- 1Institute for Frontiers in Astronomy and Astrophysics, Beijing Normal University, Beijing, China
- 2School of Physics and Astronomy, Beijing Normal University, Beijing, China
- 3Key Laboratory of Stars and Interstellar Medium, Department of Physics, Xiangtan University, Xiangtan, Hunan, China
- 4International Space Science Institute, Bern, Switzerland
- 5Physikalisches Institut, University of Bern, Bern, Switzerland
This study comprehensively analyzes type I X-ray bursts observed by Swift/BAT from 2005 to April 2024 to search for X-ray burst oscillations (XBOs) in neutron star low-mass X-ray binaries. XBOs, periodic signals detected within type I X-ray bursts, typically range from 11 to 620 Hz and are often observed in the soft X-ray data of these bursts. Using the high-sensitivity and precise timing capabilities of the Swift/BAT, we found 50 type I X-ray bursts from 37 neutron star low-mass X-ray binaries. We conducted a detailed timing analysis of these bursts. For sources with known burst oscillation frequencies, our findings largely corroborate previous studies. However, many sources displayed low confidence levels in the oscillation signals, with
1 Introduction
The X-ray emissions from low-mass X-ray binaries (LMXBs) arise from the accretion processes surrounding compact objects such as neutron stars (NS) or black holes. In the case of NS, the accreted hydrogen, helium, or mixture of them can be consumed via unstable nuclear burning on the stellar surface, leading to the observed type I X-ray bursts (Galloway et al., 2008).
X-ray burst oscillations (XBOs), identified through timing analysis, are periodic signals observed during bursts originating from the NS rotation (Strohmayer et al., 1996; Galloway and Keek, 2021). A type I X-ray burst can form a hot spot on the NS surface, leading to an uneven temperature distribution. The star’s rotation modulates this uneven distribution, producing periodic signals in the soft X-ray band (Strohmayer et al., 1997b; Goodwin et al., 2021). The standard methods for XBO detection include a fast Fourier transform (FFT) or
However, the mechanisms behind the formation and evolution of these hot spots remain incompletely understood. Proposed surface modes, such as the Rossby model, suggest that type I X-ray bursts can excite oscillations in the NS ocean (Chambers and Watts, 2020). A temperature gradient forms at different heights on the star’s surface, and the star’s rotation modulates this gradient to produce XBOs (Cumming and Bildsten, 2000; Watts, 2012; Mahmoodifar and Strohmayer, 2016). Nonetheless, these models only partially explain the observed XBO phenomena, necessitating further study into their physical processes and model interpretations.
Detecting XBO signals requires high-energy X-ray telescopes with high timing resolution, large effective areas for substantial photon accumulation, and minimal dead-time effects. Previous searches for XBO signals have utilized data from RXTE (Strohmayer, 1999; 2001; Bilous and Watts, 2019) and NICER (Mahmoodifar et al., 2018; Li et al., 2022). Observations have shown that oscillation signals in most bursts exhibit an upward frequency drift and generally occur during the burst’s tail. In some burst samples, oscillation signals have such high amplitudes that the accretion pulsar’s oscillating frequency diverges from the pulsar’s rotation frequency by a few hertz (e.g., Chakrabarty et al., 2003).
Up until now, 349 galactic NS LMXBs have been found and the number is increasing (Avakyan et al., 2023). However, less than 10% of these sources have had their spin frequency and burst oscillation measured. Detecting burst oscillations from sources with unknown spin frequencies, or from newly discovered sources, will expand the sample of accreting pulsars (Patruno et al., 2017). This will allow for a more credible study of the spin frequency distribution, and for searching coherent X-ray pulsation during outbursts. Additionally, among these unknown sources, there may be neutron stars spinning faster than 716 Hz, which would impose stronger constraints on the equation of state of compact stellar objects.
In’t Zand et al. (2019) carried out a comprehensive searching and spectral analysis of type I X-ray bursts from Galactic NSs observed by Swift BAT/XRT. They identified 28 X-ray bursts for which BAT event data were available. In this paper, we search the Swift/BAT archives for triggered events of LMXBs from 2005 to April 2024 and perform timing analysis to search burst oscillation. We introduce the observations and data analysis methods in Sect. 2. We provide the results of the type I X-ray burst oscillation in Sect. 3. In Sect. 4, we discuss and summarize the results.
