- 1Yale Center for Astronomy and Astrophysics, New Haven, CT, United States
- 2Department of Physics, Yale University, New Haven, CT, United States
- 3Department of Physics and Astronomy, Clemson University, Kinard Lab of Physics, Clemson, SC, United States
- 4Centre for Space Research, North-West University, Potchefstroom, South Africa
- 5Department of Astronomy, Yale University, New Haven, CT, United States
- 6Space Science Data Center, Agenzia Spaziale Italiana, Roma, Italy
- 7Department of Physics & McDonnell Center for the Space Sciences, Washington University in St. Louis, St Louis, MO, United States
- 8X-ray Astrophysics Laboratory, NASA Goddard Space Flight Center, Greenbelt, MD, United States
- 9Cahill Center for Astrophysics, California Institute of Technology, Pasadena, CA, United States
- 10Finnish Centre for Astronomy with ESO, University of Turku, Turku, Finland
- 11NASA Marshall Space Flight Center, Huntsville, AL, United States
- 12SLAC National Accelerator Center and Kavli Institute for Particle Astrophysics and Cosmology, Stanford University, Stanford, CA, United States
- 13INAF - Osservatorio Astronomico di Brera, Milano, Italy
- 14INAF Osservatorio Astronomico di Roma, Frascati, Monte Porzio Catone, Italy
- 15Department of Physics and Astronomy, Bowdoin College, Brunswick, ME, United States
- 16Department of Physics, National and Kapodistrian University of Athens, University Campus Zografos, Athens, Greece
- 17NASA Goddard Space Flight Center, Greenbelt, MD, United States
- 18University of Maryland, Baltimore County, Baltimore, MD, United States
- 19INAF - Osservatorio Astronomico di Brera, Merate, Italy
- 20Jet Propulsion Laboratory, California Institute of Technology, Pasadena, CA, United States
- 21Landessternwarte, Universität Heidelberg, Heidelberg, Germany
A fraction of the active supermassive black holes at the centers of galaxies in our Universe are capable of launching extreme kiloparsec-long relativistic jets. These jets are known multiband (radio to
1 Introduction
The high-energy
A unique mission such as the High Energy X-ray Probe (HEX-P; Madsen et al., 2023), with its broadband X-ray coverage
The peculiar jet orientation in blazars allows for extreme relativistic beaming, opening a window for studies of particle acceleration mechanisms and jet structure (Madau et al., 1987; Dermer, 1995; Dondi and Ghisellini, 1995). These sources are extremely bright multi-wavelength emitters (from radio up to TeV energies; see Massaro et al., 2008; Madejski and Sikora, 2016; Blandford et al., 2019), reaching apparent bolometric luminosities of
Clues towards the jets’ particle composition lie in the simultaneous characterization of the X-ray spectrum from soft X-rays (
Another observational multi-band characteristic of blazars is their variability, with flaring timescales ranging from months down to minutes, flux enhancements of up to several orders of magnitude, and cross-correlated variability patterns (or lack thereof) observed between different energy bands. To explain this behavior, several particle acceleration mechanisms have been proposed to act in these jets, related to magnetic reconnection occurring inside the jet (e.g., Giannios, 2013; Christie et al., 2019; Zhang H. et al., 2022) for the fastest time scales observed, or mildly relativistic shocks (Rees, 1978; Marscher and Gear, 1985) to describe variability on longer time scales. The most rapid variations, which are shorter than 10 min, have been observed at
Flaring can lead to a deeper understanding of the physics in AGN jets in terms of particle acceleration mechanisms and dissipative locations in the jet. Additionally, together with neutrino observations, flaring can be pivotal for understanding the particle content responsible for the photon emission. The association of the
In terms of population studies, there appears to exist an anti-correlation between the synchrotron peak position and the intrinsic bolometric luminosity of blazars, the so-called blazar sequence (see Fossati et al., 1998; Ghisellini et al., 1998; Ghisellini et al., 2017). If real, this would imply that more luminous sources (found at the highest redshifts) have higher accretion rates
Finally, jets of active galaxies have been observed to extend up to hundreds of kiloparsecs into the intergalactic medium. They are spatially best resolved in the radio band, using very-long-baseline interferometry (VLBI) observations, where low-energy synchrotron emission can be observed along the entire jet (e.g., Linfield, 1981; Boccardi et al., 2016). Moreover, many jets also show extended components (straight jets or hotspots along the jet flow) in various other energy bands including the X-ray domain (Chartas et al., 2000; Harris and Krawczynski, 2006; Stawarz et al., 2013; Marshall et al., 2018; Meyer et al., 2018), even up to the TeV energy band (e.g., Abramowski et al., 2012; H. E. S. S. Collaboration et al., 2020; Archer et al., 2020). The origin of the X-ray (and high-energy) emission from extended jets is certainly non-thermal (see e.g., Harris and Krawczynski, 2002; Harris and Krawczynski, 2006). However, it is unclear whether synchrotron radiation or inverse-Compton emission dominates. The former process seems to well describe the emission arising from low-power jets while for high-power radio galaxies and QSOs the dominant mechanism could be inverse Compton, in most cases having seed photons provided by the CMB radiation (e.g., Harris and Grindlay, 1979; Stawarz et al., 2003; Atoyan and Dermer, 2004; Zacharias and Wagner, 2016; Meyer et al., 2017; Roychowdhury et al., 2022). Observations with a mission such as HEX-P will be crucial for resolving, for the very first time in the hard X-ray band beyond
The paper is organized as follows: Section 2 describes the HEX-P mission design; Section 3 details the setup for the simulations performed throughout the paper; Section 4 addresses the contribution of HEX-P to the multi-messenger and time domain astrophysics of blazars; Section 5 highlights the prospects for different blazar classes; Section 6 showcases the importance of HEX-P in resolving jets in the hard X-ray band; and Section 7 summarizes the main science cases that HEX-P will be able to address with respect to the most powerful jets in the Universe.
2 Mission design
HEX-P (Madsen et al., 2023)is a Probe-class mission concept that offers sensitive broad-band coverage
The LET consists of a segmented mirror assembly coated with iridium on monocrystalline silicon that achieves a half-power diameter of
The HET consists of two co-aligned telescopes and detector modules. The optics are made of Ni-electroformed full shell mirror substrates, leveraging the experience of XMM-Newton (Jansen et al., 2001), and coated with Pt/C and W/Si multilayers for an effective passband of
3 Simulations
All the simulations presented here were produced with a set of response files that represent the observatory performance based on current best estimates as of Spring 2023 (see Madsen et al., 2023). The effective area is derived from ray-tracing calculations for the mirror design including obscuration by all known structures. The detector responses are based on simulations performed by the respective hardware groups, with an optical blocking filter for the LET and a Beryllium window and thermal insulation for the HET. The LET background was derived from a GEANT4 simulation (Eraerds et al., 2021) of the WFI instrument, and the HET background was derived from a GEANT4 simulation of the NuSTAR instrument; both backgrounds assume HEX-P’s Lagrange point L1 orbit.
The spectral simulations were performed in xspec (Arnaud, 1996) via the “fakeit” command with V071 response files, selecting an 80% PSF correction. The data are assumed to be extracted from a 10 arcsec region, with the anticipated background for the telescope being positioned at L1. The images and light curves were simulated using the SIXTE toolkit (Dauser et al., 2019), using the same response files as the spectral simulation.
The differential sensitivity curves shown throughout the paper are obtained by calculating for each energy bin (LET:
4 Time domain and multi-messenger astrophysics
4.1 Leptonic vs. hadronic populations in blazar jets
4.1.1 Probing the relativistic leptonic population in blazar jets
As discussed in Section 1, the location of the two peaks of the blazar’s broadband SED generally depends on its apparent luminosity (e.g., Ghisellini et al., 2017). For HSP sources, the flux in the spectral regime corresponding to the X-ray energy
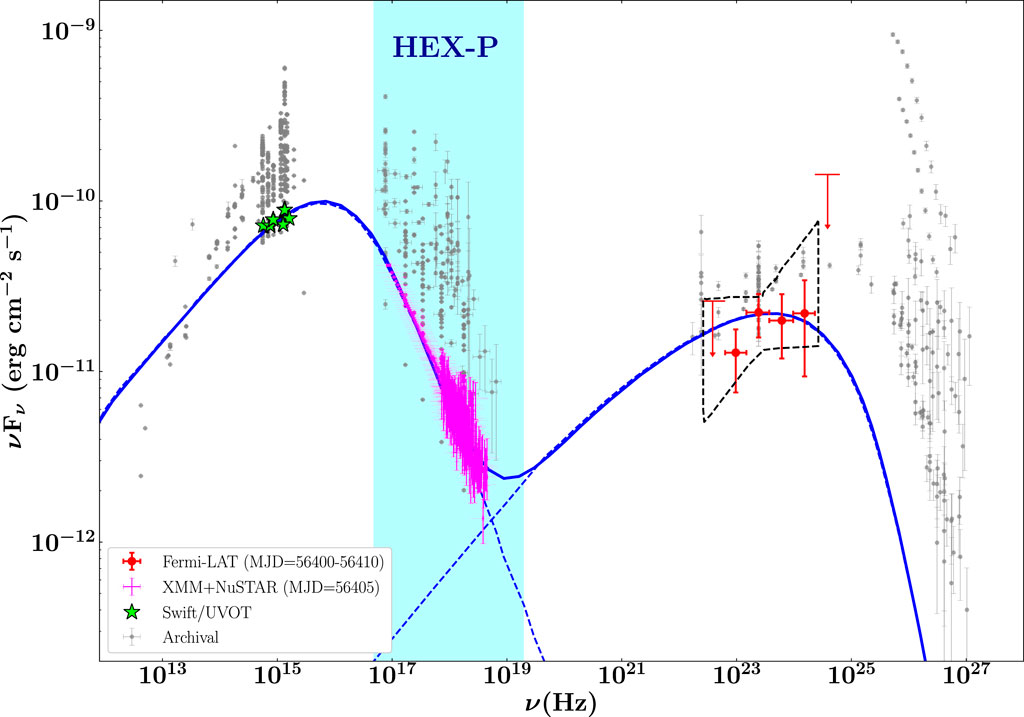
Figure 1. Broadband SED of the HSP blazar PKS 2155–304 adapted from Madejski et al. (2016). In gray, we show the multi-epoch multi-band archival data for the source, as an example of the extreme multi-band variability of blazar sources. The low-state was characterized by Madejski et al. (2016), collecting contemporaneous data from: NuSTAR and XMM-Newton-pn (MJD = 56405; magenta points), Swift-UVOT (fluxes corrected for Galactic reddening; green stars), and Fermi-LAT obtained for 10 days centered on the NuSTAR observation (data points in red; broad-band spectral fit “butterfly” in black). Also shown is a basic SED model including synchrotron and SSC components (blue lines). As mentioned in the text, the X-ray data occupy the “valley” in the SED. Highlighted in cyan is the energy band that will be simultaneously covered by HEX-P.
To see the improvement of a mission such as HEX-P for this scenario, we simulated a 100 ks observation of a broken power-law X-ray spectrum of PKS 2155–304 at the same flux level as the observation shown in Madejski et al. (2016),
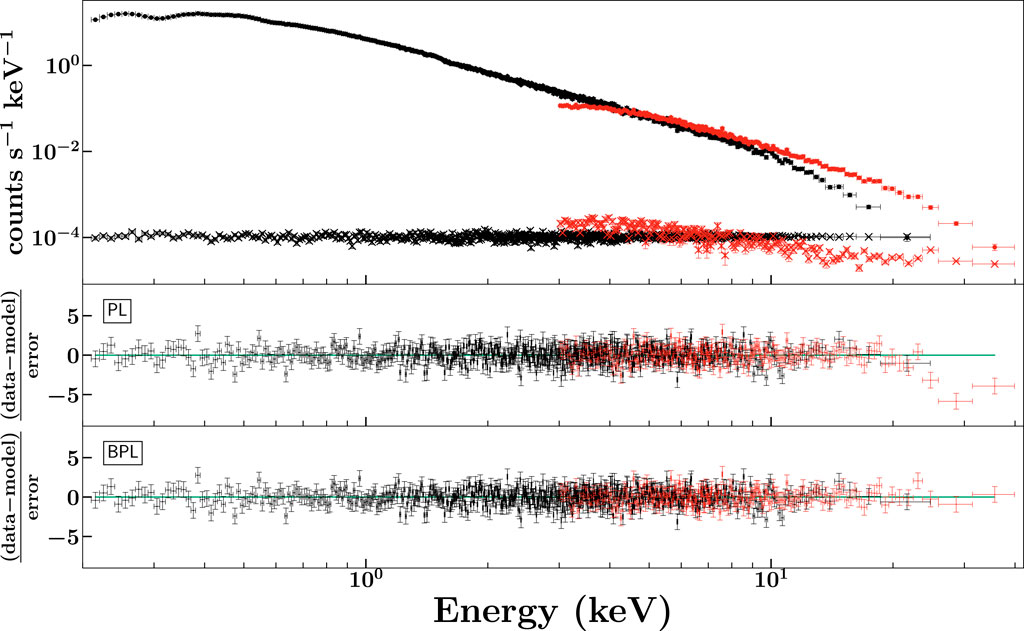
Figure 2. Simulated 100 ks observation of PKS 2155–304 with the LET (black data points) and the HET (red data points) assuming a broken power-law energy distribution of the underlying emission, with a break happening at
In the case of LSP sources, the X-ray band already probes the onset of the high-energy SED “hump”. In the context of synchro-Compton models for relativistic jets, this part of the spectrum is produced by the lowest-energy part of the distribution of radiating particles. In these sources, therefore, HEX-P will probe the low-energy tail of the particle distribution, possibly revealing the presence of low-energy cutoffs thanks to the long arm provided by broad-band sensitivity out to 80 keV in a single observation (e.g., Fabian et al., 2001; Tavecchio et al., 2007; Sbarrato et al., 2016). The broad-band capability of HEX-P is also important in ISPs sources, where the X-ray band corresponds to the cross-over between the synchrotron and inverse Compton peaks, i.e., the “valley” floor (see e.g., ON 231 and TXS 0506 + 056, Tagliaferri et al., 2000; IceCube Collaboration et al., 2018), and the hard-X-ray range provides constraints on the slope of the low-energy electrons. In both cases, given the observed photon spectrum, it is possible to infer the distribution of energies of radiating particles, and for relativistic jets, the low-energy radiating particles are the most numerous. This, in turn, provides good estimates of the total particle content of the jet, and strong constraints on the total kinetic power of the jet (Madejski et al., 2016), as well as its electron-positron pair content. Thus, for high-luminosity sources, hard X-ray observations by HEX-P will provide estimates of the jet power. We note that, in principle, one could study the low-energy end of particle distributions by examining the lowest energy part of the low-energy “hump”. However, the region producing the high-energy (X-to-
4.1.2 Probing the relativistic hadronic population in blazar jets
The high-energy blazar spectral component may consist of one or multiple radiation processes. In the leptonic model, the high-energy component may have contributions from both SSC and EC, while in the hadronic model, the hadronic cascading component can be considerable in the X-ray to MeV
To illustrate the capabilities of HEX-P in identifying spectral breaks due to the transition from leptonic to hadronic emission components, we choose Ap Librae, an intriguing LSP at redshift
We adopt Model A from Petropoulou et al. (2017) (see in Figure 3A) to simulate spectra for HEX-P, assuming a 50 ks exposure. The model predicts a broadband X-ray spectrum with leptonic and hadronic contributions in the energy range probed by HEX-P. More specifically, inverse Compton scattered (ICS) emission by accelerated electrons in the jet contributes mostly at hard X-rays (
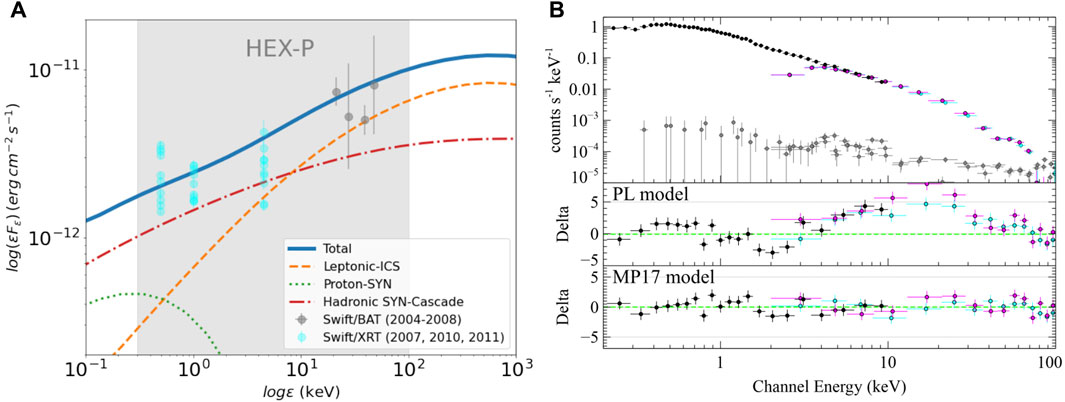
Figure 3. (A): Theoretical spectrum for the core emission of Ap Librae from Petropoulou et al. (2017) showing hadronic and leptonic contributions in the 0.2–100 keV range. Colored markers show non-simultaneous observations in soft and hard X-rays obtained with Swift/XRT and Swift/BAT, respectively. A smooth spectral break is evident at
To demonstrate also the capabilities of HEX-P in detecting spectral changes during the course of an X-ray flare, we simulate a
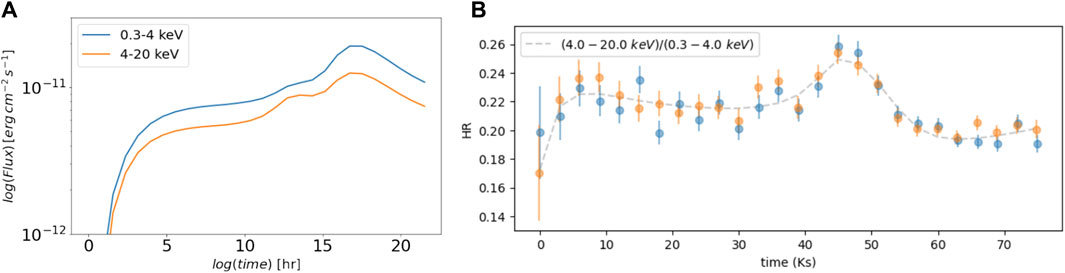
Figure 4. (A): Theoretical soft (0.3–4 keV) and hard (4–20 keV) X-ray light curves of a hypothetical flare from Ap Librae with a total duration of 21 h. (B): Evolution of the hardness ratio (HR) during the flare. The HR evolution based on the tabulated model is shown with dashed line, while the results of two simulations are overplotted with colored markers. Each point is obtained assuming a 3 ks observation with HEX-P.
4.2 Multimessenger follow-up: cosmics rays and neutrinos
Cosmic rays were first detected at the beginning of the 20th century (Wulf, 1909; Hess, 1912; Pacini, 1912), yet, more than 100 years later, the sources of the high and ultra-high energy cosmic rays remain unclear. Since protons are deflected in magnetic fields, they are not reliable tracers of their origin. Neutrinos that are produced in proton-proton and proton-photon interactions travel in a straight path, but are much harder to detect.
Blazars and AGN have long been suspected to be neutrino emitters. Material in the accretion disk and in the vicinity of the supermassive black hole includes partially or fully ionized gas, which is available for injection into the relativistic outflows. Protons in the jet can interact with photons from a stationary photon field (e.g., thermal UV emission from the disk), and produce neutral and charged pions. Charged pions decay into several products including several neutrinos, while neutral pions produce high-energy photons, detectable by HEX-P. These photo-pion processes are expected to happen in AGN jets (Biermann and Strittmatter, 1987; Mannheim et al., 1992; Mannheim, 1993) and AGN cores (Eichler, 1979; Berezinskii and Ginzburg, 1981; Protheroe and Kazanas, 1983; Begelman et al., 1990; Stecker et al., 1991). The photo-hadronic interactions likely responsible for the neutrino production in blazar jets inevitably lead to the development of electromagnetic cascades. These are initiated by synchrotron or inverse-Compton scattering by secondary electron-positron pairs produced in photo-pion and Bethe-Heitler pair production processes, and the subsequent decay of charged and neutral pions and muons. The radiative output of these cascades is expected to peak in the hard X-ray – soft
Follow-up of IceCube neutrino alerts has been promising for identifying the source of astrophysical neutrinos and therefore cosmic rays. The associations of blazars with VHE neutrino events detected by IceCube have attracted much attention in the recent literature, with increasing evidence pointing towards blazars being responsible for at least part of the IceCube VHE neutrino flux (e.g., Krauß et al., 2014; Kadler et al., 2016; Garrappa et al., 2019; Plavin et al., 2020; Buson et al., 2022; Plavin et al., 2022). In 2017, a high-energy IceCube event (IceCube-170922A) was found in positional and temporal coincidence with a blazar flare from TXS 0506 + 056 (IceCube Collaboration et al., 2018), following the previous, lower-confidence coincidence of a PeV neutrino event and a blazar flare (Kadler et al., 2016). Surprisingly, an earlier neutrino flare consistent with the position of TXS 0506 + 056 between September 2014 and March 2015 is not accompanied by a
Previous detections of possible blazar counterparts were followed by the detection of possible Seyfert AGN counterparts, that can only be identified in the X-ray band: IceCube-190331A (Krauß et al., 2020) and the recently discovered neutrino source NGC 1068 (IceCube Collaboration et al., 2022). X-ray follow-up of likely-cosmic neutrino alerts has thus proven its utility for identifying possible neutrino counterparts, and most importantly for measuring hadronic contributions to the high-energy emission. HEX-P has a response rate of the order of 1 day (see Madsen et al., 2023). While we may detect neutrino from sources in quiesence, a short flare lasting less than a day or week does not increase the fluence of the source significantly to explain the neutrino event. As only the total flare fluence is relevant, sources in quiescence or long-lasting outbursts are the most promising source of neutrinos from blazars (Kreter et al., 2020b). The response time to triggers from HEX-P is therefore well suited to perform multimessenger follow-up observations.
