- School of Information Engineering, China University of Geosciences, Beijing, China
The study of the lunar surface is a significant field in exploring the Moon. As a non-negligible geological process shaping the lunar surface, however, the mass movements on the Moon have not been deeply studied regarding all available datasets. In this paper, we report the results of the literature review and quantitative analysis of 965 articles retrieved from the Scopus, WoS, and Astrophysics Data System databases using keyword search terms between the years 1872 and 2022. The main findings show that the lunar mass movements are a lasting but recent prosperous research topic (since 2009). The top three influential journals in this area are Icarus, JGR, and P&SS. Robinson, Lucchitta, and Carr are the most three productive authors. California Institute of Technology, Arizona State University, and Johns Hopkins University are active institutions leading the lunar mass movements research, and the related institutions are mainly located in the United States, Russia, and China. Articles are primarily published in journals specializing in the fields of astronomy and physics. The index analysis of citation indicates the growth of the academic impact of articles about the lunar mass movements. This article summarizes the datasets, methods, and relevant parameters of lunar mass movements to facilitate future research, as well as discussing the limitations and trends in the field. In addition, four unsolved issues are proposed, including: 1) the lack of a comprehensive global database that records all types of landslides incidents; 2) the need for definite classification indicators to quantify the classification criteria for lunar mass movements; 3) the absence of a mathematical model to explain the triggering mechanism of mass movements on the Moon; and 4) the lack of quantitative indexes to evaluate the modification effect of mass movements on the lunar surface morphology. With the support of big data, the combination of artificial intelligence and traditional GIS methods is expected to become the main approach for addressing these issues such a bibliometric analysis can inspire future researchers by exploring the overall characteristics of the published literature on lunar mass movements.
1 Introduction
Mass wasting is a material downslope movement driven by gravity. It tends to erase surface topographic differences and form gentle reliefs (Xiao et al., 2013). The processes occur widely on Earth, mainly on steep slopes, both subaerially and underwater (e.g., Wolman and Gerson, 1978; Moore et al., 1994; Deplus et al., 2001; Corsini et al., 2009; Parker et al., 2011). Different types of mass wasting processes have also been observed on several planetary and minor bodies, such as Mars (McEwen, 1989; Shaller and Komatsu, 1994; Brunetti et al., 2014), the Moon (Pike, 1971; Lindsay, 1976; Xiao et al., 2013), Mercury (Xiao and Komatsu, 2013), Venus (Waltham et al., 2008) and some icy satellites and asteroids like Asteroid Eros, Itokawa, Lutetia, Vesta, Mathilde, Gaspra, Ida (Kereszturi, 2014; Murdoch et al., 2015) in the outer Solar System (Schenk and Bulmer, 1998).
In a terrestrial context, mass wasting represents the result of all the interactions between various geomorphological agents and processes, which act with different intensities on all types of slopes to modify the topography (Corominas et al., 2014; Pradhan and Siddique, 2019; Bhardwaj and Sam, 2022). In general, gravity is a major requirement for driving mass movements, but it is difficult to analyze the independent effects on the scales and dynamics of mass wasting by investigating terrestrial landslides alone, because of the presence of various other environmental elements on Earth, like a dense atmosphere, precipitation, and seismicity. Mass movements on other Solar System bodies share similarities. Thus, planetary studies with variable environmental conditions might help to reveal the effects of the atmosphere, subsurface fluids, precipitation, and seismicity on mass wasting, and could foster an understanding of triggering and propagation mechanisms (Sam and Bhardwaj, 2022).