2 Observations and data analysis
The Swift/BAT triggering system is designed to identify gamma-ray bursts (GRBs) and other fast transients in high energy from various sources, including black holes, NSs, and magnetars. In this study, we found 50 non-GRB events from the GCN Notice Archive. These events are from 37 distinct sources, with 22 triggers related to NS LMXBs exhibiting X-ray burst oscillations or coherent pulsations, and the remaining samples involving NS LMXBs without known spin frequency. The absolute timing accuracy, 0.1 m of the recorded event files allows us to perform timing analysys. The Swift/BAT data were processed using the batgrbproduct command, and 1-s binned light curves were extracted in the 13–20 keV energy band. Subsequently, the batbinevt command was employed to transform the event data for each X-ray burst into a mask-weighted (background-subtracted) light curve in the 13–20 keV band. The burst peak flux and duration were determined by analyzing these light curves, with the duration,
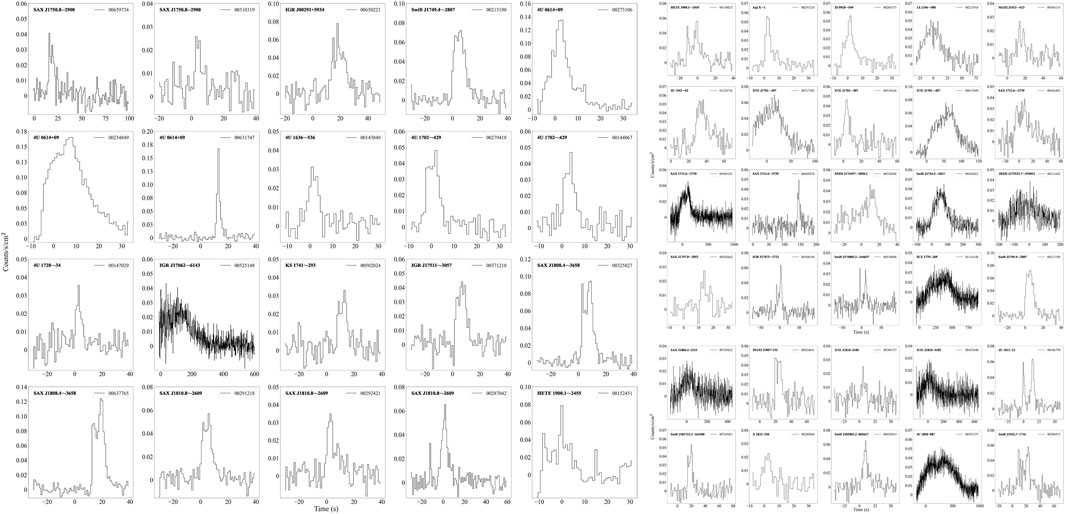
Figure 1. BAT light curves of all 50 X-ray bursts. In each panel, the start time is the trigger of the X-ray burst from in’t Zand et al. (2019) and https://gcn.gsfc.nasa.gov/swift_grbs.html. The source name and the trigger number are shown in each panel.,
Type I X-ray burst sources were categorized based on the presence or absence of previously detected XBOs or coherent pulsations. For the sources without known spin frequency, our analysis of bursts is explained as follows.
Initially, we applied FFT to the event files from each burst, segmenting the analysis into 4-s intervals with a step size of 0.5 s. For each window, statistically independent FFT was recorded between 10 and 2000 Hz with a step of 0.25 Hz. The light curve of duration
where
Oscillation signal confirmation is applied using
where
where
For sources with previously identified XBOs or coherent pulsations, a dynamic power spectrum was generated by applying the
We evaluated the confidence level for all burst oscillation signals. The total number of trials is
In addition to the oscillation frequency, the power spectrum contains information about the pulse amplitude. For the Leahy-normalized power spectrum, the root mean square (RMS) amplitude is defined as follows,
where
3 Results
Based on Equations 1–4, we carried out the XBO searching. The results of the two categories of burst sources are summarized in Tables 1, 2. Definitions of burst parameters follow those provided by in’t Zand et al. (2019).