Blazars have been ruled out as the dominant source of IceCube neutrino events in the TeV energy band through stacking analyses (e.g., Hooper et al., 2019). Blazars, especially BL Lacs are expected to produce neutrinos in the energy range of PeV to EeV (e.g., Murase et al., 2014; Padovani et al., 2015). These are energies that will be covered by the Askaryan Radio Array (ARA; Allison et al., 2016), currently under construction, as well as the IceCube-Gen 2 detector (Grant et al., 2019). IceCube Gen 2 with a 8 cubic kilometer volume is expected to be completed in 2032, which would coincide with the expected start of the HEX-P mission.
HEX-P will identify possible neutrino emitters and constrain the fluxes and emission mechanisms. One of the main challenges of neutrino follow-up is the large uncertainty regions of both cascade and track events. Track events are more promising for a direct identification of a counterpart source due to their smaller angular uncertainties,
In order to test whether HEX-P observations are able to distinguish between a single power-law and the curved up-turns expected from proton-induced cascades, we performed detailed simulations. Three model cascade spectra (synchrotron-supported cascades) from Figure 12 of Reimer et al. (2019) (for
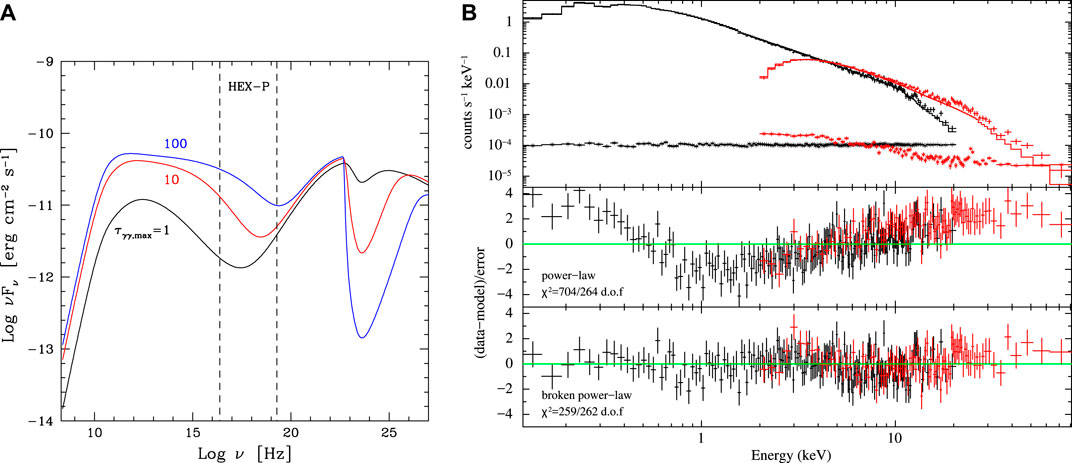
Figure 5. (A): Theoretical SEDs of TXS 0506 + 056 (including EBL absorption) calculated from simulations of photo-pion induced synchrotron-supported cascades, for values of
4.3 Variability studies of blazars
Variability is a defining feature of all blazar subclasses, and studies have been conducted across the entire electromagnetic spectrum for many years, both with a focus on long-term (weeks to years) as well as short-term (days to hours) time scales. It is increasingly recognized that simultaneous broadband variability study is crucial to understand blazar physics. But the sample sizes of these studies depend largely on monitoring capabilities in the different energy bands. In the soft X-rays, monitoring campaigns can be performed for certain objects with Swift/XRT (Giommi et al., 2019; 2021), while observations with, e.g., XMM-Newton enable investigation of variability on time scales of hours (e.g., Zhang et al., 2006; Devanand et al., 2022; Noel et al., 2022). Long-term monitoring in the hard X-ray range is possible with Swift/BAT and MAXI (and in the past with RXTE), though, only for the brightest blazars. Variability studies above 10 keV on intraday time scales have been performed with RXTE (Wang et al., 2018) and later NuSTAR (Bhatta et al., 2018). Since no existing X-ray telescopes have sensitive broadband capabilities, simultaneous coverage of soft to hard X-ray variability has to be performed by carefully coordinating multiple X-ray telescopes pointing, which has only been arranged for a small number of observations.
The combination of high sensitivity and time resolution on the order of milliseconds and up to 80 keV will enable HEX-P to probe rapid variability, i.e., fluctuations within several minutes, at hard X-ray energies for a sample of bright blazars, which has not been feasible so far. In particular, we will be able to probe HSPs, for which minute timescale variability has been seen in gamma-rays and which could become evident only in hard-X-rays, since produced by higher-energy electrons, or a hardening at high energies as the result of the harder emission of freshly accelerated electrons on top of a more persistent, softer component.
In the case of FSRQs, HEX-P follow-up observations of flaring blazars will yield an unprecedented view up to 80 keV, i.e., full coverage of the rising half of the high-energy hump, and enable time-resolved spectral studies, which might be able to track changes in the high-energy emission related to, e.g., a change from SSC to EC emission over the course of a flaring event. This part of the spectrum can be a mixture of SSC and EC under the leptonic scenario, while under the hadronic scenario it may consist of SSC, hadronic cascades, EC, or proton synchrotron (Böttcher et al., 2013; Cerruti et al., 2015; Zhang et al., 2016). Theoretical studies have shown that these radiation processes can have different variability patterns, which can lead to distinguishable spectral and temporal signatures in the broadband X-rays. For instance, in the leptonic model EC often peaks at higher energy than SSC, thus the X-ray band can become dominated by a softer SSC spectrum from higher-energy electrons (thus more variable) over a harder EC component by lower-energy electrons, leading to a “softer-when-brighter” behavior (see e.g., Ghisellini et al., 1999; Diltz et al., 2015; Zhang et al., 2015). Simultaneous broadband X-ray variability can therefore track the transition from SSC to EC, as well as unveil possible bulk-compton emission components, which are expected to be transient (Celotti et al., 2007). They can thus probe the underlying particle evolution, and put constraints on the origin of SSC and EC emissions.
Observations have shown that blazars of all types (low, intermediate or high-synchrotron-peaked objects) may experience epochs of extreme particle acceleration, resulting in its synchrotron peak shifting into or across the X-ray band. Most dramatic events have been shown for example, by Mkn 501 in 1997 (Pian et al., 1998; Tavecchio et al., 2001), 1ES 1959 + 650 in 2002 (Krawczynski et al., 2004) and more recently, again by Mkn 501 (Aliu et al., 2016; MAGIC Collaboration et al., 2020), Mkn 421 (Tramacere et al., 2009; MAGIC Collaboration et al., 2020), PKS 2005–489 (Tagliaferri et al., 2001; Chase et al., 2023) and 1ES 1215 + 303 (Valverde et al., 2020). Such phenomena are often referred to as the peak shift. The physical cause is generally understood as the emerging of a new hard emission component from freshly accelerated electrons, injected by a new dissipation event. The mechanism and location of this acceleration are instead still uncertain, being debated among shock acceleration (either in internal shocks or a standing shock), magnetic reconnection and/or hadronic origins (Böttcher et al., 2013; Zhang H. et al., 2022). Due to the significant spectral changes, observing these events needs broadband coverage. Temporal slicing of the SED evolution is therefore important to elucidate the formation of such extreme physical conditions. In Figure 6 we show as an example of a simulated magnetic reconnection event for a high-peaked BL Lac object using the model of Zhang Z. et al. (2021). With very good broadband sensitivity and timing capability, HEX-P can track the peak shifts and spectral variations in greater detail than previously possible, revealing the physical driver of such extreme particle acceleration and probe potential hadronic origins. As such, HEX-P will serve as a space-based time domain astronomy instrument; a field that has been identified as one of the key research topics in the Astro2020 decadal survey2 (National Academies of Sciences, Engineering, and Medicine, 2021).
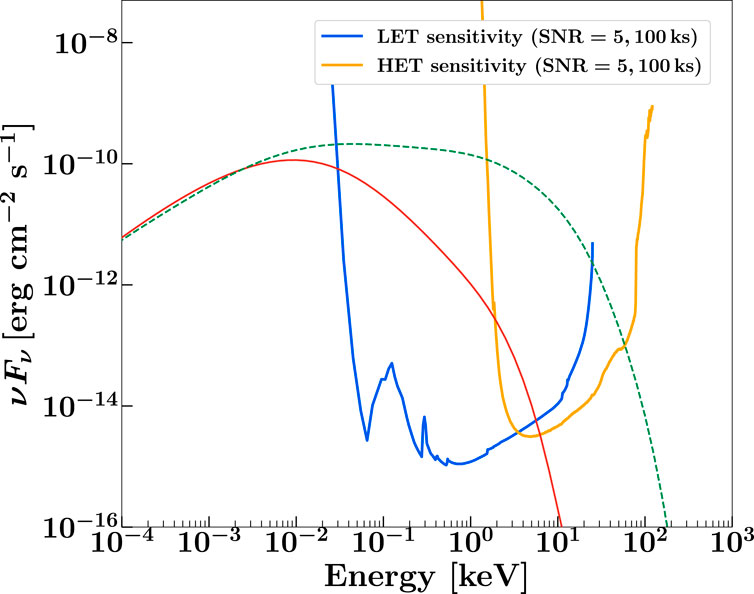
Figure 6. Simulated synchrotron peak of a high-peaked BL Lac object in quiet state (red line) versus the shifted synchrotron peak from the same source during flaring activity (dashed green line). Both the quiescent and the flaring state are produced by the magnetic reconnection in the same current sheet using the numerical simulation from Zhang H. et al. (2021). Both the quiescent and flaring state spectra result from plasmoids in the magnetic reconnection region, which has a fixed amount of initial magnetic energy. However, the flare is due to a major plasmoid merger event, which leads to significant dissipation of magnetic energy and efficient acceleration of nonthermal particles. The newly accelerated particles reach a much higher energy and harder spectrum than particles in other plasmoids, producing a peak shift. The sensitivity curves for both the LET and HET of HEX-P illustrate the excellent capability of the X-ray telescope to precisely measure changes in the position and shape of the synchrotron peak of blazars.
For high-peaked BL Lac objects, spectral changes have been observed during flares, during which the spectrum often becomes harder when the source gets brighter. The study of light curves covering different energy ranges can reveal the existence of lags, which are indicators of the efficiency of the underlying acceleration and cooling processes. Interestingly, these lags are not consistent: for example, the well-studied source Mrk 421 has shown both soft and hard lags at different times, without, so far, a consistent pattern (Zhang et al., 2010; Kapanadze et al., 2016; Noel et al., 2022). HEX-P will be an essential instrument for these studies, in particular for flare follow-up observations, as it covers both the soft and hard X-ray range with high sensitivity.
One key discovery in the last decades has been flux variations close to or below the light-crossing time scale of the super-massive black hole (SMBH, Aharonian et al., 2007b; Aleksić et al., 2014b; Ackermann et al., 2016b), which revealed extreme particle acceleration in very localized regions. These rapid flux variations provide invaluable information about the particle acceleration mechanisms in jets and are broadly connected to the capability of the jet of accelerating protons and producing neutrinos. Observed, so far, in a few exceptional flares, such rapid gamma-ray variability has shown the limitations of standard emission models (Begelman et al., 2008; Ghisellini and Tavecchio, 2008; H. E. S. S. Collaboration et al., 2019) and prompted more sophisticated scenarios (see, e.g., Aharonian et al., 2017), such as turbulent, multi-zone emission (Marscher, 2014), magnetic reconnection inside the jet or in the jet launching region (see, e.g., Cerutti et al., 2012; Giannios, 2013; Kadowaki et al., 2015; Christie et al., 2019; Zhang H. et al., 2021), jet-star interactions (Barkov et al., 2012), or acceleration in the black-hole magnetosphere (Levinson and Rieger, 2011). These scenarios predict different signatures in both spectral and temporal behaviors, but require broadband time-domain observations to further understand the jet physics. HEX-P would be able to detect a break in the broad band X-ray spectra that is predicted by some models (jet-star interaction) due to the emergence of an additional synchrotron component, which would rise in the hard X-ray band. Alternatively, simulations of processes dominated by magnetic reconnection and turbulence predict distinct temporal patterns in the X-ray band (e.g., Zhang H. et al., 2020; Zhang H. et al., 2023). Those require highly resolved timing data across the full X-ray band in order to be detected, which HEX-P would be able to provide. At VHE, CTAO is expected to probe broadband (sub-TeV to
Current theories suggest that such short timescale variability should also be present in X-ray synchrotron emission, but so far the few simultaneous data show that, even when tightly correlated, the (soft) X-ray variations have typically been of much lower amplitude than at VHE (Aharonian et al., 2009a) and in general on longer timescales (tens of minutes). While X-ray synchrotron emission may suffer from a stronger dilution of variable flux by brighter persistent emission, a key issue in observation is that current X-ray instruments lack the same broadband sensitivity as the VHE telescopes. The synchrotron emission of the rapidly-variable TeV electrons could peak at higher X-ray energies, in a band not yet investigated because of the lack of large collection areas above 5–8 keV. Additionally, several models, such as magnetic reconnection, predict distinct variability patterns in soft and hard X-ray bands (Figure 7), which require broadband coverage with good temporal sensitivity (Zhang H. et al., 2021). HEX-P will be able to probe, for the first time, such short timescales simultaneously at both soft and hard X-ray energies.
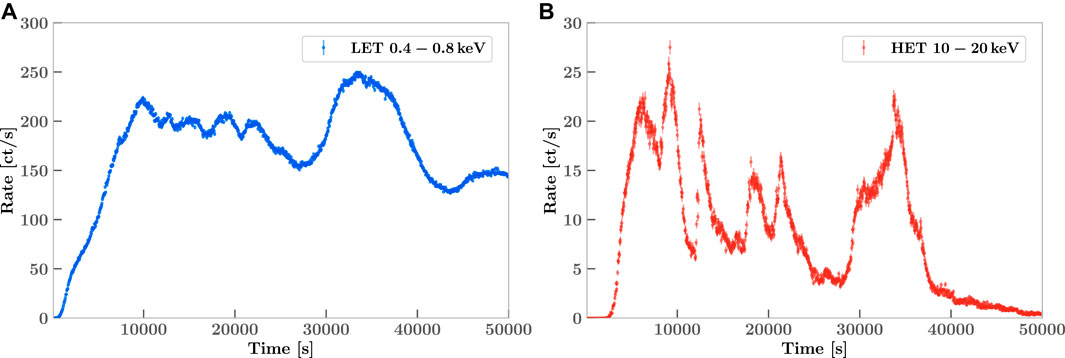
Figure 7. Simulated
Another aspect is the different inter-band variability among X-ray bands. The interplay between acceleration, cooling, and escape timescales of electrons at a shock front or dissipation region can produce different and characteristic patterns in the variation of spectral index vs. flux. For example, such variations can have different “hysteresis loops” (e.g., Kirk et al., 1998; Kirk and Mastichiadis, 1999; Böttcher and Chiang, 2002; Abeysekara et al., 2017; Wang et al., 2018; Böttcher, 2019; Chandra et al., 2021). The timescales of the flux rise and fall provide information on the acceleration mechanisms, if nearly instantaneous or progressive, and these could be different between hard and soft X-rays. So far, such studies have been limited to a few objects observed during large flares over 1 decade in energy. HEX-P will be able to investigate these relations over more than 2 decades in energy, and at lower fluxes and/or shorter timescales (see Madsen et al., 2023, for HEX-P repointing capabilities).
4.4 Synergies with other telescopes
4.4.1 CTAO
In HSP blazars, electrons accelerated to TeV energies emit by synchrotron typically in the X-ray band and by SSC in the VHE band. The possibility to perform extended simultaneous X-ray–VHE observations therefore provides a powerful diagnostic tool to understand particle acceleration and emission, allowing the evolution of a single freshly-accelerated electron population to be sampled by two different emission mechanisms. This is especially important during flares with hour and sub-hour variability. To perform such studies, an X-ray satellite must have a large visibility window at any given epoch for most of the sky and must be able to point in an antisolar direction. A large visibility window increases the chance that a source can be observed when it is found flaring, while the antisolar direction enables simultaneous observations at night with ground-based telescopes when the target culminates at the highest altitudes above the horizon, and thus can be followed by Cherenkov telescopes continuously under the best conditions for at least several hours. Such studies are not possible with XMM-Newton due to its strong solar panel constraints (Costamante, 2004). Chandra showed the potential for such studies with the detection of large flares by PKS 2155–304 in 2006, albeit with a much lower collecting area than XMM-Newon (Aharonian et al., 2009a). At an L1 orbit, HEX-P will fulfill all these operational requirements, allowing us to perform such studies for the first time with a sensitive, flagship-class X-ray telescope.
4.4.2 IXPE & COSI
High-energy polarization, a recently opened new window to the Universe, will play a very important role in future efforts to understand the matter content and high-energy emission processes in jets. In a leptohadronic scenario, emission at the trough can be dominated either by proton synchrotron or synchrotron from secondaries (Zhang H. et al., 2019), and hence it can be highly polarized in the X-ray and MeV bands. Recent IXPE observations have demonstrated that determining the shape of the X-ray spectrum is crucial to correctly recover the polarization parameters, regardless if X-rays are part of the synchrotron or high-energy spectrum (Ehlert et al., 2022; Di Gesu et al., 2022; Liodakis et al., 2022; Middei et al., 2023a; b). This is particularly important for sources like TXS 0506 + 056, i.e., ISP blazars, where the X-rays lie in the trough between the low- and high-energy humps of the SED (Peirson et al., 2022; 2023). Additionally, IceCube observations of TXS 0506 + 056 suggest that the hadronic spectral component, potentially peaking in the MeV band, can be variable (IceCube Collaboration et al., 2018). Therefore, both the spectral shape and variability of the hadronic contribution can have significant effects on the X-ray to MeV polarization degree and angle (Zhang et al., 2016), therefore requiring broadband coverage in X-ray and MeV bands. However, IXPE and future X-ray polarimeters, like the enhanced X-ray Timing and Polarimetry mission (eXTP), have limited energy ranges. For example, IXPE is sensitive in the 2–8 keV band. On the other hand, future MeV missions, such as the Compton Spectrometer and Imager (COSI, Tomsick et al., 2019), observe in the MeV band
4.4.3 SPT (CMB)
As discussed in Section 4.3, truly simultaneous, broad-band monitoring is key in unraveling blazar jets structure as well as the emission mechanisms and thus particle acceleration processes operating in blazars. One important spectral band is the millimeter regime, where, for the high-luminosity (FSRQ-type) blazars, the low-energy part of the SED peaks: blazars are generally strong mm sources and their mm emission can even be used to find counterparts of un-identified Fermi-LAT gamma-ray sources (see e.g., Zhang L. et al., 2022). This is the spectral regime where the blazar synchrotron emission is believed to become optically thin, and thus reveals the entire volume of the jet rather than its surface.
