- 1NASA Ames Research Center, Moffett Field, CA, United States
- 2Las Cumbres Observatory, Goleta, CA, United States
- 3Department of Physics, University of California, Santa Barbara, Santa Barbara, CA, United States
- 4Department of Astronomy, University of Wisconsin-Madison, Madison, WI, United States
- 5Department of Physics, Boise State University, Boise, ID, United States
In parallel with the multi-messenger revolution, major advances in time-domain astronomy across multiple science disciplines relevant to astrophysics are becoming more urgent to address. Aside from electromagnetic observations of gravitational wave events and explosive counterparts, there are a number of “classical” astrophysical areas that require new thinking for proper exploration in the time domain. How NASA, NSF, ESA, and ESO consider the 2020 USA Decadal Survey within the astronomy community, as well as the worldwide call to support and expand time domain and multi-messenger astrophysics, it is crucial that all areas of astrophysics, including stellar, galactic, Solar System, and exoplanetary science participate in the discussion, and that it not be made into an exclusive preserve of cosmological, high-energy, explosive and transient science. Time domain astronomy is used to explore many aspects of astrophysics–particularly concerning ground- and space-based mission science goals of exploring how the Universe works, understanding how did we get here, and are we alone. Time domain studies are already built into the core operations of many currently operating and future space telescopes (e.g., Roman, PLATO) as well as current and planned large areal ground-based surveys (e.g., Rubin). Time-domain observations designed for one scientific purpose, also lead to great discoveries in many other science areas. The recent advent of user-friendly hardware, software, observational approaches, and online data infrastructure has also helped make time domain observations especially suitable and appealing for citizen science projects. We provide a review of the current state of TDAMM alerts and observational protocols, revealing a wide array of software and applications, much of which is incompatible. Any conversation regarding TDAMM astrophysics should include all aspects of the field, including those aspects seen as classical applications.
1 Introduction
1.1 Time-domain astronomy
Time-Domain Astronomy (TDA) is not a novel concept. Astronomers have been making observations as a function of time for over a century. Even before that time, scientists such as Galileo Galilei (Standish and Nobili, 1997) and Tycho Brahe recorded observations of the sun, moon, stars, and supernovae over time, noting changes in their structures and positions.
However, Today, there seems to be an inherent bias in TDA, associating these observations predominantly with high-energy cosmological events that are often explosive and infrequent for any given object. However, before the term TDA was coined and added to the lexicon of astronomer-speak, observations in time existed, had other names, and involved all aspects of astronomy from Solar System objects to stars to AGN. Such observations were called time-series data, light curves, or phase-resolved spectroscopy to name a few. These temporal observations include periodic, quasi-periodic, and stochastic variations in brightness, spectrum, and/or position. Some published examples are given in Cortie (1915); Gordon and Kron (1947); Kollath (1990); Powell et al. (1969); Mason et al. (1982).
1.2 Multi-messenger astronomy
Likewise, Multi-messenger Astronomy (MMA) as used today, generally seems to require the inclusion of, or at least the possibility of, particles and gravitational waves to be a part of the messenger group. Multi-wavelength astronomy, from its humble beginnings of simultaneous or contemporaneous measurements covering a few wavelengths of light (e.g., optical and IR) moved into a golden era with the advent of UV and X-ray rocket observations, space telescopes, and simultaneous use of space and ground-based telescopes. All aspects of astrophysical research are involved in multi-messenger (wavelength) studies, some are time-sampled while others are static non-explosive phenomena. Solar System science might also be considered here, being the only branch of astrophysics where sample-return is a viable option. Some examples are Herbig (1970); Schulte-Ladbeck and Hopp (1990); Belle et al. (2005). Of course, multi-wavelength astronomy, in terms of multi-color observations, has been around for over 100 years as well, for example, Shapley (1920); Sandage et al. (1969).
