- 1Faculty of Sport Sciences, University of Nantes, Nantes, France
- 2School of Physical Therapy and Rehabilitation, IFM3R, Saint-Sébastien sur Loire, France
- 3Motion Analysis Lab, Physiotherapy and Sports Medicine Department, Swiss Olympic Medical Center, La Tour Hospital, Meyrin, Switzerland
The foot is a complex system with multiple degrees of freedom that play an essential role in running or sprinting. The intrinsic foot muscles (IFM) are the main local stabilizers of the foot and are part of the active and neural subsystems that constitute the foot core. These muscles lengthen eccentrically during the stance phase of running before shortening at the propulsion phase, as the arch recoils in parallel to the plantar fascia. They play a key role in supporting the medial longitudinal arch, providing flexibility, stability and shock absorption to the foot, whilst partially controlling pronation. Much of the foot rigidity in late stance has been attributed to the windlass mechanism – the dorsiflexion of the toes building tension up in the plantar aponeurosis and stiffening the foot. In addition, recent studies have shown that the IFM provide a necessary active contribution in late stance, in order to develop sufficient impedance in the metatarsal-phalangeal joints. This in turn facilitates the propulsive forces at push-off. These factors support the critical role of the foot in providing rigidity and an efficient lever at push-off. During running or sprinting, athletes need to generate and maintain the highest (linear) running velocity during a single effort in a sprinting lane. Acceleration and sprinting performance requires forces to be transmitted efficiently to the ground. It may be of particular interest to strengthen foot muscles to maintain and improve an optimal capacity to generate and absorb these forces. The current evidence supports multiple exercises to achieve higher strength in the foot, such as the “short foot exercise,” doming, toes curl, towing exercises or the more dynamic hopping exercises, or even barefoot running. Their real impact on foot muscle strength remains unclear and data related to its assessment remains scarce, despite a recognized need for this, especially before and after a strengthening intervention. It would be optimal to be able to assess it. In this article, we aim to provide the track and field community with an updated review on the current modalities available for foot strength assessment and training. We present recommendations for the incorporation of foot muscles training for performance and injury prevention in track and field.
Introduction
The foot is a complex joint system with multiple degrees of freedom that play an important role in athletic tasks such as running or sprinting. The compliance of the foot is remarkable and its spring-like properties—in the medial longitudinal arch (MLA) – allow mechanical energy to be stored and returned at each step (Ker et al., 1987). Previous studies have shown that this spring mechanism was provided by the elastic components of the plantar fascia or aponeurosis (PA). This may account for 8–17% of the mechanical energy required for a stride (Ker et al., 1987; Stearne et al., 2016) and it increases stiffness via the windlass mechanism. Recent studies showed however that this spring cannot simply be passive, as it cannot explain the ability of the foot to adapt to the mechanical loads of running or sprinting (Riddick et al., 2019). Kelly et al. (2018) showed during running that as speed increases, so does the dissipation of mechanical energy within the foot. This can be modulated by the muscular capacity of the intrinsic foot muscles (IFM).
The IFM are the main local stabilizers of the foot and are part of the active and neural subsystems that constitute the foot core (McKeon et al., 2015). With their anatomical insertions located under the foot, these muscles lengthen eccentrically during the early stance phase of running, producing negative work before they shorten during the late stance phase as the arch recoils to produce positive work (Fourchet and Gojanovic, 2016). This active contraction aids propulsion and is enabled by the following three muscle-tendon units: abductor hallucis (AbH), flexor digitorum brevis (FDB), and quadratus plantae (QP) (Kelly et al., 2015).
Because the IFM are usually neglected in assessment and treatment, a key component of foot core stability is not considered. We aim to provide the track and field community an updated review on the current modalities available for foot strength assessment and training. Running or sprinting is a cyclic activity involving all joints and muscles groups in the lower limbs, including the IFM, and the metatarsophalangeal (MTP) joint. We believe that it is of interest to assess the MTP joint's role during sprinting, especially looking at the link between midfoot and plantar flexors' torque before considering the various strengthening modalities and protocols. We will present recommendations for the incorporation of foot muscles training or performance and injury prevention in track and field.
The Forefoot Region and Running or Sprinting
During running or sprinting, athletes need to generate and maintain the highest (linear) running velocity during a single effort in a sprinting lane. Acceleration and sprinting performance requires forces to be transmitted efficiently to the ground. In particular, the production of horizontal force during the acceleration, more than vertical force, is related to sprinting performance. Indeed, the fastest runners at the end of the acceleration phase are not those who produce the highest total force, but those who manage to orient the forces horizontally (Morin and Samozino, 2016).
To achieve this, the sprinter must accomplish a series of segment rotations (Krell and Stefanyshyn, 2006) and gross moment generation about the lower limb joints, including hip, knee, and ankle (Tanaka et al., 2019). The small MTP joints (via dorsiflexion) may be related to sprint performance, as several studies have shown (Krell and Stefanyshyn, 2006; Bezodis et al., 2012). Stefanyshyn and Nigg (1997) investigated the energy patterns at the foot level during sprinting and found a large negative net energy balance (a lot of energy absorbed, whilst little produced) in the MTP joint during early to late stages of stance phase. These authors concluded that performance may be improved through a reduction in the energy loss at the MTP joint, but the question of how to achieve a better energy balance, hence better performance at push-off, remains. For example, Smith et al. (2014) found that varying the stiffness of spikes resulted in a significant decrease in MTP joint range of motion as well as dorsiflexion velocity when compared to barefoot. Spikes enable the MTP joint to plantar flex during push-off without affecting the windlass mechanism, which in turn facilitates propulsion by increasing the length of the moment arm (Smith et al., 2014).