Time-resolved blazar studies in the mm band were usually conducted by individual radio/mm telescopes, generally dedicated to a single object at a time, and clearly revealed the large amplitude variability on time scales of days. Such studies also revealed a strong degree of polarization (in some cases, more than 20%), which is key in determining the orientation and uniformity of the magnetic field. Current and planned Cosmic Microwave Background (CMB) facilities will provide such data as a part of the CMB measurements: while the astrophysical sources are “noise” for CMB maps, the data – obtained “for free” – are very valuable for studies of such sources. This holds particularly true for blazars, since the current and planned CMB facilities collect data by continually scanning relatively large regions of the sky, and subsequently combining the data to form sky maps. Such time-resolved data result, generally, in a relatively well-sampled time series for many sources. Importantly, these CMB facilities, in addition to flux, also collect polarization information, thus providing time series in both intensity and polarization. One recent “proof of concept” example is observations of the blazar PKS 2326–502 with the South Pole Telescope (SPT; Hood et al., 2023) which revealed correlated flaring in the mm (at 150 GHz) and GeV-range gamma-ray bands, measured with the Fermi-LAT. For bright blazars in the millimeter band, SPT will provide a time-resolved flux and polarization time series in the SPT bands. Correlating such time series with hard X-ray flux will provide additional constraints on the origin of hard X-ray flux in blazars. Moreover, for HSP objects, the combination of SPT, HEX-P, and other observatories at IR/optical wavelengths would allow us to sample the energy distribution of the accelerated particles from the lowest to the highest energies, simultaneously sampling the SED over 5 orders of magnitude and informing us about the full particle populations in the jet.
The next-generation CMB facility is known as “CMB Stage 4” and is planned for deployment in the early 2030s. This matches well with the proposed timeline for HEX-P. CMB Stage 4, described in Carlstrom et al. (2019) and Abazajian et al. (2022)3 is significantly more sensitive than the current CMB facilities and, in its full form, will cover well over 50% of the sky. The facility will have detectors sensitive over the range of 30 GHz–280 GHz. While the details of the instrumental sensitivity are still under development, it is expected that in 24 h worth of sky scanning, CMB Stage 4 should be able to measure a source flux of 30 milliJansky (5
5 Source classes (MeV blazars, extreme BL lacs, and masquerading BL lacs)
5.1 The accretion flow to jet power connection
Flat Spectrum Radio Quasars are defined as blazars with strong broad emission lines. They are generally associated with strong jet emission, peaking at low frequencies both in synchrotron and inverse Compton components. Similar to low-peaked BL Lacs, their high-energy component dominates the X-ray emission, particularly the rising section of the hump. Depending on redshift, different parts of this emission are mapped with specific telescopes: among the most widely used X-ray instruments, Swift/XRT, XMM-Newton and the Chandra X-ray Observatory map the softer X-ray emission (
Even though FSRQs have been known and studied for more than 60 years, many open questions remain about their relativistic jets: how do they relate to the accretion flow, whether their launching system can be associated with it, and how the accretion-jet system evolves accross cosmic time. The better insight on the electron energy distribution, and a better mapping of the spectral evolution of the inverse Compton emission in time will already be a significant advancement in relativistic jet physics. Nonetheless, HEX-P’s wide energy coverage and deep sensitivity has the potential to make even bigger improvements on our knowledge.
The connection between accretion flow and the relativistic jet in blazars is in fact a key ingredient to understand the process behind jet launch and acceleration. Relativistic jets are generally associated with geometrically thick accretion flows when their launching mechanism is modelled: an accretion flow funnel close to the central black hole, along with a strong magnetic field, are effective ingredients to accelerate plasma along the polar direction (Tchekhovskoy et al., 2011). In contrast, a geometrically thin accretion disk has not historically been considered a solid basis for an effective jet collimation. FSRQs show that a relativistic jet can coexist with an optically thick, highly UV-luminous accretion flow, since they show a distinct and luminous optical-UV thermal continuum that cannot be justified with jet emission, with strong and broad emission lines, along with powerful relativistic jets. Such optical-UV features are most likely interpreted as coming from a geometrically thin, optically-thick accretion disk ionizing gas clouds in its vicinity (e.g., Padovani et al., 2017). Moreover, the direct correlation between jet and accretion power in blazars suggests a significant role in the jet launching process by the accretion disk, or at least in providing power for the overall process (Jolley and Kuncic, 2008; Ghisellini et al., 2014). The coexistence of these two structures is thus under deep scrutiny: coordinated multiwavelength observations of high-energy jet emission and optical-UV radiation from the disk are crucial to test the actual relation between disk and outflows. In particular, analogous to the cyclic X-ray binaries evolution, FSRQs might show variations in geometry and emission physics of the central part of their accretion flows, corresponding to jet launching or rebrightening. In case of an accretion rate fluctuation, a thin accretion disk might thicken towards its inner radii, providing a temporary funnel, allowing for significant magnetic field advection and ultimately accelerating a new emitting region in the relativistic jet. Coordinated fluctuations would also be expected during flux variations, and they would be easily identified during coordinated optical and X-ray monitoring. On the other hand, no thick component might be necessary: in this case, the launching mechanism might be different from what we currently expect, and this specific multiwavelength simultaneous or correlated variability would not be observed. Coordinated optical-UV and X-ray observations can thus provide unique insights into the most powerful engines in the Universe. HEX-P is crucial in this sense, being able to thoroughly map the jet emission profile and its evolution over a broad energy range. Changes in jet power or acceleration would be easily detectable thanks to changes in the inverse Compton profile, well explored by the combined effort of LET and HET.
A good knowledge of the emission profile of high-energy blazar SEDs is crucial to nail the connection between jet and accretion flow in these sources. A reliable representation of the median blazar SED is necessary to identify variations in the jet emission, possibly linked to accretion fluctuations. The current state-of-the-art is mainly focused at low redshift. Blazars with different jet powers are known to differ in their pre-peak inverse Compton slope and inverse Compton peak frequency. Even though the overall SED blazar shape is always characterized by the two prominent synchrotron and inverse Compton humps, their relative dominance and peak positions vary significantly depending on the jet power. Whether physically motivated or just an observing bias, blazars appear to peak at lower frequencies and have a harder X-ray spectrum when they are intrinsically more powerful. This has been tested among the known
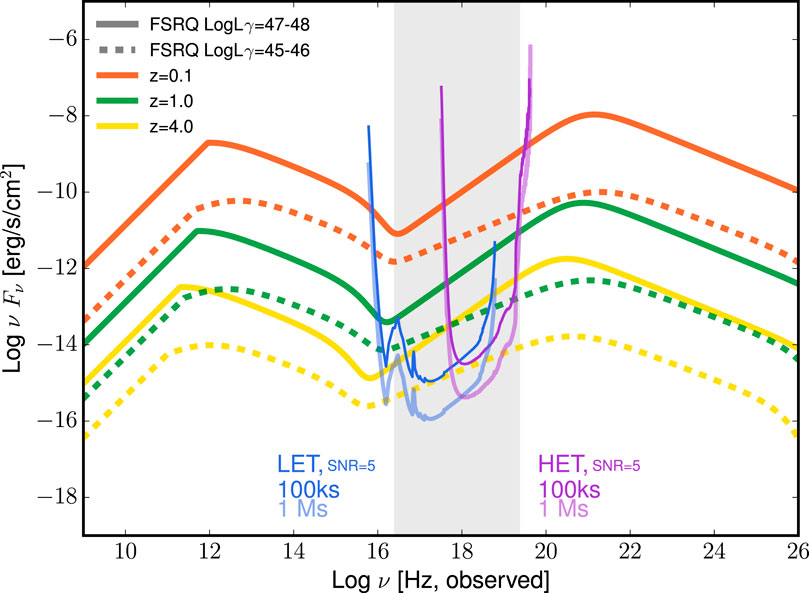
Figure 8. FSRQ SEDs with different powers and redshifts, compared with LET (blue) and HET (purple) differential sensitivities, calculated with 100 ks (thin, bright) and 1 Ms (thick, faint) exposures. The SEDs assume the Ghisellini et al. (2017) phenomenological models, corresponding to
5.2 Supermassive black holes at the dawn of time
Due to their high luminosities, we are able to trace blazars, particularly FSRQs, back to the early Universe. The entire broadband spectrum of these objects is shifted towards lower energies, and because of their high-energy hump peaking in the MeV band, they have been coined “MeV blazars” (Bloemen et al., 1995). These sources are more luminous in the hard X-ray band (Sbarrato et al., 2012), and studies made use of the NuSTAR satellite to analyse and characterise the emission processes responsible for the high-energy hump, in combination with Fermi-LAT at gamma-ray energies (Sbarrato et al., 2013; Tagliaferri et al., 2015; Marcotulli et al., 2017; 2020). The most puzzling findings about SMBHs hosted by quasars in the early Universe is that some of them exceed one billion solar masses, detected out to redshift of
A fraction of these SMBHs will power jets that, if pointing towards us, should appear as MeV blazars. In particular, the detection of these sources in the gamma-ray band is limited by the sensitivity of current instruments, paired with a very low flux due to the immense distances. Less than 30 high-redshift
AGN create feedback mechanisms in their host galaxies and can be linked to their evolution (Silk and Rees, 1998). On large scales, jets influence their surroundings by creating shocks in the surrounding gas (Schoenmakers et al., 2000; Rawlings and Jarvis, 2004; Shabala et al., 2011; Raouf et al., 2017), leading to truncation of star formation. Studying AGN-galaxy co-evolution in the early Universe has been difficult and so far has only been done up to redshift
5.3 Extreme BL lacs
Observations in the last 2 decades have shown that BL Lacs can reach much higher SED peak energies than previously thought, surpassing by 1–3 orders of magnitude or more the values of the bulk of the HSP population. They have been thus called extreme BL Lacs (Costamante et al., 2001). They come in two flavours: extreme synchrotron and extreme-TeV objects, depending on whether they show extreme peak energies in the synchrotron or gamma-ray emission. By convention, an HSP blazar starts to be called extreme if the
Conventional one-zone leptonic models (see also Section 4.1.1) struggle to model SEDs peaking at such high-energies, especially in gamma-rays. They generally require extreme conditions with very low magnetic fields, several orders of magnitude below equipartition, unusually hard electron distributions and very low radiative efficiency (Costamante et al., 2018; Biteau et al., 2020; Zech and Lemoine, 2021). The relation between extreme synchrotron and extreme TeV emission is still not well established: they should be correlated in a standard SSC scenario, but there are objects which are extreme in synchrotron but not in gamma-rays, and vice versa, although extreme-TeV sources do tend to display also extreme synchrotron peaks more often than the other way round (Biteau et al., 2020). Some objects show large peak variability, both in amplitude as well as in energy, with shifts by more than 3 orders of magnitude during flares (see, e.g., Mkn 501, Ahnen et al., 2018; MAGIC Collaboration et al., 2020). Others instead show rather steady extreme features over several years, a fact that could hint at the contribution of different mechanisms to the gamma-ray emission, in addition to inverse Compton emission by ultrarelativistic electrons (Biteau et al., 2020).
The location of the synchrotron peak in the SED and the slope of the spectrum around it are essential parameters to constrain the emission models and to study the origin of the peak itself (if due to cooling, escape, or the end of the accelerated particles’ distribution). So far, this has been possible only for peaks up to
Thanks to the unique combination of broad-band coverage, low background, and large collecting area from 0.2 to 80 keV with a single observation, HEX-P is ideally suited to identify and study these objects: it can directly measure or constrain the peak emission at flux levels or timescales one to two orders of magnitude lower than any current instrument (see Figure 9).
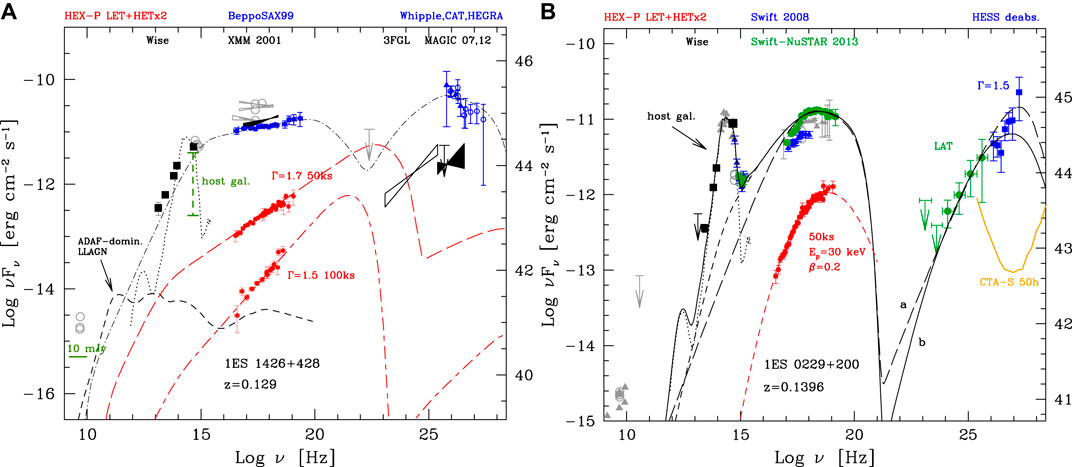
Figure 9. HEX-P simulated SEDs of two prototypical extreme BL Lacs, assuming lower flux levels. (A): 50 ks and 100 ks simulations of two extreme sources with hard power-law X-ray spectra, possibly peaking in the MeV range (adapted from Ghisellini, 1999b; Costamante et al., 2007). For reference, the figure shows also the average SED of an advection-dominated accretion flow low luminosity AGN (Nemmen et al., 2014) at
In this respect, the synergy with the upcoming CTAO—which will bring a similar improvement of one to two orders of magnitude in sensitivity at multi-TeV energies—cannot be understated. Besides characterizing VHE spectra over a wide energy band, simultaneous observations can test if and when these are indeed the same electrons emitting by two different mechanisms (synchrotron and SSC), as indicated by the tight correlations observed in HSPs down to minute timescales in Mkn 421 (Maraschi et al., 1999; Fossati et al., 2008; Baloković et al., 2016; MAGIC Collaboration et al., 2021) and PKS 2155
A crucial advancement enabled by HEX-P is in population studies of extreme blazars. Their overall luminosities tend to be low (
With 50 ks exposures, HEX-P can discover and characterize such sources down to a
For objects with peak fluxes around
CTAO is expected to discover many more TeV-emitting HSPs, as allowed by a 10-fold improvement in sensitivity at 1 TeV with respect to present arrays. Just from the planned CTAO extragalactic survey, which will cover
5.3.1 MeV-synchrotron BL lacs
In principle, blazars could reach even higher energies than shown by extreme BL Lacs. Expressing the acceleration time
There might be blazars where the acceleration reaches even higher rates than observed so far, yielding a synchrotron peak in the MeV range (Ghisellini, 1999b; a). For example,
The brightest among such objects could be detectable by COSI, but only HEX-P can pin-point and reveal the exceptional jet emission and extreme accelerator inside otherwise unremarkable objects. HEX-P can identify such a source with a peak luminosity of
5.4 Masquerading BL lacs
Masquerading BL Lacs are objects that are classified as BL Lacs because of the lack of broad emission lines in their optical spectra, but are very likely FSRQs in disguise (Giommi et al., 2013). In these objects the jet is well aligned with our line of sight and its synchrotron emission overwhelms the disk and broad line region (BLR) emission, making the latter undetectable. Several spectroscopic and photometric campaigns have started collecting accurate redshift measurements of BL Lac sources detected in
HEX-P’s deep, broadband sensitivity is a unique advantage compared to other instruments for studying masquerading BL Lacs. By measuring the synchrotron component to high energies, HEX-P can put constraints on the maximum Lorentz factor
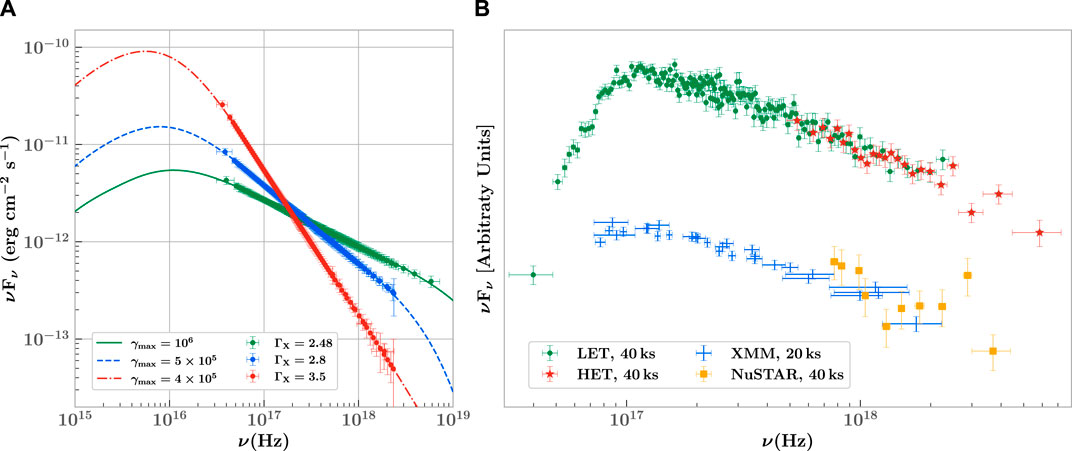
Figure 10. (A): Simulated SED of the high-redshift BL Lac 4FGL J2146.5–1,344 (
5.5 Blazar evolution
As a source class, blazars have been shown to follow different evolutionary paths in the X-ray band. The powerful FSRQs evolve positively (i.e., there is an increasing number of sources at higher redshift, and/or the sources’ luminosity increases with redshift) up to redshift
The latest blazar evolutionary model in the hard X-ray band has confirmed that the peak of the evolution of the most luminous FSRQs
Using the latest hard X-ray luminosity function, we calculated the number of blazars detectable at different redshift bins in a 1
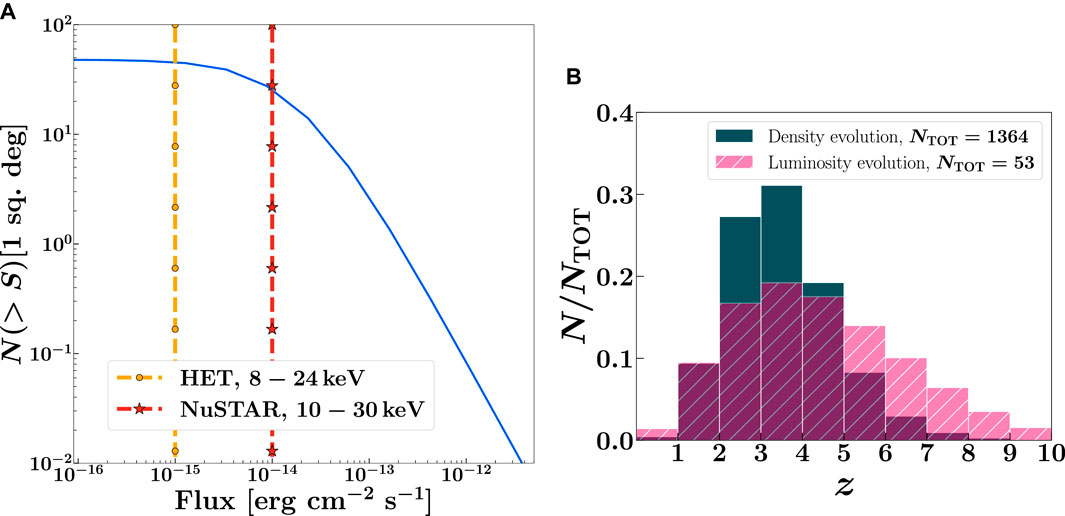
Figure 11. (A): Blazar source count distribution, logN-logS (blue line), derived from the best-fit luminosity evolution model of Marcotulli et al. (2022) expected for a 1
Moreover, these sources, though contributing only
Similarly, the prospects to finally disentangle the preferred evolutionary paths for BL Lacs in the X-ray band are bright with HEX-P. Current photometric and spectroscopic follow-up of
6 Resolving nearby jets
6.1 Blazars
Jets of active galaxies can be hundreds to thousands of kiloparsecs long (e.g., Oei et al., 2022). The extended structure is most easily observed in the radio band, where the low-energy synchrotron emission can be observed along the entire jet. However, many jets also show extended components (extended jets and/or hotspots along the jet flow) in other energy bands, including the X-ray domain. Observations with Chandra increased the number of resolved X-ray jets considerably and rekindled the debate on the X-ray emission processes in extended jets (see Harris and Krawczynski, 2006, for a review). Especially the explanation of hard X-ray spectra (harder than the radio-through-optical extrapolation) was strongly debated. Two main models emerged: Inverse-Compton scattering of CMB photons by low-energy electrons (which would produce the radio through optical emission through synchrotron emission; Tavecchio et al., 2000) or synchrotron emission of a second, high-energy electron population (e.g., Hardcastle, 2006; Jester et al., 2006). While the IC/CMB model was favored for some time, several studies over recent years have shown that this may not be the correct interpretation for many sources due to a lack of associated
For example, an extended X-ray jet has been observed in the flat-spectrum radio quasar 3C 273 (Marshall et al., 2001), revealing a
However, in a notable counterexample, the synchrotron explanation probably does not work for the blazar AP Librae (Zacharias and Wagner, 2016; Roychowdhury et al., 2022), one of the few BL Lac objects with a resolved,
A recent study by Reddy et al. (2023) on jet knots revealed that in most knots the X-ray emission peak is located upstream of the radio emission peak. This might suggest a cooling effect on the particle flow across a shock pointing towards the synchrotron process in both energy bands, as the IC/CMB model requires a one-zone interpretation, where the fluxes should peak at the same location.