2 Considerations for time domain and multi-messenger astronomy (TDAMM)
2.1 Planning across astrophysical science
In parallel with the multi-messenger revolution, major advances in time-domain astronomy across multiple science areas relevant to astrophysics are becoming more urgent to address. Aside from electromagnetic observations (EM) of Gravitational Wave (GW) events and particle measurements for explosive counterparts, there are a number of “classical” astrophysics areas that require new thinking for proper exploration in the time domain. As the astronomy community and NASA, in particular, consider the 2020 USA Decadal Survey’s recommendations to support and expand time domain and multi-messenger astrophysics,1 it is crucial that all areas of astrophysics, including stellar, galactic, Solar System, and exoplanetary, participate in the discussion, and that it not be made into an exclusive preserve of high-energy, GW, and transient explosive cosmological science. For example, the NASA Kepler mission was designed for exoplanet transit observations, but excelled as well in other astrophysical studies. Cross-discipline discussions greatly helped both science areas reach new goals. Time-domain astronomy is used to explore many aspects of astrophysics—particularly concerning many of astronomy’s primary science goals of exploring how the Universe works, understanding how did we get here, and are we alone. Time domain observations are built into the core operations of Swift, TESS, Roman, PLATO, and other missions. Any conversation regarding TDAMM needs to include all aspects of astrophysics’s goals. If we have learned anything, time-domain observations designed for one scientific purpose, also lead to great discoveries in many other science areas. With the high alert volumes expected in the near future, it will be even more important to share information across domain boundaries. Early alerts will be poorly classified with initial follow-up observations possibly being of low interest to the original team, i.e., the source was not what was expected. However, such observations may perhaps be of high value to those interested in this particular source.
2.2 Definition and scope
Not all observations that happen across time necessarily require new considerations regarding new time-domain thinking. Just because some phenomena are observed with a light curve or a series of spectra does not mean they need to be included in these discussions. The specific requirements and factors that come into play in order to place a science case in the TDA category are:
• Time-sensitive observations: Certain phenomena can only be observed at certain times, whether those times are predictable or stochastic. This naturally includes traditional Target of Opportunity-type observations, such as GW events, explosive phenomena like supernovae, the beginning of a rare eclipse, flares, special stellar configurations (like microlensing) or other unpredictable events. It also applies to predictable but rare phenomena, which have similar difficulties when it comes to planning and coordinating observations, which we can call Rare Predictable Targets (RPTs), like long-period eclipsing multiple star systems, disk eclipsing systems, transiting and eclipsing planet observations, or eccentric-orbit non-transiting planet observations where the periastron passage is rarely observable that offer rare opportunities for key observations. Often, different types of time-sensitive scientific goals require different kinds of time-sensitive observations (e.g., rapid response vs. cadence observations) and these may change during the evolution of a given target.
• Multi-facility coordination: There are a number of science cases that require observations across multiple facilities simultaneously or in a specific sequence. For example, Roman + Rubin panchromatic investigations of exoplanets transits to disentangle transit signals from stellar variability (Limbach et al., 2023). While such observations do not necessarily have to take place at a specific time, the coordination in time across facilities means that such campaigns share many of the same observing and coordination difficulties as other time-domain astronomy efforts. These observations often involve multiple wavelengths (i.e., panchromatic observations), observing modes and/or instruments (photometry and spectroscopy), messengers (photons, gravitational waves, neutrinos, or cosmic rays), and both ground-based and space-based facilities.
• Multi-mode monitoring or monitoring with a single technique at regular cadence: For some predictable events and many stochastic events, there is often a period after an event where monitoring across many facilities and multiple channels can provide valuable insight. This monitoring effort can sometimes share some of the time constraints of the other two considerations, such as a minimum cadence of subsequent observation or a need for continuous observation inaccessible to a single ground-based facility. The monitoring period depends on the science goals and might last for a preset time frame (days or years), until a given event occurs (e.g., when a microlensing event ends), or might be indefinite. Monitoring also often involves a similar type of multi-facility coordination, but also data sharing, both for the alerts and for the subsequent follow-up data.
2.3 TDAMM key issues
Given the considerations above for how TDAMM operates across all of astrophysics, there are key issues that need to be addressed as part of any TDAMM initiative.
