- 1Department of Sport, Exercise and Rehabilitation, Northumbria University, Newcastle upon Tyne, United Kingdom
- 2Department of Neurology, Oregon Health and Science University, Portland, OR, United States
- 3Veterans Affairs Portland Health Care System, Portland, OR, United States
- 4National Center for Rehabilitative Auditory Research, Veterans Affairs Portland Health Care System, Portland, OR, United States
- 5Department of Family Medicine, Oregon Health & Science University, Portland, OR, United States
- 6Orthopaedics and Rehabilitation, Oregon Health & Science University, Portland, OR, United States
Mild traumatic brain injury (mTBI), or concussion, occurs following a direct or indirect force to the head that causes a change in brain function. Many neurological signs and symptoms of mTBI can be subtle and transient, and some can persist beyond the usual recovery timeframe, such as balance, cognitive or sensory disturbance that may pre-dispose to further injury in the future. There is currently no accepted definition or diagnostic criteria for mTBI and therefore no single assessment has been developed or accepted as being able to identify those with an mTBI. Eye-movement assessment may be useful, as specific eye-movements and their metrics can be attributed to specific brain regions or functions, and eye-movement involves a multitude of brain regions. Recently, research has focused on quantitative eye-movement assessments using eye-tracking technology for diagnosis and monitoring symptoms of an mTBI. However, the approaches taken to objectively measure eye-movements varies with respect to instrumentation, protocols and recognition of factors that may influence results, such as cognitive function or basic visual function. This review aimed to examine previous work that has measured eye-movements within those with mTBI to inform the development of robust or standardized testing protocols. Medline/PubMed, CINAHL, PsychInfo and Scopus databases were searched. Twenty-two articles met inclusion/exclusion criteria and were reviewed, which examined saccades, smooth pursuits, fixations and nystagmus in mTBI compared to controls. Current methodologies for data collection, analysis and interpretation from eye-tracking technology in individuals following an mTBI are discussed. In brief, a wide range of eye-movement instruments and outcome measures were reported, but validity and reliability of devices and metrics were insufficiently reported across studies. Interpretation of outcomes was complicated by poor study reporting of demographics, mTBI-related features (e.g., time since injury), and few studies considered the influence that cognitive or visual functions may have on eye-movements. The reviewed evidence suggests that eye-movements are impaired in mTBI, but future research is required to accurately and robustly establish findings. Standardization and reporting of eye-movement instruments, data collection procedures, processing algorithms and analysis methods are required. Recommendations also include comprehensive reporting of demographics, mTBI-related features, and confounding variables.
Introduction
Eye movements are the basis of how humans gather information about the environment, which is then used to allow the perception of vital information needed for safe navigation or task performance. Eye movements have been investigated via various methods since the 1700s (Porterfield, 1752), with progression from eye-tracking that used large-scale photographic technology to invasive high resolution scleral search coils, and finally to more modern non-invasive small-scale infrared camera systems (Land, 2006). There are many eye movements that can be captured with modern technologies, such as saccades (fast eye movements), fixations (pauses on areas of interest), smooth pursuits (fixations on moving objects), and nystagmus (repetitive non-voluntary resetting eye movements; Tatler and Wade, 2003; Duchowski, 2007; Holmqvist et al., 2011). A combination of these eye movements provide the mechanisms through which we are able to explore and sample our environment (McPeek et al., 2000; Deubel and Schneider, 2003; Tatler and Wade, 2003; Marigold and Patla, 2008; Tatler, 2009; Stuart et al., 2014a). In order to derive and classify the different types of eye movements, a range of spatial-temporal and kinematic outcome variables are typically used, such as latency, velocity, acceleration, number/frequency, timing and duration (Duchowski, 2007; Holmqvist et al., 2011; Stuart et al., 2019a). Advancements in eye-tracking technologies have enabled eye movements to be monitored with small-scale devices that can be used in a variety of environments, such as research laboratories, clinics, field-based and community facilities. Similarly, collection of eye movement data with eye-tracking devices has progressed from traditional static tasks (e.g., seated or standing) to more dynamic tasks (e.g., walking or navigation of the environment), which is an important step toward understanding the impact that deficits can have on real-world function. The development of simple, high resolution, quantitative eye-tracking technologies is allowing disease or injury-specific impairments to be uncovered.
Eye movements are increasingly being studied in mild traumatic brain injury (mTBI) (commonly referred to as concussion; Thiagarajan et al., 2011; Ventura et al., 2015; Hunt et al., 2016; Snegireva et al., 2018), as eye-tracking protocols can be used to detect subtle deficits in cognitive, motor and visual processes that may occur following a head injury (Liversedge and Findlay, 2000; Maruta et al., 2010a). Detection of mTBI and monitoring of recovery of subtle impairments is not always possible with conventional means, such as neuroimaging (Eierud et al., 2014) or clinical assessments (McCrea et al., 2015). A lack of accurate and robust diagnostics, biomarkers and outcome measures leads to mTBI going undetected (Jeter et al., 2013; Kim et al., 2018; Quinones-Ossa et al., 2019). Undetected mTBI can lead to impaired functional activities, self-medication and return to sport/work/play before recovery is complete, which may lead to increased future injury risk and other health burden (McPherson et al., 2019; Reneker et al., 2019). Interestingly, the incidence of self-reported visual impairments in those following a traumatic brain injury has been reported to be as high as 90% (Ciuffreda et al., 2007), but incidence reports vary with the lowest recorded at 22% (Lara et al., 2001; Cockerham et al., 2009). This is not surprising, as the processing of vision and control of eye-movements is known to involve a large proportion of the brains circuits and regions (Antoniades et al., 2013), as well as underlying neural pathways and structures (Mays et al., 1986; Noda, 1991; Catz and Thier, 2007; Shinoda et al., 2019). For example, visual signals from the retina are sent to the superior colliculus then initially processed by the lateral geniculate nucleus, pulvina and mediordorsal thalamus, where signals are then sent for top-down visual processing at the pre-frontal cortex, frontal eye-field, supplementary eye field and lateral intraparietal area; as well as basic visual processing at the visual cortex (V1/V2, V4), middle temporal area and inferotemporal cortex, with the striatum, substantia nigra pars reticulate, and brainstem involved in eye movement initiation and control (Baluch and Itti, 2011). Therefore, as a result of an mTBI eye movements may be impaired and eye-movement recordings could provide a simple, quick and non-invasive means to quantify impairments and recovery in mTBI (Snegireva et al., 2019). Furthermore, imaging evidence suggests that saccades, smooth pursuits and nystagmus eye movements activate largely similar neural structures (Konen et al., 2005; Dieterich et al., 2009), and therefore individual eye movement tests may be of value to mTBI diagnosis.
Eye-tracking technology has been used to further understand mTBI-related impairments in eye-movements (Akhand et al., 2019), demonstrating some efficacy for use in mTBI assessment and clinical diagnostics. However, until recently most eye movement research in mTBI involved simple, subjective, clinical, self-reported, or symptom-based tasks that could be performed in the field or within clinic with minimal training [e.g., the vestibular/ocular-motor screening (VOMS) Mucha et al., 2014]. Several previous reviews of studies that have used self-report/symptom-based outcomes have reported vision impairments in mTBI (Thiagarajan et al., 2011; Hunt et al., 2016; Whitney and Sparto, 2019). However, many mTBI subjective rating scales have not had rigorous validity or reliability testing (Alla et al., 2009), and scales may miss subtle symptoms due to reliance on clinician experience and self-report (Meier et al., 2015). Progression to the use of eye-tracking devices in research that are capable of capturing eye-movements at high speed and providing quantifiable outcomes has led to an array of testing protocols (Hunt et al., 2016; Snegireva et al., 2018), indicating a lack of standardization that limits outcome interpretation and generalizability. Several recent reviews provided overviews of reported impairment of eye-movement outcome measures in mTBI (Hunt et al., 2016; Snegireva et al., 2018), but provided limited details regarding the use of specific eye-tracking methodologies and how differences in methods or devices may impact findings. Researchers who want to conduct similar research are therefore left with the choice between numerous eye-tracking devices, outcomes and protocols that differ in many respects and complexities. In the process of developing robust protocols it is helpful to have evidence-based recommendations. We therefore examined previous work that assessed eye movements in mTBI and healthy control participants in order to provide some guidance regarding the selection of appropriate methodology.
We focused the review on the following: (1) eye-tracking instrumentation used to examine people following an mTBI compared to healthy controls; (2) commonly reported eye movement outcomes from eye-tracking studies; (3) mTBI specific influences on these eye movement outcomes; and (4) recommendations concerning future protocols.
Methods
Search Strategy
The key terms were “mild Traumatic Brain Injury,” “Eye movement,” and “Eye-tracking.” A list of synonyms was created for each key term (Figure 1). Key terms were matched and expanded with medical subject headings (MeSH) in each separate database where appropriate. Databases searched included Medline/Pubmed (from 1950), PsychInfo (from 1806), CINAHL (from 1937), and Scopus to July 2019. Studies were relevant if they used terminology that focused on eye movement tracking in those with mTBI and healthy control subjects in the title, abstract or keywords. Articles with titles related to “sleep,” “monkeys,” “rats,” “mice,” or “animal” models were excluded using separate key terms.