This longer lever arm requires increased strength from the plantar flexors, and running athletes can benefit from it: a larger and more efficient horizontal force production may enhance performance (Morin and Samozino, 2016). A recent study showed that IFM activity in late stance is needed to generate sufficient impedance at the MTP joints, which in turn provides an efficient push-off (Farris et al., 2019).
Finally the MTP joint plays a key role during sprinting and that may result in the development of very strong foot muscles in sprinters population. However, Tanaka et al. (2019) demonstrated that although elite sprinters have thicker foot muscles compared with non-sprinters, AbH thickness correlates positively with their 100 m personal best. In other words a bigger AbH might be a negative factor for superior sprint performance. On the contrary, Yuasa et al. (2018) showed a significant correlation in collegiate American football players between maximal toe flexors strength with a dorsiflexed MTP and the ability to change direction in pro-agility and 3-cone tests. Abe et al. (2016) found in active subjects that fourth and fifth toe flexor strength was correlated positively with walking speed in men (r = 0.584) and women (r = 0.553), whereas Hashimoto and Sakuraba (2014) found that 8 weeks of toe flexors exercises decreased 50 m best personal time in 12 men.
Although toe flexors strength is generated by a simultaneous action of both intrinsic and extrinsic foot muscles, the IFM seem most likely to be the main contributors to MTP joints torque (Farris et al., 2019). Again, we should not underestimate the role of the MTP joints in strength training. In summary, strengthening interventions should not be limited to extrinsic foot and ankle muscles (e.g., flexor digitorum longus, triceps surae, flexor hallucis longus), but should also target intrinsic foot muscles.
The Midfoot (medial) Region and Running or Sprinting
The foot enjoys some flexibility characterized by the medial longitudinal arch (MLA) which compresses and recoils. This ability allows mechanical energy to be stored and then released sequentially with every running step. Previous studies have proposed that mobility of the MLA can partly enhance the triceps surae and longus flexor hallucis moment during the push-off (Leardini et al., 2007; Kelly et al., 2014). Fourchet et al. (2015) emphasized the important role of the MLA in transmission of force through the foot and as a load-absorbing structure in fatigued adolescent runners. This highlighted the reciprocal interaction between plantar flexors and MLA – a compliant MLA results in hyperpronation and this may impede force transmission through the foot at stance phase leading to early plantar flexor fatigue. This means that a more flexible midfoot does not really lock, and hence produces less power, inefficient force transfer through the foot lever and insufficient foot stiffness.
This relationship between MLA and plantar flexor torque has been shown in one study where hyperpronated feet showed lower concentric force of the plantar flexors when compared to neutral feet (Snook, 2001). These findings gave support to the following biomechanical theory: the lever angle of the Achilles tendon and the plantar flexors would at a disadvantage in hyperpronation, which leads some force produced by these muscles to be applied medially (no propulsive effect) rather than mostly upward (Fourchet, 2012). From a neurophysiological perspective, studies on electromyography (EMG) activity and pronation showed that hyperpronation may alter the function of foot and ankle muscles. Novacheck (1998) reported a delayed time to maximum pronation beyond 40% of stance in case of excessive pronation. This could be explained by the decreased activity of plantar flexors during fatigue: their supination effect is decreased and causes an increased load under the MLA. Fourchet et al. (2015) shown after high intensity running that plantar flexors display a reduced resistance to fatigue and an increase in relative load medially under the midfoot. We hypothesized that excessive foot pronation leads to fatigue of plantar flexor and points to the interdependence between plantar flexors and IFM, the latter being often difficult to turn on (Boon and Harper, 2003). We propose that there may be an interdependent coupling between plantar flexors and IFM, which is made biomechanically possible as plantar flexion moves the center of pressure forward and increases the load under the midfoot.
Finally, a stiff MLA seems to play a key role in ensuring a stable stance phase, and facilitating its load-absorbing task, the one which mitigates the dissipation of the mechanical energy produced by plantar flexors at push-off. Takahashi et al. (2016) demonstrated in walking that an increased foot stiffness (with shoes and orthotics) altered soleus muscle behavior, resulting in greater peak force and reduced fascicle shortening speed. Therefore, in addition to the optimization of spikes conception, implementing strengthening exercises of the intrinsic foot musculature with the aim to improve strength and stiffness of the MLA appears to be of high interest in athletes. This brings us to the necessity of specific and validated tests to assess such interventions.
Testing Foot Strength
The assessment of foot muscle strength is addressed in the literature with magnetic resonance imaging (MRI) or ultrasound imaging (USI) (Soysa et al., 2012; Gooding et al., 2016; Ridge et al., 2018) in order to quantify muscle thickness or cross sectional area, but these modalities are expensive and not applicable on the field by coaches or athletic trainers. Numerous other affordable measurement methods are available and come with an interesting level of validity and reliability. We can mention the toe flexor strength with toe-dynamometry (Spink et al., 2010) and the paper grip test (De Win et al., 2002) and there are several indirect tests assessing the strength or stiffness through the deformation or mobility of the foot arches in weight bearing and non-weight bearing conditions. They are the medial arch height (Okamura et al., 2017), the arch rigidity index (Mulligan and Cook, 2013), the navicular drop (ND), and the foot mobility measurement (FMM) (McPoil et al., 2009), which we will describe in detail.
Medial Arch Height
This assessment performed during gait and/or standing phase requires the use of the Oxford Foot Model, a 3D multi-segment foot model with a good to excellent repeatability (Okamura et al., 2017). In this test, the MLA height is defined as the normal distance of the plane of the forefoot from the proximal first metatarsal marker by the Oxford Foot Model. The medial arch height can be measured before and after a strengthening programme or a fatiguing protocol for instance (Okamura et al., 2017).
