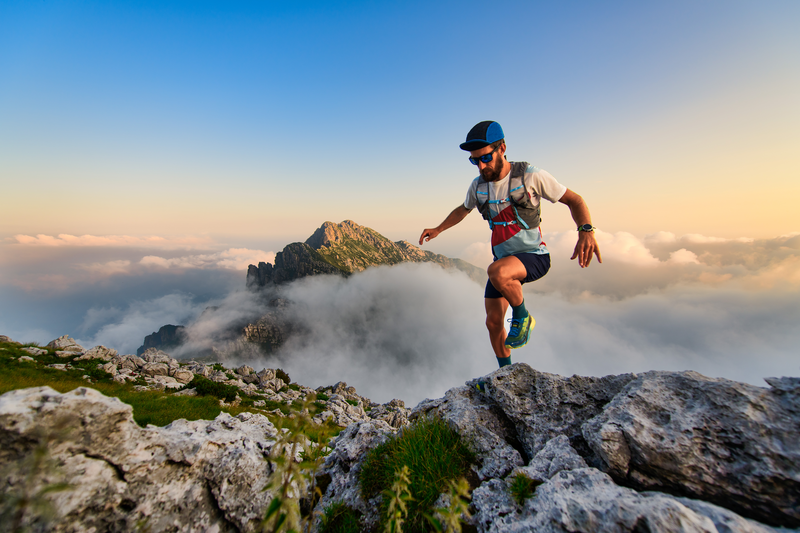
94% of researchers rate our articles as excellent or good
Learn more about the work of our research integrity team to safeguard the quality of each article we publish.
Find out more
SYSTEMATIC REVIEW article
Front. Sports Act. Living , 08 September 2022
Sec. Elite Sports and Performance Enhancement
Volume 4 - 2022 | https://doi.org/10.3389/fspor.2022.952779
Background: Agility is acknowledged as a crucial factor of performance in various open skill sports in both adult and youth athletes. However, despite its significance for sports performance the development and the trainability of agility are under-researched within the pediatric literature. A systematic scoping review was considered most appropriate to provide researchers and practitioners with an overview of the current body of literature approaching agility in youth.
Objectives: The objectives of this scoping review were to map the extent, range, and nature of existing evidence regarding trainability and “natural” development of agility and to summarize corresponding study results.
Methods: The scoping review protocol was pre-registered at Open Science Framework. Systematic searches were conducted using the databases PubMed, Scopus, ProQuest, Web of Science, SURF, and SPONET to identify sources covering agility in youth. Among other inclusion criteria, only references applying unplanned agility concepts were included.
Results: Ultimately, 41 reports were included comprising 23 observational studies, 14 experimental studies, and 4 references of secondary research. A total of 3,087 subjects were assessed in the included studies. Subject groups were predominantly male, above 10 years of age, and soccer athletes. Outcomes of observational studies indicate an effect of age and maturation on agility performance resulting in a non-linear “natural” development of agility. Furthermore, relationships between contributing perceptual-cognitive factors and agility performance tend to increase with progressing age, whereas relationships between physical factors and agility performance diminish. Evidence of training studies suggests that agility is trainable in youth, albeit with various underlying mechanisms.
Conclusions: This systematic scoping review is the first mapping of the body of literature about agility in youth. It outlines the current evidence base, reveals research gaps, and points out future directions to support researchers and practitioners in this field. Although, increasing research activity in this field is discernible, agility research in youth is still in its infancy. Considering the significance of agility for sports performance, future research is postulated to design evidence-based strategies for long-term agility development in young athletes.
The ability to execute changes in movement patterns and directions as rapidly as possible is a desired quality highly regarded by coaches and athletes across a wide array of sports, such as field, court, and combat sports (1–3). This quality is considered agility if the executed movement is an open skill task that is performed in response to a stimulus (e.g., opponent, teammate, or ball) (4). Equally, in young athletes, well-developed agility is acknowledged as a critical factor for success as it contributes to athletes' performance in various open skill sports (5). Accordingly, the development of agility competencies in youth is deemed necessary by strength and conditioning coaches (6). Moreover, agility attained recognition by its implementation in long-term athletic development models, which guide a systematic enhancement of skills and abilities throughout childhood and adolescence (7, 8).
Despite the generally accepted high significance of agility for sports performance, Lloyd and Oliver (7) stated that “agility is arguably one of the most under-researched fitness components within the pediatric literature […]”. Due to a dearth of research, unraveling the impacts of age and maturation on agility performance is difficult, and assertions about agility's optimal trainability are generally speculative (5). This situation is further exacerbated by two facts: First, unlike the paucity of agility research, a plethora of research is devoted to change-of-direction (COD) ability, which was formerly described as the integrated movement component of agility performance (9). In contrast to agility tasks, COD movements are without the requirement to react to a stimulus; thus, they are pre-planned. Furthermore, recent research indicates relatively low statistical commonalities of agility and COD performances, which are considered distinct qualities (10–17). Consequently, findings of COD research cannot automatically be extrapolated to agility (14). Second, most agility research was conducted in an adult population. The defining difference between youth and adult athletes is the consequences of growth and maturation, which are paramount in pediatric exercise science (18). Hence, equally like the limited transferability of COD research to agility, research findings of agility training ascertained in the adult population cannot implicitly be applied to young athletes (19).
Growth and maturation follow individual (in timing and tempo) and non-linear trajectories with alternating periods of stagnant changes and abundant fluctuations (20, 21). These volatile stages of growth and maturation are likely to affect the trainability of youth athletes (22), which refers to the responsiveness to a specific instructional, practice, and/or training stimulus (23). Thus, mechanisms and effects of specific and non-specific agility training probably vary with biological age. Recommendations regarding age-appropriate agility training are mainly speculative due to the sparse literature in this field (5). Nonetheless, the trainability of agility can at least be approximated by examining the trainability of the main factors that determine agility performance. Several deterministic models of agility performance have been proposed (4, 9, 13, 24–27), which have close resemblance regarding their organismic components (i.e., perceptual, cognitive, physical, and motor control factors). The contribution of these factors to agility performance is variable and presumably also growth and maturation dependent (13). Given the proven trainability of strength (28, 29) and speed (30), as well as motor (23) and anticipation skills (31) in youth and provided that these enhancements potentially transfer to agility, it might be assumed that agility is likewise trainable (5), even though the extent and underlying mechanisms differ at different developmental periods.
Cross-sectional data suggests that agility performance enhances “naturally” (i.e., apart from training) with increasing age, but with significant increases from childhood to early adolescence and a near-plateau in mid-adolescence (32). Therefore, training adaptations (in agility performance) are not solely attributable to the impacts of exposed training stimuli but also to the natural developmental processes of the young athlete (33). Thus, the origins of gains in agility performance are indistinct. It is difficult to differentiate between “natural” development and exercise-induced adaptations; this complicates the understanding of trainability in youth athletes (19, 34). In conclusion, it is imperative to consider growth and maturation aspects in youth athlete development (e.g., by regular monitoring of stature and body mass) (33), as they affect both the trainability and the “natural” development of agility throughout childhood and adolescence.
A decade has passed since the above-mentioned statement of Lloyd and Oliver (7), and it is about time to reevaluate the state of agility research in the pediatric exercise science literature. The conduct of a systematic scoping review was considered the most appropriate method to address the research question: What is known from the academic literature about the “natural” development and trainability of agility in youth? Agility has been subject to several systematic reviews approaching contributing factors (35, 36), effects of training interventions (36), and testing procedures (3, 36–38). However, the effects of growth and maturation were not explicitly considered in these reviews. Although trainability and “natural” development of agility in consideration of growth and maturation have been narratively reviewed (5, 30, 39), a systematic approach is yet to be carried out.
The scoping review had the following objectives: (1) to examine the extent, range, and nature of literature approaching agility in the youth population; (2) to reveal gaps in the literature to guide future research endeavors; and (3) to map and outline evidence regarding trainability, “natural” development, and contribution of underlying key factors of agility performance in consideration of maturation.
A systematic scoping review was conducted in accordance with the Preferred Reporting Items for Systematic Reviews and Meta-Analyses extension for scoping reviews (PRISMA-ScR) (40) guidelines to address the research questions. This study was not approved by an institutional review board as the authors collected and synthesized data from previous studies in which informed consent was already obtained. Thus, this study is exempt from ethical approval. The study protocol was drafted using an adapted registration form for the PROSPERO database and revised by the authors. The final protocol (41) was pre-registered with the Open Science Framework1 on Jul 21, 2021.
Six bibliographic databases (ProQuest, PubMed, Scopus, Web of Science, SPONET, SURF) were selected as appropriate sources for the systematic literature search, which was completely performed on Jul 22, 2021. The title, abstract, and keyword fields were searched with search terms that included synonyms for “agility” (agility OR unplanned move* OR unplanned task* OR unplanned sidestep* OR reactive move* OR reactive task* OR reactive cut*) AND “youth” (child* OR adolescen* OR youth* OR puber* OR kid* OR teen* OR girl* OR boy* OR young* OR junior*). In addition, the search string utilized in Germanophone databases (SPONET and SURF) was extended with respective German search terms (agilität AND (kind* OR jugend* OR nachwuchs* OR mädchen OR jung*)).