3.1 Individual sources with detected XBO or coherent pulsation
For sources with previously detected XBOs or coherent pulsations, we analyzed the bursts to determine the maximum
HETE 1900.1
4U 1702
4U 0614 + 09. 4U 0614 + 09 is an X-ray burster and persistent low-mass X-ray binary (LMXB) located in the direction of the anti-galactic center at a distance of approximately 3.2 kpc (Kuulkers et al., 2010). Strohmayer et al. (2008) detected an XBO from 4U 0614 + 09 at 414.75 Hz in the tail of one of two bursts. This was the first XBO found using Swift/BAT, suggesting a spin frequency of
IGR J17062
3.2 Sources without previously detected XBOs
We perform FFT timing analysis for sources without known spin frequency by selecting photon arrival times within the 13–20 keV energy band to identify potential burst oscillation signals. The FFT powers for 11 LMXBs are shown below.
2S 0918
MAXI J1421
XTE J1701
XMM J174457
Swift J1734.5
IGR J17473
Swift J174805.3
SLX 1735
MAXI J1807 + 132. MAXI J1807 + 132 is an X-ray transient discovered by the nova-search system of MAXI on 13 March 2017 (Negoro et al., 2017). Swift observed a type I X-ray burst from this source in 2015. FFT processing indicated a suspected oscillation signal at 226.26 Hz, with a maximum
Swift J181723.1
X 1832
4 Discussion
This study provides an extensive analysis of Swift/BAT observations of NS LMXBs over nearly two decades, from 2005 to April 2024. Our dataset comprised 50 type I X-ray bursts, with 21 previously analyzed for burst oscillation signals and 29 newly found. The analysis focused on both sources with previously detected XBOs or coherent pulsations and sources without prior detections, applying FFT and
For the bursts with known oscillation signals, our analysis confirmed previously reported results, such as the case of 4U 0614 + 09 in 2007 exhibited a significant oscillation signal (Strohmayer et al., 2008). However, most of the bursts showed
For sources without known spin frequencies, we employed FFT analysis to search for burst oscillation signals. The detected oscillation candidates spanned a wide frequency range from 40 to 950 Hz, with maximum
While theoretical models exist to explain X-ray burst oscillations (XBOs), they do not fully account for all observed phenomena. This highlights the necessity for continued observational efforts, enhanced theoretical models, and comprehensive analyses. Recent advancements in observational technology and analytical methodologies have considerably improved our capability to detect and analyze XBOs.
Future research will benefit from combining data from multiple observatories and utilizing advanced statistical methods to enhance the sensitivity and reliability of burst oscillation detection. Considering the low occurrence rate of burst oscillations, observing more bursts will help increase the number of burst oscillation detection. The anticipated contributions from next-generation telescopes, such as eXTP, THESEUS, ATHENA (Zhang et al., 2019; Amati et al., 2018; Barret et al., 2018), are expected to significantly enhance our observational capabilities. These telescopes, equipped with instruments featuring large effective collecting areas, wide fields of view, and high timing accuracy in the soft X-ray band, will allow for more precise measurements of neutron star properties. These advantages will enable more precise measurements of NS properties, thereby providing tighter constraints on the equation of state of dense matter. Such efforts are providing unprecedented opportunities to study XBOs with greater precision and are essential for refining theoretical models of XBOs and advancing our understanding of these complex phenomena.
Data availability statement
The original contributions presented in the study are included in the article/supplementary material, further inquiries can be directed to the corresponding author.
Author contributions
Q-XL: Writing–original draft, Writing–review and editing. ZL: Writing–original draft, Writing–review and editing. Y-YP: Writing–original draft, Writing–review and editing. MF: Writing–review and editing.
Funding
The author(s) declare that financial support was received for the research, authorship, and/or publication of this article. This work was supported by the Major Science and Technology Program of Xinjiang Uygur Autonomous Region (No. 2022A03013-3) and the National Key R&D Pro-gram of China (2021YFA0718500), and National Natural Science Foundation of China (Nos., 12103042, 12273030).
Conflict of interest
The authors declare that the research was conducted in the absence of any commercial or financial relationships that could be construed as a potential conflict of interest.
Publisher’s note
All claims expressed in this article are solely those of the authors and do not necessarily represent those of their affiliated organizations, or those of the publisher, the editors and the reviewers. Any product that may be evaluated in this article, or claim that may be made by its manufacturer, is not guaranteed or endorsed by the publisher.