Arch Rigidity Index
The arch rigidity index (ARI) is calculated by dividing the standing arch height index by the sitting arch height index and it represents the structural mobility of the MLA (Mulligan and Cook, 2013). An ARI close to 1 represents a stiffer MLA while increasing foot flexibility correlates with numbers that rise well above 1. The arch height index is calculated by dividing the height of the dorsum of the foot by the truncated length of the foot to obtain a ratio in both seated and standing positions (Figure 1). The truncated length is the distance from the most posterior aspect of the calcaneus to the center of the first metatarsal head, and the height of the dorsum of the foot can be measured with a modified carpenter's square with a bubble level arm at 50% of the total foot length.
Navicular Drop
The sit-to-stand double-leg or single-leg navicular drop test is the most popular evaluation of longitudinal arch stability in the literature. The athlete sits with his hips, knees, and ankles bent to 90° and the feet resting on the floor. The inferior border of the prominent tuberosity of the navicular bone is palpated and marked with a pen, and the distance to the ground is measured using a steel ruler (resolution: 0.5 mm). At this point the tester asks the athlete to stand barefoot on a 4-in (10.16 cm) box, full weight on the foot being measured, while the other foot rests lightly on the box (Cote et al., 2005). The difference between the two measures (sitting vs. standing) is the navicular drop. The tester must repeat three measures and the average value is recorded.
Foot Mobility Measurement
The foot mobility measurement is a composite measure of vertical and medial to lateral mobility of the midfoot, whereas ND assess only vertical mobility. It has been described as a relevant technique for the assessment of foot mobility differences between non-weight bearing and weight bearing positions (McPoil et al., 2009).
To measure the FMM, three instruments are needed: weight bearing and non-weight bearing arch height gauges and a device to measure midfoot width, both of which can be relatively easily manufactured, with the inclusion of a digital caliper (Figure 1). Refer to McPoil et al. (2009) for a full description of the method. The athlete stands on a foot measurement platform (heels placed in heel cups) in order to measure dorsal arch height and midfoot width in bipodal weight bearing. Then, the same non-weight bearing measurements are recorded with the athlete sitting, both legs hanging in a perpendicular relaxed position. The procedure used for the weight bearing measures is then repeated. A method based on the Pythagorean Theorem is used to calculate the FMM:
where “Diff AH” and “Diff MFW” are the changes in dorsal arch height and in midfoot width between weight bearing and non-weight bearing, respectively.
Foot Strengthening Strategies
Strengthening of the foot muscles responds to the same training principles as any other muscle group. IFM strengthening can be performed in isometric, concentric, eccentric or plyometric modes.
Isometric Strengthening: Short Foot Exercise, Toe-Posture Exercises, and Tower Curl
In isometric strengthening of the IFM, the most recognized exercise is the short foot exercise (SFE) (McKeon and Fourchet, 2015), where volitional control of the intrinsic foot muscles elevates the foot arches and shortens the foot. This is described as part of the foot core paradigm introduced by McKeon et al. (2015). The SFE is typically challenging to teach and learn, so that three gradual training steps have been recommended: (1) passive mode, (2) active-assisted mode, and (3) active mode. In passive mode, the athlete's foot is moved by the therapist or the coach through the short foot movement, allowing the athlete to feel, learn, and integrate the different positions. In the active-assisted mode, active contractions of the plantar IFM are added to actively obtain the short foot position. Finally the active mode consists in the athlete performing the exercise without assistance (Figure 2).
It is also possible to combine the SFE with active and resisted activities of the upper body in order to create a cross-body inversion focus (i.e., trunk and pelvis medial rotation) and promote muscular chains facilitations (Figure 3).
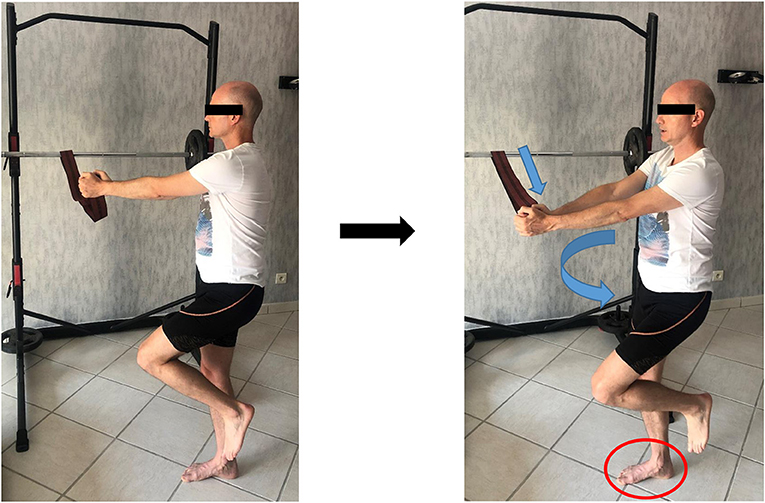
Figure 3. Short foot exercise with cross-body inversion focus (with written informed consent obtained from the subject).
It is worth mentioning that numerous others exercises commonly referred to as “toe yoga” or “toe posture exercises” have been shown to activate the IFM in a isometric contraction (Table 1). For example, the Toe-Spread-Out exercise (TSO), First To Fifth toe extension exercises are validated by Gooding et al. (2016). The TSO is carried out by a sequential extension of all toes, followed by hallux abduction, hallux flexion, and fifth toe flexion (Figure 4).
The “First-Toe Extension” or “Hallux-Extension” exercise is performed by extending the first metatarsophalangeal joint while maintaining the lesser toes (Second To Fifth) in contact with the floor (Figure 4). The “Second- To Fifth-Toes Extension” or “Lesser-Toes-Extension” exercise consists in extension of toes 2–5 whilst maintaining the hallux in contact with the ground (Figure 4).