• Communication/Coordination—There are various electronic systems for managing the flow of data between various participants in TDAMM. These include observatories that generate alerts (e.g., LIGO, Rubin), alert brokers (e.g., ANTARES), transient marshals or Target and Observation Managers (TOMs) (e.g., the Supernova Exchange, GROWTH, ExoFOP), observing schedulers (e.g., the Las Cumbres Observatory network scheduler), coordination facilitators such as Treasure Map, and other tools. Can these systems talk to each other quickly and efficiently, are they publicly accessible and documented, and can all participants connect properly to them?
• Alerts—How universally are alerts being designed, generated, and dispersed? Are the standards, protocols, and terminology the same across relevant facilities? That includes NASA and other space missions, ground-based assets, laboratory and data center facilities, international partners, and dispersed multi-institution observing networks, as well as citizen scientists. Are the alert brokers part of that same conversation?
• Monitoring—How should monitoring campaigns be organized and administered? Certain monitoring science cases include specific needs for cadence, SNR, duty cycle, wavelength coverage, spatial or spectroscopic resolution, etc.? Is there a role for decentralized networks in which voluntary observing contributions may be sufficient (e.g., citizen science or Pro-Am collaborative networks), or do we need to build up more robust versions of hierarchically controlled observing networks, such as LCO or EHT? Note, there are social networks coordinating collaborations as well as hardware/software networks providing autonomous connections.
• Participation—How can all potential observers participate in campaigns, including ToOs, RPTs, and monitoring campaigns? Observers want to know that they will be properly credited, with paper co-authorships, citations, acknowledgments, or other mechanisms that are agreed upon ahead of monitoring campaigns? How are diversity and equity brought into the participation?
• Data Sharing—How do we best take advantage of and encourage the community to share data and information to maximize the efficient use of limited resources and minimize duplication and overlapping use of those same resources? As an example, within the exoplanet community and the TESS Follow-up Observing Program, the public NASA Exoplanet Archive (ExoFOP) service has been critical to help organize such efforts; how do we best expand that approach into a community-wide paradigm regardless of the specific scientific area? Existing surveys (e.g., SDSS) developed operational models before “Big data” and TDAMM were forefront. It is likely that support in terms of software and funds will be needed to have these valuable surveys brought into the mix.
• Existing Data—An insufficiently appreciated component of TDA is prior knowledge of the sky. Historical data become more, not less, important in the era of time-sensitive science. When an event (ToO or RPT) occurs, what did that field look like beforehand, across all relevant wavelengths, timescales, and angular size scales? To do the best possible science with space and ground facilities, we need to ensure that space-based and ground-based time-domain data are reserved as well. Surveys in the time domain have often been inadequately archived, with many data sets lost forever. At the moment, many optical sky surveys such as ASAS, ASAS-SN, Evryscope, ATLAS, CRTS, and others are in some cases only partially archived, without long-term storage and access plans at federal agency levels. Other data sets, e.g., DASCH (over a century of photographic plates), are still in the process of being digitized and only exist as photographic plates.
• Archiving—To address existing data sets, NASA archives must be involved in TDA discussions from the beginning. The archival data might exist at many different repositories, operated by different agencies, with different data access protocols. Support for archiving and serving such data sets should be included in TDAMM funding models, and the existence of these data must be realized as part of the broader TDAMM program formulation.
• Training—Access to and familiarity with the many new tools and services is far from uniform, creating a diversity and equity issue across astrophysics. Support for communiy outreach and training opportunities will be an important aspect of any successful program.
• Citizen Science—TDA also lends itself especially well to pedagogically impactful and scientifically productive citizen science projects. Indeed, perhaps the first large-scale citizen science project, observation and timing of the 1715 May 3 solar eclipse popularized by Edmond Halley (Pasachoff, 1999), was arguably a TDA program. TDA projects such as asteroid occultations (Cazeneuve et al., 2023) and exoplanet transit observations (Perrocheau et al., 2022) have enjoyed considerable involvement of citizen scientists. The technical simplicity of such observations allows amateur astronomers with minimal astrophysical knowledge and only modest observational experience to contribute scientifically meaningful data. Moreover, the recent advent of commercially available complete observational systems with built-in software for uploading observations to online repositories2 substantially facilitates the curation of citizen science datasets.