References
Altamirano, D., Degenaar, N., Zand, J., Markwardt, C., and Wijnands, R. (2008) Swift/XRT follow-up observations of IGR J17473-2721, 1459. The Astronomer’s Telegram, 1.
Altamirano, D., Watts, A., Linares, M., Markwardt, C. B., Strohmayer, T., and Patruno, A. (2010). Type I X-ray bursts and burst oscillations in the accreting millisecond X-ray pulsar IGR J17511-3057. Mon. Notices R. Astronomical Soc. 409, 1136–1145. doi:10.1111/j.1365-2966.2010.17369.x
Amati, L., O’Brien, P., Götz, D., Bozzo, E., Tenzer, C., Frontera, F., et al. (2018). The THESEUS space mission concept: science case, design and expected performances. Adv. Space Res. 62, 191–244. doi:10.1016/j.asr.2018.03.010
Avakyan, A., Neumann, M., Zainab, A., Doroshenko, V., Wilms, J., and Santangelo, A. (2023). XRBcats: galactic low-mass X-ray binary catalogue. XRBcats Galact. low-mass X-ray binary Cat. 675, A199. doi:10.1051/0004-6361/202346522
Barret, D., Lam Trong, T., den Herder, J.-W., Piro, L., Cappi, M., Houvelin, J., et al. (2018). “The ATHENA X-ray integral field unit (X-IFU),” in Space telescopes and instrumentation 2018: ultraviolet to gamma ray. 10699 of Society of photo-optical instrumentation engineers (SPIE) conference series. Editors J.-W. A. den Herder, S. Nikzad, and K. Nakazawa doi:10.1117/12.2312409106991G
Barthelmy, S. D., Beardmore, A. P., Burrows, D. N., Cholden-Brown, A., Kuin, N. P. M., Lien, A. Y., et al. (2017). Swift triggers on a new galactic source: swift J181723.1-164300. GRB Coord. Netw. 21369 (1).
Bilous, A. V., and Watts, A. L. (2019). A uniform search for thermonuclear burst oscillations in the rxte legacy data set. Astrophysical J. Suppl. Ser. 245, 19. doi:10.3847/1538-4365/ab2fe1
Bilous, A. V., Watts, A. L., Galloway, D. K., and in ’t Zand, J. J. M. (2018). A millisecond oscillation in the bursting x-ray flux of sax j1810. 8–2609. Astrophysical J. Lett. 862, L4. doi:10.3847/2041-8213/aad09c
Bozzo, E., Romano, P., Falanga, M., Ferrigno, C., Papitto, A., and Krimm, H. A. (2015). Swift J1734.5-3027: a new long Type-I X-ray bursting source. Astronomy and Astrophysics 579, A56. doi:10.1051/0004-6361/201526150
Buccheri, R., Bennett, K., Bignami, G. F., Bloemen, J. B. G. M., Boriakoff, V., Caraveo, P. A., et al. (1983). Search for pulsed-ray emission from radio pulsars in the COS-B data. Astronomy and Astrophysics 128, 245–251.
Bult, P., Strohmayer, T. E., Malacaria, C., Ng, M., and Wadiasingh, Z. (2021). Long-term coherent timing of the accreting millisecond pulsar IGR j17062-6143. Astrophysical J. 912, 120. doi:10.3847/1538-4357/abf13f
Chakrabarty, D., Morgan, E. H., Muno, M. P., Galloway, D. K., Wijnands, R., van der Klis, M., et al. (2003). Nuclear-powered millisecond pulsars and the maximum spin frequency of neutron stars. Nature 424, 42–44. doi:10.1038/nature01732
Chambers, F. R. N., and Watts, A. L. (2020). Relativistic ocean r-modes during type-I X-ray bursts. Mon. Notices R. Astronomical Soc. 491, 6032–6044. doi:10.1093/mnras/stz3449
Chen, Y.-P., Li, J., Xiong, S.-L., Ji, L., Zhang, S., Peng, W.-X., et al. (2022). GECAM detection of a bright type I X-ray burst from 4U 0614+09: hint for its spin frequency at 413 hz. Astrophysical J. 935, 10. doi:10.3847/1538-4357/ac7ff8
Cumming, A., and Bildsten, L. (2000). Rotational evolution during type I X-ray bursts. Astrophysical J. 544, 453–474. doi:10.1086/317191