Eligible publications had to conform to several inclusion criteria: (1) Exclusively articles from peer-reviewed journals, (edited) books, conference works, and dissertation theses, and (2) written in English or German language were considered. It was expected that the restriction of the publication type would ensure a minimum quality of the included sources, (3) The applied concept of agility had to align with the definition of (4) (4): “a rapid whole-body movement with change of velocity or direction in response to a stimulus.” Thus, reports applying pre-planned agility concepts (i.e., COD speed [CODS]) were excluded. In addition, agility concepts involved ground-based movements; aquatic or on-ice agility was out of scope. (4) Outcome(s) had to be relevant to the review question (i.e., outcome(s) had to be related to “natural” development or trainability of agility in youth). For example, (42) evaluated a new agility test in young tennis players and assessed agility performance in youth athletes. However, this publication does not provide information about the development or trainability of agility and is consequently deemed ineligible. (5) Effects of maturation are prominent from birth until early adulthood. Therefore, findings in early childhood were out of the scope of the present review, and effects of maturation after adolescence were expected to be neglectable. Therefore, the population addressed in the included studies had to be between 6 and 19 years of age. (6) The presence of diseases, injuries, or certain disabilities might substantially impact reported results. Thus, only sources assessing healthy subjects were considered.
Duplicates were removed after the search process using EndNote 20 (Clarivate, Philadelphia, USA). Afterward, records and reports were screened by a two-step approach (title and abstract screening, full-text screening) by one investigator using DistillerSR (Evidence Partners, Ottawa, Canada). It was not necessary to review each publication by more than one expert due to the objectivity of the inclusion criteria. Uncertainties in the selection process were resolved through consultation and discussion with a second researcher. Title and abstract screening were merged in a single step because it was anticipated that in most cases, a clear identification of the applied agility concept will be impossible by screening the publication title alone. If title and abstract provided insufficient information for a decision, full text was retrieved and further reviewed for eligibility verification. Reference lists of included reports were manually searched for additional potentially eligible publications, which might have escaped the search strategy. Data sets of included publications for each step are accessible at Open Science Framework2 (43) for methodological transparency and reproducibility purposes.
Data were extracted by a single reviewer, discussed with a second researcher and categorized in observational, experimental, and secondary research. Publication characteristics (e.g., type, year, language), subject characteristics (e.g., age, maturation stage, sex, type of sport, performance level), study characteristics (e.g., sample size, measurements), and main outcome(s) were extracted and charted using Microsoft Excel (Microsoft Corp, Redmond, USA) spreadsheets. The stimuli used in the agility tests were categorized based on their specificity into generic (e.g., flashing lights, arrows, or movement instructions from a screen), video (i.e., footage of human movements), and human (i.e., live tester that initiates the movement to which the athlete must respond) stimuli. A critical appraisal or risk of bias assessment was not performed, following the recommendations of Peters et al. (44). The findings of included sources were summarized in a narrative synthesis.
Cohen's recommended criteria were used to characterize effect sizes (45). If effect sizes were not provided, Cohen's d was calculated post-hoc according to the recommendations of Cumming (46) for dependent samples. Missing confidence intervals of effect sizes were calculated in accordance with Hedgesand Olkin (47).
The search and selection process results are illustrated in detail in a flow diagram (Figure 1). A total of 7,353 records were identified from the bibliographic database search. Titles and abstracts of 3,249 publications were screened after eliminating duplicate records. The eligibility of 685 remaining reports was assessed, which resulted in the inclusion of 39 reports. The exclusion reasons were distributed as follows: ineligibility regarding agility concept (n = 496), population assessed (n = 46), publication language (n = 7), and publication type (n = 3), as well as missing content-related affiliation (n = 89) and insufficiency of provided information (n = 5). Searches of reference lists of the included publications revealed two additional eligible reports, whereby 41 reports were ultimately included in the current scoping review.
Figure 1. PRISMA 2020 flow diagram of the search and selection process (48).
The included publications (n = 41) were composed of several journal articles (n = 34) (32, 39, 49–80), conference abstracts (n = 3) (81–83), contributions in edited volumes (n = 2) (5, 30), one PhD thesis (n = 1) (84), and one monograph (n = 1) (85). Figure 2 illustrates the growth of publications per year, with publication years ranging from 2011 until 2021. The publications are categorized in reports of observational studies (n = 23) (51, 52, 59, 61, 64–69, 71–73, 75–78, 80–85), reports of experimental studies (n = 14) (49, 50, 53–58, 60, 62, 63, 70, 74, 79), and secondary research (n = 4) (5, 30, 39, 85). Relevant data extracted from respective underlying studies (n = 37) are presented for observational studies in Table 1 and for experimental studies in Table 2. Research was conducted in 17 different countries. The vast majority of studies (n = 30) (32, 51, 52, 55–57, 59–62, 64–73, 75–84) originated in Europe, with most contributions coming from Slovakia (n = 8) (32, 59, 64, 66, 67, 69, 71, 80). Other countries of origin were Australia (n = 3) (49, 54, 63), Taiwan (n = 1) (74), Tunisia (n = 2) (53, 58), and USA (n = 1) (50).
Soccer (50–53, 55–58, 60–62, 65, 66, 68, 70–73, 77, 79–84) was the most examined sport, with over two-thirds (n = 25) of the 37 included studies approaching it. Athletes of other sports, such as futsal (76), Australian rules football (54), rugby league football (49), rugby union (63), badminton (74), and table tennis (59), were assessed in single studies each. Three studies included athletes of different sports (64, 75, 79), one included inactive subjects (69), and two studies did not report subjects' sport participation (32, 67).
A total of 3,087 subjects were involved across the studies, with a sample size range of 20–553 subjects (Mdn = 72) for observational studies and 10–36 subjects (M = 25) for experimental studies. The majority (62.2%, n = 23) of studies included subjects of solely male participants (49, 52–58, 62–65, 68–70, 72, 73, 76–78, 80, 81, 83), whereas 5.4% (n = 2) included solely female subjects (50, 59), 13.5% (n = 5) included mixed groups of both sexes (32, 67, 74, 75, 79)), and in 18.9% (n = 7) subjects' sex was not reported at all (51, 60, 61, 66, 71, 82, 84). Only one study (32) comprised subject groups below 10 years of age. Maturation was estimated in eight cases (22%) (52, 53, 60, 71–73, 77, 84), applying methods of Tanner (86) (n = 1) and non-invasive estimates of Mirwald et al. (87) (n = 4) and Moore et al. (88) (n = 3).
A total of 39 agility tests were employed, with presentations of generic (n = 27) (32, 51, 52, 56, 57, 59, 61, 62, 64–77, 80–84), video (n = 4) (49, 50, 54, 83) and human stimuli (n = 8) (53, 55, 58, 60, 63, 78, 79, 81). All agility tests that used sport-specific stimuli were designed to simulate defensive scenarios. A few studies (53, 58, 83) also included attacking scenarios due to subjects' ball possession, including trials with ball dribbles. However, these studies did not clearly state if the ball carrier's movement direction was opposed to the direction of the stimuli. Response times (timeframe from the onset of the stimulus until the initiation of the response movement) were separately assessed in 17.9% (n = 7) of cases (49, 54, 61, 63, 70, 74, 78). The Y-shaped agility test and its modified variants were the most frequently applied tests (n = 18) (49, 52–55, 58, 60–62, 67, 71, 73, 76–79, 81, 84). Agility tests differed regarding cutting angles, distances covered, number of consecutive CODs, number of COD options, starting velocities, dribbles, and other characteristics.
In most studies, the youngest group (range: 7-16 years) of athletes was outperformed in agility by the group with the oldest age (range: 14–18 years) (51, 52, 61, 65, 68, 69, 71–73, 78, 82–84). A few other studies did not find differences in agility performance among age groups (59, 76, 81). Differences were rather evident between younger age groups than between groups of older athletes (61, 69, 72, 82–84).
Maturation was identified as a factor affecting agility performance across ages as observed age group differences were no longer evident when maturation was included as a covariate (52, 72). In addition, significant correlations of trivial to large magnitudes between agility and measures of CODS, sprint, and jump performance were reported (51, 52, 61, 66, 68, 72, 73, 77, 80, 84). Studies calculating regression analyses revealed several predictors of agility performance, such as maturation (adjusted R2 = 8%) (52), sex (β = −0.46), age (β = −0.30), training experience (β = −0.18) (75), and in-line lunge performance (adjusted R2 = 38%) (52).