3 The current state of tools
While many areas of astronomy (not just time domain science) can benefit from the tools developed for MMA, often MMA use cases have the most stringent rapid communication requirements. This has meant that the existing transient follow-up ecosystem infrastructure must be overhauled with MMA in mind. However, with often minimal extra effort, these tools can and should be made to serve the entire astronomical community. Significant efforts are already underway. Here we discuss the MMA use case to establish the tools currently being modified or developed, and then outline how they can or are being adapted for other areas of time domain or more general astronomy.
3.1 The multimessenger astronomy workflow: GW170817 as a case study
In Figure 1 we show a flow chart featuring a simplified version of some of the discoveries and communications surrounding GW170817. Below we discuss a more updated and generic version of a similar process.
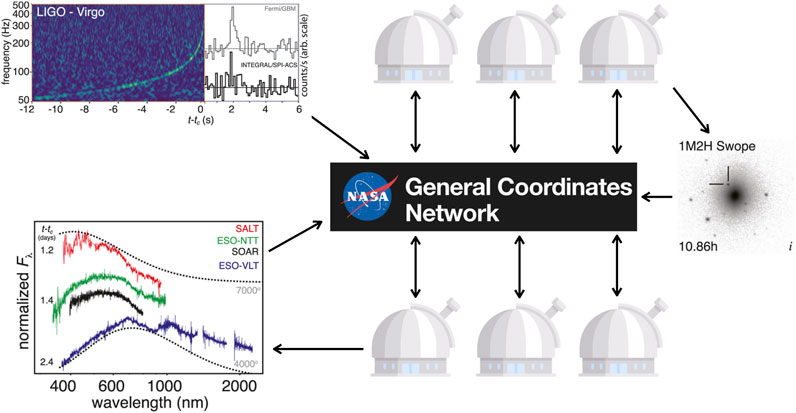
FIGURE 1. Simplified flow chart of a subset of the messenging and discoveries from GW170817. Aspects of the figure are adapted from Figure 2 of Abbott et al. (2017). After the initial discovery of gamma rays by Fermi and gravitational waves from LIGO and Virgo, messages detailing the discoveries were sent to a private version of NASA’s General Coordinates Network (GCN), although this was delayed for about 1.5 h. These messages were read by individual observatories and observers who triggered a search for the electromagnetic counterpart, and reported the results back to GCNs. Each communication introduced latency, from the up to 20 min delays from GCNs, to humans reading the messages, manually triggering telescopes, reducing the data, and writing a text verion of the results. The optical counterpart was discovered (right), nearly 11 h after the initial discovery in gamma rays. This was reported to GCNs, resulting in follow-up observations, including spectra (bottom left), taken 1.2, 1.4, and 2.4 days after discovery. Every step in this process is now faster, more robust, more machine readable, and more efficient, thanks to improvement in existing tools like TOMs and GCNs, and new tools like Treasure Map and Hermes.
3.1.1 The alert
Gamma rays are detected by several satellites, a merger of two neutron stars is detected by LIGO-Virgo-Kagra (LVK), and an alert is automatically generated and sent by various messaging systems. The NASA General Coordinates Network (GCN)3 sends machine-readable Notices, often nearly instantaneously via information from satellites. Meanwhile the GCN Circulars require a human to write them, but are not machine readable. Traditionally, both have been sent via email, but they are now being sent by Kafka, a more robust messaging platform.4 Additionally, LVK alerts are sent via Hopskotch,5 a Kafka-based messaging system created by the SCIMMA (Scalable Cyberinfrastructure for Multimessenger Astronomy)6 group funded by the NSF. Nearly any message can be sent via Hopskotch, but an extension of it, HERMES,7 provides an Application Programming Interface (API), graphical user interface, and a schema so that users know expected variable names. HERMES blends human and machine-readability so that users can specify machine-readable variable names to be sent in Javascript Object Notation (JSON), and free-text, and users can refer to named variables in the text.
