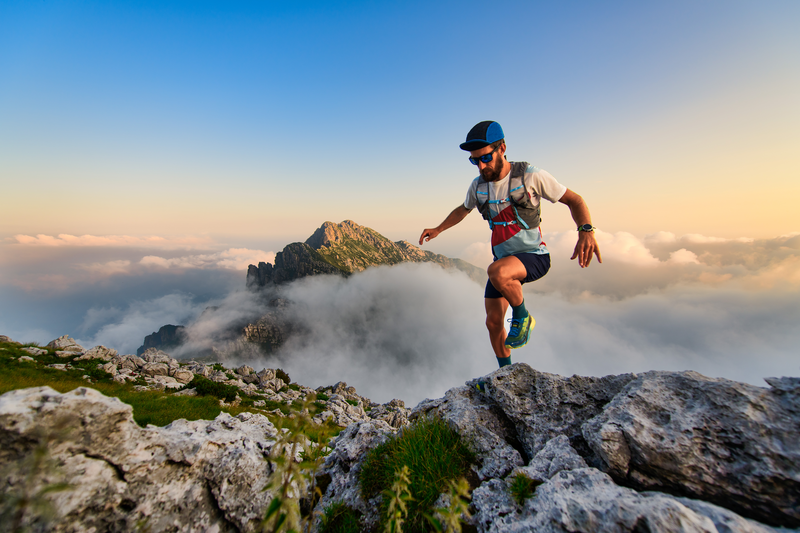
95% of researchers rate our articles as excellent or good
Learn more about the work of our research integrity team to safeguard the quality of each article we publish.
Find out more
MINI REVIEW article
Front. Astron. Space Sci. , 17 December 2024
Sec. Astrochemistry
Volume 11 - 2024 | https://doi.org/10.3389/fspas.2024.1523977
This article is part of the Research Topic Frontiers in Astronomy and Space Sciences: A Decade of Discovery and Advancement - 10th Anniversary Conference View all 9 articles
Silicate dust is found in a wide range of astrophysical environments. Nucleation and growth of silicate dust grains in circumstellar environments likely involves species with diameters ranging from <1 nm (molecular silicates) to a few nanometers (nanosilicates). When fully formed silicate grains with sizes ∼0.1 μm enter the interstellar medium, supernovae shockwaves cause collision-induced shattering which is predicted to redistribute a significant proportion of the silicate dust mass into a huge number of nanosilicates. This presumed population has thus far not been unambiguously confirmed by observation but is one of the main candidates for causing the anomalous microwave emission. By virtue of their extreme small size, nanosilicates and molecular silicates could exhibit significantly different properties to larger silicate grains, which could be of astrochemical and astrophysical importance. Herein, we briefly review the properties of these ultrasmall silicate dust species with a focus on insights arising from bottom-up atomistic computational modelling. Finally, we highlight how such modelling also has the unique potential to predict observationally verifiable spectral features of nanosilicates that may be detectable using the James Webb Space Telescope.
Cosmic dust, consisting mainly of sub-micron sized grains, permeates the interstellar medium (ISM) (Draine, 2003). Although contributing to only 1% of the mass of the ISM, dust has a disproportionately high impact, both astrophysically (e.g., shielding high energy radiation) and astrochemically (e.g., providing adsorption sites for chemicial reactions) (van Dishoeck, 2014). The majority of ISM dust grains are formed from silicates (Henning, 2010), which encompass a wide class of solids formed from the interactions between anions based on silicon and oxygen (e.g., SiO32-, SiO44-) and cations. In astronomical silicates, the cations are mainly magnesium (Mg2+) with a much smaller proportion of iron (Fe2+) (Min et al., 2007). It is usually assumed that most silicate dust has stoichiometric pyroxene or olivine chemical compositions, for which MgSiO3 (enstatite) and Mg2SiO4 (forsterite) are the corresponding Mg-based examples. Silicates with intermediate compositions have also been proposed (Fogerty et al., 2016). Observations suggest that the number of dust grains (ng) in the ISM with respect to their diameter (d) approximately follows an inverse power relationship (ng ∝ d−3.5) (Mathis et al., 1977), which massively favours higher populations of smaller grains. Such a distribution can be rationalised by models which redistribute mass from larger grains into smaller grains by collisional shattering, leading to predictions that ∼5% of the silicate dust mass in the ISM should end up in small nanosilicate grains with approximate sizes d ≤ 6 nm (Jones et al., 1996). Considering infrared (IR) emission observations of the ISM, it has been estimated that small nanosilicates (d ≤ 3 nm) could account for up to 10% of all silicon in the dust population (Li and Draine, 2001). Even if accounting for only a relatively small fraction of the total silicate dust grain mass in the ISM, nanosilicates could thus potentially dominate dust populations by number. Speculatively extrapolating such predictions would lead to the possibility that nanosilicates could be the most common class of solid object in the Universe. Herein, we focus on the smallest silicate dust species, ranging from molecular silicates containing a few atoms to nanosilicates, with approximate diameters ranging between 1–10 nm. When the dimensions of oxide materials are reduced to the nanoscale new structures and properties can emerge (Bromley et al., 2009). Compared to larger dust grains, nanosilicates are thus likely to have novel and distinct astrophysical and astrochemical impacts. Herein, we provide an overview what is currently known about molecular silicates and nanosilicates from an atomistic level, and how such knowledge can inform us on the prospects for their observational detection.