Hence, the debate continues. Observations with HEX-P will be crucial for studies of the hard X-ray emission of nearby, resolved blazar jets. The X-ray spectra do not show any spectral feature; they are described well with a power-law (e.g., Haardt et al., 1999; Marshall et al., 2001; Kaufmann et al., 2013). This suggests efficient particle acceleration in large-scale jets potentially up to the synchrotron-burnoff limit (de Jager et al., 1996). Unfortunately, the spectral range of the synchrotron-burnoff limit (
Using the 47 sources labeled as BL Lac or CDQ in the Atlas-X4 (Reddy et al., 2023) and the XJET5 catalogs (most of which are blazars), we determine that HEX-P’s angular resolution of
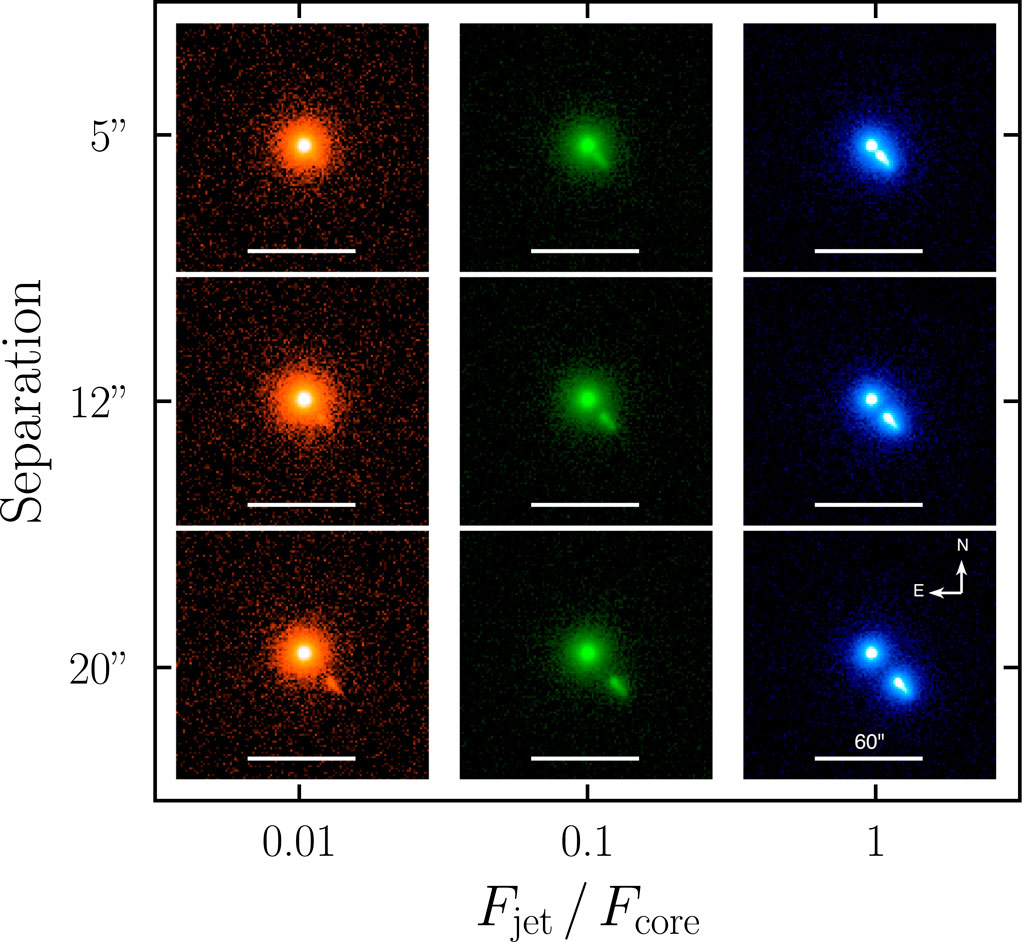
Figure 12. SIXTE simulations using as base image the blazar 3C 273 (Chandra obsid:4,876). All the images have been produced using 100 ks exposure with the LET instrument and they cover the
This implies that HEX-P will help determine the spectral features (e.g., breaks or cut-offs, if present) at X-rays beyond
6.2 TeV-detected radio galaxies
Radio galaxies are typically non-aligned AGN with significantly reduced Doppler beaming. Depending on the X-ray production process, their extended X-ray jets may still be observable, as beaming is not as important depending on the particle energies in the jet. Here, we discuss a special class of radio galaxies, namely, those which are detected at VHE
Observations with HEX-P of these extended jets, coupled with the knowledge that these jets emit TeV
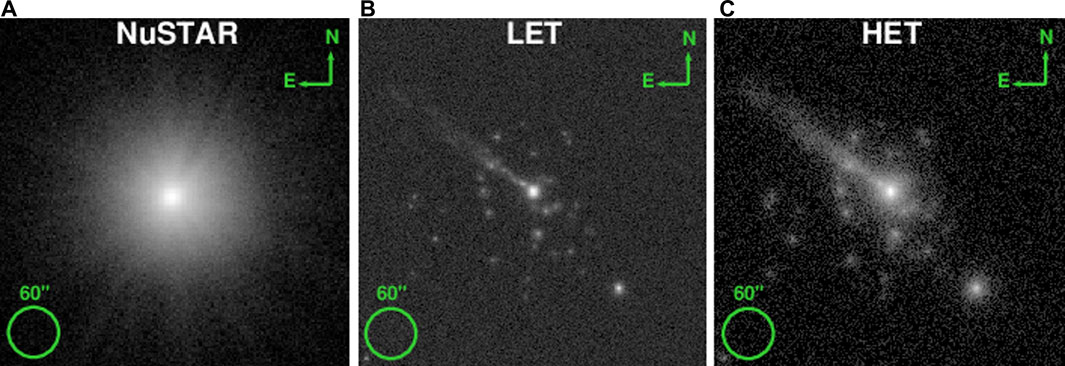
Figure 13. (A): NuSTAR FPMA
Along with HEX-P and its broad X-ray coverage, multiwavelength observations with future high-angular-resolution instruments will be crucial to unlock the secrets of these objects. Most notably, collaborative observations by HEX-P with CTAO will be important to constrain the jet morphology and processes.
7 Conclusion
The HEX-P mission offers several key strengths for studying jetted AGN. Its broad-band sensitivity, spanning from 0.2 to 80 keV, provides deep coverage in relatively short exposure times. Additionally, HEX-P exhibits excellent angular resolution at high energies and the unique capability to point in the antisolar direction. These remarkable capabilities set HEX-P apart from both current and currently planned X-ray observatories, making it an unparalleled asset for the study of blazars in the next decade. In particular:
Lastly, HEX-P exhibits strong synergies with current and future planned facilities, including CTAO, IceCube, IXPE, COSI, and the current and future CMB facilities (Section 4.4). These collaborations enable the study of extremely fast flaring blazars, the detection and study of multi-messenger sources, and the investigation of hadronic emission components in blazars. An early 2030s launch of HEX-P will significantly enhance the science provided by those facilities and answer many key questions about the most powerful jets in the Universe.
Data availability statement
The original contributions presented in the study are included in the article, further inquiries can be directed to the corresponding author.
Author contributions
LM: Writing–original draft, Writing–review and editing. MA: Writing–original draft, Writing–review and editing. MB: Writing–original draft, Writing–review and editing. PC: Writing–review and editing. LC: Writing–original draft, Writing–review and editing. LG: Writing–review and editing. ME: Writing–original draft, Writing–review and editing. JG: Writing–review and editing. AG: Writing–original draft, Writing–review and editing. IL: Writing–original draft, Writing–review and editing. GM: Writing–original draft, Writing–review and editing. KM: Writing–review and editing. AM: Writing–review and editing. RM: Writing–review and editing. FM: Writing–original draft, Writing–review and editing. MP: Writing–original draft, Writing–review and editing. BR: Writing–review and editing. TS: Writing–original draft, Writing–review and editing. DS: Writing–review and editing. GV: Writing–original draft. MZ: Writing–original draft, Writing–review and editing. HZ: Writing–original draft, Writing–review and editing.
Funding
The author(s) declare that financial support was received for the research, authorship, and/or publication of this article. The work of D.S. was carried out at the Jet Propulsion Laboratory, California Institute of Technology, under a contract with NASA. Support for this work was provided by NASA through the NASA Hubble Fellowship grant #HST-HF2-51486.001-A awarded by the Space Telescope Science Institute, which is operated by the Association of Universities for Research in Astronomy, Inc., for NASA, under contract NAS5-26555. IL was supported by the NASA Postdoctoral Program at the Marshall Space Flight Center, administered by Oak Ridge Associated Universities under contract with NASA. MZ acknowledges funding by the Deutsche Forschungsgemeinschaft (DFG, German Reserach Foundation)–project number 460248186 (PUNCH4NFDI). RM acknowledges financial support from the ASI-INAF agreement n. 2022-14-HH.0. HZ is supported by NASA under award number 80GSFC21M0002. HZ’s work is supported by Fermi GI program cycle 16 under the award number 22-FERMI22-0015.
Acknowledgments
We thank both anonymous referees for their meaningful comments that helped us to improve the manuscript.
Conflict of interest
The authors declare that the research was conducted in the absence of any commercial or financial relationships that could be construed as a potential conflict of interest.
The reviewer FT declared a shared affiliation with the author(s) AM, MR and TS to the handling editor at the time of review.
Publisher’s note
All claims expressed in this article are solely those of the authors and do not necessarily represent those of their affiliated organizations, or those of the publisher, the editors and the reviewers. Any product that may be evaluated in this article, or claim that may be made by its manufacturer, is not guaranteed or endorsed by the publisher.
Footnotes
1https://hexp.org/response-files/
2https://cor.gsfc.nasa.gov/Astro2020/26141.pdf
4https://astro.umbc.edu/Atlas-X/
5https://hea-www.cfa.harvard.edu/XJET/
References
Abazajian, K., Addison, G. E., Adshead, P., Ahmed, Z., Akerib, D., Ali, A., et al. (2022). CMB-S4: forecasting constraints on primordial gravitational waves. Astrophys. J. 926, 54. doi:10.3847/1538-4357/ac1596
Abdo, A. A., Ackermann, M., Agudo, I., Ajello, M., Aller, H. D., Aller, M. F., et al. (2010a). The spectral energy distribution of Fermi bright blazars. Astrophys. J. 716, 30–70. doi:10.1088/0004-637X/716/1/30
Abdo, A. A., Ackermann, M., Ajello, M., Antolini, E., Baldini, L., Ballet, J., et al. (2010b). Suzaku observations of luminous quasars: revealing the nature of high-energy blazar emission in low-level activity states. Astrophys. J. 716, 835–849. doi:10.1088/0004-637X/716/1/835
Abdo, A. A., Ackermann, M., Ajello, M., Atwood, W. B., Axelsson, M., Baldini, L., et al. (2010c). Spectral properties of bright fermi-detected blazars in the gamma-ray band. Astrophys. J. 710, 1271–1285. doi:10.1088/0004-637X/710/2/1271
Abdollahi, S., Acero, F., Ackermann, M., Ajello, M., Atwood, W. B., Axelsson, M., et al. (2020). Fermi large area telescope fourth source catalog. Astrophys. J. Suppl. Ser. 247, 33. doi:10.3847/1538-4365/ab6bcb
Abdollahi, S., Ackermann, M., Ajello, M., Atwood, W. B., Baldini, L., Ballet, J., et al. (2018). A gamma-ray determination of the Universe’s star formation history. Science 362, 1031–1034. doi:10.1126/science.aat8123
Abeysekara, A. U., Archambault, S., Archer, A., Benbow, W., Bird, R., Buchovecky, M., et al. (2017). A search for spectral hysteresis and energy-dependent time lags from X-ray and TeV gamma-ray observations of mrk 421. Astrophys. J. 834, 2. doi:10.3847/1538-4357/834/1/2
Abramowski, A., Acero, F., Aharonian, F., Akhperjanian, A. G., Anton, G., Balzer, A., et al. (2012). the 2010 very high energy γ-ray flare and 10 years of multi-wavelength observations of m 87. Astrophys. J. 746, 151. doi:10.1088/0004-637X/746/2/151
Acciari, V. A., Aliu, E., Arlen, T., Bautista, M., Beilicke, M., Benbow, W., et al. (2009). Radio imaging of the very-high-energy γ-ray emission region in the central engine of a radio galaxy. Science 325, 444–448. doi:10.1126/science.1175406
Acero, F., Ackermann, M., Ajello, M., Albert, A., Atwood, W. B., Axelsson, M., et al. (2015). Fermi large area telescope third source catalog. Astrophys. J. Suppl. Ser. 218, 23. doi:10.1088/0067-0049/218/2/23
Ackermann, M., Ajello, M., Allafort, A., Schady, P., Baldini, L., Ballet, J., et al. (2012). The imprint of the extragalactic background light in the gamma-ray spectra of blazars. Science 338, 1190–1192. doi:10.1126/science.1227160
Ackermann, M., Ajello, M., Baldini, L., Ballet, J., Barbiellini, G., Bastieri, D., et al. (2017). Gamma-ray blazars within the first 2 billion years. Astrophys. J. Lett. 837, L5. doi:10.3847/2041-8213/aa5fff
Ackermann, M., Anantua, R., Asano, K., Baldini, L., Barbiellini, G., Bastieri, D., et al. (2016a). Minute-timescale >100 MeV γ-Ray variability during the giant outburst of quasar 3C 279 observed by fermi-LAT in 2015 june 824, L20. doi:10.3847/2041-8205/824/2/L20
Ackermann, M., Anantua, R., Asano, K., Baldini, L., Barbiellini, G., Bastieri, D., et al. (2016b). MINUTE-TIMESCALE >100 MeV γ-RAY VARIABILITY DURING THE GIANT OUTBURST OF QUASAR 3C 279 OBSERVED BY FERMI-LAT IN 2015 JUNE. Astrophys. J. Lett. 824, L20. doi:10.3847/2041-8205/824/2/L20
Agarwal, A., Gupta, A. C., Bachev, R., Strigachev, A., Semkov, E., Wiita, P. J., et al. (2015). Multiband optical-NIR variability of blazars on diverse time-scales. Mon. Not. R. Astron. Soc. 451, 3882–3897. doi:10.1093/mnras/stv1208
Aharonian, F., Akhperjanian, A. G., Anton, G., Barres de Almeida, U., Bazer-Bachi, A. R., Becherini, Y., et al. (2009a). Simultaneous multiwavelength observations of the second exceptionalγ-ray flare of PKS 2155–304 in July 2006. Astron. Astrophys. 502, 749–770. doi:10.1051/0004-6361/200912128
Aharonian, F., Akhperjanian, A. G., Anton, G., de Almeida, U. B., Bazer-Bachi, A. R., Becherini, Y., et al. (2009b). discovery of very high energy γ-ray emission from centaurus a with h.e.s.s. Astrophys. J. Lett. 695, L40–L44. doi:10.1088/0004-637X/695/1/L40
Aharonian, F., Akhperjanian, A. G., Barres de Almeida, U., Bazer-Bachi, A. R., Behera, B., Beilicke, M., et al. (2007a). New constraints on the mid-IR EBL from the HESS discovery of VHE γ-rays from 1ES 0229+200. Astron. Astrophys. 475, L9–L13. doi:10.1051/0004-6361:20078462
Aharonian, F., Akhperjanian, A. G., Bazer-Bachi, A. R., Behera, B., Beilicke, M., Benbow, W., et al. (2007b). An exceptional very high energy gamma-ray flare of PKS 2155-304. Astrophys. J. Lett. 664, L71–L74. doi:10.1086/520635
Aharonian, F., Akhperjanian, A. G., Bazer-Bachi, A. R., Beilicke, M., Benbow, W., Berge, D., et al. (2006). Fast variability of Tera–Electron volt γ rays from the radio galaxy M87. Science 314, 1424–1427. doi:10.1126/science.1134408
Aharonian, F. A. (2004). Very high energy cosmic gamma radiation: a crucial window on the extreme Universe. World Scientific. doi:10.1142/4657
Aharonian, F. A., Barkov, M. V., and Khangulyan, D. (2017). Scenarios for ultrafast gamma-ray variability in AGN. Astrophys. J. 841, 61. doi:10.3847/1538-4357/aa7049
Ahnen, M. L., Ansoldi, S., Antonelli, L. A., Arcaro, C., Babić, A., Banerjee, B., et al. (2018). Extreme HBL behavior of markarian 501 during 2012. Astron. Astrophys. 620, A181. doi:10.1051/0004-6361/201833704
Ajello, M., Angioni, R., Axelsson, M., Ballet, J., Barbiellini, G., Bastieri, D., et al. (2020). The fourth catalog of active galactic nuclei detected by the Fermi large area telescope. Astrophys. J. 892, 105. doi:10.3847/1538-4357/ab791e
Ajello, M., Costamante, L., Sambruna, R. M., Gehrels, N., Chiang, J., Rau, A., et al. (2009). The evolution of Swift/BAT blazars and the origin of the MeV background. Astrophys. J. 699, 603–625. doi:10.1088/0004-637X/699/1/603
Ajello, M., Greiner, J., Sato, G., Willis, D. R., Kanbach, G., Strong, A. W., et al. (2008). Cosmic X-ray background and Earth albedo spectra with Swift BAT. Astrophys. J. 689, 666–677. doi:10.1086/592595
Ajello, M., Romani, R. W., Gasparrini, D., Shaw, M. S., Bolmer, J., Cotter, G., et al. (2014). The cosmic evolution of Fermi BL Lacertae objects. Astrophys. J. 780, 73. doi:10.1088/0004-637X/780/1/73
Albert, J., Aliu, E., Anderhub, H., Antoranz, P., Armada, A., Baixeras, C., et al. (2007). Variable very high energy γ-Ray emission from markarian 501 669, 862–883. doi:10.1086/521382
Aleksić, J., Ansoldi, S., Antonelli, L. A., Antoranz, P., Babic, A., Bangale, P., et al. (2014a). Black hole lightning due to particle acceleration at subhorizon scales. Science 346, 1080–1084. doi:10.1126/science.1256183
Aleksić, J., Antonelli, L. A., Antoranz, P., Babic, A., Barres de Almeida, U., Barrio, J. A., et al. (2014b). Rapid and multiband variability of the TeV bright active nucleus of the galaxy IC 310. Astron. Astrophys. 563, A91. doi:10.1051/0004-6361/201321938
Alexander, T., and Natarajan, P. (2014). Rapid growth of seed black holes in the early universe by supra-exponential accretion. Science 345, 1330–1333. doi:10.1126/science.1251053
Aliu, E., Archambault, S., Archer, A., Arlen, T., Aune, T., Barnacka, A., et al. (2016). Very high energy outburst of Markarian 501 in May 2009. Astron. Astrophys. 594, A76. doi:10.1051/0004-6361/201628744
Allison, P., Bard, R., Beatty, J. J., Besson, D. Z., Bora, C., Chen, C. C., et al. (2016). Performance of two Askaryan Radio Array stations and first results in the search for ultrahigh energy neutrinos. Phys. Rev. D. 93, 082003. doi:10.1103/physrevd.93.082003
Álvarez Crespo, N., Masetti, N., Ricci, F., Landoni, M., Patiño-Álvarez, V., Massaro, F., et al. (2016a). Optical spectroscopic observations of gamma-ray blazar candidates. V. TNG, KPNO, and OAN observations of blazar candidates of uncertain type in the northern hemisphere. Astron. J. 151, 32. doi:10.3847/0004-6256/151/2/32
Álvarez Crespo, N., Massaro, F., Milisavljevic, D., Landoni, M., Chavushyan, V., Patiño-Álvarez, V., et al. (2016b). Optical spectroscopic observations of gamma-ray blazar candidates. VI. Further observations from TNG, WHT, OAN, SOAR, and magellan telescopes. Astron. J. 151, 95. doi:10.3847/0004-6256/151/4/95
Ananna, T. T., Treister, E., Urry, C. M., Ricci, C., Hickox, R. C., Padmanabhan, N., et al. (2020). Accretion history of AGNs. II. Constraints on AGN spectral parameters using the cosmic X-ray background. Astrophys. J. 889, 17. doi:10.3847/1538-4357/ab5aef
Archer, A., Benbow, W., Bird, R., Brill, A., Buchovecky, M., Buckley, J. H., et al. (2020). VERITAS discovery of VHE emission from the radio galaxy 3C 264: a multiwavelength study. Astrophys. J. 896, 41. doi:10.3847/1538-4357/ab910e
Arnaud, K. A. (1996). “XSPEC: the first ten years,” in Astronomical data analysis software and systems V. Editors G. H. Jacoby, and J. Barnes (Astronomical Society of the Pacific Conference Series), 101.