We have stressed the importance of the MTP joint for sprint performance and some MTP strength exercises can be discussed. Previously used techniques that attempted to strengthen the IFM involved toe-flexion exercises (Hashimoto and Sakuraba, 2014) but it seems that these exercises recruit more of the extrinsic foot musculature (such as the flexor digitorum longus) and make these muscles dominant over the IFM (Lynn et al., 2012). Hashimoto and Sakuraba (2014) developed a strength training program that focused on IFM strength by excluding the extrinsic muscles as much as possible by bringing the ankle in plantar flexion. The “Towel curl exercise” or “toe flexor strength” is performed in sitting or standing position with or without added weights, where the subject is asked to slowly curl the toes and fold the towel or dynamometer under the foot by flexing the toes [interphalangeal (IP) and metatarsophalangeal (MTP) flexion] (Figure 5). This type of exercises permits also to work the horizontal strength product by the IFM with the help of the extrinsic foot muscles.
Dynamic and Plyometric Foot Strengthening
From a biomechanical perspective, isometric exercises are not reflective of how foot muscles work during locomotion (Farris et al., 2019). The magnitude of load at the midfoot during running or even walking is so high that it is not possible for the foot muscles to generate sufficient force with low load tasks like the SFE. We suggest to progress from isometric to plyometric exercises in order to get closer to the specific function of running. We can consider heel rises or any exercises shifting the CoP in front of the body, as these will likely impose a much higher load on the midfoot (Figures 6, 7).
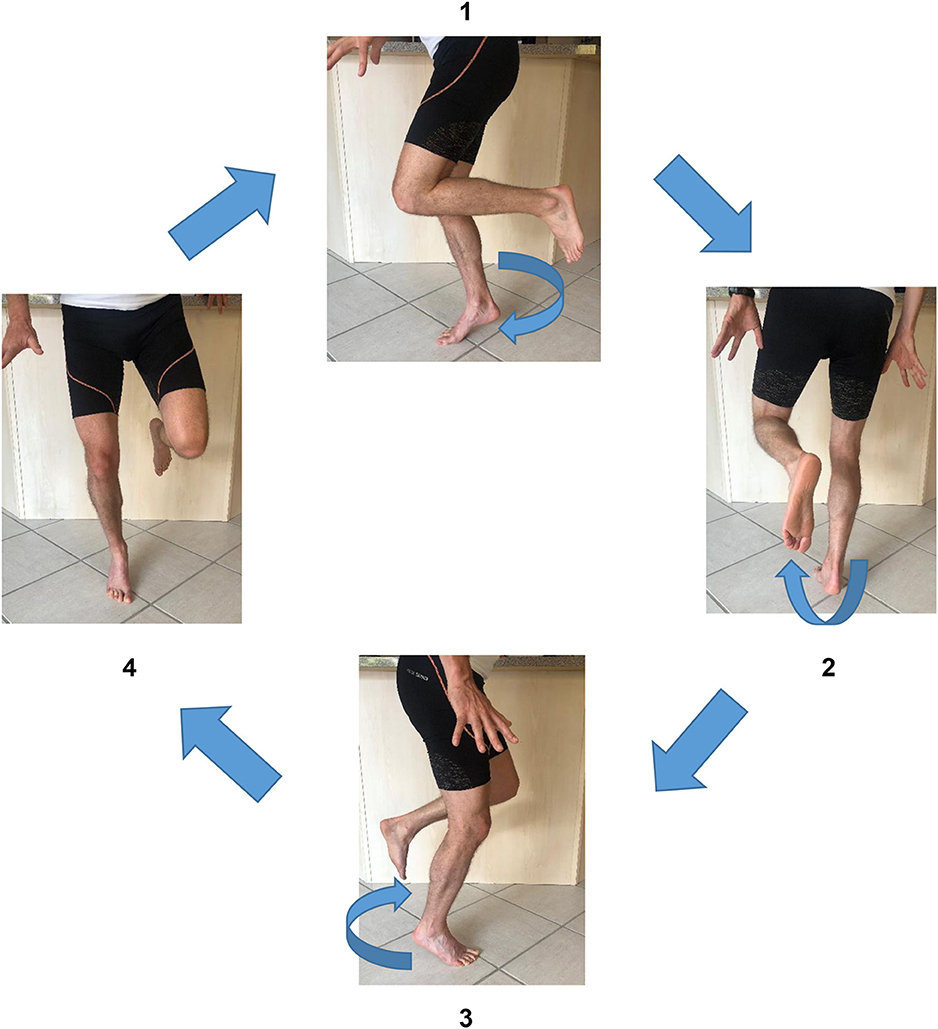
Figure 6. Short foot exercise in rotation (with written informed consent obtained from the subject).
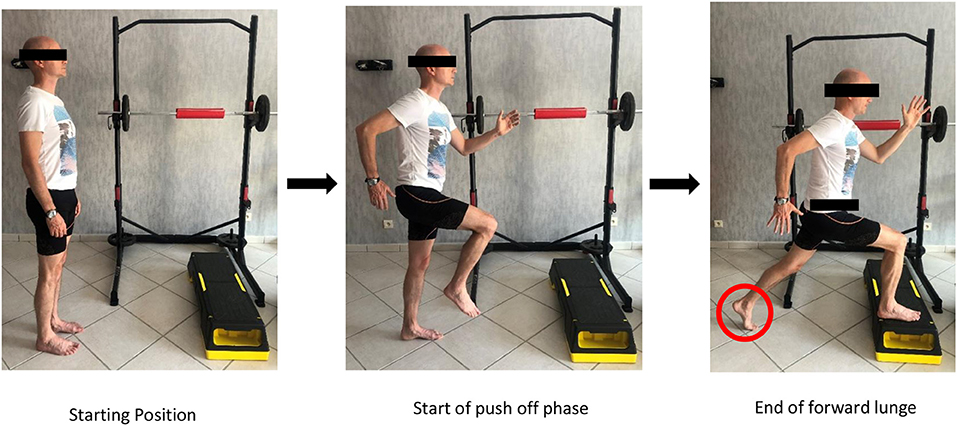
Figure 7. Short foot exercise during propulsion (with written informed consent obtained from the subject).