Degenaar, N., Wijnands, R., Reynolds, M. T., Miller, J. M., Altamirano, D., Kennea, J., et al. (2014). The peculiar galactic center neutron star X-ray binary XMM j174457-2850.3. Astrophysical J. 792, 109. doi:10.1088/0004-637X/792/2/109
Engel, M. C., Heinke, C. O., Sivakoff, G. R., Elshamouty, K. G., and Edmonds, P. D. (2012). A 2.15 hr orbital period for the low-mass X-ray binary XB 1832-330 in the globular cluster NGC 6652. Astrophysical J. 747, 119. doi:10.1088/0004-637X/747/2/119
Galloway, D. K., and Keek, L. (2021). Thermonuclear X-ray bursts. Berlin, Heidelberg: Springer Berlin Heidelberg, 209–262. doi:10.1007/978-3-662-62110-3_5
Galloway, D. K., Muno, M. P., Hartman, J. M., Psaltis, D., and Chakrabarty, D. (2008). Thermonuclear (type I) X-ray bursts observed by the rossi X-ray timing explorer. Astrophysical J. Suppl. Ser. 179, 360–422. doi:10.1086/592044
Goodwin, A. J., Heger, A., Chambers, F. R. N., Watts, A. L., and Cavecchi, Y. (2021). X-ray burst ignition location on the surface of accreting X-ray pulsars: can bursts preferentially ignite at the hotspot? Mon. Notices R. Astronomical Soc. 505, 5530–5542. doi:10.1093/mnras/stab1659
Hertz, P., and Wood, K. S. (1985). The nature of the low-luminosity globular cluster X-ray sources. Astrophysical J. 290, 171–184. doi:10.1086/162971
Juett, A. M., and Chakrabarty, D. (2003). X-ray spectroscopy of the low-mass X-ray binaries 2S 0918-549 and 4U 1543-624: evidence for neon-rich degenerate donors. Astrophysical J. 599, 498–508. doi:10.1086/379188
Kaaret, P., Morgan, E. H., Vanderspek, R., and Tomsick, J. A. (2006). Discovery of the millisecond X-ray pulsar HETE j1900.1-2455. Astrophysical J. 638, 963–967. doi:10.1086/498886
Kouveliotou, C., Meegan, C. A., Fishman, G. J., Bhat, N. P., Briggs, M. S., Koshut, T. M., et al. (1993). Identification of two classes of gamma-ray bursts. Astrophysical J. 413, L101. doi:10.1086/186969
Kuulkers, E., Zand, J. J. M., Atteia, J. L., Levine, A. M., Brandt, S., Smith, D. A., et al. (2010). What ignites on the neutron star of 4U 0614+091? Astronomy and Astrophysics 514, A65. doi:10.1051/0004-6361/200913210
Leahy, D. A., Darbro, W., Elsner, R. F., Weisskopf, M. C., Sutherland, P. G., Kahn, S., et al. (1983). On searches for pulsed emission with application to four globular cluster X-ray sources: NGC 1851, 6441, 6624 and 6712. Astrophysical J. 266, 160–170. doi:10.1086/160766
Li, Z., Yu, W., Lu, Y., Pan, Y., and Falanga, M. (2022). Discovery of a 584.65 Hz burst oscillation in the low-mass X-ray binary 4U 1730-22. Astrophysical J. 935, 123. doi:10.3847/1538-4357/ac85bb
Linares, M., Watts, A. L., Wijnands, R., Soleri, P., Degenaar, N., Curran, P. A., et al. (2009). The Swift capture of a long X-ray burst from XTE J1701-407. Mon. Notices R. Astronomical Soc. 392, L11–L15. doi:10.1111/j.1745-3933.2008.00572.x
Mahmoodifar, S., Keek, L., Strohmayer, T., Markwardt, C., Arzoumanian, Z., Gendreau, K., et al. (2018). NICER view of thermonuclear X-ray bursts and burst oscillations. 42nd COSPAR Sci. Assem. 42, E1.10–5–18.
Mahmoodifar, S., and Strohmayer, T. (2016). X-ray burst oscillations: from flame spreading to the cooling wake. Astrophysical J. 818, 93. doi:10.3847/0004-637X/818/1/93
Markwardt, C. B., Pereira, D., and Swank, J. H. (2008). New source discovered by RXTE PCA scans: XTE j1701-407. Astronomer’s Telegr. 1569, 1.