The 14 experimental studies comprised 21 intervention groups that were exposed to 15 different training programs. Table 3 gives an overview of the various training programs and their elicited effects in the respective intervention groups. Training programs can be categorized regarding their specificity to agility: Training involving neither unplanned movements nor any perceptual-cognitive elements is considered training programs of low specificity (e.g., COD drills, resistance training) (50, 53, 54, 57, 58, 62). Training programs exhibit a medium specificity if either unplanned movements or perceptual-cognitive elements are involved (e.g., video-based perception training) (55–57, 63, 74, 79). In contrast, a high specificity is achieved if both are included (e.g., agility drills, small-sided games) (49, 53, 54, 58, 60, 70). Most intervention groups experienced significant improvements in agility performance to different extents (49, 50, 53–58, 60, 62, 63, 70). Nevertheless, two groups of the moderate specificity category (74, 79) and three groups of low specific training programs (54, 57, 62) failed to improve agility performance.
This systematic scoping review aimed to (1) to map the literature approaching agility in the youth population, (2) to identify research gaps, and (3) to outline the existing literature regarding the trainability, “natural” development, and contribution of underlying key factors of agility performance in consideration of maturation. A total of 41 reports that explored agility in youth were systematically identified. Outcomes are discussed in detail, and recommendations for practice and research are made in the following subsections.
The rising number of publications per year indicates an increasing interest in the present topic. However, the completed data acquisition might explain the low number of publications in 2021 by midyear and by the COVID-19 pandemic, which potentially reduced research activity. Surprisingly, despite the early contemplation of agility as a movement in response to perceived stimuli in 1976 by (89), the first article examining this agility concept in youth was published in 2011 (49), indicating a fairly young body of literature. Although 41 reports approaching agility in youth were identified, the extent of evidence is still sparse, especially if specific research questions are sought to be answered.
Biases in the literature were detected regarding countries of origin (i.e., mainly European countries) and subjects' age, sex, and practiced sport (i.e., above 10 years of age, mostly males, and soccer, respectively); this limited the range of evidence. In addition, the lack of research on female subjects is problematic since results obtained in boys might not unconditionally be transferred to girls due to sex and maturation interaction in components of motor performance (90). Furthermore, most studies assessed agility in over 10-year-olds, which leaves questions concerning agility training in childhood and its meaningfulness regarding long-term athletic development unanswered (7, 18). Consequently, more extensive and diverse research is warranted to address these deficiencies to expand the range and extent of evidence.
The nature of the evidence is characterized by observational (cross-sectional) and experimental studies and secondary research. Included secondary research of (5), (39), and (30) examined the “natural” development and trainability of agility in young athletes. However, due to the lack of literature, assumptions of “natural” development of agility and given training recommendations were predominantly based on inferences deduced from CODS research and research of the particular determining factors of agility performance. Secondary research of (85) dedicated a section of their monograph to the “natural” development of agility. However, its content is almost entirely based on an also included study of the same authors (32).
Meta-analyses of training effects seem relatively meaningless considering the wide range of methods of included studies. Especially, the applied agility tests differ regarding stimulus presentation, cutting angle, distance covered, direction change from a stopping position or out of the movement, and the number of options and direction changes. This complicates direct comparisons of study results applying different tests since agility is considered a context-specific entity, thus dependent on task constraints (27).
Although a quality assessment is not provided in this scoping review, existing methodological and research design limitations of included literature should be acknowledged, such as the relatively small sample sizes in the experimental studies with a mean sample size of n = 13 subjects in the intervention groups, the absence of control groups in 5 of 14 studies, and the scarcely assessed response time limiting interpretation of training effects. Moreover, maturation was rarely estimated, making it difficult to draw theoretical conclusions about maturation effects.
The included studies assessed agility in defensive scenarios. However, evidence suggests that attacking and defending agility are distinct skills (91, 92). In invasion sports, agility movements in attackers vs. defenders clearly differ in regard to their goals (e.g., chasing or evading), strategies (e.g., execution of deceptive actions), imposed physical demands, performed COD techniques, and perceptual information used (15, 91–94). Thus, it should be noted that the presented results might not apply to offensive agility.
Current agility models identified various performance-determining factors such as perceptual and decision-making factors, technical qualities, and physical features (4, 9, 13, 24–27). However, the contribution of these factors depends on the imposed agility task and on the environment in which it is performed (95). Moreover, studies suggest that the relationships between agility performance and physical qualities are dependent on athletes' age.
Moderate to high relationships between jump and agility performance and high relationships between sprint and agility performance was observed in U11–U14 age groups (51, 84). High correlations between CODS and agility performance were found in U11–U13 age groups (66, 72, 84). The rather high correlations observed might result from the applied generic stimuli in the agility tests. Generic stimuli prohibit the usage of some perceptual and cognitive factors (e.g., pattern recognition, anticipation). Thus, a greater demand is placed on physical abilities to execute the agility tasks (84). The influence of physical capacities is even more pronounced in CODS, in which perceptual and cognitive demands are mostly omitted. Therefore, physical qualities are generally more associated with CODS than agility performance (10, 11, 77, 96–99).
However, as correlation coefficients tend to decline or parameters are not significantly correlated at all, several authors reported decreasing associations between physical capacities (i.e., jump, sprint, and CODS performance) and agility performance with escalating age groups (51, 61, 66, 72, 83, 84). This is not surprising since, in adulthood, physical qualities and agility are less related (97–101), and CODS and agility are even considered distinct skills (10–17) and thus, approaching a fully mature state ceases their relationship. The notion that factors other than physical capacities (i.e., technical, perceptual, and cognitive factors) are increasingly related to agility performance in older age groups is supported by findings of a stagnant sprint, jump, and CODS performance concomitant with enhanced agility performance in U17–U19 athletes (68, 78). Movement technique, perceptual, and decision-making factors have a higher relevance for agility performance in adults (100). Conversely, in young athletes, physical qualities seem to be more determinant. Due to their age, younger athletes probably exhibit less game experience and shorter training history, resulting in lower perceptual and decision-making skills, inexperienced technique, and lack of movement strategies than older athletes (51, 61, 102–104). This is also reflected by higher response times in younger athletes (61).
Nonetheless, (73) stated that the relationships between agility and sprint, jump, and CODS performance were more pronounced in U15 than in U13 athletes, conflicting with the above-mentioned findings. The authors supposed that the older group possesses a higher level of technical skill due to longer involvement in training, which enables them to exploit their sprinting and jumping qualities to a greater extent and convert them into effective agility maneuvers.
Further impacting factors apart from the discussed organismic factors were observed. For example, age and gender, training frequency, training experience, body mass in girls, and peripheral perception in boys were identified as contributing or impairing factors (75). In addition, several measures of movement proficiency of the functional movement screen (in-line lunge, deep overhead squat, active straight leg raise, and rotary stability) were found to be related to agility performance (52).
In most studies, higher agility performances in ascending age groups were observable, indicating a “natural” development of agility (51, 52, 61, 65, 68, 69, 71–73, 78, 82–84). However, significant differences were found between groups with higher age differences than between adjacent age groups. The enhancement of agility performance through the years is confirmed by regression analyses of (75), who observed a significant influence of chronological age on agility test performance.
The included observational studies are exclusively conceptualized in cross-sectional designs, accompanied by several limitations. First, cross-sectional designs do not capture individual developmental trajectories as subjects are generally assessed only once. Furthermore, age groups are arranged regarding the chronological age of the subjects. This might disguise differences between age brackets due to a potentially high intersubject variability within the age groups caused by different biological ages (i.e., maturation stage) of the peers, especially in groups with a broad age range (105). Hence, the less pronounced performance differences between adjacent age groups might be explained because maturation was rarely controlled. Therefore, athletes of different biological ages were pooled in the same chronological age group.
(52) and (72) showed that age group differences disappeared when maturation was entered as a covariate, emphasizing that maturation is one of the main contributing factors for differences between age groups. Although, contrary results were observed by (71). In this study, age group differences remained evident after adjusting to maturation, suggesting that not solely maturation but also different training regimes across age groups are probably responsible for differences in agility performance. In addition, data from a recent study described a merely moderate relationship (r = −0.36) between maturation and agility performance (77).
Longitudinal data of male soccer players indicates that CODS naturally improves with increasing age with a non-linear trajectory culminating between 13 and 14 years of age and a leveling off at the age of 17 (106). Similar results were found for agility performance in a cross-sectional study of (32) conducted on girls and boys between 7 and 18 years, albeit with a divergent slope. The increments in agility performance were rather high across childhood, but the increases diminish with ascending age groups and almost plateau in the group of 14-years-olds. The absence of a pronounced peak and the early flattening of the curve compared to the trajectory of CODS development might be explained by the mixed sample of girls and boys, who mature with different timings and thus, smoothen the curve due to higher inter-subject variability within the age groups. Unfortunately, this study is the only source providing insights into agility performance in children below 10 years of age. Longitudinal data on CODS in children suggests that changes in CODS performance depend on the mid-childhood growth spurt, which varies interindividually in terms of timing and tempo (21), thus, indicating a non-linear development of CODS (107). If this also applies to agility development in childhood is yet to be explored.