An initial title screen for relevant articles was performed by the reviewer (SS) once the searched database results had been combined. After the initial title screen, both the titles and abstracts of the selected articles were reviewed by three independent reviewers (SS, DM, LP). A review of the full text was required if it was not clear from the title or abstract whether the study met the review criteria.
Inclusion and Exclusion Criteria
Articles were included if they reported use of an eye-tracking measurement instrument to quantify eye movements (i.e., saccades, smooth pursuits, convergence, fixations etc.) in people with mTBI. Studies were included only if they tested a healthy control cohort or used a baseline pre-injury test as a control for comparison with mTBI cohorts so that injury-specific differences could be identified. If articles including another clinical cohort (i.e., whiplash, acquired brain injury, moderate to severe TBI; Samadani et al., 2015), or an additional visual assessment that was not quantified via eye-tracking (e.g., measurement of convergence), only the eye-tracking data from the mTBI and healthy control cohorts was reviewed. If clinical cohorts were combined [i.e., mTBI with traumatic whiplash (Herishanu, 1992) or mild-to-severe TBI (Vakil et al., 2019)] or studies used a control group that had experienced an mTBI and were classified as recovered compared to a symptomatic mTBI population (Heitger et al., 2009), then the data were not reviewed. Acute and chronic (including post-mTBI syndrome) mTBI cohorts were reviewed, but studies without a diagnosis of an mTBI [i.e., repetitive head injuries or those with a self-reported history of head injuries with no current symptoms or recent referral to study by a clinician (Rizzo et al., 2016)] were excluded. Rehabilitation studies that did not include a baseline examination, or did not include any cross-sectional comparison to healthy controls were not reviewed (Kaldoja et al., 2015; Johansson et al., 2017). Only articles written in English were considered for review and any abstracts, case studies, reviews, book chapters, commentaries, discussion papers, editorials or conference proceedings were excluded.
Data Extraction
Data were extracted by the reviewer (SS) and were synthesized into table format by the reviewer (SS) and a second reviewer (LP) confirmed the entered data (Tables 1–3). Data included demographic, eye movement measurement instruments, eye movement outcomes, study protocol and key findings.
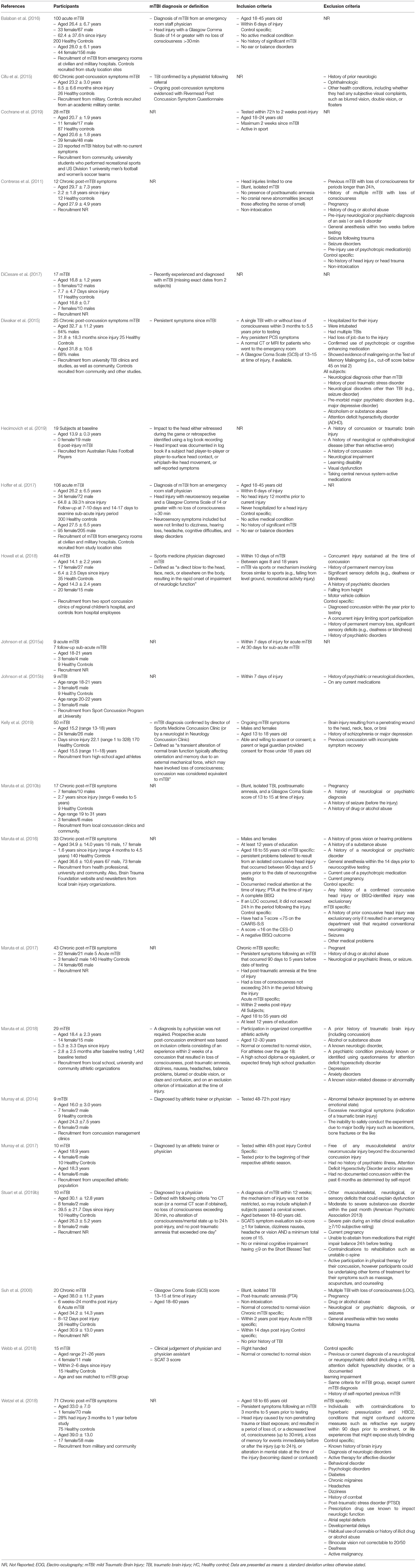
Table 1. Participant characteristics, mTBI diagnosis or definition, inclusion, and exclusion criteria.
Results
The Evidence Base
The search strategy yielded 86 articles, excluding duplicates (Figure 2–adapted from Moher et al., 2009). There was an initial screening in 128 articles of interest of which 22 were identified for inclusion for review by consensus of the screening reviewers (SS, LP, DM). Of the title screened 50 were excluded for not meeting inclusion criteria of the review. The majority of screened studies were excluded because they were either not relevant or did not provide quantitative measurement of eye movements in mTBI (Figure 2).
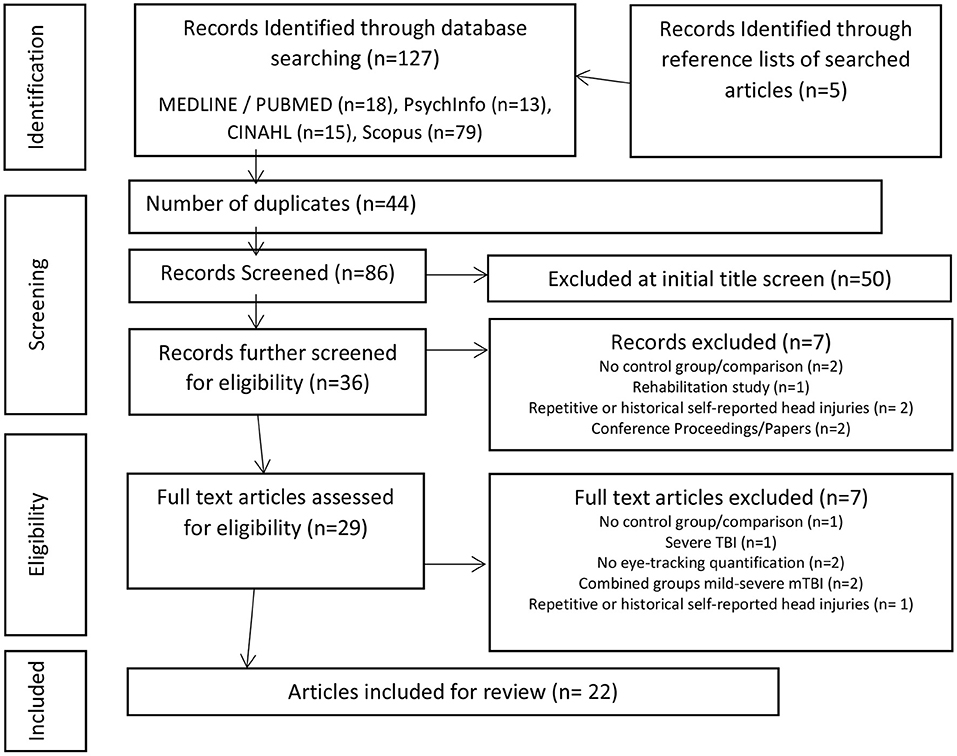
Figure 2. PRISMA flow chart of study design (Adapted from Moher et al., 2009).
Participants
The reviewed articles (n = 22) investigated healthy controls and mTBI with average age ranges between 13 and 39 years old (Table 1), with the majority of the studies including both males and females. Several studies did not provide specific demographic characteristics of participants, such as age (Maruta et al., 2010b; Johnson et al., 2015a; Webb et al., 2018), sex (Suh et al., 2006; Contreras et al., 2011; Cifu et al., 2015), time since injury (Johnson et al., 2015a,b; Maruta et al., 2017; Webb et al., 2018; Cochrane et al., 2019) etc. There were also various inclusion and exclusion criteria for participants within the reviewed studies, with little consensus and a lack of reporting in some studies. Seven of the studies examined subjects with chronic post-mTBI symptoms (time since injury ranged from 3 months to 5 years) and 13 studies examined subjects with acute/sub-acute mTBI (time since injury ranged from within 2–40 days post-injury, Table 1). The majority of the studies compared mTBI to healthy controls, but two studies (Maruta et al., 2018; Hecimovich et al., 2019) examined athletes during a pre-season baseline and then a follow-up post-injury. One study (Kelly et al., 2019) investigated mTBI subjects at an average of 22.2 days post-injury, but time since injury ranged from 1 to 328 days, so both acute and chronic mTBI subjects were included as one cohort. Similarly, two other studies grouped acute and chronic mTBI for all of their data analysis (Suh et al., 2006; Wetzel et al., 2018). Several studies also examined the same mTBI and control cohorts but produced one article on the baseline acute injury testing and another on the follow-up sub-acute periods (Johnson et al., 2015a,b; Balaban et al., 2016; Hoffer et al., 2017).