Markwardt, C. B., Strohmayer, T. E., and Swank, J. H. (1999). Observation of kilohertz quasi-periodic oscillations from the atoll source 4U 1702−429 by the [ITAL]rossi X-ray timing explorer[/ITAL]. Astrophysical J. 512, L125–L129. doi:10.1086/311886
Moutard, D., Ludlam, R., Buisson, D., Cackett, E., Degenaar, N., Fabian, A., et al. (2024). Investigating the ultra-compact X-ray binary candidate SLX 1735-269 with NICER and NuSTAR. arXiv e-prints 968, 51. arXiv:2401.12371doi. doi:10.3847/1538-4357/ad4a78
Muno, M. P., Chakrabarty, D., Galloway, D. K., and Psaltis, D. (2002). The frequency stability of millisecond oscillations in thermonuclear X-ray bursts. Astrophysical J. 580, 1048–1059. doi:10.1086/343793
Negoro, H., Kawamuro, T., Ueda, Y., Nakahira, S., Ueno, S., Tomida, H., et al. (2017). MAXI/GSC detection of a long-term soft X-ray flaring event MAXI J1807+132. Astronomer’s Telegr. 1, 10208.
Nobukawa, K. K., Nobukawa, M., and Yamauchi, S. (2023). Confirmation of dust scattering echo around MAXI J1421-613 by Swift observation. Adv. Space Res. 71, 1074–1079. doi:10.1016/j.asr.2022.09.003
Ootes, L. S., Watts, A. L., Galloway, D. K., and Wijnands, R. (2017). The accretion rate dependence of burst oscillation amplitude. Astrophysical J. 834, 21. doi:10.3847/1538-4357/834/1/21
Patruno, A., Haskell, B., and Andersson, N. (2017). The spin distribution of fast-spinning neutron stars in low-mass X-ray binaries: evidence for two subpopulations, Astrophys. J., 850, 106. doi:10.3847/1538-4357/aa927a
Roy, P., Beri, A., and Bhattacharyya, S. (2021). Thermonuclear X-ray bursts from 4U 1636 - 536 observed with AstroSat. Mon. Notices R. Astronomical Soc. 508, 2123–2133. doi:10.1093/mnras/stab2680
Sanna, A., Pintore, F., Bozzo, E., Ferrigno, C., Papitto, A., Riggio, A., et al. (2017). Spectral and timing properties of IGR J00291+5934 during its 2015 outburst. Mon. Notices R. Astronomical Soc. 466, 2910–2917. doi:10.1093/mnras/stw3332
Skinner, G. K., Willmore, A. P., Eyles, C. J., Bertram, D., Church, M. J., Harper, P. K. S., et al. (1987). Hard X-ray images of the galactic centre. Nature 330, 544–547. doi:10.1038/330544a0
Strohmayer, T., and Keek, L. (2017). IGR j17062-6143 is an accreting millisecond X-ray pulsar. Astrophysical J. 836, L23. doi:10.3847/2041-8213/aa5e51
Strohmayer, T. E. (1999). Oscillations during thermonuclear X-ray bursts: a new probe of neutron stars. arXiv e-prints. doi:10.48550/arXiv.astro-ph/9911338
Strohmayer, T. E. (2001). Oscillations during thermonuclear x-ray bursts. Adv. Space Res. 28, 511–522. doi:10.1016/S0273-1177(01)00422-7
Strohmayer, T. E., Jahoda, K., Giles, A. B., and Lee, U. (1997a). Millisecond pulsations from a low-mass X-ray binary in the galactic center region. Astrophysical J. 486, 355–362. doi:10.1086/304522
Strohmayer, T. E., and Markwardt, C. B. (2002). Evidence for a millisecond pulsar in 4U 1636-53 during a superburst. Astrophysical J. 577, 337–345. doi:10.1086/342152
Strohmayer, T. E., Markwardt, C. B., and Kuulkers, E. (2008). Discovery of the spin frequency of 4u 0614+ 09 with the swift burst alert telescope. Astrophysical J. 672, L37–L40. doi:10.1086/526546
Strohmayer, T. E., Zhang, W., and Swank, J. H. (1997b). 363 H[CLC]z[/CLC] oscillations during the rising phase of bursts from 4U 1728−34: evidence for rotational modulation. Astrophysical J. 487, L77–L80. doi:10.1086/310880
Strohmayer, T. E., Zhang, W., Swank, J. H., Smale, A., Titarchuk, L., Day, C., et al. (1996). Millisecond X-ray variability from an accreting neutron star system. Astrophysical J. 469, L9–L12. doi:10.1086/310261
Strohmayer, T. E., Zhang, W., Swank, J. H., White, N. E., and Lapidus, I. (1998). On the amplitude of burst oscillations in 4U 1636-54: evidence for nuclear-powered pulsars. Astrophysical J. 498, L135–L139. doi:10.1086/311322
Suzuki, M., Kawai, N., Tamagawa, T., Yoshida, A., Nakagawa, Y. E., Tanaka, K., et al. (2007). Discovery of a new X-ray burst/millisecond accreting pulsar, HETE j1900.1-2455. Publ. Astronomical Soc. Jpn. 59, 263–268. doi:10.1093/pasj/59.1.263
Vanderspek, R., Morgan, E., Crew, G., Graziani, C., and Suzuki, M. (2005). Possible new X-ray burst source detected by HETE. Astronomer’s Telegr. 516 (1).