Minimalist or Barefoot Running
The literature on the effects of running on foot muscular adaptations is relatively scarce and somewhat contradictory. Nevertheless, it does suggest that running could improve the cross-sectional area and the volume of foot muscles, and that this may be modulated by running mileage and experience (Miller et al., 2014; Johnson et al., 2015; Chen et al., 2016; Ridge et al., 2018). Based on the limited evidence available, there seems to be a positive effect of running on intrinsic muscle strength and size (Garofolini and Taylor, 2019). We will review some of these studies briefly.
Johnson et al. (2015) found that 10 weeks of training in minimal running shoes may be effective in increasing muscle size, especially abductor hallucis cross-sectional area. Chen et al. (2016) found that a 6-months transitioning running program to minimal shoes led to larger IFM. It is worth mentioning that a strengthening program was added in this protocol, and it is not possible to say which component was responsible for the observed changes in muscle volume (Chen et al., 2016). Similarly, after a 12 weeks transitioning programme, a significant improvement was reported in the volume and the cross-sectional area of the abductor digiti minimi in recreational runners (Miller et al., 2014).
Neuromuscular Electrical Stimulation (NMES)
An additional modality for the volitional strengthening of foot muscles is neuromuscular electrical stimulation (NMES) of the IFM. The aim is to strengthen the foot lever as the first interface between the ground and the athlete (Fourchet and Gojanovic, 2016). The incorporation of this modality has been shown to improve foot postural control and plantar pressure distribution in runners (Fourchet et al., 2009, 2011).
Scientific findings suggest that using NMES on foot muscles can decrease navicular drop after a 3-weeks programme (three sessions a week). In another study, we showed that combining NMES with other exercises during 5 weeks shifted plantar foot pressure distribution laterally, which resulted in a reduction of loads under the medial midfoot during running (Fourchet et al., 2009, 2011).
Practically speaking, the set-up is very simple: the athlete stands with feet on the ground whilst the stimulator delivers NEMS as for 15 min, and a total of ~75 contractions completed per training session. The two electrodes are placed behind the head of the first metatarsal to stimulate the medial arch intrinsic muscles (Figure 8). Biphasic symmetric regular-wave pulsed currents (85 Hz) lasting 400 ms are delivered, and each tetanic stimulation is delivered for 4 s, followed by an active rest period lasting 8 s (McKeon and Fourchet, 2015).
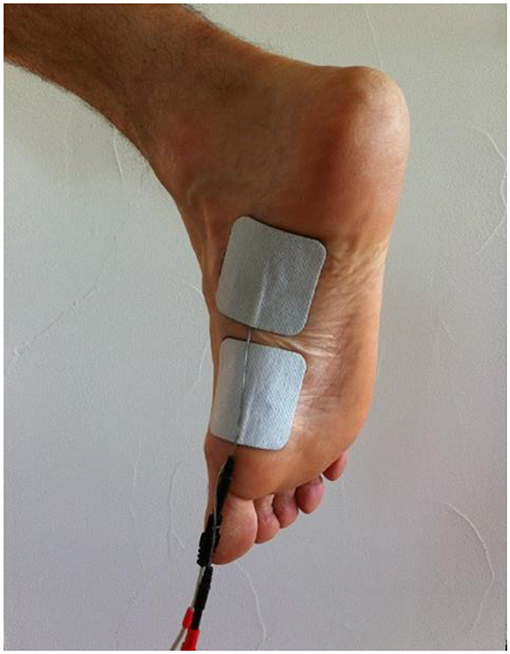
Figure 8. Placement of electrodes for NMES on medial arch intrinsic foot muscles (McKeon and Fourchet, 2015).
We recommend that the athletes performs an average of 9 to 12 NMES sessions through 3–5 weeks, as this will bring effective improvements. The athlete begins in bipodal stance during the first sessions and then progresses to single-leg stance and plyometric activities such as hopping.
Conclusion
The biomechanical specificities at the forefoot and the midfoot regions during running or sprinting require a high level of strength from the small foot muscles. The foot core system must act as a strong and rigid lever in order to best transfer lower limbs forces during propulsion, and it must also cope with significant amounts of constraints at the absorption phase, in the sense of impact attenuation.
The existing medical and scientific literature can help coaches and athletes to set up the most adapted exercises in order to strengthen their feet: variation and progression is necessary and ranges from isometric, concentric to eccentric contraction modes, from analytic to functional exercises, from volitional to electrically-assisted (NMES).
In order to track foot strength development and response to training, the athletic community can rely on several reliable and field-friendly assessments modalities.
This paper discusses aspects that are more performance-oriented or training-oriented, but the readers should keep in mind that the optimal control of the foot at stance phase is essential for the athlete's health as well. Overuse injuries linked to the control of the arch of the foot may be related to deficits in active foot stabilization during running, which may lead to increased tissue stresses. Medial tibial stress syndrome or Achilles tendinopathy are often linked to a lack of stiffness in the medial arch of the foot and its ability to cope with the changing demands for dynamic foot control.
We fully acknowledge that expert track and field coaches already apply a large body of the knowledge described in this article in their daily work with athletes. We do believe that for optimal health and performance outcomes, a close collaboration between coaches, sport scientists and medical staff is of primary interest and enables all parties to keep learning from each other.
Author Contributions
All authors listed have made a substantial, direct and intellectual contribution to the work, and approved it for publication.