3.1.2 The search
A GW event may be localized to hundreds or thousands of square degrees, so a search must be initiated to find the electromagnetic (optical/IR) counterpart. Some groups tile a broad area with large-format detectors, while others employ a galaxy-targeted approach. In the latter case, groups have special software to receive LVK alerts, and automatically generate a prioritized list of galaxies within the localization region. This is often done within a Target and Observation Manager (TOM)—web-based software that allows users to initiate automated observations, manage their data, and communicate about them. Whether a tiling or galaxy-targeted approach is used, users can trigger observations with their TOMs, which can then reduce, manage, and allow users to process the search data.
The planned and completed search pointings can be reported to the Treasure Map (Wyatt et al., 2020).8 The Treasure Map uses Aladin Lite to visualize probability contours on the GW localization region, which can be overplotted on one of dozens of sky surveys, e.g., Pan-Starrs, DSS, or H-alpha surveys. Planned and completed search areas, displayed as multiple detector footprints on the sky, are also overlaid. This allows any group to coordinate their search to avoid duplication. Other targets (e.g., information about galaxies or candidates) can also be overlaid.
3.1.3 Candidates
During a search, dozens or hundreds of candidates may be found. These are reported via the TNS, GCNs, and HERMES. Plugins for the TOM toolkit allow users to automatically parse machine-readable HERMES messages and ingest new candidates. For telescopes with APIs, with the click of a button, these can be dispatched for vetting observations from the TOM, including photometry and spectroscopy. This is reduced and displayed in the TOM, which can then be reported back to the community via the messaging services. Photometry and spectra can be sent over a Kafka topic in HERMES, which will automatically show up in other users’ TOMs if properly configured. By pooling data, the community can find the true counterpart faster, since they can combine photometry to reveal color and increases in brightness or compare spectroscopic information.
3.1.4 The electromagnetic counterpart
Finally, once the candidates have been vetted and the true, well-localized EM counterpart to the GW event is found, this is reported to the TNS, GCNs, and HERMES. Many astronomers will target this event, and data can be shared nearly instantaneously by the above mechanisms.
3.2 Use of the tools for non-MMA science
3.2.1 Messaging
Many areas of transient science have reported their findings to various messaging systems for decades, sometimes segregated by type. For example, new comets and minor planets are usually reported to the Minor Planet Electronic Circulars. GRBs, gravitational wave events, and high energy sources are usually reported to the GCNs. Supernovae are reported to the Transient Name Server (TNS),9 and their AstroNotes. High-energy neutrinos are reported in GCN Notices and Circulars, as are low-energy neutrinos from SNEWS and Super-Kamiokande. Meanwhile some variable stars, novae, and some supernovae are reported to the Astronomer’s Telegrams (ATELs).10 This has resulted in the unfortunate situation where different information on a target is reported to different systems, sometimes even under different names. For example, the gamma rays from GW170817 were first detected and eventually given the name GRB 170817A. Then gravitational waves were reported to an embargoed form (at the time) of the GCN Notices and Circulars as GW170817.11 Possible counterparts were then reported to the GCN Circulars and sometimes ATELs, often given arbitrary names, like SSS17a. Finally when the kilonova counterpart was confirmed, it was reported to the TNS and given the name AT 2017gfo, which was not always used in subsequent communications to other services (Abbott et al., 2017). Users had to monitor at least five services and sub-services to get the whole picture, and this was hindered by the fact that most of the hundreds of messages generated were not machine readable.
Different transient communities are locked into different messaging services partly by historical accident, partly because of limitations of the services, and partly because that’s where the community expects to get information. For example, not many services can handle moving objects, but the Minor Planet Center,12 which issues MPECs, is specialized for this. GCNs Notices were designed for rapid reporting of X-ray and gamma ray information, and are machine readable, but not very human-readable. The parallel GCN Circulars are human-readable, but not machine-readable. Neither service has an API (though one is being developed). The TNS is custom-built for supernovae (though it is expanding), and is machine readable, but has a separate system for longer-form human-readable messages, AstroNotes (which is not machine readable but can be cross-referenced). ATELs are not machine-readable and lack an API.