The above-mentioned stagnant agility performance starting at the age of 14 aligns with (82), who did not observe differences in agility performance in U15, U16, and U17 soccer players. Comparable results were obtained in a study of male soccer players who demonstrated differences in agility performance between age groups ranging between U12 and U16. In contrast, no changes were evident between U16 and U18 players (72). Stagnant periods were also observed in other studies (52, 59, 61, 69, 78, 81, 83, 84), albeit at different ages at entry. As opposed to this, (71) and (65) could not corroborate these findings as all age groups (U12-U16 and U10-16, respectively) differed significantly regarding agility performance in their observations. An explanation of stagnant periods in performance, although speculative, provides the concept of “adolescent awkwardness”, which refers to a temporary decline in performance or a disruption of motor coordination in adolescents as a consequence of rapid growth (20, 105, 108). This phenomenon typically emerges ~6 months before the zenith of the adolescent growth spurt (also known as peak height velocity [PHV]), which occurs at about 12 years in girls and 14 years in boys (109, 110). However, not all children experience delays or regression in performance (105).
Conflicting results were reported concerning the development of agility in late adolescence. Studies in male soccer players did not observe differences in agility performance between U19 and U17 (81) and U15 (83) age groups. In addition, adult (mean age: 28.1 ± 5.2 years) futsal players did not show higher agility performance than U19 players (76). Whereas, in studies of (68) and (78), U19 players outperformed U17 male soccer players in agility performance. Since no differences in sprinting speed, reactive strength, or jumping performance were observed between these age groups, the authors concluded that differences in agility performance were rather attributable to superior perceptual, anticipatory (78), and technical skills (68) than to enhanced physical capacities. Thus, differences were presumably predominantly induced by training and gained expertise and less by consequences of maturation (72). It might be assumed that growth- and maturation-induced differences in agility performance between age groups recede in late adolescents until they completely cease when achieving a fully mature adult state (76).
Due to the multidimensional nature of agility, there is a broad spectrum of training methods to enhance this skill (111). Although movement specificity and the representative design of practice are related to transfer, training can be remote to the target movement and sporting context and still be capable of improving agility performance (112). Thus, it is not a strict necessity to train agility as a whole, and improvements can also be attained by training individual components of agility (113).
The 14 included experimental studies evaluated the effects of various training interventions on agility performance. Significant improvements in agility performance were observed in 16 of 21 training approaches. The chosen training approaches differed regarding their specificity to agility movements and were classified into training programs of high, moderate, and low specificity (Table 3). Training programs of high specificity aim to train agility skills as a whole. In contrast, training interventions in the category of moderate specificity are characterized by the involvement of either unplanned movements or specific cognitive components. Training programs of low specificity neither involve unplanned movements nor perceptual or decision-making elements.
Agility movements are performed in response to a stimulus, whereby stimulus perception and execution of the response movement are interdependent and constitute a coherent entity (i.e., perception-action coupling). It was argued that a decoupling of perception and action might impair the transfer of training/learning to the whole agility skill (14, 114). Therefore, to maintain the integrity of the agility skill, representative tasks used in perceptual-cognitive skills training should replicate real-life performance as closely as possible (114). Likewise, sport-specific decision-making elements should be included in physical training if the movement component of agility is sought to improve (14).
In a video-based training by (49), subjects had to change movement direction in response to video footage of an attacking opponent, whereby perception-action coupling was maintained. Agility performance significantly improved, which was attributed to enhanced perception since response time improved while performance in the pre-planned test condition remained the same. Supportive findings were obtained in a study of junior soccer players in which video-based tactical training combined with sport-specific motor responses led to improved response times, response accuracy, and ball kicking movement times in a tactical decision-making test (115).
In three interventions, exercises with persistent perception-action coupling (e.g., catching/evading duels, mirror drills, ball pass in a random direction) were utilized (58, 60, 70). An intervention group practicing this training form increased linear sprinting, CODS, and agility performance in the study of (58), whereby gains in agility performance were significantly higher in comparison to gains of a CODS training group. However, results should be interpreted carefully since improvements in performance parameters were also evident in the control group. (60) and (70) agreed with findings of enhanced agility performance showing large effect sizes. In the latter study, applying a generic stimulus in the agility test possibly concealed potential improvements in anticipation performance since no changes in response time were observed.
Another way of retaining perception-action coupling and a high representativity of the training task is exercising in small-sided games in which task constraints such as field dimensions, number of players, and rules are modified (116). (53) showed an enhanced agility performance accompanied by increases in sprint, jump, and CODS performance after a training intervention of small-sided games. Gains in agility performance were superior in the intervention group practicing small-sided games compared to the COD-exercising group. Nevertheless, performance gains were partly attributed to concomitantly conducted soccer training since increases were also observed in the control group. In the study of (54), small-sided game training in U18 Australian Rules footballers significantly improved agility performance, eliciting a large effect. Improvements were exclusively due to enhanced perception and decision-making since response time was reduced, whereas movement response time remained unchanged.
Subjects in the training interventions of (56), (57), and (74) had to perform unplanned multi-directional movements in response to random generic visual cues indicating movement direction. Improvements in agility performance were solely evident in the studies of (56) and (57), displaying large effects. In the latter study, measures of physical qualities (i.e., vertical jump and sprint) did not improve, suggesting that gains in agility performance are possibly due to specific technical enhancements. Randomly lit-up LED targets had to be hit in the BATAK Pro™ training in the study of (79). An improvement in agility performance was not evident, probably due to a lack of specificity of the training movements and the generic light stimulus with the demands of the utilized agility test.
Research suggests that perception and decision-making skills are trainable in youth (31). Several studies have proven that video-based training can enhance these skills in children and adolescents (115, 117–119). For example, (119) observed improved decision accuracy and faster decision times after video-based perceptual training in young softball players, which also transferred to the field environment. These findings align with the included study of (55), in which faster and more accurate decisions induced by video-based training positively transferred to agility performance. Furthermore, (63) employed video-based warm-up interventions with either an explicit (highlighted key kinematic cues) or an implicit (no additional information given) learning strategy which both resulted in faster response times in an agility test. Likewise, prepubescent tennis players could improve their anticipation skills irrespective of the applied learning strategy (118).
The category of low specificity comprises CODS, reactive strength, and resistance training approaches that predominantly target enhancing physical capacities to improve agility performance eventually. Physical qualities and agility are less related in adults (97–101), whereby the potential of transfer of corresponding training programs to agility performance might be reduced. Associations between physical factors and agility performance are generally stronger in youth athletes, especially in younger juveniles. Thus, the positive transfer of training approaches targeting physical capacities is probably seen in younger athletes. A reasonable amount of evidence suggests that resistance training can considerably increase strength in children and adolescents (28, 29, 120). Furthermore, a recent umbrella review proved that resistance training-induced gains in strength have the potential to positively transfer to CODS performance beyond a level achievable from growth and maturation alone (121). In line with this, (50) showed large effects of regular and unstable resistance training on agility performance in female soccer players. In addition, (62) observed large effects of eccentric training with a flywheel device on agility performance. Isoinertial eccentric training has previously shown the potential to enhance CODS in young soccer athletes, according to recent findings (122, 123). Research suggests that reactive strength training can improve COD ability in youth (124), albeit with more significant gains in older youths (125). This was not observed for agility performance in the study of (62) since a reactive strength training intervention did not elicit significant changes in agility performance.
In contrast to the mentioned strength training forms, the directional change is actually performed in COD drills. Even though the movement is executed pre-planned, it resembles the COD movement performed in unplanned agility tasks and thus, is potentially more specific than resistance training exercises. Nevertheless, the included training interventions employing COD drills and repeated shuttle sprints show ambiguous results. Improved CODS performance after COD training interventions were evident in three of four studies (53, 57, 58). However, only in studies by (53) and (58) did gains in CODS transfer positively to agility performance. It must be noted that in both studies, the control group also experienced significant improvements. In the study of (54), the training group performing COD drills did not improve in CODS or agility performance.
In conclusion, the results of positive transfer of different training forms to gains in agility performance lead to the assumption that agility is indeed trainable in youth, even though the underlying mechanisms are disparate. Nevertheless, the heterogeneity of applied training procedures, performance tests, training goals, and subjects' ages, performance levels, and exploitation of performance limits reduce the comparability of study outcomes and complicate deeper analyses of how maturation affects trainability of agility and ultimately, how agility should be trained at a particular age.
This scoping review has limitations that need to be addressed. First, only reports written in English or German were considered due to feasibility reasons, which potentially led to disregarded knowledge published in other languages. Moreover, following the recommendations of (44), included studies were not critically appraised. Notwithstanding, researchers are advised to appraise the quality of these studies if a more specific research question is about to be answered.
Considering the importance of agility for sports performance, the unabated desideratum of agility research in the youth population, and the advancing research activity in this field, agility research in youth has undoubtedly the potential to become a Cinderella story in pediatric exercise science, rising from neglect to being a hot topic. Nevertheless, the extent and range of the current body of literature are still limited, indicating an abundance of research gaps that are yet to be closed. Further agility research could provide a deeper understanding of the trainability of agility by unraveling the involvement of underlying key factors with due regard to biological age. This could help practitioners design evidence-based strategies for agility development throughout childhood and adolescence, which constitute an integral component of an all-embracing long-term athletic development (7, 39).
In this light, several recommendations can be made to guide future research endeavors:
More diverse research is postulated to expand the range of evidence, i.e., research in middle childhood, female subjects, and sports other than soccer.