Conflict of Interest
The authors declare that the research was conducted in the absence of any commercial or financial relationships that could be construed as a potential conflict of interest.
References
Abe, T., Tayashiki, K., Nakatani, M., and Watanabe, H. (2016). Relationships of ultrasound measures of intrinsic foot muscle cross-sectional area and muscle volume with maximum toe flexor muscle strength and physical performance in young adults. J. Phys. Ther. Sci. 28, 14–19. doi: 10.1589/jpts.28.14
Bezodis, N. E., Salo, A. I. T., and Trewartha, G. (2012). Modeling the stance leg in two-dimensional analyses of sprinting: inclusion of the MTP joint affects joint kinetics. J. Appl. Biomech. 28, 222–227. doi: 10.1123/jab.28.2.222
Boon, A. J., and Harper, C. M. (2003). Needle EMG of abductor hallucis and peroneus tertius in normal subjects. Muscle Nerve 27, 752–756. doi: 10.1002/mus.10356
Chen, T. L.-W., Sze, L. K. Y., Davis, I. S., and Cheung, R. T. H. (2016). Effects of training in minimalist shoes on the intrinsic and extrinsic foot muscle volume. Clin. Biomech. 36, 8–13. doi: 10.1016/j.clinbiomech.2016.05.010
Cote, K. P., Brunet, M. E., Gansneder, B. M., and Shultz, S. J. (2005). Effects of pronated and supinated foot postures on static and dynamic postural stability. J. Athl. Train. 40, 41–46.
De Win, M. M. L., Theuvenet, W. J., Roche, P. W., de Bie, R. A., and van Mameren, H. (2002). The paper grip test for screening on intrinsic muscle paralysis in the foot of leprosy patients. Int. J. Leprosy Other Mycobact. Dis. 70, 16–24.
Farris, D. J., Kelly, L. A., Cresswell, A. G., and Lichtwark, G. A. (2019). The functional importance of human foot muscles for bipedal locomotion. Proc. Natl. Acad. Sci. U.S.A. 116, 1645–1650. doi: 10.1073/pnas.1812820116
Fourchet, F. (2012). Foot-Ankle Injury Prevention in Adolescent Athletes. Reims: URCA University of Reims Champagne Ardennes.
Fourchet, F., Girard, O., Kelly, L., Horobeanu, C., and Millet, G. P. (2015). Changes in leg spring behaviour, plantar loading and foot mobility magnitude induced by an exhaustive treadmill run in adolescent middle-distance runners. J. Sci. Med. Sport 18, 199-203. doi: 10.1016/j.jsams.2014.01.007
Fourchet, F., and Gojanovic, B. (2016). Foot core strengthening: relevance in injury prevention and rehabilitation for runners. Swiss Sports Exerc. Med. 64, 26–30. Available online at: https://sgsm.ch/fileadmin/user_upload/Zeitschrift/64-2016-1/1-2016_3_Fourchet.pdf
Fourchet, F., Kilgallon, M., Loepelt, H., and Millet, G.-P. (2009). Électrostimulation des muscles plantaires et chute de l'os naviculaire. Sci. Sports, 24, 262–264. doi: 10.1016/j.scispo.2009.03.005
Fourchet, F., Kuitunen, S., Girard, O., Beard, A. J., and Millet, G. P. (2011). Effects of combined foot/ankle electromyostimulation and resistance training on the in-shoe plantar pressure patterns during sprint in young athletes. J. Sports Sci. Med. 10, 292-300. Available online at: https://www.jssm.org/hf.php?id=jssm-10-292.xml
Fraser, J. J., and Hertel, J. (2018). Effects of a 4-week intrinsic foot muscle exercise program on motor function: a preliminary randomized control trial. J. Sport Rehabil. 1–11. doi: 10.1123/jsr.2017-0150
Garofolini, A., and Taylor, S. (2019). The effect of running on foot muscles and bones: a systematic review. Hum. Mov. Sci. 64, 75-88. doi: 10.1016/j.humov.2019.01.006
Goldmann, J.-P., Sanno, M., Willwacher, S., Heinrich, K., and Brüggemann, G.-P. (2013). The potential of toe flexor muscles to enhance performance. J. Sports Sci. 31, 424–433. doi: 10.1080/02640414.2012.736627
Gooding, T. M., Feger, M. A., Hart, J. M., and Hertel, J. (2016). Intrinsic foot muscle activation during specific exercises: a T2 time magnetic resonance imaging study. J. Athl. Train. 51, 644-650. doi: 10.4085/1062-6050-51.10.07
Hashimoto, T., and Sakuraba, K. (2014). Strength training for the intrinsic flexor muscles of the foot: effects on muscle strength, the foot arch, and dynamic parameters before and after the training. J. Phys. Ther. Sci. 26, 373-376. doi: 10.1589/jpts.26.373
Johnson, A., Myrer, J., Mitchell, U., Hunter, I., and Ridge, S. (2015). The effects of a transition to minimalist shoe running on intrinsic foot muscle size. Int. J. Sports Med. 37, 154-158. doi: 10.1055/s-0035-1559685
Jung, D.-Y., Kim, M.-H., Koh, E.-K., Kwon, O.-Y., Cynn, H.-S., and Lee, W.-H. (2011a). A comparison in the muscle activity of the abductor hallucis and the medial longitudinal arch angle during toe curl and short foot exercises. Phys. Ther. Sport 12, 30-35. doi: 10.1016/j.ptsp.2010.08.001
Jung, D.-Y., Koh, E.-K., and Kwon, O.-Y. (2011b). Effect of foot orthoses and short-foot exercise on the cross-sectional area of the abductor hallucis muscle in subjects with pes planus: a randomized controlled trial1. J. Back Musculoskelet. Rehabil. 24, 225–231. doi: 10.3233/BMR-2011-0299