Atoyan, A., and Dermer, C. D. (2004). Synchrotron versus compton interpretations for extended X-ray jets. Astrophys. J. 613, 151–158. doi:10.1086/422499
Baloković, M., Paneque, D., Madejski, G., Furniss, A., Chiang, J., Ajello, M., et al. (2016). Multiwavelength study of quiescent states of mrk 421 with unprecedented hard X-ray coverage provided by NuSTAR in 2013. Astrophys. J. 819, 156. doi:10.3847/0004-637X/819/2/156
Barkov, M. V., Aharonian, F. A., Bogovalov, S. V., Kelner, S. R., and Khangulyan, D. (2012). Rapid TeV variability in blazars as a result of jet-star interaction. Astrophys. J. 749, 119. doi:10.1088/0004-637X/749/2/119
Bassett, L. C., Brandt, W. N., Schneider, D. P., Vignali, C., Chartas, G., and Garmire, G. P. (2004). Chandra observations of radio-loud quasars at z>4: X-rays from the radio beacons of the early universe. Astron. J. 128, 523–533. doi:10.1086/422019
Beckmann, V., Engels, D., Bade, N., and Wucknitz, O. (2003). The HRX-BL lac sample - evolution of BL lac objects. Astron. Astrophys. 401, 927–938. doi:10.1051/0004-6361:20030184
Beckmann, V., Wolter, A., Celotti, A., Costamante, L., Ghisellini, G., Maccacaro, T., et al. (2002). BeppoSAX spectral survey of BL Lacs - new spectra and results. Astron. Astrophys. 383, 410–422. doi:10.1051/0004-6361:20011752
Begelman, M. C., Fabian, A. C., and Rees, M. J. (2008). Implications of very rapid TeV variability in blazars. Mon. Not. R. Astron. Soc. 384, L19–L23. doi:10.1111/j.1745-3933.2007.00413.x
Begelman, M. C., Rudak, B., and Sikora, M. (1990). Consequences of relativistic proton injection in active galactic nuclei. Astrophys. J. 362, 38. doi:10.1086/169241
Begelman, M. C., and Volonteri, M. (2017). Hyperaccreting black holes in galactic nuclei. Mon. Not. R. Astron. Soc. 464, 1102–1107. doi:10.1093/mnras/stw2446
Begelman, M. C., Volonteri, M., and Rees, M. J. (2006). Formation of supermassive black holes by direct collapse in pre-galactic haloes. Mon. Not. R. Astron. Soc. 370, 289–298. doi:10.1111/j.1365-2966.2006.10467.x
Belladitta, S., Moretti, A., Caccianiga, A., Spingola, C., Severgnini, P., Della Ceca, R., et al. (2020). The first blazar observed at z > 6. Astron. Astrophys. 635, L7. doi:10.1051/0004-6361/201937395
Berezinskii, V. S., and Ginzburg, V. L. (1981). On high-energy neutrino radiation of quasars and active galactic nuclei. Mon. Not. R. Astron. Soc. 194, 3–14. doi:10.1093/mnras/194.1.3
Bhatta, G., Mohorian, M., and Bilinsky, I. (2018). Hard X-ray properties of NuSTAR blazars. Astron. Astrophys. 619, A93. doi:10.1051/0004-6361/201833628
Biermann, P. L., and Strittmatter, P. A. (1987). Synchrotron emission from shock waves in active galactic nuclei. Astrophys. J. 322, 643. doi:10.1086/165759
Biteau, J., Prandini, E., Costamante, L., Lemoine, M., Padovani, P., Pueschel, E., et al. (2020). Progress in unveiling extreme particle acceleration in persistent astrophysical jets. Nat. Astron. 4, 124–131. doi:10.1038/s41550-019-0988-4
Blandford, R., Meier, D., and Readhead, A. (2019). Relativistic jets from active galactic nuclei. ARAA 57, 467–509. doi:10.1146/annurev-astro-081817-051948
Blinov, D., Kiehlmann, S., Pavlidou, V., Panopoulou, G. V., Skalidis, R., Angelakis, E., et al. (2021). RoboPol: AGN polarimetric monitoring data. Mon. Not. R. Astron. Soc. 501, 3715–3726. doi:10.1093/mnras/staa3777
Bloemen, H., Bennett, K., Blom, J. J., Collmar, W., Hermsen, W., Lichti, G. G., et al. (1995). New COMPTEL gamma-ray source (GRO J0516-609) near PKS 0506-612/0522-611: first evidence for “MeV Blazars”. Astron. Astrophys. 293, L1–L4.
Boccardi, B., Krichbaum, T. P., Bach, U., Bremer, M., and Zensus, J. A. (2016). First 3 mm-VLBI imaging of the two-sided jet in Cygnus A. Zooming into the launching region. Astron. Astrophys. 588, L9. doi:10.1051/0004-6361/201628412
Boella, G., Butler, R. C., Perola, G. C., Piro, L., Scarsi, L., and Bleeker, J. A. M. (1997). BeppoSAX, the wide band mission for X-ray astronomy. Astron. Astrophys. Suppl. Ser. 122, 299–307. doi:10.1051/aas:1997136
Boller, T., Freyberg, M. J., Trümper, J., Haberl, F., Voges, W., and Nandra, K. (2016). Second ROSAT all-sky survey (2RXS) source catalogue. Astron. Astrophys. 588, A103. doi:10.1051/0004-6361/201525648
Böttcher, M. (2019). Progress in multi-wavelength and multi-messenger observations of blazars and theoretical challenges. Galaxies 7, 20. doi:10.3390/galaxies7010020
Böttcher, M., and Chiang, J. (2002). X-ray spectral variability signatures of flares in BL Lacertae objects. Astrophys. J. 581, 127–142. doi:10.1086/344155
Böttcher, M., Reimer, A., Sweeney, K., and Prakash, A. (2013). Leptonic and hadronic modeling of fermi-detected blazars. Astrophys. J. 768, 54. doi:10.1088/0004-637X/768/1/54
Boula, S., and Mastichiadis, A. (2022). Expanding one-zone model for blazar emission. Astron. Astrophys. 657, A20. doi:10.1051/0004-6361/202142126
Breiding, P., Meyer, E. T., Georganopoulos, M., Keenan, M. E., DeNigris, N. S., and Hewitt, J. (2017). Fermi non-detections of four X-ray jet sources and implications for the IC/CMB mechanism. Astrophys. J. 849, 95. doi:10.3847/1538-4357/aa907a
Bromm, V., and Loeb, A. (2003). Formation of the first supermassive black holes. Astrophys. J. 596, 34–46. doi:10.1086/377529
Brunner, H., Liu, T., Lamer, G., Georgakakis, A., Merloni, A., Brusa, M., et al. (2022). The eROSITA final equatorial depth survey (eFEDS). X-Ray catalogue. Astron. Astrophys. 661, A1. doi:10.1051/0004-6361/202141266
Buson, S., Tramacere, A., Pfeiffer, L., Oswald, L., de Menezes, R., Azzollini, A., et al. (2022). Beginning a journey across the universe: the discovery of extragalactic neutrino factories. Astrophys. J. Lett. 933, L43. doi:10.3847/2041-8213/ac7d5b
Caccianiga, A., Moretti, A., Belladitta, S., Della Ceca, R., Antón, S., Ballo, L., et al. (2019). The space density of z > 4 blazars. Mon. Not. R. Astron. Soc. 484, 204–217. doi:10.1093/mnras/sty3526
Cappelluti, N., Li, Y., Ricarte, A., Agarwal, B., Allevato, V., Tasnim Ananna, T., et al. (2017). The Chandra COSMOS legacy survey: energy spectrum of the cosmic X-ray background and constraints on undetected populations. Astrophys. J. 837, 19. doi:10.3847/1538-4357/aa5ea4
Caputo, R., Ajello, M., Kierans, C., Perkins, J., Racusin, J., Baldini, L., et al. (2022). The all-sky medium energy gamma-ray observatory eXplorer (AMEGO-X) mission concept. arXiv e-prints , arXiv:2208.04990.
Carlstrom, J., Abazajian, K., Addison, G., Adshead, P., Ahmed, Z., Allen, S. W., et al. (2019). CMB-S4. Bull. Am. Astron. Soc. 51, 209. doi:10.48550/arXiv.1908.01062
Catanese, M., and Sambruna, R. M. (2000). A rapid X-ray flare from markarian 501. Astrophys. J. Lett. 534, L39–L42. doi:10.1086/312637
Celotti, A., Ghisellini, G., and Fabian, A. C. (2007). Bulk Comptonization spectra in blazars. Mon. Not. R. Astron. Soc. 375, 417–424. doi:10.1111/j.1365-2966.2006.11289.x
Cerruti, M., Zech, A., Boisson, C., and Inoue, S. (2015). A hadronic origin for ultra-high-frequency-peaked BL Lac objects. Mon. Not. R. Astron. Soc. 448, 910–927. doi:10.1093/mnras/stu2691
Cerutti, B., Werner, G. R., Uzdensky, D. A., and Begelman, M. C. (2012). Beaming and rapid variability of high-energy radiation from relativistic pair plasma reconnection. Astrophys. J. Lett. 754, L33. doi:10.1088/2041-8205/754/2/L33
Chandra, S., Boettcher, M., Goswami, P., Singh, K. P., Zacharias, M., Kaur, N., et al. (2021). X-ray observations of 1ES 1959+650 in its high-activity state in 2016-2017 with AstroSat and Swift. Astrophys. J. 918, 67. doi:10.3847/1538-4357/ac01d1
Chartas, G., Worrall, D. M., Birkinshaw, M., Cresitello-Dittmar, M., Cui, W., Ghosh, K. K., et al. (2000). The Chandra X-ray observatory resolves the X-ray morphology and spectra of a jet in PKS 0637-752. Astrophys. J. 542, 655–666. doi:10.1086/317049
Chase, O., McBride, F., Gokus, A., Lucchini, M., Zhang, H., Ojha, R., et al. (2023). The peculiar variable X-ray spectrum of the active galactic nucleus PKS 2005-489. Astrophys. J. 948, 2. doi:10.3847/1538-4357/acc575
Chatterjee, R., Das, S., Khasnovis, A., Ghosh, R., Kumari, N., Naik, S., et al. (2021). Short-timescale variability of the blazar Mrk 421 from AstroSat and simultaneous multi-wavelength observations. J. Astrophys. Astron. 42, 80. doi:10.1007/s12036-021-09709-3
Cherenkov Telescope Array Consortium Acharya, B. S., Agudo, I., Al Samarai, I., Alfaro, R., Alfaro, J., et al. (2019). Science with the Cherenkov telescope Array. World Scientific. doi:10.1142/10986
Christie, I. M., Petropoulou, M., Sironi, L., and Giannios, D. (2019). Radiative signatures of plasmoid-dominated reconnection in blazar jets. Mon. Not. R. Astron. Soc. 482, 65–82. doi:10.1093/mnras/sty2636
Civano, F., Zhao, X., Boorman, P., Marchesi, S., Ananna, T., Creech, S., et al. (2023). The high energy X-ray probe (HEX-P): bringing the cosmic X-ray background into focus. arXiv e-prints, 04832doi. arXiv:2311. doi:10.48550/arXiv.2311.04832
Connor, T., Bañados, E., Stern, D., Carilli, C., Fabian, A., Momjian, E., et al. (2021). Enhanced X-ray emission from the most radio-powerful quasar in the universe’s first billion years. Astrophys. J. 911, 120. doi:10.3847/1538-4357/abe710
Costamante, L. (2004). A brief (blazar oriented) overview on topics for multiwavelength observations with TeV photons. NAR 48, 497–503. doi:10.1016/j.newar.2003.12.029
Costamante, L. (2020). TeV-peaked candidate BL Lac objects. Mon. Not. R. Astron. Soc. 491, 2771–2778. doi:10.1093/mnras/stz3018
Costamante, L., Aharonian, F., and Khangulyan, D. (2007). “GLAST answers about high-energy peaked BL Lacs: double-humped γ-ray peak and extreme accelerators,” in The first GLAST symposium. Editors S. Ritz, P. Michelson, and C. A. Meegan (American Institute of Physics Conference Series), 921, 157–159. doi:10.1063/1.2757291
Costamante, L., Bonnoli, G., Tavecchio, F., Ghisellini, G., Tagliaferri, G., and Khangulyan, D. (2018). The nustar view on hard-tev bl lacs. Mon. Not. R. Astron. Soc. 477, 4257–4268. doi:10.1093/mnras/sty857
Costamante, L., Ghisellini, G., Giommi, P., Tagliaferri, G., Celotti, A., Chiaberge, M., et al. (2001). Extreme synchrotron BL Lac objects. Stretching the blazar sequence. Astron. Astrophys. 371, 512–526. doi:10.1051/0004-6361:20010412
Dauser, T., Falkner, S., Lorenz, M., Kirsch, C., Peille, P., Cucchetti, E., et al. (2019). SIXTE: a generic X-ray instrument simulation toolkit. Astron. Astrophys. 630, A66. doi:10.1051/0004-6361/201935978
De Angelis, A., Tatischeff, V., Tavani, M., Oberlack, U., Grenier, I., Hanlon, L., et al. (2017). The e-ASTROGAM mission. Exploring the extreme Universe with gamma rays in the MeV - GeV range. Exp. Astron. 44, 25–82. doi:10.1007/s10686-017-9533-6
de Jaeger, T., Shappee, B. J., Kochanek, C. S., Hinkle, J. T., Garrappa, S., Liodakis, I., et al. (2023). Optical/γ-ray blazar flare correlations: understanding the high-energy emission process using ASAS-SN and Fermi light curves. Mon. Not. R. Astron. Soc. 519, 6349–6380. doi:10.1093/mnras/stad060
de Jager, O. C., Harding, A. K., Michelson, P. F., Nel, H. I., Nolan, P. L., Sreekumar, P., et al. (1996). Gamma-ray observations of the Crab nebula: a study of the synchro-compton spectrum. Astrophys. J. 457, 253. doi:10.1086/176726
Dermer, C. D. (1995). On the beaming statistics of gamma-ray sources. Astrophys. J. Lett. 446, L63. doi:10.1086/187931
Dermer, C. D., Schlickeiser, R., and Mastichiadis, A. (1992). High-energy gamma radiation from extragalactic radio sources. Astron. Astrophys. 256, L27–L30. doi:10.1086/187931
Desai, A., Helgason, K., Ajello, M., Paliya, V., Domínguez, A., Finke, J., et al. (2019). A GeV-TeV measurement of the extragalactic background light. Astrophys. J. Lett. 874, L7. doi:10.3847/2041-8213/ab0c10
Desai, A., Marchesi, S., Rajagopal, M., and Ajello, M. (2019). Identifying the 3fhl catalog. III. results of the CTIO-COSMOS optical spectroscopy campaign. APSS 241, 5. doi:10.3847/1538-4365/ab01fc
Devanand, P. U., Gupta, A. C., Jithesh, V., and Wiita, P. J. (2022). Study of X-ray intraday variability of HBL blazars based on observations obtained with XMM-Newton. Astrophys. J. 939, 80. doi:10.3847/1538-4357/ac9064
Devecchi, B., and Volonteri, M. (2009). Formation of the first nuclear clusters and massive black holes at high redshift. Astrophys. J. 694, 302–313. doi:10.1088/0004-637X/694/1/302
Diana, A., Caccianiga, A., Ighina, L., Belladitta, S., Moretti, A., and Della Ceca, R. (2022). The evolution of the heaviest supermassive black holes in jetted AGNs. Mon. Not. R. Astron. Soc. 511, 5436–5447. doi:10.1093/mnras/stac364
Di Gesu, L., Donnarumma, I., Tavecchio, F., Agudo, I., Barnounin, T., Cibrario, N., et al. (2022). The X-ray polarization view of mrk 421 in an average flux state as observed by the imaging X-ray Polarimetry explorer. Astrophys. J. Lett. 938, L7. doi:10.3847/2041-8213/ac913a
Diltz, C., Böttcher, M., and Fossati, G. (2015). Time dependent hadronic modeling of flat spectrum radio quasars. Astrophys. J. 802, 133. doi:10.1088/0004-637X/802/2/133
Disney, M. J., Peterson, B. A., and Rodgers, A. W. (1974). The redshift and composite nature of AP Librae (pks 1514-24). Astrophys. J. Lett. 194, L79. doi:10.1086/181673
Domínguez, A., Láinez, M., Paliya, V. S., Álvarez-Crespo, N., Ajello, M., Finke, J., et al. (2023). Constraints on redshifts of blazars from extragalactic background light attenuation using Fermi-LAT data. arXiv e-prints , arXiv:2307.10083doi. doi:10.48550/arXiv.2307.10083
Donato, D., Sambruna, R. M., and Gliozzi, M. (2005). Six years of BeppoSAX observations of blazars: a spectral catalog. Astron. Astrophys. 433, 1163–1169. doi:10.1051/0004-6361:20034555
Dondi, L., and Ghisellini, G. (1995). Gamma-ray-loud blazars and beaming. Mon. Not. R. Astron. Soc. 273, 583–595. doi:10.1093/mnras/273.3.583
Ehlert, S. R., Ferrazzoli, R., Marinucci, A., Marshall, H. L., Middei, R., Pacciani, L., et al. (2022). Limits on X-ray polarization at the core of Centaurus A as observed with the imaging X-ray Polarimetry explorer. Astrophys. J. 935, 116. doi:10.3847/1538-4357/ac8056
Eichler, D. (1979). High-energy neutrino astronomy: a probe of galactic nuclei? Astrophys. J. 232, 106–112. doi:10.1086/157269
Eraerds, T., Antonelli, V., Davis, C., Hall, D., Hetherington, O., Holland, A., et al. (2021). Enhanced simulations on the athena/wide field imager instrumental background. J. Astron. Telesc. Instrum. Syst. 7, 034001. doi:10.1117/1.JATIS.7.3.034001
Fabian, A. C., Celotti, A., Iwasawa, K., McMahon, R. G., Carilli, C. L., Brandt, W. N., et al. (2001). PMN J0525-3343: soft X-ray spectral flattening in a blazar at z=4.4. Mon. Not. R. Astron. Soc. 323, 373–379. doi:10.1046/j.1365-8711.2001.04181.x
Fan, X., Narayanan, V. K., Lupton, R. H., Strauss, M. A., Knapp, G. R., Becker, R. H., et al. (2001). A survey of [CLC] [ITAL]z[/ITAL] [/CLC] > 5.8 quasars in the sloan digital sky survey. I. Discovery of three new quasars and the spatial density of luminous quasars at [CLC] [ITAL]z[/ITAL] [/CLC] ∼ 6. Astron. J. 122, 2833–2849. doi:10.1086/324111
Fan, X.-L., and Wu, Q. (2018). The radio/gamma connection of blazars from high to low radio frequencies. Astrophys. J. 869, 133. doi:10.3847/1538-4357/aaeece
Fermi-LAT Collaboration Abdollahi, S., Ackermann, M., Ajello, M., Atwood, W.-B., Baldini, L., et al. (2018). A gamma-ray determination of the Universe’s star formation history. Science 362, 1031–1034. doi:10.1126/science.aat8123
Fossati, G., Buckley, J. H., Bond, I. H., Bradbury, S. M., Carter-Lewis, D. A., Chow, Y. C. K., et al. (2008). Multiwavelength observations of markarian 421 in 2001 March: an unprecedented view on the X-ray/TeV correlated variability. Astrophys. J. 677, 906–925. doi:10.1086/527311
Fossati, G., Maraschi, L., Celotti, A., Comastri, A., and Ghisellini, G. (1998). A unifying view of the spectral energy distributions of blazars. Mon. Not. R. Astron. Soc. 299, 433–448. doi:10.1046/j.1365-8711.1998.01828.x
Gao, S., Fedynitch, A., Winter, W., and Pohl, M. (2019). Modelling the coincident observation of a high-energy neutrino and a bright blazar flare. Nat. Astron. 3, 88–92. doi:10.1038/s41550-018-0610-1
Gao, S., Pohl, M., and Winter, W. (2017). On the direct correlation between gamma-rays and PeV neutrinos from blazars. Astrophys. J. 843, 109. doi:10.3847/1538-4357/aa7754
Garrappa, S., Buson, S., Franckowiak, A., Shappee, B. J., Beacom, J. F., Dong, S., et al. (2019). Investigation of two fermi-LAT gamma-ray blazars coincident with high-energy neutrinos detected by IceCube. Astrophys. J. 880, 103. doi:10.3847/1538-4357/ab2ada
Gaur, H., Gupta, A. C., Bachev, R., Strigachev, A., Semkov, E., Wiita, P. J., et al. (2019). Optical variability of TeV blazars on long time-scales. Mon. Not. R. Astron. Soc. 484, 5633–5644. doi:10.1093/mnras/stz322
