hopker J, Griffin J, Brookhouse J, Peters J, Schumacher YO, Iljukov S, et al. Performance profiling as an intelligence-led approach to antidoping in sports. Drug Test Anal. (2020) 12:402–9. doi: 10.1002/dta.2748
60. Puchowicz MJ, Mizelman E, Yogev A, Koehle MS, Townsend NE, Clarke DC, et al. The critical power model as a potential tool for anti-doping. Front Physiol. (2018) 9:643. doi: 10.3389/fphys.2018.00643
Keywords: anti-doping, Athlete Biological Passport, blood, urine, serum, biomarkers
Citation: Krumm B, Botrè F, Saugy JJ and Faiss R (2022) Future opportunities for the Athlete Biological Passport. Front. Sports Act. Living 4:986875. doi: 10.3389/fspor.2022.986875
Received: 05 July 2022; Accepted: 18 October 2022;
Published: 02 November 2022.
Edited by:
Angela Jo-Anne Schneider, Western University, CanadaReviewed by:
Pascal Borry, KU Leuven, BelgiumThomas Piper, German Sport University Cologne, Germany
Adam Cawley, Racing NSW, Australia
Giuseppe D'Onofrio, Università Cattolica del Sacro Cuore, Italy
Copyright © 2022 Krumm, Botrè, Saugy and Faiss. This is an open-access article distributed under the terms of the Creative Commons Attribution License (CC BY). The use, distribution or reproduction in other forums is permitted, provided the original author(s) and the copyright owner(s) are credited and that the original publication in this journal is cited, in accordance with accepted academic practice. No use, distribution or reproduction is permitted which does not comply with these terms.
*Correspondence: Raphael Faiss, raphael.faiss@unil.ch
†ORCID: Bastien Krumm orcid.org/0000-0002-9132-606X
Raphael Faiss orcid.org/0000-0001-6029-9516