Some existing services are adding new functionality. For example, the TNS is beginning to process Fast Radio Bursts. The GCNs are moving to a Kafka-based system and adding an API. But a new system (Hopskotch and HERMES) has been developed from the ground up to overcome the limitations of previous systems. Hopskotch is a Kafka-based messaging service with built-in Identity and Access Management (IAM). It imposes no structure on messages, and users can create new topics, so that users can send any kind of message, not just discovery reports, as has been traditional. HERMES is a layer on top of Hopskotch, which adds structure to support standardization and machine readability. It is backed by an API so that it can easily be built into TOM systems, but also has a standalone web-based user interface. The same message can have both machine and human-readable components, sent via JSON. To overcome the distributed information problem, users can browse any Kafka topic, including GCNs and HERMES messages in the same place, with integrated search. Users can simultaneously send a single message to multiple systems (currently HERMES/Hopskotch, GCNs, and the TNS). The HERMES version of the message has machine readability, but a text-only message is sent to systems that do not support it.
HERMES is designed to be useful for all of astronomy, not just MMA. Named variables are as generic as possible, and where specific ones are necessary for a subfield (e.g., a cross-reference to a GW event), they are not required. Users can add additional machine readable key/value pairs if there are new needs that the developers did not consider. Moving targets are also supported. Cross-linking to other messages, or adding document object identifiers (DOIs), or other references are also supported.
3.2.2 The TOM toolkit
There are many examples of TOM systems built for a specific purpose (e.g., SkyPortal, YSE-PZ, the Supernova Exchange). Recognizing the need to not keep reinventing the wheel, Las Cumbres Observatory developed the TOM Toolkit13 to allow any user in any area of astronomy to create their own TOM. Emphasis is placed on modularity so that users can swap out components that suit their needs (e.g., support for moving targets, cross-referencing to SIMBAD, alert broker plug-ins). The code is open-source so that users can add new modules or improvements, which is then reviewed by the development team before it is included in the broader toolkit. Documentation and support are also prioritized to encourage adoption. TOMs have been built with the TOM toolkit in many areas of astrophysics, including supernovae, gravitational wave events, microlensing, near earth objects, AGN, gravitational wave events, variable stars, observatory support, and cosmology.
The TOM Toolkit has built-in support for Hopskotch and HERMES, so that the users of one TOM can click a button and immediately share photometry that shows up on a different TOM. This is done by writing to and reading from a Hopskotch topic. This can be made to work with any TOM, regardless of whether it was built with the TOM Toolkit. Support for the instant sharing of spectroscopy is in the works.
The TOM Toolkit also has native broker integration. ZTF discoveries are currently shared via Kafka, as LSST discoveries will be. Alert brokers ingest these alerts, and allow searching, filtering, and inferences derived from machine learning. So directly in their TOM, users can see new discoveries filtered by certain criteria, and then add a new target to their TOM and send it to observatories for additional observations with a few clicks.
3.2.3 The treasure map
In addition to gravitational wave events, Treasure Map is adding support for neutrino localizations and searches. It also supports joint localizations between GRBs and GW events. In principle, support could be added for other poorly localized astrophysical phenomena, such as GRBs, Fast Radio Bursts, or comets. Other areas of transient science could use the tool for, e.g., planning their observations, or seeing where their targets are located on the sky, and what has been detected across the EM spectrum in those regions.
4 Conclusion
Major advances in time-domain astronomy across multiple science disciplines relevant to astrophysics are becoming more urgent to address. Multi-messenger astrophysics (e.g., GW and particles) are beginning what will no doubt become a major revolution in our understanding of the Universe. However, aside from electromagnetic observations of gravitational wave events and explosive counterparts related to cosmological events, there are a number of “classical” astrophysical areas that require new thinking for proper exploration in the time domain. Time domain studies are already built into the core operations of many currently operating and future space telescopes as well as current and planned large areal ground-based surveys. Any conversation regarding TDAMM astrophysics should include all aspects of the field, including those aspects seen as classical applications. As a community, we want to assure that each type of TDAMM activity is supported, as we will learn even more about our Universe by being broadly inclusive of all TDAMM science.