Kamonseki, D. H., Gonçalves, G. A., Yi, L. C., and Júnior, I. L. (2016). Effect of stretching with and without muscle strengthening exercises for the foot and hip in patients with plantar fasciitis: a randomized controlled single-blind clinical trial. Man. Ther. 23, 76–82. doi: 10.1016/j.math.2015.10.006
Kelly, L. A., Cresswell, A. G., Racinais, S., Whiteley, R., and Lichtwark, G. (2014). Intrinsic foot muscles have the capacity to control deformation of the longitudinal arch. J. R. Soc. Interface 11, 20131188–20131188. doi: 10.1098/rsif.2013.1188
Kelly, L. A., Farris, D. J., Cresswell, A. G., and Lichtwark, G. A. (2018). Intrinsic foot muscles contribute to elastic energy storage and return in the human foot. J. Appl. Physiol. 126, 231–238. doi: 10.1152/japplphysiol.00736.2018
Kelly, L. A., Lichtwark, G., and Cresswell, A. G. (2015). Active regulation of longitudinal arch compression and recoil during walking and running. J. R. Soc. Interface 12, 20141076–20141076. doi: 10.1098/rsif.2014.1076
Ker, R. F., Bennett, M. B., Bibby, S. R., Kester, R. C., and Alexander, R. M. (1987). The spring in the arch of the human foot. Nature 325, 147–149. doi: 10.1038/325147a0
Kim, E.-K., and Kim, J. S. (2016). The effects of short foot exercises and arch support insoles on improvement in the medial longitudinal arch and dynamic balance of flexible flatfoot patients. J. Phys. Ther. Sci. 28, 3136–3139. doi: 10.1589/jpts.28.3136
Kim, M.-H., Kwon, O.-Y., Kim, S.-H., and Jung, D.-Y. (2013). Comparison of muscle activities of abductor hallucis and adductor hallucis between the short foot and toe-spread-out exercises in subjects with mild hallux valgus. J. Back Musculoskelet. Rehabil. 26, 163–168. doi: 10.3233/BMR-2012-00363
Kim, M.-H., Yi, C.-H., Weon, J.-H., Cynn, H.-S., Jung, D.-Y., and Kwon, O.-Y. (2015). Effect of toe-spread-out exercise on hallux valgus angle and cross-sectional area of abductor hallucis muscle in subjects with hallux valgus. J. Phys. Ther. Sci. 27, 1019–1022. doi: 10.1589/jpts.27.1019
Krell, J. B., and Stefanyshyn, D. J. (2006). The relationship between extension of the metatarsophalangeal joint and sprint time for 100 m Olympic athletes. J. Sports Sci. 24, 175–180. doi: 10.1080/02640410500131621
Leardini, A., Benedetti, M. G., Berti, L., Bettinelli, D., Nativo, R., and Giannini, S. (2007). Rear-foot, mid-foot and fore-foot motion during the stance phase of gait. Gait Posture 25, 453–462. doi: 10.1016/j.gaitpost.2006.05.017
Lynn, S. K., Padilla, R. A., and Tsang, K. K. W. (2012). Differences in static- and dynamic-balance task performance after 4 weeks of intrinsic-foot-muscle training: the short-foot exercise versus the towel-curl exercise. J. Sport Rehabil. 21, 327–333. doi: 10.1123/jsr.21.4.327
McKeon, P. O., and Fourchet, F. (2015). Freeing the Foot. Clin. Sports Med. 34, 347–361. doi: 10.1016/j.csm.2014.12.002
McKeon, P. O., Hertel, J., Bramble, D., and Davis, I. (2015). The foot core system: a new paradigm for understanding intrinsic foot muscle function. Br. J. Sports Med. 49, 290–290. doi: 10.1136/bjsports-2013-092690
McPoil, T. G., Vicenzino, B., Cornwall, M. W., Collins, N., and Warren, M. (2009). Reliability and normative values for the foot mobility magnitude: a composite measure of vertical and medial-lateral mobility of the midfoot. J. Foot Ankle Res. 2:6. doi: 10.1186/1757-1146-2-6
Miller, E. E., Whitcome, K. K., Lieberman, D. E., Norton, H. L., and Dyer, R. E. (2014). The effect of minimal shoes on arch structure and intrinsic foot muscle strength. J. Sport Health Sci. 3, 74–85. doi: 10.1016/j.jshs.2014.03.011
Moon, D., Kim, K., and Lee, S. (2014). Immediate effect of short-foot exercise on dynamic balance of subjects with excessively pronated feet. J. Phys. Ther. Sci. 26, 117–119. doi: 10.1589/jpts.26.117
Morin, J.-B., and Samozino, P. (2016). Interpreting power-force-velocity profiles for individualized and specific training. Int. J. Sports Physiol. Perform. 11, 267–272. doi: 10.1123/ijspp.2015-0638
Mulligan, E. P., and Cook, P. G. (2013). Effect of plantar intrinsic muscle training on medial longitudinal arch morphology and dynamic function. Man. Ther. 18, 425–430. doi: 10.1016/j.math.2013.02.007
Novacheck (1998). The biomechanics of running. Gait Posture 7, 77–95. doi: 10.1016/S0966-6362(97)00038-6
Okamura, K., Kanai, S., Oki, S., Tanaka, S., Hirata, N., Sakamura, Y., et al. (2017). Does the weakening of intrinsic foot muscles cause the decrease of medial longitudinal arch height? J. Phys. Ther. Sci. 29, 1001–1005. doi: 10.1589/jpts.29.1001
Panichawit, C., Bovonsunthonchai, S., Vachalathiti, R., and Limpasutirachata, K. (2015). Effects of foot muscles training on plantar pressure distribution during gait, foot muscle strength, and foot function in persons with flexible flatfoot. J. Med. Assoc. Thai. 98 (Suppl. 5), S12–17. Available online at: http://www.jmatonline.com/index.php/jmat/article/view/6337