Due the extreme small sizes of nanoscale grain species it is often extremely difficult to characterise them experimentally. Here, atomistic level computational modelling can play an essential role in providing realistic nanoscale structural models and atomic/electronic level insights, which would be otherwise unobtainable (Bromley et al., 2014). Atomistically detailed nano-models can subsequently be used to calculate properties (e.g., atomic and electronic structures, chemical reactivity, spectroscopy), which can be compared with, and used together with, any available experimental/observational data. Even when the latter is unavailable, such bottom-up computational modelling can provide detailed predictions and helpful insights which can help to guide future experimental/observational research. In Figure 1 we show a range of ultrasmall silicate species for which atomistic computational modelling has provided a wealth of astronomical relevant information. Below we summarise results from selected studies on molecular silicates (<1 nm diameter) and nanosilicates with respect to: i) nucleation, ii) energetic processing, iii) chemical interactions and iv) spectroscopic signatures.
Figure 1. Examples of small silicate dust species studied with atomistic computational modelling ranging from molecular silicates containing of the order 10 atoms [i.e., O (10)] to small nanosilicates containing O (1,000) atoms. Representative structures for silicate species and their characteristic predicted IR spectra are approximately positioned with respect to the three corresponding silicate size classes (blue bar). Calculated microwave emission spectra are shown for a molecular silicate (left) and for three different silicate sizes (right): black (5 atoms), red (21 atoms), blue (50 atoms). All spectra are shown with intensity in arbitrary units.
Most silicate dust is thought to originate in the circumstellar envelopes of oxygen-rich M-type asymptotic giant branch (AGB) stars. Here, the excess oxygen strongly binds with abundant silicon atoms, leading to the formation of silicon monoxide (SiO) molecules (González Delgado et al., 2003). The formation of silicates should involve several chemical processes which enable the incorporation of Mg (and Fe) and the increase of a 1:1 silicon-to-oxygen stoichiometry in SiO monomers to that found in silicates (e.g., MgSiO3, Mg2SiO4). These required chemical transitions suggest that silicate nucleation pathways likely involve complex homogeneous molecular processes in the gas phase and heterogeneous processes on existing grains. Based on typical elemental abundances and physical conditions in a circumstellar silicate dust condensation zone (1,000–1,200 K, 0.1–0.001 Pa), the available chemical pathways for silicates are likely dictated by the abundance of stable oxygen-containing molecules (H2O, OH and SiO), while Mg is stable in atomic form (Gail and Sedlmayr, 1986; Gail and Sedlmayr, 1998). Systematic searches using accurate computer modelling can reveal molecular silicate species resulting from the chemical reactions of these components which are stable under such conditions (Goumans and Bromley, 2012). Using this data, a general alternating oxidation/metal-incorporation silicate nucleation scheme has been proposed and validated in detailed kinetic nucleation theory models of dust formation in supernovae (Sarangi and Cherchneff, 2013) and AGB stars (Gobrecht et al., 2016), see Figure 2. At the high temperatures in circumstellar environments, the initial production of small silicon oxide molecules [e.g., (SiO)2, Si2O3] is most difficult and is a bottleneck to subsequent nucleation (Bromley et al., 2016; Andersson et al., 2023). It is thus likely that the very first species that initiate circumstellar silicate nucleation are, as yet unidentified, extremely stable refractory seeds (e.g., Ti-based (Jeong et al., 2003; Goumans and Bromley, 2013; Plane, 2013) or Al-based (Gobrecht et al., 2022; Gobrecht et al., 2023) oxide species). We note that this does not rule out homogeneous SiO nucleation being involved in silicate dust formation in lower temperature environments [e.g., cold dense clouds (Krasnokutski et al., 2014), around Mira-type variable stars (Gail et al., 2016)].
Figure 2. Possible thermodynamically viable initial steps in silicate dust nucleation starting from the SiO dimer (i.e., Si2O2) up to the pyroxene dimer (i.e., Mg2Si2O6) molecular silicate. Steps show incorporation of Mg or O (via H2O or OH) with respect to the previous step. The lowest energy structure is shown for all molecular species. See Goumans and Bromley (2012) for more details.
At the end of their growth phase around evolved stars, silicate dust grains are thought to attain a typical radius of ∼0.1 μm before they enter the ISM. The morphology of these grains is unknown but will depend on the efficiency of nucleation and growth, and the availability of monomeric species. For example, the highly inefficient monomeric-based growth of SiOx grains can leads to a high number of nanosized particles, which can condense into larger fractal aggregates (Kimura et al., 2022). For silicates, laboratory studies show that dust formation often also proceeds via primary particles of a few nanometres in radius (i.e., nanosilicates), followed by rapid growth through agglomeration producing a smaller number of large fractal-like particles (Saunders and Plane, 2006). A similar nanosilicate aggregation process could potentially also play a role in dust (re)formation in the ISM (Rimola and Bromley, 2021). Relative to more spherical compact grains, such nanosilicate aggregates (see Figure 1) possess high surface areas and porosities which could have astrochemical implications (Potapov et al., 2024).