New tools are being developed for MMA research to solve several problems limiting the rapid sharing of information in that field. Many of these tools are being developed with flexibility in mind, so that they can be used by any area of astrophysics. However, this will only succeed if there is wide adoption by the community. This requires community outreach, and support from federal funding agencies. A particular hurdle is that NASA is mandated to support space-based missions, while the National Science Foundation supports ground-based research. We must ensure that groups funded by either agency work together to make interoperable tools. Finally, long-term support is necessary to enable new functionality, battle code rot, and increase interoperability.
Author contributions
SH: Writing–original draft, Writing–review and editing. DH: Writing–original draft, Writing–review and editing. RS: Writing–original draft, Writing–review and editing. MS-F: Writing–original draft, Writing–review and editing. BJ: Writing–original draft, Writing–review and editing. TG: Writing–original draft, Writing–review and editing.
Funding
The author(s) declare financial support was received for the research, authorship, and/or publication of this article. SH acknowledges NASA Grant number 132379.04.07.01.81. MSF gratefully acknowledges the generous support provided by NASA through Hubble Fellowship grant HST-HF2-51493.001-A awarded by the Space Telescope Science Institute, which is operated by the Association of Universities for Research in Astronomy, In., for NASA, under the contract NAS 5-26555. BJ acknowledges NASA Exoplanets Research Program grant 80NSSC22K0317.
Acknowledgments
The authors wish to thank Joshua Pepper for motivating and contributing to this treatise and Eric Mamajek for useful comments. The Editors of this Research Topic, RS and Francesca Civano are to be commended for leading such a timely compilation related to TDAMM astrophysics.
Conflict of interest
The authors declare that the research was conducted in the absence of any commercial or financial relationships that could be construed as a potential conflict of interest.
The author(s) declared that they were an editorial board member of Frontiers, at the time of submission. This had no impact on the peer review process and the final decision.
Publisher’s note
All claims expressed in this article are solely those of the authors and do not necessarily represent those of their affiliated organizations, or those of the publisher, the editors and the reviewers. Any product that may be evaluated in this article, or claim that may be made by its manufacturer, is not guaranteed or endorsed by the publisher.
Footnotes
1https://www.nationalacademies.org/our-work/decadal-survey-on-astronomy-and-astrophysics-2020-astro2020
2https://www.unistellar.com/citizen-science/
4GCN Classic has also offered socket connection since 1993, and VOEvent for 10 years. GCN Circulars now also offer a web form for submission, and are developing an API for compatibility with HERMES.
10https://astronomerstelegram.org
11The archive of the embargoed GCNs are at https://gcn.gsfc.nasa.gov/other/G298048.gcn3
12https://www.minorplanetcenter.net
13https://lco.global/tomtoolkit/
References
Abbott, B. P., Abbott, R., Abbott, T. D., Acernese, F., Ackley, K., Adams, C., et al. (2017). Multi-messenger observations of a binary neutron star merger. Astrophysical J. Lett. 848. doi:10.3847/2041-8213/aa91c9848L.12A
Belle, K. E., Howell, S. B., Mukai, K., Szkody, P., Nishikida, K., Ciardi, D. R., et al. (2005). Simultaneous X-ray and optical observations of EX hydrae. Astronomical J. 129, 1985–1992. doi:10.1086/428487
Cazeneuve, D., Marchis, F., Blaclard, G., Dalba, P. A., Martin, V., and Asencio, J. (2023). ODNet: a convolutional neural network for asteroid occultation detection. Astronomical J. 165, 11. doi:10.3847/1538-3881/ac9c69
Cortie, A. L. (1915). The magnetic storm of june 17 and solar disturbances. Nature 95, 618. doi:10.1038/095618b0
Gordon, K. C., and Kron, G. E. (1947). Photoelectric light-curves of RR lyrae, TU cassiopeiae, and T monocerotis. Astrophysical J. 106, 318. doi:10.1086/144968
Herbig, G. H. (1970). VY Canis majoris. II. Interpretation of the energy distribution. Astrophysical J. 162, 557. doi:10.1086/150688
Kollath, Z. (1990). Chaotic behaviour in the light variation of the RV Tauri star R Scuti. Mon. Notices RAS 247, 377.