Gaur, H., Mohan, P., and Pandey, A. (2021). Breaks in the X-ray spectra of high-redshift blazars and the intervening medium. Astrophys. J. 914, 46. doi:10.3847/1538-4357/abfa9d
Ghisellini, G. (1999a). Extreme blazars. Astropart. Phys. 11, 11–18. doi:10.1016/S0927-6505(99)00018-3
Ghisellini, G. (1999b). Mev synchrotron BL Lacs. Astrophys. Lett. Commun. 39, 17. doi:10.48550/arXiv.astro-ph/9812419
Ghisellini, G., Celotti, A., Fossati, G., Maraschi, L., and Comastri, A. (1998). A theoretical unifying scheme for gamma-ray bright blazars. Mon. Not. R. Astron. Soc. 301, 451–468. doi:10.1046/j.1365-8711.1998.02032.x
Ghisellini, G., Costamante, L., Tagliaferri, G., Maraschi, L., Celotti, A., Fossati, G., et al. (1999). The blazar PKS 0528+134: new results from BeppoSAX observations. Astron. Astrophys. 348, 63–70. doi:10.48550/arXiv.astro-ph/9906165
Ghisellini, G., and Maraschi, L. (1989). Bulk acceleration in relativistic jets and the spectral properties of blazars. Astrophys. J. 340, 181–189. doi:10.1086/167383
Ghisellini, G., Perri, M., Costamante, L., Tagliaferri, G., Sbarrato, T., Campitiello, S., et al. (2019). A NuSTAR view of powerful γ-ray loud blazars. Astron. Astrophys. 627, A72. doi:10.1051/0004-6361/201935750
Ghisellini, G., Righi, C., Costamante, L., and Tavecchio, F. (2017). The Fermi blazar sequence. Mon. Not. R. Astron. Soc. 469, 255–266. doi:10.1093/mnras/stx806
Ghisellini, G., and Tavecchio, F. (2008). Rapid variability in TeV blazars: the case of PKS2155-304. Mon. Not. R. Astron. Soc. 386, L28–L32. doi:10.1111/j.1745-3933.2008.00454.x
Ghisellini, G., and Tavecchio, F. (2009). Canonical high-power blazars. Mon. Not. R. Astron. Soc. 397, 985–1002. doi:10.1111/j.1365-2966.2009.15007.x
Ghisellini, G., Tavecchio, F., Foschini, L., Sbarrato, T., Ghirlanda, G., and Maraschi, L. (2012). Blue Fermi flat spectrum radio quasars. Mon. Not. R. Astron. Soc. 425, 1371–1379. doi:10.1111/j.1365-2966.2012.21554.x
Ghisellini, G., Tavecchio, F., Maraschi, L., Celotti, A., and Sbarrato, T. (2014). The power of relativistic jets is larger than the luminosity of their accretion disks. Nature 515, 376–378. doi:10.1038/nature13856
Giannios, D. (2013). Reconnection-driven plasmoids in blazars: fast flares on a slow envelope. Mon. Not. R. Astron. Soc. 431, 355–363. doi:10.1093/mnras/stt167
Gilli, R. (2013). The cosmic X-ray background: abundance and evolution of hidden black holes. MEMSAI 84, 647. . doi:10.48550/arXiv.1304.3665
Giommi, P., Brandt, C. H., Barres de Almeida, U., Pollock, A. M. T., Arneodo, F., Chang, Y. L., et al. (2019). Open Universe for Blazars: a new generation of astronomical products based on 14 years of Swift-XRT data. Astron. Astrophys. 631, A116. doi:10.1051/0004-6361/201935646
Giommi, P., Padovani, P., and Polenta, G. (2013). A simplified view of blazars: the γ-ray case. Mon. Not. R. Astron. Soc. 431, 1914–1922. doi:10.1093/mnras/stt305
Giommi, P., Padovani, P., Polenta, G., Turriziani, S., D’Elia, V., and Piranomonte, S. (2012). A simplified view of blazars: clearing the fog around long-standing selection effects. Mon. Not. R. Astron. Soc. 420, 2899–2911. doi:10.1111/j.1365-2966.2011.20044.x
Giommi, P., Perri, M., Capalbi, M., D’Elia, V., Barres de Almeida, U., Brandt, C. H., et al. (2021). X-ray spectra, light curves and SEDs of blazars frequently observed by Swift. Mon. Not. R. Astron. Soc. 507, 5690–5702. doi:10.1093/mnras/stab2425
Giroletti, M., Massaro, F., D’Abrusco, R., Lico, R., Burlon, D., Hurley-Walker, N., et al. (2016). High-energy sources at low radio frequency: the Murchison Widefield Array view of Fermi blazars. Astron. Astrophys. 588, A141. doi:10.1051/0004-6361/201527817
Gopal-Krishna, , Goyal, A., Joshi, S., Karthick, C., Sagar, R., Wiita, P. J., et al. (2011). Rapid optical variability of TeV blazars. Mon. Not. R. Astron. Soc. 416, 101–117. doi:10.1111/j.1365-2966.2011.19014.x
Grant, D., Ackermann, M., Karle, A., and Kowalski, M. (2019). Neutrino astronomy with the next generation IceCube neutrino observatory. Bull. Am. Astron. Soc. 51, 288. doi:10.48550/arXiv.1911.02561
Haardt, F., Grandi, P., Celotti, A., Treves, A., Ghisellini, G., Maraschi, L., et al. (1999). BeppoSAX observations of 3C 273. Nucl. Phys. B Proc. Suppl. 69, 449–452. doi:10.1016/S0920-5632(98)00260-6
Hardcastle, M. J. (2006). Testing the beamed inverse-Compton model for jet X-ray emission: velocity structure and deceleration. Mon. Not. R. Astron. Soc. 366, 1465–1474. doi:10.1111/j.1365-2966.2005.09923.x
Harris, D. E., and Grindlay, J. E. (1979). The prospects for X-ray detection of inverse-Compton emission from radio source electrons and photons of the microwave background. Mon. Not. R. Astron. Soc. 188, 25–37. doi:10.1093/mnras/188.1.25
Harris, D. E., and Krawczynski, H. (2002). X-ray emission processes in radio jets. Astrophys. J. 565, 244–255. doi:10.1086/324544
Harris, D. E., and Krawczynski, H. (2006). X-ray emission from extragalactic jets. ARAA 44, 463–506. doi:10.1146/annurev.astro.44.051905.092446
Harrison, F. A., Craig, W. W., Christensen, F. E., Hailey, C. J., Zhang, W. W., Boggs, S. E., et al. (2013). The nuclear spectroscopic telescope Array (NuSTAR) high-energy X-ray mission. Astrophys. J. 770, 103. doi:10.1088/0004-637X/770/2/103
Hess, V. (1912). On the observations of the penetrating radiation during seven balloon flights, 15. arXiv:1808.02927.
H. E. S. S., Collaboration, Abdalla, H., Adam, R., Aharonian, F., Ait Benkhali, F., Angüner, E. O., et al. (2019). Constraints on the emission region of 3C 279 during strong flares in 2014 and 2015 through VHE γ-ray observations with H.E.S.S. Astron. Astrophys. 627, A159. doi:10.1051/0004-6361/201935704
H. E. S. S., Collaboration, Abdalla, H., Adam, R., Aharonian, F., Ait Benkhali, F., Angüner, E. O., et al. (2020). Resolving acceleration to very high energies along the jet of Centaurus A. Nat 582, 356–359. doi:10.1038/s41586-020-2354-1
H. E. S. S., Collaboration, Abramowski, A., Acero, F., Aharonian, F., Akhperjanian, A. G., Anton, G., et al. (2013). Measurement of the extragalactic background light imprint on the spectra of the brightest blazars observed with H.E.S.S. Astron. Astrophys. 550, A4. doi:10.1051/0004-6361/201220355
H. E. S. S., Collaboration, Abramowski, A., Aharonian, F., Ait Benkhali, F., Akhperjanian, A. G., Angüner, E., et al. (2015). The high-energyγ-ray emission of AP Librae. Astron. Astrophys. 573, A31. doi:10.1051/0004-6361/201321436
Hood, I. J. C., Simpson, A., McDaniel, A., Foster, A., Ade, P. A. R., Ajello, M., et al. (2023). Simultaneous millimeter-wave, gamma-ray, and optical monitoring of the blazar PKS 2326-502 during a flaring state. Astrophys. J. Lett. 945, L23. doi:10.3847/2041-8213/acbf45
Hooper, D., Linden, T., and Vieregg, A. (2019). Active galactic nuclei and the origin of IceCube’s diffuse neutrino flux. J. Cosmol. Astropart. Phys. 2019, 012. doi:10.1088/1475-7516/2019/02/012
Hota, J., Shah, Z., Khatoon, R., Misra, R., Pradhan, A. C., and Gogoi, R. (2021). Understanding the X-ray spectral curvature of Mkn 421 using broad-band AstroSat observations. Mon. Not. R. Astron. Soc. 508, 5921–5934. doi:10.1093/mnras/stab2903
Hovatta, T., Valtaoja, E., Tornikoski, M., and Lähteenmäki, A. (2009). Doppler factors, Lorentz factors and viewing angles for quasars, BL Lacertae objects and radio galaxies. Astron. Astrophys. 494, 527–537. doi:10.1051/0004-6361:200811150
IceCube CollaborationFermi-LAT CollaborationINTEGRAL TeamSwift/NuSTAR Team Ackermann, M., Adams, J., et al. (2018b). Multimessenger observations of a flaring blazar coincident with high-energy neutrino IceCube-170922A. Science 361, eaat1378. doi:10.1126/science.aat1378
IceCube Collaboration Aartsen, M. G., Ackermann, M., Adams, J., Aguilar, J. A., Ahlers, M., et al. (2018a). Neutrino emission from the direction of the blazar TXS 0506+056 prior to the IceCube-170922A alert. Science 361, 147–151. doi:10.1126/science.aat2890
IceCube Collaboration Abbasi, R., Ackermann, M., Adams, J., Aguilar, J. A., Ahlers, M., et al. (2022). Evidence for neutrino emission from the nearby active galaxy NGC 1068. Science 378, 538–543. doi:10.1126/science.abg3395
Ighina, L., Caccianiga, A., Moretti, A., Belladitta, S., Della Ceca, R., Ballo, L., et al. (2019). X-ray properties of z > 4 blazars. Mon. Not. R. Astron. Soc. 489, 2732–2745. doi:10.1093/mnras/stz2340
Ighina, L., Caccianiga, A., Moretti, A., Belladitta, S., Della Ceca, R., and Diana, A. (2021). The impact of the CMB on the evolution of high-z blazars. Mon. Not. R. Astron. Soc. 505, 4120–4128. doi:10.1093/mnras/stab1612
Ighina, L., Moretti, A., Tavecchio, F., Caccianiga, A., Belladitta, S., Dallacasa, D., et al. (2022). Direct observation of an extended X-ray jet at z = 6.1. Astron. Astrophys. 659, A93. doi:10.1051/0004-6361/202142676
Jansen, F., Lumb, D., Altieri, B., Clavel, J., Ehle, M., Erd, C., et al. (2001). XMM-Newton observatory. I. The spacecraft and operations. Astron. Astrophys. 365, L1–L6. doi:10.1051/0004-6361:20000036
Jester, S., Harris, D. E., Marshall, H. L., and Meisenheimer, K. (2006). New Chandra observations of the jet in 3C 273. I. Softer X-ray than radio spectra and the X-ray emission mechanism. Astrophys. J. 648, 900–909. doi:10.1086/505962
Johnson, J. L., and Upton Sanderbeck, P. R. (2022). A simple condition for sustained super-eddington black hole growth. Astrophys. J. 934, 58. doi:10.3847/1538-4357/ac7b81
Jolley, E. J. D., and Kuncic, Z. (2008). Jet-enhanced accretion growth of supermassive black holes. Mon. Not. R. Astron. Soc. 386, 989–994. doi:10.1111/j.1365-2966.2008.13082.x
Jorstad, S. G., Marscher, A. P., Stevens, J. A., Smith, P. S., Forster, J. R., Gear, W. K., et al. (2007). Multiwaveband polarimetric observations of 15 active galactic nuclei at high frequencies: correlated polarization behavior. Astron. J. 134, 799–824. doi:10.1086/519996
Kadler, M., Krauß, F., Mannheim, K., Ojha, R., Müller, C., Schulz, R., et al. (2016). Coincidence of a high-fluence blazar outburst with a PeV-energy neutrino event. Nat. Phys. 12, 807–814. doi:10.1038/nphys3715
Kadowaki, L. H. S., de Gouveia Dal Pino, E. M., and Singh, C. B. (2015). The role of fast magnetic reconnection on the radio and gamma-ray emission from the nuclear regions of microquasars and low luminosity AGNs. Astrophys. J. 802, 113. doi:10.1088/0004-637X/802/2/113
Kapanadze, B., Dorner, D., Vercellone, S., Romano, P., Aller, H., Aller, M., et al. (2016). X-ray flaring activity of mrk 421 in the first half of 2013. Astrophys. J. 831, 102. doi:10.3847/0004-637X/831/1/102
Kataoka, J., Mattox, J. R., Quinn, J., Kubo, H., Makino, F., Takahashi, T., et al. (1999). High-energy emission from the TEV blazar markarian 501 during multiwavelength observations in 1996. Astrophys. J. 514, 138–147. doi:10.1086/306918
Kaufmann, S., Wagner, S. J., and Tibolla, O. (2013). Discovery of an extended X-ray jet in AP Librae. Astrophys. J. 776, 68. doi:10.1088/0004-637X/776/2/68
Kaur, A., Ajello, M., Marchesi, S., and Omodei, N. (2019). Identifying the 3FHL catalog. I. Archival Swift observations and source classification. Astrophys. J. 871, 94. doi:10.3847/1538-4357/aaf649
Keenan, M., Meyer, E. T., Georganopoulos, M., Reddy, K., and French, O. J. (2021). The relativistic jet dichotomy and the end of the blazar sequence. Mon. Not. R. Astron. Soc. 505, 4726–4745. doi:10.1093/mnras/stab1182
Keivani, A., Murase, K., Petropoulou, M., Fox, D. B., Cenko, S. B., Chaty, S., et al. (2018). A multimessenger picture of the flaring blazar TXS 0506+056: implications for high-energy neutrino emission and cosmic-ray acceleration. Astrophys. J. 864, 84. doi:10.3847/1538-4357/aad59a
Kirk, J. G., and Mastichiadis, A. (1999). Variability patterns of synchrotron and inverse Compton emission in blazars. Astropart. Phys. 11, 45–48. doi:10.1016/S0927-6505(99)00023-7
Kirk, J. G., Rieger, F. M., and Mastichiadis, A. (1998). Particle acceleration and synchrotron emission in blazar jets. Astron. Astrophys. 333, 452–458. doi:10.48550/arXiv.astro-ph/9801265
Komossa, S., Grupe, D., Gallo, L. C., Gonzalez, A., Yao, S., Hollett, A. R., et al. (2021). MOMO. IV. The complete Swift X-ray and UV/optical light curve and characteristic variability of the blazar OJ 287 during the last two decades. Astrophys. J. 923, 51. doi:10.3847/1538-4357/ac1442
Koyama, K., Tsunemi, H., Dotani, T., Bautz, M. W., Hayashida, K., Tsuru, T. G., et al. (2007). X-ray imaging spectrometer (XIS) on board Suzaku. Publ. Astron. Soc. Jpn. Nihon. Tenmon. Gakkai. 59, 23–33. doi:10.1093/pasj/59.sp1.S23
Krauß, F., Calamari, E., Keivani, A., Coleiro, A., Evans, P. A., Fox, D. B., et al. (2020). Multimessenger observations of counterparts to IceCube-190331A. Mon. Not. R. Astron. Soc. 497, 2553–2561. doi:10.1093/mnras/staa2148
Krauß, F., Deoskar, K., Baxter, C., Kadler, M., Kreter, M., Langejahn, M., et al. (2018). Fermi/LAT counterparts of IceCube neutrinos above 100 TeV. Astron. Astrophys. 620, A174. doi:10.1051/0004-6361/201834183
Krauß, F., Kadler, M., Mannheim, K., Schulz, R., Trüstedt, J., Wilms, J., et al. (2014). TANAMI blazars in the IceCube PeV-neutrino fields. Astron. Astrophys. 566, L7. doi:10.1051/0004-6361/201424219
Krawczynski, H., Hughes, S. B., Horan, D., Aharonian, F., Aller, M. F., Aller, H., et al. (2004). Multiwavelength observations of strong flares from the TeV blazar 1ES 1959+650. Astrophys. J. 601, 151–164. doi:10.1086/380393
Kreter, M., Gokus, A., Krauss, F., Kadler, M., Ojha, R., Buson, S., et al. (2020a). Search for high-redshift blazars with Fermi/LAT. Astrophys. J. 903, 128. doi:10.3847/1538-4357/abb8da
Kreter, M., Kadler, M., Krauß, F., Mannheim, K., Buson, S., Ojha, R., et al. (2020b). On the detection potential of blazar flares for current neutrino telescopes. Astrophys. J. 902, 133. doi:10.3847/1538-4357/abb5b1
Lehmer, B. D., Garofali, K., Binder, B. A., Fornasini, F., Vulic, N., Zezas, A., et al. (2023). The high energy X-ray probe: resolved X-ray populations in extragalactic environments. arXiv e-prints , arXiv:2311.04735. doi:10.48550/arXiv.2311.04735
Levinson, A., and Rieger, F. (2011). Variable TeV emission as a manifestation of jet formation in M87? Astrophys. J. 730, 123. doi:10.1088/0004-637X/730/2/123
Liao, N.-H., Li, S., and Fan, Y.-Z. (2018). Fermi-LAT detection of a transient γ-ray source in the direction of a distant blazar B3 1428+422 at z = 4.72. Astrophys. J. Lett. 865, L17. doi:10.3847/2041-8213/aae20d
Linfield, R. (1981). VLBI observations of jets in double radio galaxies. Astrophys. J. 244, 436–446. doi:10.1086/158720
Liodakis, I., Marscher, A. P., Agudo, I., Berdyugin, A. V., Bernardos, M. I., Bonnoli, G., et al. (2022). Polarized blazar X-rays imply particle acceleration in shocks. Nature 611, 677–681. doi:10.1038/s41586-022-05338-0
Lodato, G., and Natarajan, P. (2006). Supermassive black hole formation during the assembly of pre-galactic discs. Mon. Not. R. Astron. Soc. 371, 1813–1823. doi:10.1111/j.1365-2966.2006.10801.x
Lucchini, M., Markoff, S., Crumley, P., Krauß, F., and Connors, R. M. T. (2019). Breaking degeneracy in jet dynamics: multi-epoch joint modelling of the BL Lac PKS 2155-304. Mon. Not. R. Astron. Soc. 482, 4798–4812. doi:10.1093/mnras/sty2929
Madau, P., Ghisellini, G., and Persic, M. (1987). Beaming in blazars. Mon. Not. R. Astron. Soc. 224, 257–264. doi:10.1093/mnras/224.1.257
Madau, P., and Rees, M. J. (2001). Massive black holes as population III remnants. Astrophys. J. Lett. 551, L27–L30. doi:10.1086/319848
Madejski, G. G., and Sikora, M. (2016). Gamma-ray observations of active galactic nuclei. ARAA 54, 725–760. doi:10.1146/annurev-astro-081913-040044
Madejski, G. M., Nalewajko, K., Madsen, K. K., Chiang, J., Baloković, M., Paneque, D., et al. (2016). First NuSTAR observations of the BL lac-type blazar PKS 2155-304: constraints on the jet content and distribution of radiating particles. Astrophys. J. 831, 142. doi:10.3847/0004-637X/831/2/142
Madsen, K. K., García, J. A., Stern, D., Armini, R., Basso, S., Coutinho, D., et al. (2023). The high energy X-ray probe (HEX-P): instrument and mission profile. arXiv e-prints , arXiv:2312.04678. doi:10.48550/arXiv.2312.04678
MAGIC Collaboration Acciari, V. A., Ansoldi, S., Antonelli, L. A., Babić, A., Banerjee, B., et al. (2020). Study of the variable broadband emission of Markarian 501 during the most extreme Swift X-ray activity. Astron. Astrophys. 637, A86. doi:10.1051/0004-6361/201834603
MAGIC Collaboration Acciari, V. A., Ansoldi, S., Antonelli, L. A., Arbet Engels, A., Artero, M., et al. (2021). Investigation of the correlation patterns and the Compton dominance variability of Mrk 421 in 2017. Astron. Astrophys. 655, A89. doi:10.1051/0004-6361/202141004