Varun, M., Thomas, N. T., Gudennavar, S. B., and Bubbly, S. G. (2024). Spectral and type I X-ray burst studies of 4U 1702-429 using AstroSat observations. Mon. Notices R. Astronomical Soc. 529, 2234–2241. doi:10.1093/mnras/stae636
Watts, A. L. (2012). Thermonuclear burst oscillations. Annu. Rev. Astronomy Astrophysics 50, 609–640. doi:10.1146/annurev-astro-040312-132617
Watts, A. L., Altamirano, D., Markwardt, C. B., and Strohmayer, T. E. (2009). Discovery of 245 Hz burst oscillations from the accreting millisecond pulsar IGR J17511-3057. Astronomer’s Telegr. 2199, 1.
Zand, J. J. M., Cumming, A., van der Sluys, M. V., Verbunt, F., and Pols, O. R. (2005). On the possibility of a helium white dwarf donor in the presumed ultracompact binary 2S 0918-549. Astronomy and Astrophysics 441, 675–684. doi:10.1051/0004-6361:20053002
Zand, J. J. M., Kries, M. J. W., Palmer, D. M., and Degenaar, N. (2019). Searching for the most powerful thermonuclear X-ray bursts with the neil gehrels swift observatory. Astronomy and Astrophysics 621, A53. doi:10.1051/0004-6361/201834270
Zhang, S., Santangelo, A., Feroci, M., Xu, Y., Lu, F., Chen, Y., et al. (2019). The enhanced X-ray Timing and Polarimetry mission—eXTP. Sci. China Phys. Mech. Astronomy 62, 29502. doi:10.1007/s11433-018-9309-2
Zhang, W., Jahoda, K., Kelley, R. L., Strohmayer, T. E., Swank, J. H., and Zhang, S. N. (1998). Millisecond oscillations in the persistent and bursting flux of aquila X-1 during an outburst. Astrophysical J. 495, L9–L12. doi:10.1086/311210
Keywords: neutron star binaries, neutron star (NS), x-ray burst, burst oscillation, x-ray timing
Citation: Li Q-X, Li Z, Pan Y-Y and Falanga M (2024) Search for thermonuclear burst oscillations in the Swift/BAT data set. Front. Astron. Space Sci. 11:1477677. doi: 10.3389/fspas.2024.1477677
Received: 08 August 2024; Accepted: 23 September 2024;
Published: 08 October 2024.
Edited by:
Yongfeng Huang, Nanjing University, ChinaReviewed by:
Carlos Frajuca, Federal University of Rio Grande, BrazilAntonio Martin-Carrillo, University College Dublin, Ireland
Copyright © 2024 Li, Li, Pan and Falanga. This is an open-access article distributed under the terms of the Creative Commons Attribution License (CC BY). The use, distribution or reproduction in other forums is permitted, provided the original author(s) and the copyright owner(s) are credited and that the original publication in this journal is cited, in accordance with accepted academic practice. No use, distribution or reproduction is permitted which does not comply with these terms.
*Correspondence: Zhaosheng Li, bGl6aGFvc2hlbmdAeHR1LmVkdS5jbg==