Limbach, M. A., Soares-Furtado, M., Vanderburg, A., Best, W. M. J., Cody, A. M., D’Onghia, E., et al. (2023). The TEMPO survey. I. Predicting yields of transiting exosatellites, moons, and planets from a 30 days survey of orion with the roman space telescope. Publ. Astronomical Soc. Pac. 135, 014401. doi:10.1088/1538-3873/acafa4
Mason, K. O., Murdin, P. G., Tuohy, I. R., Seitzer, P., and Branduardi-Raymont, G. (1982). Phase resolved optical spectroscopy of the compact X-ray binary 2A 1822-371. Mon. Notices RAS 200, 793–805. doi:10.1093/mnras/200.3.793
Pasachoff, J. M. (1999). Halley as an eclipse pioneer: his maps and observations of the total solar eclipses of 1715 and 1724. J. Astronomical Hist. Herit. 2, 39–54. doi:10.3724/sp.j.1440-2807.1999.01.04
Perrocheau, A., Esposito, T. M., Dalba, P. A., Marchis, F., Avsar, A. M., Carrera, E., et al. (2022). A 16 hr Transit of Kepler-167 e Observed by the Ground-based Unistellar Telescope Network. Astrophysical J. Lett. 940, L39. doi:10.3847/2041-8213/aca073
Powell, J. R., Trumbo, D. E., and Lynds, C. R. (1969). A technique for recording phase-resolved spectra of regularly-varying faint light sources. Publ. Astronomical Soc. Pac. 81, 601. doi:10.1086/128825
Sandage, A. R., Becklin, E. E., and Neugebauer, G. (1969). Ubvrihkl photometry of the central region of M31. Astrophysical J. 157, 55. doi:10.1086/150049
Schulte-Ladbeck, R. E., and Hopp, U. (1990). A multiwavelength study of the galaxy UGC 11000. Astronomy Astrophysics 239, 69–239.
Shapley, H. (1920). Studies based on the colors and magnitudes in stellar clusters. XV. A photometric analysis of the globular cluster system Messier 68. Astrophysical J. 51, 49–61. doi:10.1086/142523
Standish, E. M., and Nobili, A. M. (1997). Galileo’s observations of neptune. Balt. Astron. 6, 97–104. doi:10.1515/astro-1997-0117
Keywords: time-domain astronomy, multi-messenger astronomy, time-series observations, phase-resolved observations, multi-wavelength observations
Citation: Howell SB, Howell DA, Street RA, Soares-Furtado M, Jackson B and Greene TP (2024) The dynamic universe: realizing the potential of classical time domain and multimessenger astrophysics. Front. Astron. Space Sci. 11:1304616. doi: 10.3389/fspas.2024.1304616
Received: 29 September 2023; Accepted: 02 January 2024;
Published: 15 January 2024.
Edited by:
Rita Sambruna, National Aeronautics and Space Administration, United StatesReviewed by:
Judith Racusin, National Aeronautics and Space Administration, United StatesEric Perlman, Florida Institute of Technology, United States
Copyright © 2024 Howell, Howell, Street, Soares-Furtado, Jackson and Greene. This is an open-access article distributed under the terms of the Creative Commons Attribution License (CC BY). The use, distribution or reproduction in other forums is permitted, provided the original author(s) and the copyright owner(s) are credited and that the original publication in this journal is cited, in accordance with accepted academic practice. No use, distribution or reproduction is permitted which does not comply with these terms.
*Correspondence: Steve B. Howell , c3RldmUuYi5ob3dlbGxAbmFzYS5nb3Y=