Research designs with cross-sectional chronological age group comparisons have inherent deficiencies (105). Longitudinal study designs can offset these limitations and are endorsed when examining developmental effects.
Considering the influence of maturation on agility performance, it is advised to employ appropriate methods to estimate and control the maturation stage in youths.
Generic stimuli in agility tests disregard perceptual-cognitive factors of agility performance. This should be considered especially when testing older youths since perceptual-cognitive factors are increasingly determinant to agility performance with progressing age. Aside from the fact that representative design of agility practice and testing are endorsed in general (14, 36, 38, 126, 127), sport-specific stimuli should be employed in particular if agility tests are utilized to assess the effects of training approaches targeting the improvement of perceptual-cognitive factors.
Adults show distinctive perceptual-cognitive and biomechanical differences between attacking and defending agility (91–93). These differences are likely to be seen in young athletes as well, which needs further investigation. Thus, training and testing of athletes should be specifically designed for both conditions (15, 92–94, 128).
Outcome measures of agility performance in the included studies were exclusively time-based. Since not all in-game agility events happen at maximal speed (15, 113), a qualitative, more direct, and possibly more task-representative approach is to assess the immediate outcome of an agility event (e.g., successful or failed evasion or tackle) (92). In addition, athletes could potentially better exploit their repertoire of agility actions in outcome-based tests (e.g., successive application of different agility techniques or feints). Further research is required to assess the feasibility of such outcome-based agility testing in young athletes. Thus, practitioners and researchers should consider carefully whether an outcome-related or time-based measure best represents agility performance success (129).
Comprehensive assessments of determining factors are necessary to draw inferences about the origins of changes in agility performance (38). Those assessments are especially relevant for practitioners to expose athletes' strengths and deficits for adequate training prescription (96). Although physical performance parameters were frequently measured in conjunction with agility assessments, perceptual-cognitive factors (e.g., estimated by response time) were less often assessed. Surprisingly, technical qualities were not assessed, probably due to the absence of validated screening tools to assess COD technique regarding performance (26). Nonetheless, technical qualities in terms of appropriate sequencing of muscle actions, adoption of an appropriate body position, and coordination of force and impulse are requirements for fast agility movements (15, 27). Therefore, qualitative analyses of the COD technique can provide highly valuable information for the practitioner (129). Furthermore, since motor coordination explains a significant amount of variance in agility performance in adolescents (130), it might be assumed that technical qualities are similarly important for agility performance.
A decade ago, agility was described as one of the most under-researched fitness components within the pediatric literature (7). Today, agility research in youth might still be in its infancy, but the progressively accumulating evidence base over the years evinces an increasing research activity and interest in this topic.
This systematic scoping review is the first mapping of the body of literature about agility in youth to the best of our knowledge. Its research questions aimed to examine and synthesize the current state of knowledge about trainability and “natural” development of agility. Present key findings may have implications for researchers and practitioners and can be outlined as follows: Evidence suggests that agility performance is influenced by chronological and biological age and thus, “naturally” improves throughout childhood and adolescence in a non-linear manner. Furthermore, various training approaches with different underlying mechanisms could enhance agility performance confirming the trainability of agility in youths in general, even though influences of maturation remain cloudy. Moreover, results indicate that with progressing age, perceptual-cognitive factors are increasingly associated with agility performance, whereas relations with physical factors diminish. The relationship between technical factors and agility performance remains unknown and needs further investigation.
In the light of the significance of agility for sports performance, future research in this field is postulated to provide practitioners with evidence-based recommendations for maturation-dependent training prescription. This will help pave the way for an overarching long-term athletic development strategy, with agility as one of its integral components.
The datasets presented in this study can be found in online repositories. The names of the repository/repositories and accession number(s) can be found below: https://osf.io/8y9nk.
LT and DB contributed to the study conception, study design, the pre-registration of the study protocol, data analysis, and interpretation. LT contributed to literature search, screening and selection as well as performed the data charting, and wrote the first draft of the manuscript. All authors iteratively revised the manuscript until mutual approval of the final version.
The authors gratefully acknowledge the support of the Deutsche Forschungsgemeinschaft (DFG) and the Open Access Publication Fund of the Library and Information System (BIS; Carl von Ossietzky University Oldenburg, Germany) for covering article processing charges.
The authors declare that the research was conducted in the absence of any commercial or financial relationships that could be construed as a potential conflict of interest.
All claims expressed in this article are solely those of the authors and do not necessarily represent those of their affiliated organizations, or those of the publisher, the editors and the reviewers. Any product that may be evaluated in this article, or claim that may be made by its manufacturer, is not guaranteed or endorsed by the publisher.
1. Bourgeois FA, McGuigan MR, Gill ND, and Gamble P. Physical characteristics and performance in change of direction tasks: a brief review and training considerations. J Aust Strength Cond. (2017) 25:104–17.
2. HorníkováH, and Zemková E. Relationship between physical factors and change of direction speed in team sports. Appl Sci. (2021) 11:655. doi: 10.3390/app11020655
3. Jansen MG, Huijgen BC, Faber IR, and Elferink-Gemser MT. Measuring agility in tennis, badminton, and squash: a systematic review. Strength Cond J. (2021) 43:53–67. doi: 10.1519/SSC.0000000000000640
4. Sheppard JM, and Young WB. Agility literature review: classifications, training and testing. J Sports Sci. (2006) 24:919–32. doi: 10.1080/02640410500457109
5. Jeffreys I. Agility training for young athletes. In: Lloyd RS, Oliver LJ, editors. Strength Conditioning for Young Athletes: Science Application. New York, NY; London: Routledge (2020). p. 228–47.
6. Burton AM, Eisenmann JC, Cowburn I, Lloyd RS, and Till K. Developing motor competency in youths: perceptions and practices of strength and conditioning coaches. J Sports Sci. (2021) 39:2649–57. doi: 10.1080/02640414.2021.1949189
7. Lloyd RS, and Oliver JL. The youth physical development model. Strength Cond J. (2012) 34:61–72. doi: 10.1519/SSC.0b013e31825760ea
8. McBurnie AJ, Parr J, Kelly DM, and Dos'Santos T. Multidirectional speed in youth soccer players. Strength Cond J. (2021) 44:10–32. doi: 10.1519/SSC.0000000000000657
9. Young WB, James R, and Montgomery I. Is muscle power related to running speed with changes of direction? J Sports Med Phys Fit. (2002) 42:282–8.
10. Sheppard JM, Young WB, Doyle TL, Sheppard TA, and Newton RU. An evaluation of a new test of reactive agility and its relationship to sprint speed and change of direction speed. J Sci Med Sport. (2006) 9:342–9. doi: 10.1016/j.jsams.2006.05.019
11. Lockie RG, Jeffriess MD, McGann TS, Callaghan SJ, and Schultz AB. Planned and reactive agility performance in semiprofessional and amateur basketball players. Int J Sports Physiol Perform. (2014) 9:766–71. doi: 10.1123/ijspp.2013-0324
12. Spasic M, Krolo A, Zenic N, Delextrat A, and Sekulic D. Reactive agility performance in handball; development and evaluation of a sport-specific measurement protocol. J Sports Sci Med. (2015) 14:501–6.
13. Young WB, Dawson B, and Henry GJ. Agility and change-of-direction speed are independent skills: implications for training for agility in invasion sports. Int J Sports Sci Coach. (2015). 10:159–69. doi: 10.1260/1747-9541.10.1.159
14. Young WB, Rayner R, and Talpey S. It's time to change direction on agility research: a call to action. Sports Med. Open. (2021) 7:12. doi: 10.1186/s40798-021-00304-y
15. Young W. Dos'Santos T, Harper D, Jefferys I, Talpey S. Agility in invasion sports: position stand of the IUSCA. Int J Strength Cond. (2022) 2:1–25. doi: 10.47206/ijsc.v2i1.126
16. Matlák J, Tihanyi J, and Rácz L. Relationship between reactive agility and change of direction speed in amateur soccer players. J Strength Cond Res. (2016) 30:1547–52. doi: 10.1519/JSC.0000000000001262
17. Čoh M, Vodičar J, Žvan M, Šimenko J, Stodolka J, Rauter S, et al. Are change-of-direction speed and reactive agility independent skills even when using the same movement pattern? J Strength Cond. Res. (2018) 32:1929–36. doi: 10.1519/JSC.0000000000002553
18. Williams CA, Oliver JL, Lloyd RS, and Granacher U. Talent development. In: Lloyd RS. LJ Oliver, editors. Strength Conditioning for Young Athletes: Science Application, London, New York, NY: Routledge (2020). p. 45–61.