Mannheim, K. (1993). The proton blazar. Astron. Astrophys. 269, 67–76. doi:10.48550/arXiv.astro-ph/9302006
Mannheim, K., Stanev, T., and Biermann, P. L. (1992). Neutrinos from flat-spectrum radio quasars. Astron. Astrophys. 260, L1–L3. doi:10.48550/arXiv.astro-ph/9302006
Mao, L., and Zhang, X. (2016). Long-term optical variability properties of blazars in the SDSS Stripe 82. APSS 361, 345. doi:10.1007/s10509-016-2934-6
Maraschi, L. (1999). A first look at blazars with BeppoSAX. Nucl. Phys. B Proc. Suppl. 69, 389–396. doi:10.1016/S0920-5632(98)00247-3
Maraschi, L., Fossati, G., Tavecchio, F., Chiappetti, L., Celotti, A., Ghisellini, G., et al. (1999). Simultaneous X-Ray and T[CLC]e[/CLC]V Observations of a Rapid Flare from Markarian 421. Astrophys. J. Lett. 526, L81–L84. doi:10.1086/312370
Marchesi, S., Kaur, A., and Ajello, M. (2018). Identifying the 3FHL catalog. II. Results of the KOSMOS optical spectroscopy campaign. Astron. J. 156, 212. doi:10.3847/1538-3881/aae201
Marcotulli, L., Ajello, M., Urry, C. M., Paliya, V. S., Koss, M., Oh, K., et al. (2022). BASS. XXXIII. Swift-BAT blazars and their jets through cosmic time. Astrophys. J. 940, 77. doi:10.3847/1538-4357/ac937f
Marcotulli, L., Paliya, V., Ajello, M., Kaur, A., Marchesi, S., Rajagopal, M., et al. (2020). NuSTAR perspective on high-redshift MeV blazars. Astrophys. J. 889, 164. doi:10.3847/1538-4357/ab65f5
Marcotulli, L., Paliya, V. S., Ajello, M., Kaur, A., Hartmann, D. H., Gasparrini, D., et al. (2017). High-redshift blazars through NuSTAR eyes. Astrophys. J. 839, 96. doi:10.3847/1538-4357/aa6a17
Marscher, A. P. (2014). Turbulent, extreme multi-zone model for simulating flux and polarization variability in blazars. Astrophys. J. 780, 87. doi:10.1088/0004-637X/780/1/87
Marscher, A. P., and Gear, W. K. (1985). Models for high-frequency radio outbursts in extragalactic sources, with application to the early 1983 millimeter-to-infrared flare of 3C 273. Astrophys. J. 298, 114–127. doi:10.1086/163592
Marshall, H. L., Gelbord, J. M., Worrall, D. M., Birkinshaw, M., Schwartz, D. A., Jauncey, D. L., et al. (2018). An X-ray imaging survey of quasar jets: the complete survey. Astrophys. J. 856, 66. doi:10.3847/1538-4357/aaaf66
Marshall, H. L., Harris, D. E., Grimes, J. P., Drake, J. J., Fruscione, A., Juda, M., et al. (2001). Structure of the X-ray emission from the jet of 3C 273. Astrophys. J. Lett. 549, L167–L171. doi:10.1086/319161
Massaro, E., Maselli, A., Leto, C., Marchegiani, P., Perri, M., Giommi, P., et al. (2015). The 5th edition of the Roma-BZCAT. A short presentation. APSS 357, 75. doi:10.1007/s10509-015-2254-2
Massaro, E., Perri, M., Giommi, P., and Nesci, R. (2004). Log-parabolic spectra and particle acceleration in the BL Lac object Mkn 421: spectral analysis of the complete BeppoSAX wide band X-ray data set. Astron. Astrophys. 413, 489–503. doi:10.1051/0004-6361:20031558
Massaro, E., Tramacere, A., Perri, M., Giommi, P., and Tosti, G. (2006). Log-parabolic spectra and particle acceleration in blazars: III. SSC emission in the TeV band from Mkn 501. Astron. Astrophys. 448, 861–871. doi:10.1051/0004-6361:20053644
Massaro, F., Álvarez Crespo, N., D’Abrusco, R., Landoni, M., Masetti, N., Ricci, F., et al. (2016). The gamma-ray blazar quest: new optical spectra, state of art and future perspectives. APSS 361, 337. doi:10.1007/s10509-016-2926-6
Massaro, F., Giroletti, M., Paggi, A., D’Abrusco, R., Tosti, G., and Funk, S. (2013). Blazar spectral properties at 74 MHz. Astrophys. J. Suppl. Ser. 208, 15. doi:10.1088/0067-0049/208/2/15
Massaro, F., Masetti, N., D’Abrusco, R., Paggi, A., and Funk, S. (2014). Optical spectroscopic observations of blazars and γ-ray blazar candidates in the sloan digital sky survey data release nine. Astron. J. 148, 66. doi:10.1088/0004-6256/148/4/66
Massaro, F., Tramacere, A., Cavaliere, A., Perri, M., and Giommi, P. (2008). X-ray spectral evolution of TeV BL Lacertae objects: eleven years of observations with BeppoSAX, XMM-Newton and Swift satellites. Astron. Astrophys. 478, 395–401. doi:10.1051/0004-6361:20078639
Meidinger, N., Albrecht, S., Beitler, C., Bonholzer, M., Emberger, V., Frank, J., et al. (2020). “Development status of the wide field imager instrument for Athena,” in Space telescopes and instrumentation 2020: ultraviolet to gamma ray. Editors J.-W. A. den Herder, S. Nikzad, and K. Nakazawa (Society of Photo-Optical Instrumentation Engineers Conference Series), 11444. doi:10.1117/12.2560507
Meyer, E. T., Breiding, P., Georganopoulos, M., Oteo, I., Zwaan, M. A., Laing, R., et al. (2017). New ALMA and Fermi/LAT observations of the large-scale jet of PKS 0637-752 strengthen the case against the IC/CMB model. Astrophys. J. Lett. 835, L35. doi:10.3847/2041-8213/835/2/L35
Meyer, E. T., Petropoulou, M., Georganopoulos, M., Chiaberge, M., Breiding, P., and Sparks, W. B. (2018). Detection of an optical/UV jet/counterjet and multiple spectral components in M84. Astrophys. J. 860, 9. doi:10.3847/1538-4357/aabf39
Meyer, M., Scargle, J. D., and Blandford, R. D. (2019). Characterizing the gamma-ray variability of the brightest flat spectrum radio quasars observed with the Fermi LAT 877, 39. doi:10.3847/1538-4357/ab1651
Middei, R., Giommi, P., Perri, M., Turriziani, S., Sahakyan, N., Chang, Y. L., et al. (2022). The first hard X-ray spectral catalogue of Blazars observed by NuSTAR. Mon. Not. R. Astron. Soc. 514, 3179–3190. doi:10.1093/mnras/stac1185
Middei, R., Liodakis, I., Perri, M., Puccetti, S., Cavazzuti, E., Di Gesu, L., et al. (2023a). X-ray polarization observations of BL Lacertae. Astrophys. J. Lett. 942, L10. doi:10.3847/2041-8213/aca281
Middei, R., Perri, M., Puccetti, S., Liodakis, I., Di Gesu, L., Marscher, A. P., et al. (2023b). IXPE and multiwavelength observations of blazar PG 1553+113 reveal an orphan optical polarization swing. Astrophys. J. Lett. 953, L28. doi:10.3847/2041-8213/acec3e
Mohorian, M., Bhatta, G., Adhikari, T. P., Dhital, N., Pánis, R., Dinesh, A., et al. (2022). X-ray timing and spectral variability properties of blazars S5 0716 + 714, OJ 287, Mrk 501, and RBS 2070. Mon. Not. R. Astron. Soc. 510, 5280–5301. doi:10.1093/mnras/stab3738
Mortlock, D. J., Warren, S. J., Venemans, B. P., Patel, M., Hewett, P. C., McMahon, R. G., et al. (2011). A luminous quasar at a redshift of z = 7.085. Nature 474, 616–619. doi:10.1038/nature10159
Mücke, A., Protheroe, R. J., Engel, R., Rachen, J. P., and Stanev, T. (2003). BL Lac objects in the synchrotron proton blazar model. Astropart. Phys. 18, 593–613. doi:10.1016/S0927-6505(02)00185-8
Mundo, S. A., and Mushotzky, R. (2023). Long-term hard X-ray variability properties of Swift-BAT blazars. Mon. Not. R. Astron. Soc. 526, 4040–4050. doi:10.1093/mnras/stad2991
Murase, K., Inoue, Y., and Dermer, C. D. (2014). Diffuse neutrino intensity from the inner jets of active galactic nuclei: impacts of external photon fields and the blazar sequence. Phys. Rev. D. 90, 023007. doi:10.1103/PhysRevD.90.023007
Murase, K., Oikonomou, F., and Petropoulou, M. (2018). Blazar flares as an origin of high-energy cosmic neutrinos? Astrophys. J. 865, 124. doi:10.3847/1538-4357/aada00
Nandra, K., Barret, D., Barcons, X., Fabian, A., den Herder, J.-W., Piro, L., et al. (2013). The hot and energetic universe: a white paper presenting the science theme motivating the Athena+ mission. arXiv e-prints , arXiv:1306.2307. doi:10.48550/arXiv.1306.2307
National Academies of Sciences, Engineering, and Medicine (2021). Pathways to discovery in astronomy and astrophysics for the 2020s. Washington, DC: The National Academies Press. doi:10.17226/26141
Nemmen, R. S., Storchi-Bergmann, T., and Eracleous, M. (2014). Spectral models for low-luminosity active galactic nuclei in LINERs: the role of advection-dominated accretion and jets. Mon. Not. R. Astron. Soc. 438, 2804–2827. doi:10.1093/mnras/stt2388
Nieppola, E., Valtaoja, E., Tornikoski, M., Hovatta, T., and Kotiranta, M. (2008). Blazar sequence - an artefact of Doppler boosting. Astron. Astrophys. 488, 867–872. doi:10.1051/0004-6361:200809716
Noel, A. P., Gaur, H., Gupta, A. C., Wierzcholska, A., Ostrowski, M., Dhiman, V., et al. (2022). X-ray intraday variability of the TeV blazar markarian 421 with XMM-Newton. Astrophys. J. Suppl. Ser. 262, 4. doi:10.3847/1538-4365/ac7799
Oei, M. S. S. L., van Weeren, R. J., Hardcastle, M. J., Botteon, A., Shimwell, T. W., Dabhade, P., et al. (2022). The discovery of a radio galaxy of at least 5 Mpc. Astron. Astrophys. 660, A2. doi:10.1051/0004-6361/202142778
Oh, K., Koss, M., Markwardt, C. B., Schawinski, K., Baumgartner, W. H., Barthelmy, S. D., et al. (2018). The 105-month swift-BAT all-sky hard X-ray survey. Astrophys. J. Suppl. Ser. 235, 4. doi:10.3847/1538-4365/aaa7fd
Otero-Santos, J., Acosta-Pulido, J. A., Becerra González, J., Raiteri, C. M., Carnerero, M. I., Castro Segura, N., et al. (2023). Variability and evolution of the optical polarization of a sample of gamma-ray blazars. Mon. Not. R. Astron. Soc. 523, 4504–4519. doi:10.1093/mnras/stad1722
Pacini, D. (1912). La radiazione penetrante alla superficie ed in seno alle acque. Il Nuovo Cimento 3, 93–100. doi:10.1007/bf02957440
Padovani, P., Alexander, D. M., Assef, R. J., De Marco, B., Giommi, P., Hickox, R. C., et al. (2017). Active galactic nuclei: what’s in a name? AAPR 25, 2. doi:10.1007/s00159-017-0102-9
Padovani, P., Boccardi, B., Falomo, R., and Giommi, P. (2022a). PKS 1424+240: yet another masquerading BL Lac object as a possible IceCube neutrino source. Mon. Not. R. Astron. Soc. 511, 4697–4701. doi:10.1093/mnras/stac376
Padovani, P., Costamante, L., Ghisellini, G., Giommi, P., and Perlman, E. (2002). BeppoSAX observations of synchrotron X-ray emission from radio quasars. Astrophys. J. 581, 895–911. doi:10.1086/344406
Padovani, P., Giommi, P., Falomo, R., Oikonomou, F., Petropoulou, M., Glauch, T., et al. (2022b). The spectra of IceCube neutrino (SIN) candidate sources - II. Source characterization. Mon. Not. R. Astron. Soc. 510, 2671–2688. doi:10.1093/mnras/stab3630
Padovani, P., Oikonomou, F., Petropoulou, M., Giommi, P., and Resconi, E. (2019). TXS 0506+056, the first cosmic neutrino source, is not a BL Lac. Mon. Not. R. Astron. Soc. 484, L104–L108. doi:10.1093/mnrasl/slz011
Padovani, P., Petropoulou, M., Giommi, P., and Resconi, E. (2015). A simplified view of blazars: the neutrino background. Mon. Not. R. Astron. Soc. 452, 1877–1887. doi:10.1093/mnras/stv1467
Paggi, A., Milisavljevic, D., Masetti, N., Jiménez-Bailón, E., Chavushyan, V., D’Abrusco, R., et al. (2014). Optical spectroscopic observations of γ-ray blazar candidates. i. preliminary results. Astron. J. 147, 112. doi:10.1088/0004-6256/147/5/112
Paiano, S., Falomo, R., Franceschini, A., Treves, A., and Scarpa, R. (2017). Optical spectroscopic survey of a sample of unidentified Fermi objects. Astrophys. J. 851, 135. doi:10.3847/1538-4357/aa9af4
Paliya, V. S., Ajello, M., Cao, H. M., Giroletti, M., Kaur, A., Madejski, G., et al. (2020). Blazars at the cosmic dawn. Astrophys. J. 897, 177. doi:10.3847/1538-4357/ab9c1a
Paliya, V. S., Ajello, M., Ojha, R., Angioni, R., Cheung, C. C., Tanada, K., et al. (2019a). Detection of a gamma-ray flare from the high-redshift blazar DA 193. Astrophys. J. 871, 211. doi:10.3847/1538-4357/aafa10
Paliya, V. S., Koss, M., Trakhtenbrot, B., Ricci, C., Oh, K., Ajello, M., et al. (2019b). BAT AGN spectroscopic survey. XVI. General physical characteristics of BAT blazars. Astrophys. J. 881, 154. doi:10.3847/1538-4357/ab2f8b
Peirson, A. L., Liodakis, I., and Romani, R. W. (2022). Testing high-energy emission models for blazars with X-ray Polarimetry. Astrophys. J. 931, 59. doi:10.3847/1538-4357/ac6a54
Peirson, A. L., Negro, M., Liodakis, I., Middei, R., Kim, D. E., Marscher, A. P., et al. (2023). X-ray polarization of BL Lacertae in outburst. Astrophys. J. Lett. 948, L25. doi:10.3847/2041-8213/acd242
Peña-Herazo, H. A., Paggi, A., García-Pérez, A., Amaya-Almazán, R. A., Massaro, F., Ricci, F., et al. (2021). Optical spectroscopic observations of gamma-ray blazar candidates. XI. Optical observations from SOAR, blanco, NTT and OAN-SPM. The story so far. Astron. J. 162, 177. doi:10.3847/1538-3881/ac1da7
Petropoulou, M., Dimitrakoudis, S., Padovani, P., Mastichiadis, A., and Resconi, E. (2015). Photohadronic origin of γ-ray BL Lac emission: implications for IceCube neutrinos. Mon. Not. R. Astron. Soc. 448, 2412–2429. doi:10.1093/mnras/stv179
Petropoulou, M., and Mastichiadis, A. (2015). Bethe–Heitler emission in BL Lacs: filling the gap between X-rays and γ-rays. Mon. Not. R. Astron. Soc. 447, 36–48. doi:10.1093/mnras/stu2364
Petropoulou, M., Murase, K., Santander, M., Buson, S., Tohuvavohu, A., Kawamuro, T., et al. (2020). Multi-epoch modeling of TXS 0506+056 and implications for long-term high-energy neutrino emission. Astrophys. J. 891, 115. doi:10.3847/1538-4357/ab76d0
Petropoulou, M., Vasilopoulos, G., and Giannios, D. (2017). The TeV emission of Ap Librae: a hadronic interpretation and prospects for CTA. Mon. Not. R. Astron. Soc. 464, 2213–2222. doi:10.1093/mnras/stw2453
Pian, E., Vacanti, G., Tagliaferri, G., Ghisellini, G., Maraschi, L., Treves, A., et al. (1998). [ITAL]BeppoSAX[/ITAL] observations of unprecedented synchrotron activity in the BL Lacertae object markarian 501. Astrophys. J. Lett. 492, L17–L20. doi:10.1086/311083
Plavin, A., Kovalev, Y. Y., Kovalev, Y. A., and Troitsky, S. (2020). Observational evidence for the origin of high-energy neutrinos in parsec-scale nuclei of radio-bright active galaxies. Astrophys. J. 894, 101. doi:10.3847/1538-4357/ab86bd
Plavin, A. V., Kovalev, Y. Y., Kovalev, Y. A., and Troitsky, S. V. (2022). Growing evidence for high-energy neutrinos originating in radio blazars. arXiv e-prints. arXiv:2211.09631doi. doi:10.48550/arXiv.2211.09631
Poitevineau, R., Castignani, G., and Combes, F. (2023). Black hole and galaxy co-evolution in radio-loud active galactic nuclei at z ∼ 0.3–4. Astron. Astrophys. 672, A164. doi:10.1051/0004-6361/202244560
Potter, W. J., and Cotter, G. (2015). New constraints on the structure and dynamics of black hole jets. Mon. Not. R. Astron. Soc. 453, 4071–4089. doi:10.1093/mnras/stv1657
Protheroe, R. J., and Kazanas, D. (1983). On the origin of relativistic particles and gamma-rays in quasars. Astrophys. J. 265, 620–624. doi:10.1086/160707
Pushkarev, A. B., Kovalev, Y. Y., Lister, M. L., and Savolainen, T. (2017). MOJAVE - XIV. Shapes and opening angles of AGN jets. Mon. Not. R. Astron. Soc. 468, 4992–5003. doi:10.1093/mnras/stx854
Rajagopal, M., Marchesi, S., Kaur, A., Domínguez, A., Silver, R., and Ajello, M. (2021). Identifying the 3FHL catalog. V. Results of the CTIO-COSMOS optical spectroscopy campaign 2019. Astrophys. J. Suppl. Ser. 254, 26. doi:10.3847/1538-4365/abf656
Rajagopal, M., Marcotulli, L., Ajello, M., Kaur, A., Paliya, V., and Hartmann, D. (2020). NuSTAR observations and multiwavelength modeling of the high-redshift BL Lacertae object 4FGL j2146.5-1344. Astrophys. J. 889, 102. doi:10.3847/1538-4357/ab6226
Rajagopal, M., Marcotulli, L., Labrie, K., Marchesi, S., and Ajello, M. (2023). Identifying the 3FHL catalog. VI. Results of the 2019 gemini optical spectroscopy. Astron. J. 165, 42. doi:10.3847/1538-3881/aca1be
Rajput, B., Stalin, C. S., and Rakshit, S. (2020). Long term γ-ray variability of blazars. Astron. Astrophys. 634, A80. doi:10.1051/0004-6361/201936769
Raouf, M., Shabala, S. S., Croton, D. J., Khosroshahi, H. G., and Bernyk, M. (2017). The many lives of active galactic nuclei-II: the formation and evolution of radio jets and their impact on galaxy evolution. Mon. Not. R. Astron. Soc. 471, 658–670. doi:10.1093/mnras/stx1598
Rau, A., Schady, P., Greiner, J., Salvato, M., Ajello, M., Bottacini, E., et al. (2012). BL Lacertae objects beyond redshift 1.3 - UV-to-NIR photometry and photometric redshift for Fermi/LAT blazars. Astron. Astrophys. 538, A26. doi:10.1051/0004-6361/201118159
Ravasio, M., Tagliaferri, G., Ghisellini, G., Tavecchio, F., Böttcher, M., and Sikora, M. (2003). <b>Beppo</b>SAX and multiwavelength observations of BL Lacertae in 2000. Astron. Astrophys. 408, 479–491. doi:10.1051/0004-6361:20031015
Rawlings, S., and Jarvis, M. J. (2004). Evidence that powerful radio jets have a profound influence on the evolution of galaxies. Mon. Not. R. Astron. Soc. 355, L9–L12. doi:10.1111/j.1365-2966.2004.08234.x
Reddy, K., Georganopoulos, M., Meyer, E. T., Keenan, M., and Kollmann, K. E. (2023). Offsets between X-ray and radio components in X-ray jets: the AtlasX. Astrophys. J. Suppl. Ser. 265, 8. doi:10.3847/1538-4365/aca321
Rees, M. J. (1978). The M87 jet: internal shocks in a plasma beam? Mon. Not. R. Astron. Soc. 184, 61P–65P. doi:10.1093/mnras/184.1.61P