Newly formed silicate dust from evolved AGB stars is thought to be Mg-rich, with an olivinic chemical composition (Dorschner et al., 1995) and with ∼10% being crystalline (Molster et al., 2002). From analysis of IR spectra, the extent of crystallinity of silicates in the ISM is estimated to be between 0%–5% by mass (Kemper et al., 2004; Li et al., 2007), suggesting that the original crystalline fraction of the grain population is amorphized in the ISM. Laboratory experiments have shown that amorphisation of silicates could result from impacts from high velocity ions in supernovae shocks and heavy ions in cosmic rays (Demyk et al., 2001; Jäger et al., 2003a; Bringa et al., 2007). However, it is often overlooked that, below a certain size, nanosilicate grains are inherently not able to maintain long range crystalline order, thus being effectively amorphous. Energetically stable ultrasmall nanosilicates (≤1 nm diameter) of pyroxene and olivine composition have been confirmed to be inherently non-crystalline by accurate quantum chemical calculations (Maciá Escatller et al., 2019). Computational modelling of larger nanosilicate grains up to approximately 5 nm diameter, still show that amorphous structures obtained by thermal annealing are more energetically stable than crystalline nanograins (Zamirri et al., 2019). Aggregates formed from nanosilicate building blocks will thus also tend to be amorphous. Indications of crystallinity have been inferred in laboratory produced silicate grains with diameters of a few 10 s of nm, indicating a possible lower grain size for maintaining crystalline order (Ishizuka et al., 2015). Generally, the fact that crystallinity is only possible in larger silicate grains should be considered when interpreting observed spectra.
In the diffuse ISM, dust grains are subject to energetic processing by supernovae shockwaves which lead to shattering in grain-grain collisions. Shattering leads to a redistribution of mass from a smaller number of larger grains into a larger number of smaller grains tending to lead to an abundance of nanosized grains (Jones et al., 1996). For porous nanosilicate aggregates, such processing would likely lead to disaggregation. Smaller grains are also more resilient than larger grains to shattering which could help them persist in the harsh conditions of the diffuse ISM (Jones et al., 1994). Shockwave-induced collisions and other processes can also lead to vaporization of grain mass into the gas phase (i.e., dust destruction). Models of grain processing typically predict that the estimated dust destruction rate in the ISM is significantly more than the stardust production rate (Slavin et al., 2015). This situation is clearly at odds with the observed abundance of dust in the ISM. The discrepancy probably reflects the large uncertainties in our estimates of dust lifetimes, based on our current understanding of dust formation/destruction (Jones and Nuth, 2011).
Through their interaction with the strong ultraviolet (UV) radiation field in the ISM, dust grains with sizes of only a few nanometres are also susceptible to sporadic temperature spikes (Duley, 1973). Stochastically heated nanosilicate grains with 10 nm diameters are expected to exhibit temperature fluctuations up to 20–40 K, which is predicted to have a significant impact on their role in astrochemical processes (Cuppen et al., 2006; Chen et al., 2018). For ultrasmall nanosilicates possessing only a few tens of atoms (≤1 nm diameter) the fluctuations in temperature from stochastic heating has been estimated to be up to ∼1000 K (Draine and Li, 2001), with, as yet, unknown consequences for astrochemistry. We note that molecular silicates and ultrasmall silicates are found to be surprisingly structurally stable in molecular dynamics simulations at such elevated temperatures where they exhibit only small conformational changes (Mariñoso Guiu et al., 2021a; Oueslati et al., 2015). The temperature and emissivity of stochastically heated nanograins depends on the rates of UV energy absorption and subsequent IR emission, which, in turn is intimately linked to their specific heat capacity. Typical estimates of stochastic heating employ empirical fits to bulk silicate heat capacities (Draine and Li, 2001; Camps et al., 2015). The heat capacity of a system depends on the range of available vibrational modes, which is dramatically affected by size constraints in nanosized solids. Accurate quantum chemical calculation of vibrational modes in ultrasmall nanosilcates have shown that empirically fitted models tend to underestimate the stochastic heating of small nanosilicates by tens of Kelvin (Mariñoso Guiu and Bromley, 2022). This could imply that ultrasmall nanosilicates could be easier to detect by their IR emission than previously expected.
The strong UV field in the ISM can also lead to ionisation of small dust grains. Due to the dominance of photoelectric electron ejection over recombination in very small grains in the ISM, small nanosilicates are also likely to be positively ionized by their interaction with high energy UV photons (Weingartner and Draine, 2001). UV-induced charging of small grains has been suggested as a way to enhancing their role as seed species for dust (re)nucleation in the ISM (Zhukovska et al., 2016). Cationic molecular silicates have also been produced in cluster beam experiments and their structures and properties elucidated by quantum chemical calculations (Mariñoso Guiu et al., 2022). These studies also suggest that ionised molecular silicates could act as sinks for oxygen depletion, potentially contributing to the unresolved problem of the “missing” oxygen in the ISM (Whittet, 2010).
Processing from ion irradiation (e.g., protons, He+) in supernovae shocks also induces chemical tranformations in silicate dust grains in the ISM. From early laboratory studies of the effect of the solar wind on lunar surface silicate material, two important intertwined chemical processes were established to be the result of proton-iradiation: (i) the formation of hydroxyls (Zeller et al., 1996), and (ii) the depletion of magnesium and oxygen through preferential sputtering (Keller and McKay, 1997). Confirmation of these results has come from more recent experiments (Demyk et al., 2001; Jäger et al., 2003b; Bringa et al., 2007) and from analysis of interplanetary dust (Bradley, 1994). Hydroxylated silicate grains formed by such processing could also be relevant as potential reservoirs of water during the formation of planetary systems (Djouadi et al., 2011).