19. Matos N, and Winsley RJ. Trainability of young athletes and overtraining. J Sports Sci Med. (2007) 6:353–67.
20. Beunen G, and Malina RM. Growth and physical performance relative to the timing of the adolescent spurt. Exerc Sport Sci Rev. (1988) 16:503–40. doi: 10.1249/00003677-198800160-00018
21. Pereira S, Garbeloto F, Guimaræs E, Santos C, Baxter-Jones A, Tani G, et al. Physical fitness spurts in childhood: a study in boys. Scand J Med Sci Sports. (2021) 31:47–55. doi: 10.1111/sms.13800
22. Viru A, Loko J, Harro M, Volver A, Laaneots L, and Viru M. Critical periods in the development of performance capacity during childhood and adolescence. Eur J Phys Educ. (1999) 4:75–119. doi: 10.1080/1740898990040106
23. Malina RM. Skill acquisition in childhood and adolescence. In: Hebestreit H, Bar-Or O, editors. The Young Athlete. Malden: Blackwell Publishing (2008). p. 96–111.
24. Jeffreys I. A task-based approach to developing context-specific agility. Strength Cond J. (2011) 33:52–9. doi: 10.1519/SSC.0b013e318222932a
25. Hewit JK, Cronin JB, and Hume PA. Understanding change of direction performance: a technical analysis of a 180° aerial catch and turn task. Int J Sports Sci Coach. (2012) 7:503–14. doi: 10.1260/1747-9541.7.3.503
26. Sheppard JM, Dawes JJ, Jeffreys I, Spiteri T, and Nimphius S. Broadening the view of agility: a scientific review of the literature. J Aust Strength Cond. (2014) 22:6–25.
27. Spiteri T, McIntyre F, Specos C, and Myszka S. Cognitive training for agility: the integration between perception and action. Strength Cond J. (2018) 40:39–46. doi: 10.1519/SSC.0000000000000310
28. Blimkie CJ, and Bar-Or O. Muscle strength, endurance, power: trainability during childhood. In: Hebestreit H, Bar-Or O, editors. The Young Athlete. Malden: Blackwell Publishing (2008). p. 65–83.
29. Behringer M, vom Heede A, Yue Z, and Mester J. Effects of resistance training in children and adolescents: a meta-analysis. Pediatrics. (2010) 126:1199–210. doi: 10.1542/peds.2010-0445
30. Oliver JL, and Lloyd RS. Speed agility training. In: Armstrong N, van Mechelen W, editors. Oxford Textbook of Children's Sport Exercise Medicine. Oxford: Oxford University Press (2017). p. 507–18.
31. Williams AM, and Ward P. Perceptual expertise: development in sport. In: Starkes JL. Ericsson AK, editors. In: Expert Performance in Sports: Advances in Research on Sport Expertise. Champaign; Windsor; Leeds: Human Kinetics (2003). p. 219–49.
32. Zemková E, and Hamar D. Age-related changes in agility time in children and adolescents. Int J Sci Res. (2014) 3:280–5.
33. Harrison CB, and McGuigan M. Monitoring assessment of young athletes. In: Lloyd RS, Oliver LJ, editors. Strength Conditioning for Young Athletes: Science Application. New York, NY; London: Routledge (2020). 62–76.
34. Baxter-Jones AD, and Sherar LB. Growth and maturation. In: Armstrong N, editors. Paediatric Exercise Physiology: Advances in Sport and Exercise Science Series. Saintt Louis: Elsevier Health Sciences) (2006). p. 1–26.
35. Hojka V, Stastny P, Rehak T, Gołas A, Mostowik A, Zawart M, et al. A systematic review of the main factors that determine agility in sport using structural equation modeling. J Hum Kinet. (2016) 52:115–23. doi: 10.1515/hukin-2015-0199
36. Paul DJ, Gabbett TJ, and Nassis GP. Agility in team sports: testing, training and factors affecting performance. Sports Med. (2016) 46:421–42. doi: 10.1007/s40279-015-0428-2
37. Inglis P, and Bird SP. Reactive agility tests - review and practical applications. J Aust Strength Cond. (2016) 24:62–9.
38. Morral-Yepes M, Moras G, Bishop C, and Gonzalo-Skok O. Assessing the reliability and validity of agility testing in team sports: a systematic review. J Strength Cond Res. (2020) 36:2035–49. doi: 10.1519/JSC.0000000000003753
39. Lloyd RS, Read P, Oliver JL, Meyers RW, Nimphius S, and Jeffreys I. Considerations for the development of agility during childhood and adolescence. Strength Cond J. (2013) 35:2–11. doi: 10.1519/SSC.0b013e31827ab08c
40. Tricco AC, Lillie E, Zarin W, O'Brien KK, Colquhoun H, Levac D, et al. PRISMA Extension for Scoping Reviews (PRISMA-ScR): checklist and explanation. Ann Intern Med. (2018) 169:467–73. doi: 10.7326/M18-0850
41. Thieschäfer L, Büsch, D,. Development Trainability of Agility in Youth: A Scoping Review Protocol (2021). Available online at: https://osf.io/73cxs (accessed July 21, 2021).
42. Jansen MG, Elferink-Gemser MT, Hoekstra AE, Faber IR, and Huijgen BC. Design of a tennis-specific agility test (TAT) for monitoring tennis players. J Hum Kinet. (2021). 80:239–50. doi: 10.2478/hukin-2021-0094
43. Thieschäfer L, and Büsch D. Data from: development and trainability of agility in youth: a systematic scoping review. Open Sci Framework. (2021b). doi: 10.17605/OSF.IO/8Y9NK
44. Peters MD, Marnie C, Tricco AC, Pollock D, Munn Z, Alexander L, et al. Updated methodological guidance for the conduct of scoping reviews. JBI Evid Implement. (2021) 19:3–10. doi: 10.1097/XEB.0000000000000277
45. Cohen J. Statistical Power Analysis for the Behavioral Sciences. 2nd ed. Hillsdale: Lawrence Erlbaum Associates (2013).
46. Cumming G. Cohen's d needs to be readily interpretable: comment on Shieh. Behav Res Methods. (2013) 45:968–71. doi: 10.3758/s13428-013-0392-4
48. Ardern CL, Büttner F, Andrade R, Weir A, Ashe MC, Holden S, et al. Implementing the 27 PRISMA 2020 statement items for systematic reviews in the sport and exercise medicine, musculoskeletal rehabilitation and sports science fields: the PERSiST (implementing Prisma in Exercise, Rehabilitation, Sport medicine and SporTs science) guidance. Br J Sports Med. (2022) 56:175–95. doi: 10.1136/bjsports-2021-103987
49. Serpell BG, Young WB, and Ford M. Are the perceptual and decision-making components of agility trainable? A preliminary investigation. J Strength Cond Res. (2011) 25:1240–8. doi: 10.1519/JSC.0b013e3181d682e6
50. Oberacker LM, Davis SE, Haff GG, Witmer CA, and Moir GL. The Yo-Yo IR2 test: physiological response, reliability, and application to elite soccer. J Strength Cond Res. (2012) 26:2734–40. doi: 10.1519/JSC.0b013e318242a32a
51. Knoop M, Fernandez-Fernandez J, and Ferrauti A. Evaluation of a specific reaction and action speed test for the soccer goalkeeper. J Strength Cond Res. (2013) 27:2141–48. doi: 10.1519/JSC.0b013e31827942fa
52. Lloyd RS, Oliver JL, Radnor JM, Rhodes BC, Faigenbaum AD, and Myer GD. Relationships between functional movement screen scores, maturation and physical performance in young soccer players. J Sports Sci. (2015) 33:11–19. doi: 10.1080/02640414.2014.918642
53. Chaouachi A, Chtara M, Hammami R, Chtara H, Turki O, and Castagna C. Multidirectional sprints and small-sided games training effect on agility and change of direction abilities in youth soccer. J Strength Cond Res. (2014) 28:3121–7. doi: 10.1519/JSC.0000000000000505
54. Young WB, and Rogers N. Effects of small-sided game and change-of-direction training on reactive agility and change-of-direction speed. J Sports Sci. (2014) 32:307–14. doi: 10.1080/02640414.2013.823230
55. Nimmerichter A, Weber NJ, Wirth K, and Haller A. Effects of video-based visual training on decision-making and reactive agility in adolescent football players. Sports. (2015) 1:4. doi: 10.3390/sports4010001
56. Bartels T, Proeger S, Meyer D, Rabe J, Brehme K, Pyschik M, et al. Hochreaktives Training bei jugendlichen Fußballspielern. [Fast response training in youth soccer players]. Sportverletz Sportschaden. (2016) 30:143–8. doi: 10.1055/s-0042-110250
57. Born D-P, Zinner C, Düking P, and Sperlich B. Multi-directional sprint training improves change-of-direction speed and reactive agility in young highly trained soccer players. J Sports Sci Med. (2016) 15:314–9.