Reimer, A., Böttcher, M., and Buson, S. (2019). Cascading constraints from neutrino-emitting blazars: the case of TXS 0506+056. Astrophys. J. 881, 46. doi:10.3847/1538-4357/ab2bff
Reimer, A., Costamante, L., Madejski, G., Reimer, O., and Dorner, D. (2008). A hard X-ray view of two distant VHE blazars: 1ES 1101-232 and 1ES 1553+113. Astrophys. J. 682, 775–783. doi:10.1086/589641
Ricci, F., Massaro, F., Landoni, M., D’Abrusco, R., Milisavljevic, D., Stern, D., et al. (2015). Optical spectroscopic observations of gamma-ray blazar candidates. IV. Results of the 2014 follow-up campaign. Astron. J. 149, 160. doi:10.1088/0004-6256/149/5/160
Roychowdhury, A., Meyer, E. T., Georganopoulos, M., Breiding, P., and Petropoulou, M. (2022). Circumnuclear dust in AP Librae and the source of its VHE emission. ApJ 924, 57. doi:10.3847/1538-4357/ac34f1
Safna, P. Z., Stalin, C. S., Rakshit, S., and Mathew, B. (2020). Long-term optical and infrared variability characteristics of Fermi blazars. Mon. Not. R. Astron. Soc. 498, 3578–3591. doi:10.1093/mnras/staa2622
Sandrinelli, A., Covino, S., and Treves, A. (2014). Long and short term variability of seven blazars in six near-infrared/optical bands. Astron. Astrophys. 562, A79. doi:10.1051/0004-6361/201321558
Sbarrato, T. (2021). Big and Young supermassive black holes in the early universe. Galaxies 9, 23. doi:10.3390/galaxies9020023
Sbarrato, T., Ghisellini, G., Nardini, M., Tagliaferri, G., Foschini, L., Ghirlanda, G., et al. (2012). SDSS J102623.61+254259.5: the second most distant blazar at z = 5.3. Mon. Not. R. Astron. Soc. 426, L91–L95. doi:10.1111/j.1745-3933.2012.01332.x
Sbarrato, T., Ghisellini, G., Tagliaferri, G., Perri, M., Madejski, G. M., Stern, D., et al. (2016). Extremes of the jet-accretion power relation of blazars, as explored by NuSTAR. Mon. Not. R. Astron. Soc. 462, 1542–1550. doi:10.1093/mnras/stw1730
Sbarrato, T., Tagliaferri, G., Ghisellini, G., Perri, M., Puccetti, S., Baloković, M., et al. (2013). NuSTAR detection of the blazar B2 1023+25 at redshift 5.3. Astrophys. J. 777, 147. doi:10.1088/0004-637X/777/2/147
Schmidt, M. (1968). Space distribution and luminosity functions of quasi-stellar radio sources. Astrophys. J. 151, 393. doi:10.1086/149446
Schneider, R., Ferrara, A., Natarajan, P., and Omukai, K. (2002). First stars, very massive black holes, and metals. Astrophys. J. 571, 30–39. doi:10.1086/339917
Schoenmakers, A. P., de Bruyn, A. G., Röttgering, H. J. A., van der Laan, H., and Kaiser, C. R. (2000). Radio galaxies with a ‘double-double morphology’ - I. Analysis of the radio properties and evidence for interrupted activity in active galactic nuclei. Mon. Not. R. Astron. Soc. 315, 371–380. doi:10.1046/j.1365-8711.2000.03430.x
Shabala, S. S., Kaviraj, S., and Silk, J. (2011). Active galactic nucleus feedback drives the colour evolution of local galaxies. Mon. Not. R. Astron. Soc. 413, 2815–2826. doi:10.1111/j.1365-2966.2011.18353.x
Shaw, M. S., Romani, R. W., Cotter, G., Healey, S. E., Michelson, P. F., Readhead, A. C. S., et al. (2013). Spectroscopy of the largest ever γ-ray-selected bl lac sample. Astrophys. J. 764, 135. doi:10.1088/0004-637X/764/2/135
Shen, Y., Richards, G. T., Strauss, M. A., Hall, P. B., Schneider, D. P., Snedden, S., et al. (2011). A catalog of quasar properties from sloan digital sky survey data release 7. Astrophys. J. Suppl. Ser. 194, 45. doi:10.1088/0067-0049/194/2/45
Sheng, Y., Rajagopal, M., Kaur, A., Ajello, M., Dominguez, A., Rau, A., et al. (2022). Revealing high-z Fermi-LAT BL Lacs using Swift and SARA data with photometric analysis. arXiv e-prints , arXiv:2209.09877. doi:10.48550/arXiv.2209.09877
Sikora, M., Begelman, M. C., and Rees, M. J. (1994). Comptonization of diffuse ambient radiation by a relativistic jet: the source of gamma rays from blazars? Astrophys. J. 421, 153. doi:10.1086/173633
Silk, J., and Rees, M. J. (1998). Quasars and galaxy formation. Astron. Astrophys. 331, L1–L4. doi:10.48550/arXiv.astro-ph/9801013
Sol, H., Zech, A., Boisson, C., Barres de Almeida, U., Biteau, J., Contreras, J. L., et al. (2013). Active galactic nuclei under the scrutiny of CTA. Astropart. Phys. 43, 215–240. doi:10.1016/j.astropartphys.2012.12.005
Stawarz, Ł., Sikora, M., and Ostrowski, M. (2003). High-energy gamma rays from FR I jets. Astrophys. J. 597, 186–201. doi:10.1086/378290
Stawarz, Ł., Tanaka, Y. T., Madejski, G., O’Sullivan, S. P., Cheung, C. C., Feain, I. J., et al. (2013). Giant lobes of Centaurus A radio galaxy observed with the Suzaku X-ray satellite. Astrophys. J. 766, 48. doi:10.1088/0004-637X/766/1/48
Stecker, F. W., Done, C., Salamon, M. H., and Sommers, P. (1991). High-energy neutrinos from active galactic nuclei. Phys. Rev. Lett. 66, 2697–2700. doi:10.1103/physrevlett.66.2697
Stein, W. A., Odell, S. L., and Strittmatter, P. A. (1976). The BL Lacertae objects. ARAA 14, 173–195. doi:10.1146/annurev.aa.14.090176.001133
Tagliaferri, G., Ghisellini, G., Giommi, P., Celotti, A., Chiaberge, M., Chiappetti, L., et al. (2001). The 0.1-200 keV spectrum of the blazar PKS 2005-489 during an active state. Astron. Astrophys. 368, 38–43. doi:10.1051/0004-6361:20010011
Tagliaferri, G., Ghisellini, G., Giommi, P., Chiappetti, L., Maraschi, L., Celotti, A., et al. (2000). The concave X-ray spectrum of the blazar ON 231: the signature of intermediate BL Lacertae objects. Astron. Astrophys. 354, 431–438. doi:10.48550/arXiv.astro-ph/9912055
Tagliaferri, G., Ghisellini, G., Perri, M., Hayashida, M., Baloković, M., Covino, S., et al. (2015). NuSTAR and multifrequency study of the two high-redshift blazars S5 0836+710 and PKS 2149-306. Astrophys. J. 807, 167. doi:10.1088/0004-637X/807/2/167
Tagliaferri, G., Ghisellini, G., and Ravasio, M. (2002). BeppoSAX ToO observations on BLAZARS. arXiv e-prints , astro-ph/0207017 10. 48550/arXiv.astro-ph/0207017.
Takahashi, T., Abe, K., Endo, M., Endo, Y., Ezoe, Y., Fukazawa, Y., et al. (2007). Hard X-ray detector (HXD) on board Suzaku, Publ. Astron. Soc. Jpn. Nihon. Tenmon. Gakkai. 59, 35–51. doi:10.1093/pasj/59.sp1.S35
Tavecchio, F., Maraschi, L., Ghisellini, G., Kataoka, J., Foschini, L., Sambruna, R. M., et al. (2007). Low-energy cutoffs and hard X-ray spectra in high-z radio-loud quasars: the Suzaku view of RBS 315. Astrophys. J. 665, 980–989. doi:10.1086/519156
Tavecchio, F., Maraschi, L., Pian, E., Chiappetti, L., Celotti, A., Fossati, G., et al. (2001). Theoretical implications from the spectral evolution of markarian 501 observed with BeppoSAX. Astrophys. J. 554, 725–733. doi:10.1086/321394
Tavecchio, F., Maraschi, L., Sambruna, R. M., and Urry, C. M. (2000). The X-ray jet of PKS 0637-752: inverse compton radiation from the cosmic microwave background? Astrophys. J. Lett. 544, L23–L26. doi:10.1086/317292
Tchekhovskoy, A., Narayan, R., and McKinney, J. C. (2011). Efficient generation of jets from magnetically arrested accretion on a rapidly spinning black hole. Mon. Not. R. Astron. Soc. 418, L79–L83. doi:10.1111/j.1745-3933.2011.01147.x
Tomsick, J., Zoglauer, A., Sleator, C., Lazar, H., Beechert, J., Boggs, S., et al. (2019). The compton spectrometer and imager. Bull. Am. Astron. Soc. 51, 98. doi:10.48550/arXiv.1908.04334
Tramacere, A., Giommi, P., Perri, M., Verrecchia, F., and Tosti, G. (2009). Swift observations of the very intense flaring activity of Mrk 421 during 2006. I. Phenomenological picture of electron acceleration and predictions for MeV/GeV emission. Astron. Astrophys. 501, 879–898. doi:10.1051/0004-6361/200810865
Uchiyama, Y., Urry, C. M., Cheung, C. C., Jester, S., Van Duyne, J., Coppi, P., et al. (2006). Shedding new light on the 3C 273 jet with the spitzer space telescope. Astrophys. J. 648, 910–921. doi:10.1086/505964
Ueda, Y., Akiyama, M., Hasinger, G., Miyaji, T., and Watson, M. G. (2014). Toward the standard population synthesis model of the X-ray background: evolution of X-ray luminosity and absorption functions of active galactic nuclei including compton-thick populations. Astrophys. J. 786, 104. doi:10.1088/0004-637X/786/2/104
Urry, C. M., and Padovani, P. (1995). Unified schemes for radio-loud active galactic nuclei. PASP 107, 803. doi:10.1086/133630
Urry, C. M., and Shafer, R. A. (1984). Luminosity enhancement in relativistic jets and altered luminosity functions for beamed objects. Astrophys. J. 280, 569–573. doi:10.1086/162027
Urry, C. M., Treves, A., Maraschi, L., Marshall, H. L., Kii, T., Madejski, G., et al. (1997). Multiwavelength monitoring of the BL Lacertae object PKS 2155-304 in 1994 may. III. Probing the inner jet through multiwavelength correlations. Astrophys. J. 486, 799–809. doi:10.1086/304536
Valverde, J., Horan, D., Bernard, D., Fegan, S., Fermi-LAT CollaborationAbeysekara, A. U., et al. (2020). A decade of multiwavelength observations of the TeV blazar 1ES 1215+303: extreme shift of the synchrotron peak frequency and long-term optical-gamma-ray flux increase. Astrophys. J. 891, 170. doi:10.3847/1538-4357/ab765d
Wang, J.-S., Reville, B., Liu, R.-Y., Rieger, F. M., and Aharonian, F. A. (2021). Particle acceleration in shearing flows: the case for large-scale jets. Mon. Not. R. Astron. Soc. 505, 1334–1341. doi:10.1093/mnras/stab1458
Wang, Y., Xue, Y., Zhu, S., and Fan, J. (2018). Systematic investigation of X-ray spectral variability of TeV blazars during flares in the RXTE era. Astrophys. J. 867, 68. doi:10.3847/1538-4357/aae307
Weaver, Z. R., Jorstad, S. G., Marscher, A. P., Morozova, D. A., Troitsky, I. S., Agudo, I., et al. (2022). Kinematics of parsec-scale jets of gamma-ray blazars at 43 GHz during 10 yr of the VLBA-BU-BLAZAR Program. Astrophys. J. Suppl. Ser. 260, 12. doi:10.3847/1538-4365/ac589c
Weisskopf, M. (2022). “The imaging X-ray Polarimetry explorer (IXPE) mission overview,” in AAS/High energy astrophysics division (AAS/High Energy Astrophysics Division), 54.
Williamson, K. E., Jorstad, S. G., Marscher, A. P., Larionov, V. M., Smith, P. S., Agudo, I., et al. (2014). Comprehensive monitoring of gamma-ray bright blazars. I. Statistical study of optical, X-ray, and gamma-ray spectral slopes. Astrophys. J. 789, 135. doi:10.1088/0004-637X/789/2/135
Wu, X.-B., Wang, F., Fan, X., Yi, W., Zuo, W., Bian, F., et al. (2015). An ultraluminous quasar with a twelve-billion-solar-mass black hole at redshift 6.30. Nature 518, 512–515. doi:10.1038/nature14241
Wulf, T. (1909). Beobachtungen über die Strahlung hoher Durchdringungsfähigkeit auf dem Eiffelturm. Phys. Z. 10, 997.
Yang, X., Yao, S., Yang, J., Ho, L. C., An, T., Wang, R., et al. (2020). Radio activity of supermassive black holes with extremely high accretion rates. Astrophys. J. 904, 200. doi:10.3847/1538-4357/abb775
Young, M., Elvis, M., and Risaliti, G. (2009). The fifth data release sloan digital sky survey/XMM-Newton quasar survey. Astrophys. J. Suppl. Ser. 183, 17–32. doi:10.1088/0067-0049/183/1/17
Zacharias, M. (2021). Studying the influence of external photon fields on blazar spectra using a one-zone hadro-leptonic time-dependent model. Physics 3, 1098–1111. doi:10.3390/physics3040069
Zacharias, M., Reimer, A., Boisson, C., and Zech, A. (2022). EXHALE-JET: an extended hadro-leptonic jet model for blazars - I. Code description and initial results. Mon. Not. R. Astron. Soc. 512, 3948–3971. doi:10.1093/mnras/stac754
Zacharias, M., and Wagner, S. J. (2016). The extended jet of AP Librae: origin of the very high-energy γ-ray emission? A&A 588, A110. doi:10.1051/0004-6361/201526698
Zech, A., and Lemoine, M. (2021). Electron-proton co-acceleration on relativistic shocks in extreme-TeV blazars. Astron. Astrophys. 654, A96. doi:10.1051/0004-6361/202141062
Zhang, B. T., Petropoulou, M., Murase, K., and Oikonomou, F. (2020a). A neutral beam model for high-energy neutrino emission from the blazar TXS 0506+056. Astrophys. J. 889, 118. doi:10.3847/1538-4357/ab659a
Zhang, H., Chen, X., Böttcher, M., Guo, F., and Li, H. (2015). Polarization swings reveal magnetic energy dissipation in blazars. Astrophys. J. 804, 58. doi:10.1088/0004-637X/804/1/58
Zhang, H., Diltz, C., and Böttcher, M. (2016). Radiation and polarization signatures of the 3D multizone time-dependent hadronic blazar model. Astrophys. J. 829, 69. doi:10.3847/0004-637X/829/2/69
Zhang, H., Fang, K., Li, H., Giannios, D., Böttcher, M., and Buson, S. (2019a). Probing the emission mechanism and magnetic field of neutrino blazars with multiwavelength polarization signatures. Astrophys. J. 876, 109. doi:10.3847/1538-4357/ab158d
Zhang, H., Li, X., Giannios, D., and Guo, F. (2021a). First-principles prediction of X-ray polarization from magnetic reconnection in high-frequency BL Lacertae objects. Astrophys. J. 912, 129. doi:10.3847/1538-4357/abf2be
Zhang, H., Li, X., Giannios, D., Guo, F., Liu, Y.-H., and Dong, L. (2020b). Radiation and polarization signatures from magnetic reconnection in relativistic jets. I. A systematic study. Astrophys. J. 901, 149. doi:10.3847/1538-4357/abb1b0
Zhang, H., Li, X., Giannios, D., Guo, F., Thiersen, H., Böttcher, M., et al. (2022a). Radiation and polarization signatures from magnetic reconnection in relativistic jets. II. Connection with γ-rays. Astrophys. J. 924, 90. doi:10.3847/1538-4357/ac3669
Zhang, H., Marscher, A. P., Guo, F., Giannios, D., Li, X., and Negro, M. (2023). First-principles-integrated study of blazar synchrotron radiation and polarization signatures from magnetic turbulence. Astrophys. J. 949, 71. doi:10.3847/1538-4357/acc657
Zhang, L., Vieira, J. D., Ajello, M., Malkan, M. A., Archipley, M. A., Capota, J., et al. (2022b). New identifications and multiwavelength properties of extragalactic Fermi gamma-ray sources in the SPT-SZ survey field. Astrophys. J. 939, 117. doi:10.3847/1538-4357/ac966f
Zhang, Y., Hou, Z., Shao, L., and Zhang, P. (2010). Discovery of a high confidence soft lag from an X-ray flare of Markarian 421. Sci. China Phys. Mech. Astron. 53, 224–227. doi:10.1007/s11433-010-0017-1
Zhang, Y. H., Bai, J. M., Zhang, S. N., Treves, A., Maraschi, L., and Celotti, A. (2006). Multiwavelength observations of the BL Lacertae object PKS 2155-304 with XMM-Newton. Astrophys. J. 651, 782–790. doi:10.1086/507601
Zhang, Z., Gupta, A. C., Gaur, H., Wiita, P. J., An, T., Gu, M., et al. (2019b). X-ray intraday variability of the TeV blazar mrk 421 with Suzaku. Astrophys. J. 884, 125. doi:10.3847/1538-4357/ab3f3a
Zhang, Z., Gupta, A. C., Gaur, H., Wiita, P. J., An, T., Lu, Y., et al. (2021b). X-ray intraday variability of the TeV blazar PKS 2155-304 with Suzaku during 2005-2014. Astrophys. J. 909, 103. doi:10.3847/1538-4357/abdd38
Zhu, S. F., Brandt, W. N., Wu, J., Garmire, G. P., and Miller, B. P. (2019). Investigating the X-ray enhancements of highly radio-loud quasars at z > 4. Mon. Not. R. Astron. Soc. 482, 2016–2038. doi:10.1093/mnras/sty2832
Zhu, S. F., Xue, Y. Q., Brandt, W. N., Cui, W., and Wang, Y. J. (2018). Extremely rapid X-ray flares of TeV blazars in the RXTE era. Astrophys. J. 853, 34. doi:10.3847/1538-4357/aa9f21
Keywords: blazar, supermassive black hole, jet, high energy astrophysical phenomena, X-ray mission, time domain astrophysics, multimessenger astronomy
Citation: Marcotulli L, Ajello M, Böttcher M, Coppi P, Costamante L, Di Gesu L, Errando M, García JA, Gokus A, Liodakis I, Madejski G, Madsen K, Moretti A, Middei R, McBride F, Petropoulou M, Rani B, Sbarrato T, Stern D, Vasilopoulos G, Zacharias M, Zhang H and the HEX-P Collaboration (2024) The high energy X-ray probe (HEX-P): the most powerful jets through the lens of a superb X-ray eye. Front. Astron. Space Sci. 11:1290057. doi: 10.3389/fspas.2024.1290057
Received: 06 September 2023; Accepted: 10 July 2024;
Published: 19 August 2024.
Edited by:
Aditi Agarwal, Centre for Cosmology and Science Popularization, IndiaReviewed by:
Reinaldo Roberto Rosa, National Institute of Space Research (INPE), BrazilCarlos Frajuca, Federal University of Rio Grande, Brazil
Fabrizio Tavecchio, National Institute of Astrophysics (INAF), Italy
Francesco Massaro, University of Turin, Italy
Copyright © 2024 Marcotulli, Ajello, Boettcher, Coppi, Costamante, Di Gesu, Errando, García, Gokus, Liodakis, Madejski, Madsen, Moretti, Middei, McBride, Petropoulou, Rani, Sbarrato, Stern, Vasilopoulos, Zacharias, Zhang and the HEX-P Collaboration. This is an open-access article distributed under the terms of the Creative Commons Attribution License (CC BY). The use, distribution or reproduction in other forums is permitted, provided the original author(s) and the copyright owner(s) are credited and that the original publication in this journal is cited, in accordance with accepted academic practice. No use, distribution or reproduction is permitted which does not comply with these terms.
*Correspondence: Lea Marcotulli, lea.marcotulli@yale.edu
†NHFP Einstein Fellow