Starting from Mg-rich olivine nanograins, sputtering of magnesium and oxygen would tend to lead to a transformation towards a pyroxene-like chemical composition. Elevated levels of pyroxene (relative to the initial dominance of olivine) is consistent with estimates of the amorphous pyroxene fraction of the grain population in the ISM (Kemper et al., 2004), and with the pyroxene-rich environments of protostars (Demyk et al., 1999). Combining chemical processing with energetic processing (i.e., shockwave-induced shattering and ionisation), the extreme end result would be molecular pyroxene-based cations (e.g., MgSiO3+). Higher proton-induced sputtering could potentially lead to extreme reduction of Mg2+ and hydroxyl formation from H+ implantation, leading to (SixOyHz)+ species. By approximately mimicking silicate dust grain processing occurring in the diffuse ISM by ablation/cooling of a mixed Si/Mg source in the presence of O2, clusters based on discrete ionized pyroxene monomers (MgSiO3+) were readily formed (Mariñoso Guiu et al., 2022). Similar experiments, using a pure Si source and a small amount of water vapour, led to the production of small (SixOyHz)+ clusters with one or two -OH groups (de Donato et al., 2024). This indicates that such ionised molecular silicate species could also be a common result of energetic and chemical processing in the ISM. More speculatively, such experiments could also suggest that molecular silicate formation from hot gas phase atoms could directly compete with dust destruction during energetic processing.
Quantum chemical calculations have also shown that formation of Si-OH groups is highly energetically favourable on pyroxene nanosilicates (Goumans and Bromley, 2011) and (SiO2)N nanoclusters (Jelfs et al., 2013; Maciá Escatller et al., 2017). Such hydroxylation is likely to be a precursor stage to the subsequent formation of water ice mantles. However, UV irradiation is known to reduce hydroxylation of silica surfaces (Imai et al., 1996) and lead to water photodesorption. As such, high degrees of nanosilicate hydroxylation and water ice mantle growth are only likely to occur in interstellar clouds that are opaque enough to shield the intense UV field of the ISM. Bare nanosilicates (i.e., without mantles) been predicted to be able to promote dissociation and/or formation of H2 molecules (Kerkeni and Bromley, 2013). Other theoretical studies have shown that such chemical processes can be significantly affected by the low degree of hydroxylation likely to be possible in the diffuse/translucent ISM (Kerkeni et al., 2017; Kerkeni et al., 2019).
The best current observational evidence for the presence of nanosilicates is from their proposed role as the source of the anomalous microwave emission (AME) (Hensley and Draine, 2017; Hoang et al., 2016). The AME is a broad foreground ISM feature centred at around 30 GHz which is most likely to originate from a population of fast spinning nanosized grains with a sufficiently large dipoles (Ali-Haïmoud et al., 2018). Quantum chemical modelling has shown that nanosilicates (unlike most carbonaceous species) have high inherent dipoles. As such, it would only require 1% of the available silicon dust mass to be in ultrasmall nanosilicates to make them a viable source of the AME (Maciá Escatller and Bromley, 2020). Similar studies have shown that this results still holds if we consider weak hydroxylation of the nanosilicates (Mariñoso Guiu et al., 2021b). The lack of distinct features in AME spectra means that a definite assignment to nanosilicates is difficult. However, for small molecular silicates with specific structures and high dipoles, the microwave emission spectrum would be more detailed and potentially assignable to specific species (e.g., pyroxene monomers) (Maciá Escatller and Bromley, 2020; Valencia et al., 2020). See Figure 1 for a comparison of microwave emission spectra expected for small molecular silicates and ultrasmall nanosilicates.
Interpretations of silicate dust IR observations typically employ laboratory-based measurements of bulk silicate samples. Such measurements confirm the typically-observed IR spectra of silicate dust characterised by two broad peaks at 9.8 μm (Si-O stretching mode) and 18–20 μm (O-Si-O bending mode) (Jäger et al., 2003b; Henning, 2010). Experimental IR spectra of laboratory produced silicate grains with diameters of a few 10 s of nm also show these characteristic modes (Ishizuka et al., 2015). For much smaller molecular silicates, IR spectra can also be obtained using cluster beam experiments. Here, the spectra exhibit sharp individual peaks which allow for accurate assignments of structure and composition via quantum chemical calculations. Clearly, between these two extremes there should be a size-dependent transition of the IR spectra of silicate dust.
Calculations suggest that the emergence of bulk-like double-peaked IR spectra should emerge for non-porous nanosilicate grains with diameters ≥5 nm (Zamirri et al., 2019), or upon aggregation of ultrasmall nanosilicates (Rimola and Bromley, 2021). This implies that the IR spectra of smaller nanosilicates should have specific non-bulk-like IR spectra, as explicitly confirmed by accurate quantum chemical modelling for ultrasmall nanosilicates. A summary of the size-dependency of IR spectra of small silicates is shown in Figure 1. As populations of ultrasmall silicates are expected to be extremely numerous, their cumulative IR spectra may be observationally discernible from the dominant IR signal of larger silicates. We note that the highly sensitive spectrometers of the Mid-IR instrument (MIRI) on the James Webb Space telescope (JWST) are well suited to probe the signature wavelength range for silicates (i.e., approximately between 8–20 μm). Using accurately calculated individual IR spectra of 10 s of ultrasmall nanosilicates, characteristic IR spectra of populations of ultrasmall nanosilicates with pyroxene and olivine compositions have been produced. Assuming that the silicate dust population has 3%–10% of its mass of Si in ultrasmall nanosilicates, several small but distinct influences on the bulk-like silicate IR spectra are predicted (e.g., increased intensity slightly above and below 9.8 μm) (Zeegers et al., 2023). In principle, such subtle effects should be detectable with JWST observations using MIRI, which should thus be able to confirm and/or place limits on the nanosilicate content of the diffuse ISM.