58. Chaalali A, Rouissi M, Chtara M, Owen A, Bragazzi NL, Moalla W, et al. Agility training in young elite soccer players: promising results compared to change of direction drills. Biol. Sport. (2016) 33:345–51. doi: 10.5604/20831862.1217924
59. Hornigova H, Dolezajova L, Sedlacek J, Sagat P, and Balint G. Determination of female table tennis sport performance by senzor motoric reaction time. Gymnasium. (2016) 17. doi: 10.29081/gsjesh.2016.17.2.18
60. Trecroci A, Milanović Z, Rossi A, Broggi M, Formenti D, and Alberti G. Agility profile in sub-elite under-11 soccer players: is SAQ training adequate to improve sprint, change of direction speed and reactive agility performance?Res. Sports Med. (2016) 24:331–40. doi: 10.1080/15438627.2016.1228063
61. Fiorilli G, Mitrotasios M, Iuliano E, Pistone EM, Aquino G, Calcagno G, et al. Agility and change of direction in soccer: differences according to the player ages. J Sports Med Phys Fit. (2017) 57:1597–604. doi: 10.23736/S0022-4707.16.06562-2
62. Fiorilli G, Mariano I, Iuliano E, Giombini A, Ciccarelli A, Buonsenso A, et al. Isoinertial eccentric-overload training in young soccer players: effects on strength, sprint, change of direction, agility and soccer shooting precision. J Sports Sci Med. (2020) 19:213–23.
63. Holding R, Meir R, and Zhou S. Can previewing sport-specific video influence reactive-agility response time? Int J Sports Physiol Perform. (2017) 12:224–9. doi: 10.1123/ijspp.2015-0803
64. Šimonek J, Horička P, and Hianik J. The differences in acceleration, maximal speed and agility between soccer, basketball, volleyball and handball players. J Hum Sport Exerc. (2017) 12:73–82. doi: 10.14198/jhse.2017.121.06
65. Bekris E, Gissis I, and Kounalakis S. The dribbling agility test as a potential tool for evaluating the dribbling skill in young soccer players. Res Sports Med. (2018) 26:425–35. doi: 10.1080/15438627.2018.1492395
66. Horicka P, Simonek J, and Brodani J. Diagnostics of reactive and running agility in young football players. Phys Act Rev. (2018) 6:1–7. doi: 10.16926/par.2018.06.05
67. Horička P, Šimonek J, Paška L Relationship between reactive agility, cognitive abilities, and intelligence in adolescents. J Phys Educ Sport. (2020) 20:2263–8. doi: 10.7752/jpes.2020.s3304
68. Pojskic H, Åslin E, Krolo A, Jukic I, Uljevic O, Spasic M, et al. Importance of reactive agility and change of direction speed in differentiating performance levels in junior soccer players: reliability and validity of newly developed soccer-specific tests. Front Physiol. (2018) 9:506. doi: 10.3389/fphys.2018.00506
69. Horníková H, Doležajová L, and Krasňanová I Lednický A. Differences in reaction time and agility time in 11 to 14 years old schoolboys. J Phys Educ Sport. (2019) 19:1355–60. doi: 10.7752/jpes.2019.s4196
70. Zouhal H, Abderrahman AB, Dupont G, Truptin P, Le Bris R, Le Postec E, et al. Effects of neuromuscular training on agility performance in elite soccer players. Front Physiol. (2019) 10:947. doi: 10.3389/fphys.2019.00947
71. Bakal'ár I, Šimonek J, Kanásová J, Krčmárová B, and Krčmár M. Multiple athletic performances, maturation, and functional movement screen total and individual scores across different age categories in young soccer players. J Exerc Rehabil. (2020) 16:432–41. doi: 10.12965/jer.2040546.273
72. Di Mascio M, Ade J, Musham C, Girard O, and Bradley PS. Soccer-specific reactive repeated-sprint ability in elite youth soccer players: maturation trends and association with various physical performance tests. J Strength Cond Res. (2020) 34:3538–45. doi: 10.1519/JSC.0000000000002362
73. Krolo A, Gilic B, Foretic N, Pojskic H, Hammami R, Spasic M, et al. Agility testing in youth football (soccer)players; evaluating reliability, validity, and correlates of newly developed testing protocols. Int J Environ Res Public Health. (2020) 17:294. doi: 10.3390/ijerph17010294
74. Kuo KP, Tsai HH, Lin CY, and Wu WT. Verification and evaluation of a visual reaction system for badminton training. Sensors. (2020) 20:6808. doi: 10.3390/s20236808
75. Popowczak M, Domaradzki J, Rokita A, Zwierko M, and Zwierko T. Predicting visual-motor performance in a reactive agility task from selected demographic, training, anthropometric, and functional variables in adolescents. Int J Environ Res Public Health. (2020) 17:5322. doi: 10.3390/ijerph17155322
76. Sekulic D, Gilic B, Foretic N, Spasic M, Uljević O, and Veršić Š. Fitness profiles of professional futsal players: identifying age-related differences. Biomed Hum Kinet. (2020) 12:212–20. doi: 10.2478/bhk-2020-0027
77. Veršić Š, Foretić N, Spasić M, and Uljević O. Predictors of agility in youth soccer players: contextualizing the influence of biological maturity. Kinesiol Slov. (2020) 26:31–47.
78. AndrašićS, Gušić M, Stanković M, Mačak D, Bradić A, Sporiš G, et al. Speed, change of direction speed and reactive agility in adolescent soccer players: age related differences. Int J Environ Res Public Health. (2021) 18:5883. doi: 10.3390/ijerph18115883
79. Arede J, Carvalho M, Esteves P, de las Heras B, and Leite N. Exploring the effects of LED lighting training program on motor performance among young athletes. Creativity Res J. (2021) 33:63–73. doi: 10.1080/10400419.2020.1817693
80. Horicka P, and Simonek J. Age-related changes of reactive agility in football. Phys Act Rev. (2021) 9:16–23. doi: 10.16926/par.2021.09.03
81. Gušić M, Andrašić S, Molnar S, and Cvetković M. Speed, change of direction speed reactive agility in adolescent soccer players: a comparative study. In: Pantelić S, editor. XX Scientific Conference “FIS Communications 2017” in Physical Education Sport Recreation. Niš (2017). p. 347–50.
82. Büchel D, Lehmann T, and Baumeister J. Effect of age on reactive multidirectional speed in highly trained young soccer players. In: Sperlich B, Kunz P, Düking P, editors. Book of Abstract-German Exercise Science Training Conference (GEST19) of the German Society of Sport Science (dvs). Berlin: Springer (2019). p. 9.
83. Savolainen E, Vänttinen T, Ahtiainen JP, and Agility Performance With Without Ball During a Novel Football-Specific Video Stimulus Test in Junior Players (2020). Available online at: https://www.jyu.fi/sport/en/research/congresses/past-events/strength-and-endurance/abstracts/agility-performance-with-and-without-ball-during-a-novel-football-specific-video-stimulus-test-in-junior-players (accessed on October 25, 2021).
84. Dugdale JH. Towards a Better Understanding of the Value of Physical Fitness Testing Within the Identification and Development of Youth Soccer Players. (dissertation). Stirling: University of Stirling (2019).
85. Zemková E, and Hamar D. Toward an Understanding of Agility Performance. 2nd ed. Boskovice: Albert (2014).
87. Mirwald RL, Baxter-Jones AD, Bailey DA, and Beunen GP. An assessment of maturity from anthropometric measurements. Med Sci Sports Exerc. (2002) 34:689–94. doi: 10.1249/00005768-200204000-00020
88. Moore SA, McKay HA, MacDonald H, Nettlefold L, Baxter-Jones AD, Cameron N, et al. Enhancing a somatic maturity prediction model. Med Sci Sports Exerc. (2015) 47:1755–64. doi: 10.1249/MSS.0000000000000588
89. Chelladurai P. Manifestations of agility. J Can Assoc Health Phys Edu. Recreation. (1976) 42:36–41.
90. Stratton G, and Oliver JL. The impact of growth maturation on physical performance. In: Lloyd RS. Oliver JL, editors. Strength Conditioning for Young Athletes: Science Application. London; New York, NY: Routledge (2020). p. 3–20.
91. Drake D, Kennedy R, Davis J, Godfrey M, MacLeod S, and Davis A. A step towards a field based agility test in team sports. Int J Sport Exerc Med. (2017) 3:079. doi: 10.23937/2469-5718/1510079
92. Young WB, and Murray MP. Reliability of a field test of defending and attacking agility in Australian football and relationships to reactive strength. J Strength Cond Res. (2017) 31:509–16. doi: 10.1519/JSC.0000000000001498
93. Spiteri T, Hart NH, and Nimphius S. Offensive and defensive agility: a sex comparison of lower body kinematics and ground reaction forces. J Appl Biomech. (2014). 30:514–20. doi: 10.1123/jab.2013-0259
94. Rayner R. Training and Testing of 1v1 Agility in Australian Football (dissertation). Ballarat: Federation University Australia (2020).
95. Liefeith A, Kiely J, Collins D, and Richards J. Back to the Future-in support of a renewed emphasis on generic agility training within sports-specific developmental pathways. J Sports Sci. (2018) 36:2250–5. doi: 10.1080/02640414.2018.1449088
96. Gabbett TJ, Kelly JN, and Sheppard JM. Speed, change of direction speed, and reactive agility of rugby league players. J Strength Cond Res. (2008) 22:174–81. doi: 10.1519/JSC.0b013e31815ef700
97. Spiteri T, Nimphius S, Hart NH, Specos C, Sheppard JM, and Newton RU. Contribution of strength characteristics to change of direction and agility performance in female basketball athletes. J Strength Cond Res. (2014). 28:2415–23.