Instruments
Eye movements in the reviewed articles were measured using a variety of eye-tracking instruments, which largely depended on the desired eye-movement outcome or task being evaluated. For example, stationary eye-trackers were used for activities where restricted head movement was required, whereas mobile eye-trackers tended to be used for tasks that allowed head movement (i.e., walking or playing a computer game while standing balance was also examined; Murray et al., 2014, 2017; Stuart et al., 2019b). The 22 articles described an array of instrumentation including desk or computer-mounted infrared eye-trackers, rotary chairs within enclosed rooms, tethered head-mounted eye-trackers and fully mobile eye-trackers (Table 2). The sampling frequencies used to record eye movements varied considerably, despite many studies using similar devices (frequency range 60–1,000 Hz, Table 2). One study (Hecimovich et al., 2019) using the King-Devick eye-tracking system did not report the sampling frequency of the device.
Reliability and Validity
Of particular importance was that none of the reviewed studies reported the validity or reliability of the eye-tracking instrumentation used, and studies provided no detail regarding manufacturer specifications of the equipment (i.e., accuracy of tracking).
There was also very poor reporting of specific data processing or analysis methods used to derive the outcomes of interest within the reviewed studies. Only one study comprehensively provided their eye-tracking data processing method to derive eye movement outcomes, as the article developed and validated an eye-tracker algorithm to derive saccades while walking in mTBI and controls (Stuart et al., 2019b). Whereas another study reported that if there was <70% of eye-tracking data available then their instrumentation stated the trial was not valid and it was repeated until a valid trial was collected (Cochrane et al., 2019). One study also reported a comparison of their smooth pursuit synchronization index outcome with a traditional measure of velocity error to validate their outcome measure (Contreras et al., 2011). One study used commercial software (Oculogica, Inc.) to process their data (Howell et al., 2018) and referred to several previous studies that had also used this software to suggest its validity. However, validity of the commercial software is unclear, as it should be noted that none of the studies that were referenced examined or reported the data processing involved.
Test re-test reliability was performed in one study to examine their eye movement outcomes (Cochrane et al., 2019), but it was only performed in healthy controls. Results showed that their eye tracker (I-Portal, Neuro Kinetics Inc.) had poor to moderate reliability [0.02 to 0.71 inter-class correlation coefficient (ICC)] for saccadic accuracy and smooth pursuits, but saccadic latencies and optokinetic gains had better reliability (0.57–0.74 ICC).
Outcome Measures
Reviewed studies provided outcomes on saccadic (n = 12), fixation (n = 3), smooth pursuit (n = 13), and nystagmus (n = 4) eye movements, and there were a plethora of outcomes reported for these eye movements (Table 2, Supplementary Table 1). However, the majority of the reviewed studies did not define their eye movement classifications (i.e., no thresholds or criteria for eye movement detection and measurement). Several studies (Maruta et al., 2010b; Contreras et al., 2011; Cifu et al., 2015; Diwakar et al., 2015; DiCesare et al., 2017; Wetzel et al., 2018; Stuart et al., 2019b) did provide some details regarding definitions but these substantially varied between the studies (Table 2, Supplementary Table 1). Three studies provided no outcomes specific to traditional eye movements (Suh et al., 2006; Murray et al., 2014; Howell et al., 2018), but instead reported on novel outcomes of “Gaze Stabilization” (a fixation measure), “Eye Skew” (an asymmetry measure), and “Oculomotor error” (a smooth pursuit measure) that authors developed for their individual studies. Overall, reporting of possible eye movement outcomes from the eye-tracking devices substantially varied between studies.
Interpretation of Outcomes
Eye movements (saccades, smooth pursuits, fixations etc.) were generally impaired in mTBI compared to controls or baseline tests, regardless of acute or chronic mTBI status (Table 3). Yet, the influence of mTBI on specific outcomes was inconsistent. For example, several studies found deficits in saccades in people with mTBI during anti-saccadic tests (Johnson et al., 2015a,b; Balaban et al., 2016; DiCesare et al., 2017; Hoffer et al., 2017; Murray et al., 2017; Webb et al., 2018), whereas others found no differences (Wetzel et al., 2018; Cochrane et al., 2019; Kelly et al., 2019). Studies that did not find differences, however, may have been impacted by methodological issues, such as grouping all stages of mTBI together (acute/sub-acute and chronic) to make a larger cohort (Wetzel et al., 2018; Kelly et al., 2019), which limits comparison and understanding of potential deficits at different stages.
Other notable methodological limitations were found in the reviewed studies that may impact outcome interpretation. Studies examined the same eye movements but with slightly different protocols. For example, smooth pursuits were examined with a range of frequencies (0.1–1.25 Hz) and the visual stimulus (e.g., colored dots or shapes on a computer or LED board) used for eye movement tasks varied across all studies (Table 2). Although studies reported eye movement outcomes and discussed the relationships between deficits and underlying cognitive or motor impairments due to mTBI, only two studies (Maruta et al., 2010b, 2018) correlated eye movement outcomes with symptoms or other tests for these or other (e.g., age, gender, depression state etc.) relevant features. None of the reviewed articles controlled for the impact of cognition or basic visual function (visual acuity or contrast sensitivity) on eye movements, and only one study (Stuart et al., 2019b) reported basic visual function scores. Many of the studies did not assess cognition and similarly many only reported that they excluded subjects based on visual function (i.e., eye chart screening or self-reported questionnaires) but provided no scores or results to verify this (Suh et al., 2006; DiCesare et al., 2017; Murray et al., 2017; Howell et al., 2018; Webb et al., 2018; Wetzel et al., 2018; Cochrane et al., 2019; Kelly et al., 2019). None of the studies provided any information on the use of corrective eye wear by the participants during the eye-tracking assessments, with several articles reporting that subjects had “normal or corrected to normal vision,” but it was unclear if individuals with vision correction were included in reported results.
Summary of Common Study Features
In order to refine the information provided in our detailed tables (Tables 1, 2) a brief overview of the most common features of the reviewed studies is presented below;
• The majority of the reviewed studies that provided mTBI diagnostic criteria involved a clinician with experience of sports injuries.
• Included participants tended to be free of previous mTBI, musculoskeletal, cognitive, emotional, visual, or neurological issues.
• Eye-tracker sampling frequency of 500 Hz.
• Seated/static testing using computer screens to provide visual stimuli
• The most common eye-tracker metrics were based upon the latency, gain, velocity, duration, or positional error rates of the specific eye movement assessments, with the velocity of eye movements being the most prominent outcome reported across studies.
Discussion
This review examined 22 studies that reported quantified eye movements in healthy controls and mTBI subjects. We reviewed; (i) how eye-movements were measured; (ii) reported eye-movement outcomes and their definitions; and (iii) differences reported between mTBI and controls in eye-movement outcomes. Across all of the reviewed studies there was a lack of basic methodological reporting, as there was little consensus or reporting of participant mTBI classification (acute/sub-acute or chronic), demographic characteristics (age, sex, time since injury etc.) and inclusion or exclusion criteria. This limits the generalizability of results to mTBI populations and influences the reproducibility of the methods and results of the reviewed studies. Despite these limitations, this review has demonstrated that eye-movement measurement in mTBI is emerging, but further work is required to establish the validity and reliability of instrumentation and methods to derive eye-movement outcomes, as well as the nature of eye-movement impairments in mTBI.
Instruments
There is currently no “gold standard” instrument for eye-movement measurement, which is likely the reason for the numerous different instruments used in the reviewed studies. The majority of studies used static infra-red eye-tracking devices in constrained seated activities (e.g., chin rest in place in front of a computer screen), but several studies did show progression to unconstrained dynamic eye-tracking protocols (Maruta et al., 2010b, 2016; Stuart et al., 2019b). High resolution (>100 Hz) mobile eye-tracking during functional activities (e.g., walking or standing) may provide deeper understanding of the impact that subtle eye-movement deficits may have on those with mTBI.
Within the reviewed studies eye-tracking instrumentation sampling frequency substantially varied, which impacts on instrument validity. For example, accurate saccadic detection requires a minimum of 50 Hz and 200 Hz to accurately measure saccadic durations (Andersson et al., 2010; Leube et al., 2017). However several of the reviewed studies only had high enough sampling frequency (60 Hz) to detect saccades (Andersson et al., 2010) but not to accurately measure all of the reported features (Johnson et al., 2015a,b; DiCesare et al., 2017), which limits understanding of subtle deficits that may be missed as a result.
Importantly, clear evidence of the validity and reliability of instrumentation is essential for confidence in reported outcomes. We found that the reviewed studies did not adequately address this, with no studies reporting the validity or reliability of their instrumentation, and four studies inadequately reporting eye-movement outcome validation. Reporting the validity and reliability of eye-tracking instruments is advocated due to the influences of technological [e.g., parallax and calibration error (Pelz and Canosa, 2001; Nystrom et al., 2013)] and physiological [e.g., head or body movement (Zhu and Ji, 2005; Marx et al., 2012)] factors that can impact measurement. Generally, our review revealed a lack of detail regarding instrument design (e.g., binocular, monocular etc.), calibration procedures, sampling frequencies, control for artifact movement etc. To improve the quality of research in this emerging area, there is a need for reporting of the validity and reliability of instruments used to measure eye-movements in mTBI.