Riddick, R., Farris, D. J., and Kelly, L. A. (2019). The foot is more than a spring: human foot muscles perform work to adapt to the energetic requirements of locomotion. J. R. Soc. Interface 16:20180680. doi: 10.1098/rsif.2018.0680
Ridge, S. T., Olsen, M. T., Bruening, D. A., Jurgensmeier, K., Griffin, D., Davis, I. S., et al. (2018). Walking in minimalist shoes is effective for strengthening foot muscles. Med. Sci. Sports Exerc. 1:104–113. doi: 10.1249/MSS.0000000000001751
Smith, G., Lake, M., and Lees, A. (2014). Metatarsophalangeal joint function during sprinting: a comparison of barefoot and sprint spike shod foot conditions. J. Appl. Biomech. 30, 206–212. doi: 10.1123/jab.2013-0072
Snook, A. G. (2001). The relationship between excessive pronation as measured by navicular drop and isokinetic strength of the ankle musculature. Foot Ankle Int. 22, 234–240. doi: 10.1177/107110070102200311
Soysa, A., Hiller, C., Refshauge, K., and Burns, J. (2012). Importance and challenges of measuring intrinsic foot muscle strength. J. Foot Ankle Res. 5:29. doi: 10.1186/1757-1146-5-29
Spink, M. J., Fotoohabadi, M. R., and Menz, H. B. (2010). Foot and ankle strength assessment using hand-held dynamometry: reliability and age-related differences. Gerontology 56, 525–532. doi: 10.1159/000264655
Stearne, S. M., McDonald, K. A., Alderson, J. A., North, I., Oxnard, C. E., and Rubenson, J. (2016). The foot's arch and the energetics of human locomotion. Sci. Rep. 6:19403. doi: 10.1038/srep19403
Stefanyshyn, D. J., and Nigg, B. M. (1997). Mechanical energy contribution of the metatarsophalangeal joint to running and sprinting. J. Biomech. 30, 1081–1085. doi: 10.1016/S0021-9290(97)00081-X
Sudhakar, S., Veena Kirthika, S., Padmanabhan, K., Ramachandran, S., Senthil Nathan, C. V., Mohan Kumar, G., et al. (2018). Efficacy of plantar short foot muscle exercise among middle distance runners: a single blind randomized controlled, pilot study. J. Bodywork Mov. Ther. doi: 10.1016/j.jbmt.2018.06.009
Sulowska, I., Mika, A., Oleksy, Ł., and Stolarczyk, A. (2019). The influence of plantar short foot muscle exercises on the lower extremity muscle strength and power in proximal segments of the kinematic chain in long-distance runners. Biomed. Res. Int. 2019:6947273. doi: 10.1155/2019/6947273
Sulowska, I., Oleksy, Ł., Mika, A., Bylina, D., and Sołtan, J. (2016). The influence of plantar short foot muscle exercises on foot posture and fundamental movement patterns in long-distance runners, a non-randomized, non-blinded clinical trial. PLoS ONE 11:e0157917. doi: 10.1371/journal.pone.0157917
Taddei, U. T., Matias, A. B., Ribeiro, F. I. A., Inoue, R. S., Bus, S. A., and Sacco, I. C. N. (2018). Effects of a therapeutic foot exercise program on injury incidence, foot functionality and biomechanics in long-distance runners: feasibility study for a randomized controlled trial. Phys. Ther. Sport 34, 216–226. doi: 10.1016/j.ptsp.2018.10.015
Takahashi, K. Z., Gross, M. T., van Werkhoven, H., Piazza, S. J., and Sawicki, G. S. (2016). Adding stiffness to the foot modulates soleus force-velocity behaviour during human walking. Sci. Rep. 6:29870. doi: 10.1038/srep29870
Tanaka, T., Suga, T., Imai, Y., Ueno, H., Misaki, J., Miyake, Y., et al. (2019). Characteristics of lower leg and foot muscle thicknesses in sprinters: does greater foot muscles contribute to sprint performance? Eur. J. Sport Sci. 19, 442–450. doi: 10.1080/17461391.2018.1534991
Unver, B., Erdem, E. U., and Akbas, E. (2019). Effects of short-foot exercises on foot posture, pain, disability, and plantar pressure in Pes Planus. J Sport Rehabil. 16, 1–5. doi: 10.1123/jsr.2018-0363
Keywords: intrinsic foot muscles, foot strengthening, assessment, track and field athletics, exercises
Citation: Tourillon R, Gojanovic B and Fourchet F (2019) How to Evaluate and Improve Foot Strength in Athletes: An Update. Front. Sports Act. Living 1:46. doi: 10.3389/fspor.2019.00046
Received: 17 July 2019; Accepted: 24 September 2019;
Published: 11 October 2019.
Edited by:
Olivier Girard, Murdoch University, AustraliaReviewed by:
Alexandre Igor Araripe Medeiros, Universidade Federal Do Ceará, BrazilHelen Bayne, University of Pretoria, South Africa
Copyright © 2019 Tourillon, Gojanovic and Fourchet. This is an open-access article distributed under the terms of the Creative Commons Attribution License (CC BY). The use, distribution or reproduction in other forums is permitted, provided the original author(s) and the copyright owner(s) are credited and that the original publication in this journal is cited, in accordance with accepted academic practice. No use, distribution or reproduction is permitted which does not comply with these terms.
*Correspondence: Romain Tourillon, romain.tourillon@etu.univ-nantes.fr; Boris Gojanovic, boris.gojanovic@latour.ch; François Fourchet, francois.fourchet@latour.ch