In summary, we highlight the importance of molecular silicates and nanosilicates in the formation and processing of larger silicate dust grains. It is expected that these ultrasmall species should be prevalent in the ISM where their astrochemical influence could be highly significant. While currently lacking direct observational conformation of nanosilicates, we show the role that atomistic bottom-up computational modelling, often together with laboratory experiments, can play in providing detailed insights into these species. Looking to the future, we expect that the combination of computational modelling and detailed observations will be indispensable for confirming the presence and assessing the abundance of nanosilicates in the ISM and other astrophysical environments.
SB: Conceptualization, Funding acquisition, Writing–original draft, Writing–review and editing.
The author(s) declare that financial support was received for the research, authorship, and/or publication of this article. Financial support from the Spanish Ministerio de Ciencia e Innovación and Agencia Estatal de Investigación (AEI) MCIN/AEI/10.13039/501100011033 through projects PID2021-127957NB-I00 and TED2021-132550B-C21 and CEX2021-001202-M (Maria de Maeztu program for Spanish Structures of Excellence) and from the Generalitat de Catalunya through project grant 2021SGR00354 are all acknowledged.
The author declares that the research was conducted in the absence of any commercial or financial relationships that could be construed as a potential conflict of interest.
The author(s) declared that they were an editorial board member of Frontiers, at the time of submission. This had no impact on the peer review process and the final decision.
All claims expressed in this article are solely those of the authors and do not necessarily represent those of their affiliated organizations, or those of the publisher, the editors and the reviewers. Any product that may be evaluated in this article, or claim that may be made by its manufacturer, is not guaranteed or endorsed by the publisher.
Ali-Haïmoud, Y., Barr, A., Battistelli, E. S., Bell, A., Bernstein, L., Casassus, S., et al. (2018). The state-of-play of anomalous microwave emission (AME) research, new astron. New.Astron. Rev 80, 1–28. doi:10.1016/j.newar.2018.02.001
Andersson, S., Gobrecht, D., and Valero, R. (2023). Mechanisms of SiO oxidation: implications for dust formation. Front. Astron. Space Sci. 10, 1135156. doi:10.3389/fspas.2023.1135156
Bradley, J. P. (1994). Chemically anomalous, preaccretionally irradiated grains in interplanetary dust from comets. Science 265, 925–929. doi:10.1126/science.265.5174.925
Bringa, E. M., Kucheyev, S. O., Loeffler, R. A., Baragiola, R. A., Tielens, A., Da, Z. R., et al. (2007). Energetic processing of interstellar silicate grains by cosmic rays. Astrophys. J. 662, 372–378. doi:10.1086/517865
Bromley, S. T., Gómez Martín, J. C., and Plane, J. C. (2016). Under what conditions does (SiO)N nucleation occur? A bottom-up kinetic modelling evaluation. Phys. Chem. Chem. Phys. 18, 26913–26922. doi:10.1039/c6cp03629e
Bromley, S. T., Goumans, T. P. M., Herbst, E., Jones, A. P., and Slater, B. (2014). Challenges in modelling the reaction chemistry of interstellar dust. Phys. Chem. Chem. Phys. 16, 18623. doi:10.1039/c4cp00774c
Bromley, S. T., Moreira, I., de, P. R., Neyman, K. M., and Illas, F. (2009). Approaching nanoscale oxides: models and theoretical methods. Chem. Soc. Rev. 38, 2657–2670. doi:10.1039/b806400h
Camps, P., Misselt, K., Bianchi, S., Lunttila, T., Pinte, C., Natale, G., et al. (2015). Benchmarking the calculation of stochastic heating and emissivity of dust grains in the context of radiative transfer simulations. Astron. Astrophys. 580, A87. doi:10.1051/0004-6361/201525998
Chen, L.-F., Chang, Q., and Xi, H.-W. (2018). Effect of stochastic grain heating on cold dense clouds chemistry. Mon. Not. R. Astron. Soc. 479, 2988–3001. doi:10.1093/mnras/sty1525
Cuppen, H. M., Morata, O., and Herbst, E. (2006). Monte Carlo simulations of H2 formation on stochastically heated grains. Mon. Not. R. Astron. Soc. 367, 1757–1765. doi:10.1111/j.1365-2966.2006.10079.x
de Donato, A. A., Ghejan, B.-A., Bakker, J. M., Bernhardt, T. M., Bromley, S. T., and Lang, S. M. (2024). Gas-phase production of hydroxylated silicon oxide cluster cations: structure, infrared spectroscopy, and astronomical relevance. ACS Earth Space Chem. 8, 1154–1164. doi:10.1021/acsearthspacechem.3c00346
Demyk, K., Carrez, Ph., Leroux, H., Cordier, P., Jones, A. P., Borg, J., et al. (2001). Structural and chemical alteration of crystalline olivine under low energy He+ irradiation. Astron. Astrophys. 368, L38–L41. doi:10.1051/0004-6361:20010208
Demyk, K., Jones, A. P., Dartois, E., Cox, P., and d´Hendecourt, L. (1999). The chemical composition of the silicate dust around RAFGL7009S and IRAS 19110+1045 Astron. Astrophys. 349, 267–275.
Djouadi, Z., Robert, F., Le Sergeant d´Hendecourt, L., Mostefaoui, S., Leroux, H., Jones, A. P., et al. (2011). Hydroxyl radical production and storage in analogues of amorphous interstellar silicates: a possible “wet” accretion phase for inner telluric planets. Astron. Astrophys. 531, A96. doi:10.1051/0004-6361/201116722
Dorschner, J., Begemann, B., Henning, T., Jäger, C., and Mutschke, H. (1995). Steps toward interstellar silicate mineralogy. II. Study of Mg-Fe-silicate glasses of variable composition. Astron. Astrophys. 300, 503–520.