98. Young WB, Miller IR, and Talpey SW. Physical qualities predict change-of-direction speed but not defensive agility in Australian rules football. J Strength Cond Res. (2015). 29:206–12. doi: 10.1519/JSC.0000000000000614
99. Bayraktar I. The influences of speed, COD speed and balance on reactive agility performance in team handball. Int J Environ Sci Educ. (2017) 12:451–61. doi: 10.12973/ijese.2017.2140a
100. Scanlan A, Humphries B, Tucker PS, and Dalbo V. The influence of physical and cognitive factors on reactive agility performance in men basketball players. J Sports Sci. (2014) 32:367–74. doi: 10.1080/02640414.2013.825730
101. Henry GJ, Dawson B, Lay BS, and Young WB. Relationships between reactive agility movement time and unilateral vertical, horizontal, lateral jumps. J Strength Cond Res. (2016) 30:2514–21. doi: 10.1519/JSC.0b013e3182a20ebc
102. Abernethy B. The effects of age and expertise upon perceptual skill development in a racquet sport. Res Q Exerc Sport. (1988) 59:210–21. doi: 10.1080/02701367.1988.10605506
103. Thomas KT. The development of sport expertise: from leeds to MVP legend. Quest. (1994) 46:199–210. doi: 10.1080/00336297.1994.10484121
104. Vänttinen T, Blomqvist M, Luhtanen P, and Häkkinen K. Effects of age and soccer expertise on general tests of perceptual and motor performance among adolescent soccer players. Percept Mot Skills. (2010) 110:675–92. doi: 10.2466/pms.110.3.675-692
105. Quatman-Yates CC, Quatman CE, Meszaros AJ, Paterno MV, and Hewett TE. A systematic review of sensorimotor function during adolescence: a developmental stage of increased motor awkwardness? Br J Sports Med. (2012) 46:649–55. doi: 10.1136/bjsm.2010.079616
106. Vänttinen T, Blomqvist M, Nyman K, and Häkkinen K. Changes in body composition, hormonal status, and physical fitness in 11-, 13-, and 15-year-old Finnish regional youth soccer players during a two-year follow-up. J Strength Cond Res. (2011) 25:3342–51. doi: 10.1519/JSC.0b013e318236d0c2
107. Golle K, Muehlbauer T, Wick D, and Granacher U. Physical fitness percentiles of german children aged 9-12 years: findings from a longitudinal study. PLoS ONE. (2015) 10:e0142393. doi: 10.1371/journal.pone.0142393
108. Philippaerts RM, Vaeyens R, Janssens M, van Renterghem B, Matthys D, Craen R, et al. The relationship between peak height velocity and physical performance in youth soccer players. J Sports Sci. (2006) 24:221–30. doi: 10.1080/02640410500189371
109. Beunen G, and Malina RM. Growth biologic maturation: relevance to athletic performance. In: Hebestreit H, Bar-Or O, editors. The Young Athlete. Malden: Blackwell Publishing (2008). p. 3–17.
110. Lloyd RS, Oliver JL, Faigenbaum AD, Myer GD, and de Ste Croix MBA. Chronological age vs. biological maturation: implications for exercise programming in youth. J Strength Cond Res. (2014) 28:1454–64. doi: 10.1519/JSC.0000000000000391
111. Mota T, Afonso J, Sá M, and Clemente FM. An agility training continuum for team sports: from cones and ladders to small-sided games. Strength Cond J. (2022) 44:46–56. doi: 10.1519/SSC.0000000000000653
113. Dos'Santos T, McBurnie A, Thomas C, Jones PA, and Harper D. Attacking agility actions: match play contextual applications with coaching and technique guidelines. Strength Cond J. (2022) 1–17. doi: 10.1519/SSC.0000000000000697
114. Broadbent DP, Causer J, Williams AM, and Ford PR. Perceptual-cognitive skill training and its transfer to expert performance in the field: future research directions. Eur J Sport Sci. (2015) 15:322–31. doi: 10.1080/17461391.2014.957727
115. Hagemann N, Lotz S, and Cañal-Bruland R. Wahrnehmungs-Handlungs-Kopplung beim taktischen Entscheidungstraining - eine exploratorische Studie. E J Beweg Train. (2008) 2:17–27.
116. Davids K, Araújo D, Correia V, and Vilar L. How small-sided and conditioned games enhance acquisition of movement and decision-making skills. Exerc Sport Sci Rev. (2013) 41:154–61. doi: 10.1097/JES.0b013e318292f3ec
117. Farrow D, and Abernethy B. Can anticipatory skills be learned through implicit video-based perceptual training? J Sports Sci. (2002) 20:471–85. doi: 10.1080/02640410252925143
118. Smeeton NJ, Williams AM, Hodges NJ, and Ward P. The relative effectiveness of various instructional approaches in developing anticipation skill. J Exp Psychol Appl. (2005) 11:98–110. doi: 10.1037/1076-898X.11.2.98
119. Gabbett T, Rubinoff M, Thorburn L, and Farrow D. Testing and training anticipation skills in softball fielders. Int J Sports Sci Coach. (2007) 2:15–24. doi: 10.1260/174795407780367159
120. Lloyd RS, Faigenbaum AD, Stone MH, Oliver JL, Jeffreys I, Moody JA, et al. Position statement on youth resistance training: the 2014 International Consensus. Br J Sports Med. (2014). 48:498–505. doi: 10.1136/bjsports-2013-092952
121. Lesinski M, Herz M, Schmelcher A, and Granacher U. Effects of resistance training on physical fitness in healthy children and adolescents: an umbrella review. Sports Med. (2020) 50:1901–1928. doi: 10.1007/s40279-020-01327-3
122. Tous-Fajardo J, Gonzalo-Skok O, Arjol-Serrano JL, and Tesch P. Enhancing change-of-direction speed in soccer players by functional inertial eccentric overload and vibration training. Int J Sports Physiol Perform. (2016) 11:66–73. doi: 10.1123/ijspp.2015-0010
123. Bourgeois FA, Gamble P, Gill ND, and McGuigan MR. Effects of a six-week strength training programme on change of direction performance in youth team sport athletes. Sports. (2017) 5:83. doi: 10.3390/sports5040083
124. Nygaard Falch H, Guldteig Rædergård H, and van den Tillaar R. Effect of different physical training forms on change of direction ability: a systematic review and meta-analysis. Sports Med Open. (2019) 5:53. doi: 10.1186/s40798-019-0223-y
125. Asadi A, Arazi H, Ramirez-Campillo R, Moran J, and Izquierdo M. Influence of maturation stage on agility performance gains after plyometric training: a systematic review and meta-analysis. J Strength Cond Res. (2017) 31:2609–17. doi: 10.1519/JSC.0000000000001994
126. Henry GJ, Dawson B, Lay BS, and Young WB. Validity of a reactive agility test for Australian football. Int J Sports Physiol Perform. (2011) 6:534–45. doi: 10.1123/ijspp.6.4.534
127. Young WB, Farrow D, Pyne D, McGregor W, and Handke T. Validity and reliability of agility tests in junior Australian football players. J Strength Cond Res. (2011) 25:3399–403. doi: 10.1519/JSC.0b013e318215fa1c
128. Spiteri T, Nimphius S, and Wilkie J. Comparison of running times during reactive offensive and defensive agility protocols. J Aust Strength Cond. (2012) 20:73–8.
129. Nimphius S, Callaghan SJ, Bezodis NE, and Lockie RG. Change of direction and agility tests. Strength Cond J. (2018) 40:26–38. doi: 10.1519/SSC.0000000000000309
Keywords: reactive agility, unplanned change-of-direction, multi-directional speed, pediatric performance, youth fitness, maturation
Citation: Thieschäfer L and Büsch D (2022) Development and trainability of agility in youth: A systematic scoping review. Front. Sports Act. Living 4:952779. doi: 10.3389/fspor.2022.952779
Received: 25 May 2022; Accepted: 08 August 2022;
Published: 08 September 2022.
Edited by:
Thomas Dos'Santos, Manchester Metropolitan University, United KingdomReviewed by:
Scott Talpey, Federation University Australia, AustraliaCopyright © 2022 Thieschäfer and Büsch. This is an open-access article distributed under the terms of the Creative Commons Attribution License (CC BY). The use, distribution or reproduction in other forums is permitted, provided the original author(s) and the copyright owner(s) are credited and that the original publication in this journal is cited, in accordance with accepted academic practice. No use, distribution or reproduction is permitted which does not comply with these terms.
*Correspondence: Lutz Thieschäfer, THV0ei5UaGllc2NoYWVmZXJAdW5pLW9sZGVuYnVyZy5kZQ==
Disclaimer: All claims expressed in this article are solely those of the authors and do not necessarily represent those of their affiliated organizations, or those of the publisher, the editors and the reviewers. Any product that may be evaluated in this article or claim that may be made by its manufacturer is not guaranteed or endorsed by the publisher.
Research integrity at Frontiers
Learn more about the work of our research integrity team to safeguard the quality of each article we publish.