Outcomes
At present there are also no “gold standard” algorithms or definitions for the detection and measurement or reporting of eye-movements (Larsson et al., 2015; Stuart et al., 2019a). This may explain why many of the reviewed studies did not provide definitions for their reported eye-movement outcomes and why reported definitions lacked consensus. As a result, velocity thresholds for saccade detection varied from 20 to 240°/s, with high speeds used for more dynamic tasks (e.g., walking) to rule out the influence of vestibular-ocular reflexes on outcomes. Similarly, eye-movement assessment protocols varied between studies, with different smooth pursuit frequencies, various visual stimuli, overlap or no-overlap designs, different amplitudes of step targets, or speeds and durations of projected dot patterns. The definition of eye-movement outcomes and the protocol used to derive outcomes impacts the generalizability of findings, as valuable information may be discarded or irrelevant data included depending on the thresholds set or the stimulus used. For example, a velocity-based algorithm with a 240°/s threshold will detect saccades over ~5° (Holmqvist et al., 2011), but below this data would be classified as a fixation. However, depending on the specific aims of the study, this algorithm may not be relevant or may not provide an accurate portrayal of performance. This was evident within the reviewed studies where different frequencies of smooth pursuit examination led to studies not finding deficits in mTBI on the same testing paradigms, such as continuous tracking where Diwakar et al. (2015) found no difference at 0.4 Hz but Kelly et al. (2019) found a difference at 1.25 Hz. Creating a gold-standard for eye-movement outcome detection and measurement reporting is challenging due to the variations in instrumentation and different protocols used. Nonetheless, based on the findings within this review, we feel it is necessary for consensus to be adopted for mTBI literature. Such consensus should include the reporting of eye-movement definitions and use of standardized methods [e.g., internationally recognized anti-saccade protocol Antoniades et al., 2013 or algorithms for comprehensive smooth pursuit evaluation (Larsson et al., 2015)] for examining eye-movements.
Within the reviewed studies there was a wide range of eye-movement outcome measures reported, which highlighted the emerging and exploratory nature of eye-movement measurement in mTBI. The majority of the reviewed studies examined smooth pursuits or saccades, with few studies examining fixation and optokinetic nystagmus eye movement outcomes. Focus on smooth pursuits and saccades is likely due to the large amount of underpinning neural regions involved in performance (Ventura et al., 2016), with saccades and pursuits sharing very similar functional architecture within the central nervous system (Krauzlis, 2004). Fixations and saccades have an intimate relationship, as fixations are the pauses in between saccades (Krauzlis et al., 2017), and similarly smooth pursuits could be classified as fixations as they are pauses on moving objects, which may be the reasons why fixations tended to be overlooked within assessments in mTBI. Alternatively, nystagmus eye movements may not have been examined in many studies due to difficulties in monitoring this type of eye movement with infrared eye-trackers. For example, measurement is influenced by head position (Pettorossi et al., 2011), and electro-oculography is usually used due to accuracy and high sampling frequency requirements (Haslwanter and Clarke, 2010). In addition, stimuli that evoke sustained nystagmus eye movements commonly exacerbate mTBI-related symptoms, with reviewed studies reporting having to stop testing, as a result, which led to reduced cohort sizes for further investigation (Cochrane et al., 2019). Similarly, many of the reviewed studies had small (n < 30) mTBI cohorts and as a result the number of outcomes reported may lead to inappropriate statistical analysis or reporting due to the number of performed statistical comparisons (e.g., Type I or II statistical error; von Der Malsburg and Angele, 2017). Similar to other behavioral measures (e.g., gait; Verghese et al., 2007), use of data reduction techniques, such as principle component or factor analysis, in future studies may help to reduce the risk of inappropriate outcome reporting by developing relevant eye-movement domains or factors for further analysis.
Despite the huge number of outcome measures reported, many of the outcomes were reported in a task-dependent manner. For example, static seated eye-movement assessments tended to comprehensively report many outcomes from long testing protocols, whereas dynamic standing or walking tasks tended to report a small number of largely saccadic outcomes (e.g., saccade number, velocity, amplitude; Murray et al., 2014, 2017; Stuart et al., 2019b). This task-dependent reporting of eye-movement outcomes likely stems from the complexity of data processing and analysis with increasingly dynamic tasks (Zhu and Ji, 2005; Stuart et al., 2014b, 2019a). Unlike controlled static seated assessments, dynamic tasks introduce other factors (e.g., head movement, vestibular-ocular reflexes, lighting conditions of testing) that can impact recordings and need to be controlled for as these factors have been demonstrated to influence eye-tracking outcomes (Stuart et al., 2017). Comparison of several studies that reported saccade velocity indicated that there may be task-dependent mTBI impairments. For example, seated studies reported reduced saccadic velocity in mTBI compared to controls (Cifu et al., 2015; Cochrane et al., 2019; Kelly et al., 2019), whereas dynamic studies reported the opposite (i.e., greater saccadic velocity in mTBI; Murray et al., 2017). However, due to the limited number of studies available for review and methodological variations, definitive conclusions cannot currently be drawn. This confirms the need to appropriately quantify eye-movements in mTBI during a range of different tasks to uncover impairments that are relevant to performance of “real-life” activities.
Interpretation of Outcomes
Generally, the reviewed studies showed that saccades, smooth pursuits, fixations and nystagmus were impaired in mTBI compared to controls. However, eye-movement outcome interpretation was complicated by many methodological limitations, particularly the inconsistent or lack of reporting of basic demographic and mTBI-related information (e.g., mTBI diagnosis criteria, time since injury etc.). There is currently no universally accepted definition or diagnostic criteria for mTBI (Carroll et al., 2004; Management of Concussion/mTBI and Working Group, 2009; Mccrory et al., 2017; Eisenberg and Mannix, 2018; Voormolen et al., 2018; Chancellor et al., 2019), which is likely the reason why the majority of studies did not describe the criteria that were met or how/who provided an mTBI diagnosis. However, many of the reviewed studies also provided very little demographic information regarding their participants, with some studies not reporting basic features such as participant sex or specific time since mTBI (e.g., days, weeks, months, or years since injury). Lack of reporting accompanied by variable inclusion and exclusion criteria between studies makes the generalization across the literature difficult, and may explain some of the conflicting reports of specific eye-movement deficits (i.e., reports of anti-saccadic impairment was variable). Lack of a standardized mTBI diagnosis criteria and reporting of basic features makes interpretation of outcomes complex (King, 2019), therefore studies should report these features as fully as possible to aid understanding.
There were a vast number of outcomes being recorded and reported, which ranged from relatively standard (e.g., pro-saccades, anti-saccades, smooth pursuits etc.) to novel [e.g., eye skew (Howell et al., 2018), gaze stabilization (Murray et al., 2014), oculomotor error (Suh et al., 2006)] outcomes. Development and application of novel eye-movement outcomes may allow deficits to be uncovered that may otherwise not be found (Harezlak and Kasprowski, 2018). However, the lack of reporting on novel outcome validation and data processing in the reviewed studies limits the generalizability of results and does not allow replication in other cohorts. Our findings suggest that validity and reliability assessment of novel outcome measures should be reported alongside standardized eye-movement outcomes from traditional testing batteries, which would situate novel outcomes in the context of traditional measures to aid interpretation.
We were surprised that only two studies (Maruta et al., 2010b, 2018) assessed for cognitive function and only one study reported data on visual function (Stuart et al., 2019b), with other studies only reporting that participants had “normal or corrected to normal vision” (Suh et al., 2006; DiCesare et al., 2017; Murray et al., 2017; Howell et al., 2018; Webb et al., 2018; Wetzel et al., 2018; Cochrane et al., 2019; Kelly et al., 2019). Eye-movements are underpinned by cognitive processes (Hutton, 2008; Mele and Federici, 2012), even from an early stage before the automatic bottom-up cascade of visual processing occurs (Baluch and Itti, 2011). Cognitive function was shown to relate to eye-movement performance in mTBI in several reviewed studies (Suh et al., 2006; Maruta et al., 2010b, 2018), with cognitive deficits leading to abnormal performance on quantified eye-movement examinations. Eye-movements may therefore be a proxy for cognitive function in mTBI, but without assessment and controlling for cognitive function within eye-movement analysis these links may be missed (Stuart et al., 2014a). Similarly, impairments in basic visual functions, such as visual acuity and contrast sensitivity, have been found to lead to abnormal eye-movement performance (Williams et al., 1995; Gottlob et al., 1996; Palidis et al., 2017). Visual acuity deficits can be corrected with prescription glasses or contact lenses (Sloan, 1951), however the reviewed studies provided no details regarding whether participants used visual correction during testing. This is important as visual correction, through glasses and contact lenses, can impact infrared eye-tracking due to the refraction of infrared light that is used to detect the location of the participants pupil, which would lead to inaccurate tracking and lost data collection ability (Stuart et al., 2014b, 2016; Fuhl et al., 2016). Age (Munoz et al., 1998), depression (Emslie et al., 1990), and medication use [e.g., opioids (Grace et al., 2010)] have also been implicated in eye-movement performance in various populations. Therefore, the measurement and reporting of basic demographic features, cognitive and visual function is required when investigating eye-movements in mTBI.