Draine, B. T. (2003). Interstellar dust grains. Annu. Rev. Astron. Astrophys. 41, 241–289. doi:10.1146/annurev.astro.41.011802.094840
Draine, B. T., and Li, A. (2001). Infrared emission from interstellar dust. I. Stochastic heating of small grains. Astrophys. J. 551, 807–824. doi:10.1086/320227
Duley, W. W. (1973). Fluctuations in interstellar grain temperatures. Astrophys. Space Sci. 23, 43–50. doi:10.1007/bf00647650
Fogerty, S., Forrest, W., Watson, D. M., Sargent, B. A., and Koch, I. (2016). Silicate composition of the interstellar medium. Astrophys. J. 830, 71. doi:10.3847/0004-637x/830/2/71
Gail, H.-P., Scholz, M., and Pucci, A. (2016). Silicate condensation in Mira variables. Astron. Astrophys. J. 591, A17. doi:10.1051/0004-6361/201628113
Gail, H. P., and Sedlmayr, E. (1986). The primary condensation process for dust around late M-type stars. Astron. Astrophys. 166, 225–236.
Gail, H. P., and Sedlmayr, E. (1998). Inorganic dust formation in astrophysical environments. Faraday Discuss. 109, 303–319. doi:10.1039/a709290c
Gobrecht, D., Cherchneff, I., Sarangi, A., Plane, J. M. C., and Bromley, S. T. (2016). Dust formation in the oxygen-rich AGB star Ik Tauri. Astron. Astrophys. 585, A6. doi:10.1051/0004-6361/201425363
Gobrecht, D., Hashemi, S. R., Plane, J. M. C., Bromley, S. T., Nyman, G., and Decin, L. (2023). Bottom-up dust nucleation theory in oxygen-rich evolved stars II. Magnesium and calcium aluminate clusters. Astron. Astrophys. 680, A18. doi:10.1051/0004-6361/202347546
Gobrecht, D., Plane, J. M. C., Bromley, S. T., Decin, L., Cristallo, S., and Sekaran, S. (2022). Bottom-up dust nucleation theory in oxygen-rich evolved stars I. Aluminium oxide clusters. Astron. Astrophys. 658, A167. doi:10.1051/0004-6361/202141976
González Delgado, D., Olofsson, H., Kerschbaum, F., Schöier, F. L., Lindqvist, M., and Groenewegen, M. A. T. (2003). “Thermal” SiO radio line emission towards M-type AGB stars: a probe of circumstellar dust formation and dynamics. Astron. Astrophys. 411, 123–147. doi:10.1051/0004-6361:20031068
Goumans, T. P. M., and Bromley, S. T. (2011). Hydrogen and oxygen adsorption on a nanosilicate – a quantum chemical study. Mon. Not. R. Astron. Soc. 141, 1285–1291. doi:10.1111/j.1365-2966.2011.18463.x
Goumans, T. P. M., and Bromley, S. T. (2012). Efficient nucleation of stardust silicates via heteromolecular homogeneous condensation. Mon. Not. R. Astron. Soc. 420, 3344–3349. doi:10.1111/j.1365-2966.2011.20255.x
Goumans, T. P. M., and Bromley, S. T. (2013). Stardust silicate nucleation kick-started by SiO+TiO2. Phil. Trans. R. Soc. A 371, 20110580. doi:10.1098/rsta.2011.0580
Henning, T. (2010). Cosmic silicates. Annu. Rev. Astron. Astrophys. 48, 21–46. doi:10.1146/annurev-astro-081309-130815
Hensley, B. S., and Draine, B. T. (2017). Modeling the anomalous microwave emission with spinning nanoparticles: No PAHs required. Astrophys. J. 836, 179–192. doi:10.3847/1538-4357/aa5c37
Hoang, T., Vinh, N.-A., and Lan, N. Q. (2016). Spinning dust emission from ultra-small silicates: emissivity and polarization spectrum. Astrophys. J. 824, 18–28. doi:10.3847/0004-637x/824/1/18
Imai, H., Yasumori, M., Hirashima, H., Awazu, K., and Onuki, H. (1996). Significant densification of sol-gel derived amorphous silica films by vacuum ultraviolet irradiation. J. Appl. Phys. 79, 8304–8309. doi:10.1063/1.362541
Ishizuka, Y., Kimura, I., and Sakon, (2015). In-situ infrared measurements of free-flying silicate during condensation in the laboratory. Astrophysical J. 803, 88. doi:10.1088/0004-637x/803/2/88
Jager, C., Dorschner, J., Mutschke, H., Posch, Th., and Henning, Th. (2003b). Steps toward interstellar silicate mineralogy: VII. Spectral properties and crystallization behaviour of magnesium silicates produced by the sol-gel method. Astron. Astrophys. 408, 193–204. doi:10.1051/0004-6361:20030916
Jäger, C., Fabian, D., Schrempel, F., Dorschner, J., Henning, Th., and Wesch, W. (2003a). Structural processing of enstatite by ion bombardment. Astron. Astrophys. 401, 57–65. doi:10.1051/0004-6361:20030002
Jelfs, K. E., Flikkema, E., and Bromley, S. T. (2013). Hydroxylation of silica nanoclusters (SiO2)M(H2O)N, M = 4, 8, 16, 24: stability and structural trends. Phys. Chem. Chem. Phys. 15, 20438. doi:10.1039/c3cp53347f
Jeong, K. S., Winters, J. M., Le Bertre, T., and Sedlmayr, E. (2003). Self-consistent modeling of the outflow from the O-rich Mira IRC –20197. Astron. Astrophys. 407, 191–206. doi:10.1051/0004-6361:20030693