Test Protocols
Previous eye-tracking studies have generally involved static seated eye-movement assessment tasks (Pelz and Canosa, 2001; Maruta et al., 2010a), which have provided valuable information regarding potential deficits in mTBI compared to controls. However, while these experiments allow for complete experimental control, they lack functional validity because eye-movements in the real-world are goal-oriented (Salverda et al., 2011) and often occur during co-ordination of multiple motor, cognitive and visual processes (Hayhoe et al., 2003; Hayhoe and Ballard, 2005; Ventura et al., 2016). Although we found that segmentation of individual eye-movement features revealed some eye-movement impairments in mTBI, inconsistencies in reported deficits may be due to compensatory mechanisms (i.e., using additional attentional resources to improve performance of eye-movement tasks) similar to those found in aging research (Wiegand et al., 2014). Whereas, when individuals are required to complete complex real-world tasks (e.g., walking, turning, balancing etc.), that simultaneously involve motor, cognitive and visual processes, deficits may become prominent as they may be unable to compensate, consistent with results from the dual-task literature in mTBI (Cicerone, 1996; Howell et al., 2013). Future studies should therefore robustly examine eye-movements within mTBI during a range of static and dynamic tasks to further understand the functional impact of deficits.
Many previous studies of eye-movements in mTBI have incorporated subjective, clinical assessments that can be performed is any environment, such as the VOMS (Mucha et al., 2014) or King-Devick reading test (Leong et al., 2015; Galetta et al., 2016; Walsh et al., 2016). These clinical tests provide valuable information concerning the aggravation of mTBI-related symptoms when performing eye-movements (Capó-Aponte et al., 2018), but provide limited quantifiable information to measure deficits or monitor recovery. These subjective or symptom-based eye-movement examinations provide only global performance measures and symptom scores following an mTBI, but results cannot highlight subtle changes in performance and may be limited due to the reliance on self-report by those with mTBI (Lovell et al., 2002; Heitger et al., 2007). In contrast, the 22 studies included in this review examined eye-movements using quantitative eye-tracking technology that can provide information related to subtle changes in eye-movements, and link them to specific mechanisms behind impairments through experimental manipulation. Future adoption of quantitative eye-tracking technologies within clinical practice will allow eye-movement examination to become standardized and may detect deficits that could be missed with traditional clinical techniques.
Conclusion
Aspects of eye-movements are impaired following an mTBI, as demonstrated by the reviewed studies that quantified impairments in either saccadic, smooth pursuit, fixation or nystagmus eye movements. While there is evidence from these studies, it is not yet strong enough to adopt quantitative eye-movement assessment within clinical practice. This review has highlighted that methodological issues across the current literature limit the understanding and generalizability of the reported findings in mTBI. There is a need for consensus on methods used and reporting of eye movement data, including eye-movement instruments, data collection procedures, processing algorithms and analysis methods are required. Comprehensive reporting of demographics, mTBI-related features, and confounding variables are also recommended for future work in this area (Table 4). Development and implementation of a standardized approach to quantitative eye-movement examination will ensure accurate and appropriate data interpretation. This will allow robust evidence to be established that can be implemented in future clinical practice.
Author Contributions
SS wrote the first draft and revisions of the manuscript. SS, LP, and DM designed the search strategy and conducted the literature search. SS and LK designed, implemented, and oversaw the topic area. SS, LP, DM, RP, JC, and LK were involved in interpretation of the data, writing the manuscript and revisions, final approval of the version to be published, and agree to be accountable for all aspects of the work.
Funding
This work was supported by the Assistant Secretary of Defense for Health Affairs (Award No. W81XWH-15-1-0620, W81XWH-17-1-0424). Opinions, interpretations, conclusions, and recommendations are those of the author and are not necessarily endorsed by the Department of Defense. SS was supported in part by a Postdoctoral Fellowship from the Parkinson's Foundation (Grant Number PF-FBS-1898).
Conflict of Interest
The authors declare that the research was conducted in the absence of any commercial or financial relationships that could be construed as a potential conflict of interest.
Supplementary Material
The Supplementary Material for this article can be found online at: https://www.frontiersin.org/articles/10.3389/fspor.2020.00005/full#supplementary-material
References
Akhand, O., Rizzo, J. R., Rucker, J. C., Hasanaj, L., Galetta, S. L., and Balcer, L. J. (2019). History and future directions of vision testing in head trauma. J. Neuroophthalmol. 39, 68–81. doi: 10.1097/WNO.0000000000000726
Alla, S., Sullivan, S. J., Hale, L., and Mccrory, P. (2009). Self-report scales/checklists for the measurement of concussion symptoms: a systematic review. Br. J. Sports Med. 43:i3. doi: 10.1136/bjsm.2009.058339
Andersson, R., Nyström, M., and Holmqvist, K. (2010). Sampling frequency and eye-tracking measures: how speed affects durations, latencies, and more. J. Eye Mov. Res. 3, 1–12. doi: 10.16910/jemr.3.3.6
Antoniades, C., Ettinger, U., Gaymard, B., Gilchrist, I., Kristjánsson, A., Kennard, C., et al. (2013). An internationally standardised antisaccade protocol. Vis. Res. 84, 1–5. doi: 10.1016/j.visres.2013.02.007
Balaban, C., Hoffer, M. E., Szczupak, M., Snapp, H., Crawford, J., Murphy, S., et al. (2016). Oculomotor, vestibular, and reaction time tests in mild traumatic brain injury. PLoS ONE 11:e0162168. doi: 10.1371/journal.pone.0162168
Baluch, F., and Itti, L. (2011). Mechanisms of top-down attention. Trends Neurosci. 34, 210–224. doi: 10.1016/j.tins.2011.02.003
Capó-Aponte, J. E., Beltran, T. A., Walsh, D. V., Cole, W. R., and Dumayas, J. Y. (2018). Validation of visual objective biomarkers for acute concussion. Mil. Med. 183, 9–17. doi: 10.1093/milmed/usx166
Carroll, L., Cassidy, J., Holm, L., Kraus, J., and Coronado, V. (2004). WHO collaborating centre task force on mild traumatic brain, injury. methodological issues and research recommendations for mild traumatic brain injury: the WHO collaborating centre task force on mild traumatic brain injury. J. Rehabil. Med. 43, 113–125. doi: 10.1080/16501960410023877
Catz, N., and Thier, P. (2007). “Neural control of saccadic eye movements,” in Neuro-Ophthalmology Dev Ophthalmol, Vol. 40, eds A. Straube and U. Büttner (Basel: Karger Publishers), 52–75. doi: 10.1159/000100349
Chancellor, S. E., Franz, E. S., Minaeva, O. V., and Goldstein, L. E. (2019). Pathophysiology of concussion. Seminars Pediatric Neurol. Elsevier 30, 14–25. doi: 10.1016/j.spen.2019.03.004
Cicerone, K. D. (1996). Attention deficits and dual task demands after mild traumatic brain injury. Brain Inj. 10, 79–90. doi: 10.1080/026990596124566
Cifu, D. X., Wares, J. R., Hoke, K. W., Wetzel, P. A., Gitchel, G., and Carne, W. (2015). Differential eye movements in mild traumatic brain injury versus normal controls. J. Head Trauma Rehabil. 30, 21–28. doi: 10.1097/HTR.0000000000000036
Ciuffreda, K. J., Kapoor, N., Rutner, D., Suchoff, I. B., Han, M. E., and Craig, S. (2007). Occurrence of oculomotor dysfunctions in acquired brain injury: a retrospective analysis. Optometry 78, 155–161. doi: 10.1016/j.optm.2006.11.011