Jones, A. P., and Nuth, J. A. (2011). Dust destruction in the ISM: a re-evaluation of dust lifetimes. Astron. Astrophys. 530, A44. doi:10.1051/0004-6361/201014440
Jones, A. P., Tielens, A. G. G. M., and Hollenbach, D. J. (1996). Grain shattering in shocks: the interstellar grain size distribution. Astrophys. J. 469, 740. doi:10.1086/177823
Jones, A. P., Tielens, A. G. G. M., Hollenbach, D. J., and McKee, C. F. (1994). Grain destruction in shocks in the interstellar medium. Astrophys. J. 433, 797. doi:10.1086/174689
Keller, L. P., and McKay, D. S. (1997). The nature and origin of rims on lunar soil grains. Geochim. Cosmochim. Acta 61, 2331–2341. doi:10.1016/s0016-7037(97)00085-9
Kemper, F., Vriend, W. J., and Tielens, A. (2004). The absence of crystalline silicates in the diffuse interstellar medium. Astrophys. J. 609, 826–837. doi:10.1086/421339
Kerkeni, B., Bacchus-Montabonel, M.-C., and Bromley, S. T. (2017). How hydroxylation affects hydrogen adsorption and formation on nanosilicates. Mol. Astrophys. 7, 1–8. doi:10.1016/j.molap.2017.04.001
Kerkeni, B., Bacchus-Montabonel, M. C., Shan, X., and Bromley, S. T. (2019). Understanding H2 formation on hydroxylated pyroxene nanoclusters: ab initio study of reaction energetics and kinetics. J. Phys. Chem. A 123, 9282–9291. doi:10.1021/acs.jpca.9b06713
Kerkeni, B., and Bromley, S. T. (2013). Competing mechanisms of catalytic H2 formation and dissociation on ultrasmall silicate nanocluster dust grains. Mon. Not. R. Astron. Soc. 435, 1486–1492. doi:10.1093/mnras/stt1389
Kimura, Y., Tanaka, K. K., Inatomi, Y., Ferguson, F. T., and Nuth, J. A. (2022). Inefficient growth of SiOx grains: implications for circumstellar outflows. Astrophys. J. Lett. 934, L10. doi:10.3847/2041-8213/ac8002
Krasnokutski, S. A., Rouille, G., Jäger, C., Huisken, F., Zhukovska, S., and Henning, Th. (2014). Formation of silicon oxide grains at low temperature. Astrophys. J. 782, 15. doi:10.1088/0004-637x/782/1/15
Li, A., and Draine, B. T. (2001). On ultrasmall silicate grains in the diffuse interstellar medium. Astrophys. J. 550, L213–L217. doi:10.1086/319640
Li, M. P., Zhao, G., and Li, A. G. (2007). On the crystallinity of silicate dust in the interstellar medium. Mon. Not. R. Astron. Soc. 382, L26–L29. doi:10.1111/j.1745-3933.2007.00382.x
Macià Escatllar, A., and Bromley, S. T. (2020). Assessing the viability of silicate nanoclusters as carriers of the anomalous microwave emission: a quantum mechanical study. Astron. Astrophys. 634, A77. doi:10.1051/0004-6361/201936419
Macià Escatllar, A., Ugliengo, P., and Bromley, S. T. (2017). Modeling hydroxylated nanosilica: testing the performance of ReaxFF and FFSiOH force fields. J. Chem. Phys. 146, 224704. doi:10.1063/1.4985083
Macià Escatller, A., Lazaukas, T., Woodley, S. M., and Bromley, S. T. (2019). Structure and properties of nanosilicates with olivine (Mg2SiO4)N and pyroxene (MgSiO3)N compositions. ACS Earth Space Chem. 3, 2390–2403. doi:10.1021/acsearthspacechem.9b00139
Mariñoso Guiu, J., and Bromley, S. T. (2022). Efficiency of interstellar nanodust heating: accurate bottom-up calculations of nanosilicate specific heat capacities. J. Phys. Chem. A 126, 3854–3862. doi:10.1021/acs.jpca.2c02199
Mariñoso Guiu, J., Ferrero, S., Macià Escatllar, A., Rimola, A., and Bromley, S. T. (2021b). Does processing or formation of water ice mantles affect the capacity of nanosilicates to be the source of anomalous microwave emission? Front. Astron. Space Sci. 8, 676548. doi:10.3389/fspas.2021.676548
Mariñoso Guiu, J., Ghejan, B.-A., Bernhardt, T. M., Bakker, J. M., Lang, S. M., and Bromley, S. T. (2022). Cluster beam study of (MgSiO3)+-based monomeric silicate species and their interaction with oxygen: implications for interstellar astrochemistry. ACS Earth Space Chem. 6 (10), 2465–2470. doi:10.1021/acsearthspacechem.2c00186
Mariñoso Guiu, J., Macià Escatllar, A., and Bromley, S. T. (2021a). How does temperature affect the infrared vibrational spectra of nanosized silicate dust? ACS Earth Space Chem. 5 (4), 812–823. doi:10.1021/acsearthspacechem.0c00341
Mathis, J. S., Rumple, W., and Nordsieck, K. H. (1977). The size distribution of interstellar grains. Astrophys. J. 217, 425. doi:10.1086/155591
Min, M., Waters, L. B. F. M., de Koter, A., Hovenier, J. W., Keller, L. P., and Markwick-Kemper, F. (2007). The shape and composition of interstellar silicate grains. Astron. Astrophys. 46, 667–676. doi:10.1051/0004-6361:20065436