Cochrane, G. D., Christy, J. B., Almutairi, A., Busettini, C., Swanson, M. W., and Weise, K. K. (2019). Visuo-oculomotor function and reaction times in athletes with and without concussion. Opt. Vis. Sci. 96, 256–265. doi: 10.1097/OPX.0000000000001364
Cockerham, G. C., Goodrich, G. L., Weichel, E. D., Orcutt, J. C., Rizzo, J. F., Bower, K. S., et al. (2009). Eye and visual function in traumatic brain injury. J. Rehabil. Res. Dev. 46, 811–818. doi: 10.1682/jrrd.2008.08.0109
Contreras, R., Ghajar, J., Bahar, S., and Suh, M. (2011). Effect of cognitive load on eye-target synchronization during smooth pursuit eye movement. Brain Res. 1398, 55–63. doi: 10.1016/j.brainres.2011.05.004
Deubel, H., and Schneider, W. X. (2003). Delayed saccades, but not delayed manual aiming movements, require visual attention shifts. Ann. N. Y. Acad. Sci. 1004, 289–296. doi: 10.1196/annals.1303.026
DiCesare, C. A., Kiefer, A. W., Nalepka, P., and Myer, G. D. (2017). Quantification and analysis of saccadic and smooth pursuit eye movements and fixations to detect oculomotor deficits. Behav. Res. Methods 49, 258–266. doi: 10.3758/s13428-015-0693-x
Dieterich, M., Müller-Schunk, S., Stephan, T., Bense, S., Seelos, K., and Yousry, T. A. (2009). Functional magnetic resonance imaging activations of cortical eye fields during saccades, smooth pursuit, and optokinetic nystagmus. Ann. N. Y. Acad. Sci. 1164, 282–292. doi: 10.1111/j.1749-6632.2008.03718.x
Diwakar, M., Harrington, D. L., Maruta, J., Ghajar, J., El-Gabalawy, F., Muzzatti, L., et al. (2015). Filling in the gaps: anticipatory control of eye movements in chronic mild traumatic brain injury. Neuroimage 8, 210–223. doi: 10.1016/j.nicl.2015.04.011
Duchowski, A. T. (2007). “Eye tracking methodology,” in Theory and Practice, Vol. 328 (Cham: Springer), 2–3. doi: 10.1007/978-3-319-57883-5
Eierud, C., Craddock, R. C., Fletcher, S., Aulakh, M., King-Casas, B., Kuehl, D., et al. (2014). Neuroimaging after mild traumatic brain injury: review and meta-analysis. Neuroimage 4, 283–294. doi: 10.1016/j.nicl.2013.12.009
Eisenberg, M., and Mannix, R. (2018). Acute concussion: making the diagnosis and state of the art management. Curr. Opin. Pediatr. 30, 344–349. doi: 10.1097/MOP.0000000000000620
Emslie, G. J., Rush, A. J., Weinberg, W. A., Rintelmann, J. W., and Roffwarg, H. P. (1990). Children with major depression show reduced rapid eye movement latencies. Arch. Gen. Psychiatry 47, 119–124. doi: 10.1001/archpsyc.1990.01810140019003
Fuhl, W., Tonsen, M., Bulling, A., and Kasneci, E. (2016). Pupil detection for head-mounted eye tracking in the wild: an evaluation of the state of the art. Mach. Vis. Appl. 27, 1275–1288. doi: 10.1007/s00138-016-0776-4
Galetta, K. M., Liu, M., Leong, D. F., Ventura, R. E., Galetta, S. L., and Balcer, L. J. (2016). The king-devick test of rapid number naming for concussion detection: meta-analysis and systematic review of the literature. Concussion 1:CNC8. doi: 10.2217/cnc.15.8
Gottlob, I., Wizov, S. S., and Reinecke, R. D. (1996). Head and eye movements in children with low vision. Graefe's Arch. Clin. Exper. Ophthalmol. 234, 369–377. doi: 10.1007/bf00190713
Grace, P. M., Stanford, T., Gentgall, M., and Rolan, P. E. (2010). Utility of saccadic eye movement analysis as an objective biomarker to detect the sedative interaction between opioids and sleep deprivation in opioid-naive and opioid-tolerant populations. J. Psychopharmacol. 24, 1631–1640. doi: 10.1177/0269881109352704
Harezlak, K., and Kasprowski, P. (2018). Application of eye tracking in medicine: a survey, research issues and challenges. Comput. Med. Imag. Graph. 65, 176–190. doi: 10.1016/j.compmedimag.2017.04.006
Haslwanter, T., and Clarke, A. H. (2010). Chapter 5 - Eye Movement Measurement: Electro-Oculography and Video-Oculography. Handbook of Clinical Neurophysiology. Oxford, UK: Elsevier. doi: 10.1016/S1567-4231(10)09005-2
Hayhoe, M., and Ballard, D. (2005). Eye movements in natural behavior. Trends Cogn. Sci. 9, 188–194. doi: 10.1016/j.tics.2005.02.009
Hayhoe, M. M., Shrivastava, A., Mruczek, R., and Pelz, J. B. (2003). Visual memory and motor planning in a natural task. J. Vis. 3, 49–63. doi: 10.1167/3.1.6
Hecimovich, M., King, D., Dempsey, A., Gittins, M., and Murphy, M. (2019). In situ use of the king-devick eye tracking test and changes seen with sport-related concussion: saccadic and blinks counts. Phys. Sportsmed. 47, 78–84. doi: 10.1080/00913847.2018.1525261
Heitger, M. H., Jones, R. D., Frampton, C. M., Ardagh, M. W., and Anderson, T. J. (2007). Recovery in the first year after mild head injury: divergence of symptom status and self-perceived quality of life. J. Rehabil. Med. 39, 612–621. doi: 10.2340/16501977-0100
Heitger, M. H., Jones, R. D., Macleod, A. D., Snell, D. L., Frampton, C. M., and Anderson, T. J. (2009). Impaired eye movements in post-concussion syndrome indicate suboptimal brain function beyond the influence of depression, malingering or intellectual ability. Brain 132, 2850–2870. doi: 10.1093/brain/awp181
Herishanu, Y. O. (1992). Abnormal cancellation of the vestibulo-ocular reflex (VOR) after mild head and/or neck trauma. Neuro-Ophthalmol. 12, 237–240. doi: 10.3109/01658109209058144
Hoffer, M. E., Balaban, C., Szczupak, M., Buskirk, J., Snapp, H., Crawford, J., et al. (2017). The use of oculomotor, vestibular, and reaction time tests to assess mild traumatic brain injury (mTBI) over time. Laryngoscope Investigative Otolaryngol. 2, 157–165. doi: 10.1002/lio2.74
Holmqvist, K., Nyström, M., Andersson, R., Dewhurst, R., Jarodzka, H., and Van De Weijer, J. (2011). Eye Tracking: A Comprehensive Guide to Methods and Measures. Oxford, UK: OUP Oxford.
Howell, D. R., Brilliant, A. N., Storey, E. P., Podolak, O. E., Meehan, W. P., and Master, C. L. (2018). Objective eye tracking deficits following concussion for youth seen in a sports medicine setting. J. Child Neurol. 33, 794–800. doi: 10.1177/0883073818789320
Howell, D. R., Osternig, L. R., and Chou, L.-S. (2013). Dual-task effect on gait balance control in adolescents with concussion. Arch. Phys. Med. Rehabil. 94, 1513–1520. doi: 10.1016/j.apmr.2013.04.015
Hunt, A. W., Mah, K., Reed, N., Engel, L., and Keightley, M. (2016). Oculomotor-based vision assessment in mild traumatic brain injury: a systematic review. J. Head Trauma Rehabil. 31, 252–261. doi: 10.1097/HTR.0000000000000174
Hutton, S. B. (2008). Cognitive control of saccadic eye movements. Brain Cogn. 68, 327–340. doi: 10.1016/j.bandc.2008.08.021
Jeter, C. B., Hergenroeder, G. W., Hylin, M. J., Redell, J. B., Moore, A. N., and Dash, P. K. (2013). Biomarkers for the diagnosis and prognosis of mild traumatic brain injury/concussion. J. Neurotrauma 30, 657–670. doi: 10.1089/neu.2012.2439
Johansson, J., Nygren De Boussard, C., Öqvist Seimyr, G., and Pansell, T. (2017). The effect of spectacle treatment in patients with mild traumatic brain injury: a pilot study. Clin. Exper. Optomet. 100, 234–242. doi: 10.1111/cxo.12458
Johnson, B., Hallett, M., and Slobounov, S. (2015a). Follow-up evaluation of oculomotor performance with fMRI in the subacute phase of concussion. Neurology 85, 1163–1166. doi: 10.1212/WNL.0000000000001968
Johnson, B., Zhang, K., Hallett, M., and Slobounov, S. (2015b). Functional neuroimaging of acute oculomotor deficits in concussed athletes. Brain Imag. Behav. 9, 564–573. doi: 10.1007/s11682-014-9316-x
Kaldoja, M. L., Saard, M., Lange, K., Raud, T., Teeveer, O. K., and Kolk, A. (2015). Neuropsychological benefits of computer-assisted cognitive rehabilitation (using FORAMENRehab program) in children with mild traumatic brain injury or partial epilepsy: a pilot study. J. Pediatr. Rehabil. Med. 8, 271–283. doi: 10.3233/PRM-150346
Kelly, K. M., Kiderman, A., Akhavan, S., Quigley, M. R., Snell, E. D., Happ, E., et al. (2019). Oculomotor, vestibular, and reaction time effects of sports-related concussion: video-oculography in assessing sports-related concussion. J. Head Trauma Rehabil. 34, 176–188. doi: 10.1097/HTR.0000000000000437
Kim, H. J., Tsao, J. W., and Stanfill, A. G. (2018). The current state of biomarkers of mild traumatic brain injury. JCI Insight 3:97105. doi: 10.1172/jci.insight.97105
King, N. S. (2019). Mild traumatic brain injury and sport-related concussion: different languages and mixed messages? Brain Inj. 33, 1556–1563. doi: 10.1080/02699052.2019.1655794
Konen, C. S., Kleiser, R., Seitz, R. J., and Bremmer, F. (2005). An fMRI study of optokinetic nystagmus and smooth-pursuit eye movements in humans. Exper. Brain Res. 165, 203–216. doi: 10.1007/s00221-005-2289-7
Krauzlis, R. J. (2004). Recasting the smooth pursuit eye movement system. J. Neurophysiol. 91, 591–603. doi: 10.1152/jn.00801.2003
Krauzlis, R. J., Goffart, L., and Hafed, Z. M. (2017). Neuronal control of fixation and fixational eye movements. Philos. Trans. Royal Soc. Lond. Series B Biol. Sci. 372:20160205. doi: 10.1098/rstb.2016.0205
Land, M. F. (2006). Eye movements and the control of actions in everyday life. Prog. Retin. Eye Res. 25, 296–324. doi: 10.1016/j.preteyeres.2006.01.002
Lara, F., Cacho, P., García, A., and Megías, R. (2001). General binocular disorders: prevalence in a clinic population. Ophthalmic Physiol. Opt. 21, 70–74. doi: 10.1046/j.1475-1313.2001.00540.x
Larsson, L., Nyström, M., Andersson, R., and Stridh, M. (2015). Detection of fixations and smooth pursuit movements in high-speed eye-tracking data. Biomed. Signal Process. Control 18, 145–152. doi: 10.1016/j.bspc.2014.12.008
Leong, D. F., Balcer, L. J., Galetta, S. L., Evans, G., Gimre, M., and Watt, D. (2015). The king–devick test for sideline concussion screening in collegiate football. J. Optom. 8, 131–139. doi: 10.1016/j.optom.2014.12.005
Leube, A., Rifai, K., and Wahl, S. (2017). Sampling rate influences saccade detection in mobile eye tracking of a reading task. J. Eye Mov. Res. 10:3. doi: 10.16910/jemr.10.3.3
Liversedge, S. P., and Findlay, J. M. (2000). Saccadic eye movements and cognition. Trends Cogn. Sci. 4, 6–14. doi: 10.1016/s1364-6613(99)01418-7
Lovell, M., Collins, M., Maroon, J., Cantu, R., Hawn, M., Burke, C., et al. (2002). Inaccuracy of symptom reporting following concussion in athletes. Med. Sci. Sports Exerc. 34:S298. doi: 10.1097/00005768-200205001-01680
Management of Concussion/mTBI and Working Group (2009). VA/DoD clinical practice guideline for management of concussion/mild traumatic brain injury. J. Rehabi. Res. Dev. 46, CP1–CP68. doi: 10.1682/JRRD.2009.06.0076
Marigold, D. S., and Patla, A. E. (2008). Visual information from the lower visual field is important for walking across multi-surface terrain. Exper. Brain Res. 188, 23–31. doi: 10.1007/s00221-008-1335-7
Maruta, J., Jaw, E., Modera, P., Rajashekar, U., Spielman, L. A., and Ghajar, J. (2017). Frequency responses to visual tracking stimuli may be affected by concussion. Mil. Med. 182, 120–123. doi: 10.7205/MILMED-D-16-00093
Maruta, J., Lee, S. W., Jacobs, E. F., and Ghajar, J. (2010a). A unified science of concussion. Ann. N. Y. Acad. Sci. 1208, 58–66. doi: 10.1111/j.1749-6632.2010.05695.x
Maruta, J., Spielman, L. A., Rajashekar, U., and Ghajar, J. (2018). Association of visual tracking metrics with post-concussion symptomatology. Front. Neurol. 9:611. doi: 10.3389/fneur.2018.00611
Maruta, J., Spielman, L. A., Yarusi, B. B., Wang, Y., Silver, J. M., and Ghajar, J. (2016). Chronic post-concussion neurocognitive deficits. II. relationship with persistent symptoms. Front. Human Neurosci. 10:45. doi: 10.3389/fnhum.2016.00045
Maruta, J., Suh, M., Niogi, S. N., Mukherjee, P., and Ghajar, J. (2010b). Visual tracking synchronization as a metric for concussion screening. J. Head Trauma Rehabil. 25, 293–305. doi: 10.1097/HTR.0b013e3181e67936
Marx, S., Respondek, G., Stamelou, M., Dowiasch, S., Stoll, J., Bremmer, F., et al. (2012). Validation of mobile eye-tracking as novel and efficient means for differentiating progressive supranuclear palsy from Parkinson's disease. Front. Behav. Neurosci. 6:88. doi: 10.3389/fnbeh.2012.00088
Mays, L. E., Porter, J. D., Gamlin, P., and Tello, C. A. (1986). Neural control of vergence eye movements: neurons encoding vergence velocity. J. Neurophysiol. 56, 1007–1021. doi: 10.1152/jn.1986.56.4.1007
McCrea, M., Broshek, D. K., and Barth, J. T. (2015). Sports concussion assessment and management: future research directions. Brain Inj. 29, 276–282. doi: 10.3109/02699052.2014.965216
Mccrory, P., Meeuwisse, W., Dvorák, J., Aubry, M., Bailes, J., Broglio, S., et al. (2017). Consensus statement on concussion in sport—the 5th international conference on concussion in sport held in Berlin, october 2016. Br. J. Sports Med. 51, 838–847. doi: 10.1136/bjsports-2017-097699
McPeek, R. M., Skavenski, A. A., and Nakayama, K. (2000). Concurrent processing of saccades in visual search. Vis. Res. 40, 2499–2516. doi: 10.1016/s0042-6989(00)00102-4
McPherson„, A. L, Nagai, T., Webster, K. E., and Hewett, T. E. (2019). Musculoskeletal injury risk after sport-related concussion: a systematic review and meta-analysis. Am. J. Sports Med. 47, 1754–1762. doi: 10.1177/0363546518785901
Meier, T. B., Brummel, B. J., Singh, R., Nerio, C. J., Polanski, D. W., and Bellgowan, P. S. (2015). The underreporting of self-reported symptoms following sports-related concussion. J. Sci. Med. Sport 18, 507–511. doi: 10.1016/j.jsams.2014.07.008
Mele, M. L., and Federici, S. (2012). Gaze and eye-tracking solutions for psychological research. Cogn. Process. 13, 261–265. doi: 10.1007/s10339-012-0499-z
Moher, D., Liberati, A., Tetzlaff, J., Altman, D. G., and Group, T. P. (2009). Preferred reporting items for systematic reviews and meta-analyses: The PRISMA statement. PLoS Med. 6:e1000097. doi: 10.1371/journal.pmed.1000097
Mucha, A., Collins, M. W., Elbin, R. J., Furman, J. M., Troutman-Enseki, C., Dewolf, R. M., et al. (2014). A brief vestibular/ocular motor screening (VOMS) assessment to evaluate concussions: preliminary findings. Am. J. Sports Med. 42, 2479–2486. doi: 10.1177/0363546514543775
Munoz, D. P., Broughton, J. R., Goldring, J. E., and Armstrong, I. T. (1998). Age-related performance of human subjects on saccadic eye movement tasks. Exper. Brain Res. 121, 391–400. doi: 10.1007/s002210050473
Murray, N. G., Ambati, V., Contreras, M. M., Salvatore, A. P., and Reed-Jones, R. J. (2014). Assessment of oculomotor control and balance post-concussion: a preliminary study for a novel approach to concussion management. Brain Inj. 28, 496–503. doi: 10.3109/02699052.2014.887144
Murray, N. G., D'amico, N. R., Powell, D., Mormile, M. E., Grimes, K. E., Munkasy, B. A., et al. (2017). ASB clinical biomechanics award winner 2016: assessment of gaze stability within 24–48 hours post-concussion. Clin. Biomech. 44, 21–27. doi: 10.1016/j.clinbiomech.2017.03.002
Noda, H. (1991). Cerebellar control of saccadic eye movements: its neural mechanisms and pathways. Jpn. J. Physiol. 41, 351–368. doi: 10.2170/jjphysiol.41.351
Nyström, M., Andersson, R., Holmqvist, K., and Van De Weijer, J. (2013). The influence of calibration method and eye physiology on eyetracking data quality. Behav. Res. Methods 45, 272–288. doi: 10.3758/s13428-012-0247-4
Palidis, D. J., Wyder-Hodge, P. A., Fooken, J., and Spering, M. (2017). Distinct eye movement patterns enhance dynamic visual acuity. PLoS ONE 12:e0172061. doi: 10.1371/journal.pone.0172061
Pelz, J. B., and Canosa, R. (2001). Oculomotor behavior and perceptual strategies in complex tasks. Vis. Res. 41, 3587–3596. doi: 10.1016/s0042-6989(01)00245-0
Pettorossi, V., Ferraresi, A., Botti, F. M., Panichi, R., and Barmack, N. H. (2011). Head position modulates optokinetic nystagmus. Exper. Brain Res. 213, 141–152. doi: 10.1007/s00221-011-2785-x
Porterfield, W. (1752). An Essay Concerning the Motions of our Eyes, Part II. Medical Essays. Edinburgh: Hamilton, Balfour and Neill.