Molster, F. J., Waters, L. B. F. M., Tielens, A. G. G. M., Koike, C., and Chihara, H. (2002). Crystalline silicate dust around evolved stars III. A correlations study of crystalline silicate features. Astron. Astrophys. 382, 241–255. doi:10.1051/0004-6361:20011552
Oueslati, I., Kerkeni, B., and Bromley, S. T. (2015). Trends in the adsorption and reactivity of hydrogen on magnesium silicate nanoclusters. Phys. Chem. Chem. Phys. 17, 8951–8963. doi:10.1039/c4cp05128a
Plane, J. M. C. (2013). On the nucleation of dust in oxygen-rich stellar outflows. Phil. Trans. R. Soc. A 371, 20120335. doi:10.1098/rsta.2012.0335
Potapov, A., Jäger, C., Mutschke, H., and Henning, T. (2024). Trapped water on silicates in the laboratory and in astrophysical environments. Astrophys. J. 965, 48. doi:10.3847/1538-4357/ad2c07
Rimola, A., and Bromley, S. T. (2021). Formation of interstellar silicate dust via nanocluster aggregation: insights from quantum chemistry simulations. Front. Astron. Space Sci. 8, 659494. doi:10.3389/fspas.2021.659494
Sarangi, A., and Cherchneff, I. (2013). The chemically controlled synthesis of dust in type II-P supernovae. Astrophys. J. 776, 107–126. doi:10.1088/0004-637x/776/2/107
Saunders, R. W., and Plane, J. M. C. (2006). A laboratory study of meteor smoke analogues: composition, optical properties and growth kinetics. J. Atmos. Solar-Terr. Phys. 68, 2182–2202. doi:10.1016/j.jastp.2006.09.006
Slavin, J. D., Dwek, E., and Jones, A. P. (2015). Destruction of interstellar dust in evolving supernova remnant shock waves. Astrophys. J. 803, 7. doi:10.1088/0004-637x/803/1/7
Valencia, E. M., Worth, C. J., and Fortenberry, R. C. (2020). Enstatite (MgSiO3) and forsterite (Mg2SiO4) monomers and dimers: highly detectable infrared and radioastronomical molecular building blocks. Mon. Not. R. Astron. Soc. 492, 276–282. doi:10.1093/mnras/stz3209
van Dishoeck, E. F. (2014). Astrochemistry of dust, ice and gas: introduction and overview. Faraday Discuss. 168, 9–47. doi:10.1039/c4fd00140k
Weingartner, J. C., and Draine, B. T. (2001). Photoelectric emission from interstellar dust: grain charging and gas heating. Astrophys. J. Suppl. Ser. 134, 263–281. doi:10.1086/320852
Whittet, D. C. B. (2010). Oxygen depletion in the interstellar medium: implications for grain models and the distribution of elemental oxygen. Astrophys. J. 710, 1009–1016. doi:10.1088/0004-637x/710/2/1009
Zamirri, L., Macià Escatllar, A., Mariñoso Guiu, J., Ugliengo, P., and Bromley, S. T. (2019). What can infrared spectra tell us about the crystallinity of nanosized interstellar silicate dust grains? ACS Earth Space Sci. 3, 2323–2338. doi:10.1021/acsearthspacechem.9b00157
Zeegers, S. T., Guiu, J. M., Kemper, F., Marshall, J. P., and Bromley, S. T. (2023). Predicting observable infrared signatures of nanosilicates in the diffuse interstellar medium. Faraday Discuss. 245, 609–619. doi:10.1039/d3fd00055a
Zeller, E. J., Ronca, L. B., and Levy, P. W. (1966). Proton-induced hydroxyl formation on the lunar surface. J. Geophys. Res. 71, 4855–4860. doi:10.1029/jz071i020p04855
Keywords: cosmic nanosilicates, interstellar dust, ultrasmall grains, circumstellar nucleation, astrophysical dust processing, IR spectra, microwave emission
Citation: Bromley ST (2024) Nanosilicates and molecular silicate dust species: properties and observational prospects . Front. Astron. Space Sci. 11:1523977. doi: 10.3389/fspas.2024.1523977
Received: 06 November 2024; Accepted: 25 November 2024;
Published: 17 December 2024.
Edited by:
Cristina Puzzarini, University of Bologna, ItalyCopyright © 2024 Bromley. This is an open-access article distributed under the terms of the Creative Commons Attribution License (CC BY). The use, distribution or reproduction in other forums is permitted, provided the original author(s) and the copyright owner(s) are credited and that the original publication in this journal is cited, in accordance with accepted academic practice. No use, distribution or reproduction is permitted which does not comply with these terms.
*Correspondence: Stefan T. Bromley, cy5icm9tbGV5QHViLmVkdQ==
Disclaimer: All claims expressed in this article are solely those of the authors and do not necessarily represent those of their affiliated organizations, or those of the publisher, the editors and the reviewers. Any product that may be evaluated in this article or claim that may be made by its manufacturer is not guaranteed or endorsed by the publisher.
Research integrity at Frontiers
Learn more about the work of our research integrity team to safeguard the quality of each article we publish.