Quinones-Ossa, G., Padilla-Zambrano, H., Pal, R., Ghosh, A., Moscote-Salazar, L., Kumar, V. K., et al. (2019). Biomarkers in acute brain trauma: a narrative review. J. Acute Dis. 8:1. doi: 10.4103/2221-6189.250370
Reneker, J. C., Babl, R., and Flowers, M. M. (2019). History of concussion and risk of subsequent injury in athletes and service members: a systematic review and meta-analysis. Musculoskeletal Sci. Pract. 42, 173–185. doi: 10.1016/j.msksp.2019.04.004
Rizzo, J. R., Hudson, T. E., Dai, W., Birkemeier, J., Pasculli, R. M., Selesnick, I., et al. (2016). Rapid number naming in chronic concussion: eye movements in the king–devick test. Ann. Clin. Transl. Neurol. 3, 801–811. doi: 10.1002/acn3.345
Salverda, A. P., Brown, M., and Tanenhaus, M. K. (2011). A goal-based perspective on eye movements in visual world studies. Acta Psychol. 137, 172–180. doi: 10.1016/j.actpsy.2010.09.010
Samadani, U., Ritlop, R., Reyes, M., Nehrbass, E., Li, M., Lamm, E., et al. (2015). Eye tracking detects disconjugate eye movements associated with structural traumatic brain injury and concussion. J. Neurotrauma 32, 548–556. doi: 10.1089/neu.2014.3687
Shinoda, Y., Takahashi, M., and Sugiuchi, Y. (2019). Brainstem neural circuits for fixation and generation of saccadic eye movements. Prog. Brain Res. 249, 95–104. doi: 10.1016/bs.pbr.2019.04.007
Sloan, L. L. (1951). Measurement of visual acuity: a critical review. AMA Arch. Ophthalmol. 45, 704–725. doi: 10.1001/archopht.1951.01700010719013
Snegireva, N., Derman, W., Patricios, J., and Welman, K. E. (2018). Eye tracking technology in sports-related concussion: a systematic review and meta-analysis. Physiol. Meas. 39:12TR01. doi: 10.1088/1361-6579/aaef44
Snegireva, N., Derman, W., Patricios, J., and Welman, K. E. (2019). Awareness and perceived value of eye tracking technology for concussion assessment among sports medicine clinicians: a multinational study. Phys. Sportsmed. 1, 1–8. doi: 10.1080/00913847.2019.1645577
Stuart, S., Alcock, L., Galna, B., Lord, S., and Rochester, L. (2014a). The measurement of visual sampling during real-world activity in Parkinson's disease and healthy controls: a structured literature review. J. Neurosci. Methods 222, 175–188. doi: 10.1016/j.jneumeth.2013.11.018
Stuart, S., Alcock, L., Godfrey, A., Lord, S., Rochester, L., and Galna, B. (2016). Accuracy and re-test reliability of mobile eye-tracking in Parkinson's disease and older adults. Med. Eng. Phys. 38, 308–315. doi: 10.1016/j.medengphy.2015.12.001
Stuart, S., Galna, B., Lord, S., Rochester, L., and Godfrey, A. (2014b). “Quantifying saccades while walking: validity of a novel velocity-based algorithm for mobile eye tracking,” in 2014 36th Annual International Conference of the IEEE Engineering in Medicine and Biology Society. Chicago, IL: IEEE, 5739–5742.
Stuart, S., Hickey, A., Galna, B., Lord, S., Rochester, L., and Godfrey, A. (2017). iTrack: instrumented mobile electrooculography (EOG) eye-tracking in older adults and Parkinson's disease. Physiol. Meas. 38, N16–N31. doi: 10.1088/1361-6579/38/1/N16
Stuart, S., Hickey, A., Vitorio, R., Welman, K., Foo, S., Keen, D., et al. (2019a). Eye-tracker algorithms to detect saccades during static and dynamic tasks: a structured review. Physiol Meas. 40:02tr01. doi: 10.1088/1361-6579/ab02ab
Stuart, S., Parrington, L., Martini, D., Popa, B., Fino, P. C., and King, L. A. (2019b). Validation of a velocity-based algorithm to quantify saccades during walking and turning in mild traumatic brain injury and healthy controls. Physiol. Meas. 40:044006. doi: 10.1088/1361-6579/ab159d
Suh, M., Basu, S., Kolster, R., Sarkar, R., Mccandliss, B., and Ghajar, J. (2006). Increased oculomotor deficits during target blanking as an indicator of mild traumatic brain injury. Neurosci. Lett. 410, 203–207. doi: 10.1016/j.neulet.2006.10.001
Tatler, B. W. (2009). Current understanding of eye guidance. Vis. Cogn. 17, 777–789. doi: 10.1080/13506280902869213
Tatler, B. W., and Wade, N. J. (2003). On nystagmus, saccades, and fixations. Perception 32, 167–184. doi: 10.1068/p3395
Thiagarajan, P., Ciuffreda, K. J., and Ludlam, D. P. (2011). Vergence dysfunction in mild traumatic brain injury (mTBI): a review. Ophthal. Physiol. Optics 31, 456–468. doi: 10.1111/j.1475-1313.2011.00831.x
Vakil, E., Aviv, O., Mishael, M., Schwizer Ashkenazi, S., and Sacher, Y. (2019). Direct and indirect measures of context in patients with mild-to-severe traumatic brain injury (TBI): the additive contribution of eye tracking. J. Clin. Exp. Neuropsychol. 41, 644–652. doi: 10.1080/13803395.2019.1604946
Ventura, R. E., Balcer, L. J., Galetta, S. L., and Rucker, J. C. (2016). Ocular motor assessment in concussion: current status and future directions. J. Neurol. Sci. 361, 79–86. doi: 10.1016/j.jns.2015.12.010
Ventura, R. E., Jancuska, J. M., Balcer, L. J., and Galetta, S. L. (2015). Diagnostic tests for concussion: is vision part of the puzzle? J. Neuro-Ophthalmol. 35:73–81. doi: 10.1097/WNO.0000000000000223
Verghese, J., Wang, C., Lipton, R. B., Holtzer, R., and Xue, X. (2007). Quantitative gait dysfunction and risk of cognitive decline and dementia. J. Neurol. Neurosurg. Psychiatr. 78, 929–935. doi: 10.1136/jnnp.2006.106914
von Der Malsburg, T., and Angele, B. (2017). False positives and other statistical errors in standard analyses of eye movements in reading. J. Mem. Lang. 94, 119–133. doi: 10.1016/j.jml.2016.10.003
Voormolen, D. C., Cnossen, M. C., Polinder, S., Von Steinbuechel, N., Vos, P. E., and Haagsma, J. A. (2018). Divergent classification methods of post-concussion syndrome after mild traumatic brain injury: prevalence rates, risk factors, and functional outcome. J. Neurotrauma 35, 1233–1241. doi: 10.1089/neu.2017.5257
Walsh, D. V., Capó-Aponte, J. E., Beltran, T., Cole, W. R., Ballard, A., and Dumayas, J. Y. (2016). Assessment of the king-devick®(KD) test for screening acute mTBI/concussion in warfighters. J. Neurol. Sci. 370, 305–309. doi: 10.1016/j.jns.2016.09.014
Webb, B., Humphreys, D., and Heath, M. (2018). Oculomotor executive dysfunction during the early and later stages of sport-related concussion recovery. J. Neurotrauma 35, 1874–1881. doi: 10.1089/neu.2018.5673
Wetzel, P. A., Lindblad, A. S., Raizada, H., James, N., Mulatya, C., Kannan, M. A., et al. (2018). Eye tracking results in postconcussive syndrome versus normative participants. Investigat. Ophthalmol. Vis. Sci. 59, 4011–4019. doi: 10.1167/iovs.18-23815
Whitney, S. L., and Sparto, P. J. (2019). Eye movements, dizziness, and mild traumatic brain injury (mTBI): a topical review of emerging evidence and screening measures. J. Neurol. Phys. Ther. 43, S31–S36. doi: 10.1097/NPT.0000000000000272
Wiegand, I., Töllner, T., Dyrholm, M., Müller, H. J., Bundesen, C., and Finke, K. (2014). Neural correlates of age-related decline and compensation in visual attention capacity. Neurobiol. Aging 35, 2161–2173. doi: 10.1016/j.neurobiolaging.2014.02.023
Williams, M. C., May, J. G., Solman, R., and Zhou, H. (1995). The effects of spatial filtering and contrast reduction on visual search times in good and poor readers. Vis. Res. 35, 285–291. doi: 10.1016/0042-6989(94)00140-h
Keywords: mild traumatic brain injury, eye-tracking, eye movement, methods, vision
Citation: Stuart S, Parrington L, Martini D, Peterka R, Chesnutt J and King L (2020) The Measurement of Eye Movements in Mild Traumatic Brain Injury: A Structured Review of an Emerging Area. Front. Sports Act. Living 2:5. doi: 10.3389/fspor.2020.00005
Received: 04 October 2019; Accepted: 08 January 2020;
Published: 28 January 2020.
Edited by:
Fabio Augusto Barbieri, State University Paulista Júlio de Mesquita Filho, BrazilReviewed by:
Sérgio Tosi Rodrigues, Universidade Estadual Paulista Júlio de Mesquita Filho (UNESP), BrazilChesney Elizabeth Craig, Manchester Metropolitan University, United Kingdom
Copyright © 2020 Stuart, Parrington, Martini, Peterka, Chesnutt and King. This is an open-access article distributed under the terms of the Creative Commons Attribution License (CC BY). The use, distribution or reproduction in other forums is permitted, provided the original author(s) and the copyright owner(s) are credited and that the original publication in this journal is cited, in accordance with accepted academic practice. No use, distribution or reproduction is permitted which does not comply with these terms.
*Correspondence: Samuel Stuart, c2FtLnN0dWFydCYjeDAwMDQwO25vcnRodW1icmlhLmFjLnVr