As the sole natural satellite of the planet, the Moon is an important member of the inventory of planetary bodies that host mass movements. The mass movements of the Moon could bury ice and store it (Kereszturi, 2023), change the geomorphology of the lunar surface at different scales, impact the formation rate and process of lunar soil, affect the longevity of small craters, influence the dating of moderately young lunar surfaces, and record time clues about the lunar topography’s evolution. Therefore, it is an important step to study the degree and mechanism of modification of the lunar surface topography by lunar mass movements to fully clarify the evolution of the lunar surface topography, which helps to understand the ancient state of the Earth, the origin, and evolution of the Solar System. Mass movements have been distributed on various terrains on the Moon, including impact craters, volcanic domes, tectonic scarps, rilles, valleys, and wrinkle ridges (Xiao et al., 2013), but most of the landslides occur on the walls of impact craters; including Fall, Slide, Slump, Creep, and Flow, five kinds of gravity erosion processes (Bickle et al., 2021), such as the creep produced so called “girlands” identified recently in deGerlache crater (Kereszturi et al., 2022). The surface gravity of the Moon is small, just one-sixth that of those on the Earth, which means that the Moon can support proportionally higher topography than the Earth for the same material strength (Melosh, 2011). Therefore, the lunar mass movements are on a grander scale usually. On the other hand, the small gravity causes less dynamic fragmentation, so the typical debris of the mass movements on the Moon is many times bigger than similar-sized terrestrial mass movements (Xiao, 2021).
Multiresolution and multisensory remote sensing datasets from lunar exploration missions are constantly improving our understanding of the Moon. Since Pike (1971) published the first paper about mass movements on the Moon using images from the Apollo 10 Mission, a variety of mass-movement features have been reported by researchers (e.g., Lindsay, 1976; Bart, 2007; Bray et al., 2010; Perumal, 2012; Xiao et al., 2013; Otto et al., 2014; Li et al., 2018). These studies provide a qualitative description of the general morphological traits of the lunar mass movements, and based on the preliminary investigation, the causes and effects of the lunar mass movements are examined, and certain classification criteria are formulated. Several approaches have been used to study the lunar mass movements, as corroborated by publications. These approaches include image processing and remote sensing, which are employed to map the shape of mass movements. Mapping tools are utilized to generate inventory maps of mass movements, while methods for classifying lunar mass movements are also explored. Additionally, comparative planetology is employed to examine physical and mineralogical parameters that may impact mass movements. Terrain parameters are utilized to evaluate the effects of morphology modification. Furthermore, model simulation is utilized to replicate the movement process and investigate the factors that influence mass movements.
Xiao. (2021) exhaustively summarized the patterns, causes, and effects of lunar mass movements based on the geological background of the Moon. Despite this, there is a lack of systematic analysis of mass movement studies on the Moon. To evaluate the research status of lunar mass movements, this review examines available literature on mass movements on the Moon. We conducted a quantitative analysis of the publications, authorships, disciplines, and quotations of 965 articles to explore trends, leading scholars, research fields, and academic impact in the study of the lunar mass movements. And then we carried out discussions: 1) compiled a list of the data catalogue used for lunar mass movements studies; 2) summarized the research techniques for studying mass movements; 3) highlighted the parameters used in terrain analysis and cause-effect analysis; 4) discussed the research gaps and future research trends. The review allowed to identify data, methods, and opportunities in the research of geomorphic processes, and geological evolution of the Moon.
2 Search strategy
Our search was conducted in Scopus, Web of Science (WoS), and Astrophysics Data System (ADS), a digital library for astronomy and astrophysics researchers. The created database spans 151 years (from 1 January 1872 to 18 May 2022) and provides an overview of contemporary research trends. Ten key phrases were used to search articles about lunar mass movements: lunar landslide, lunar mass movements, lunar mass wasting, lunar granular flow, lunar debris avalanche, lunar rock slide, lunar debris slide, lunar rock avalanche, lunar rockfalls, lunar boulder falls. We separated the keywords with quote marks and joined them with the OR logic operator. We examined each database (Scopus, WoS, and ADS) to find duplicate articles, and articles identified in two datasets are deleted, leaving just one version of the duplicated article. The results are primarily from ADS, complemented with Scopus and Wos search results. Abstracts, patents, and news releases did not contribute to the content of this review, since they did not provide a detailed description of the lunar mass movements study.
3 Statistical analysis
In this section, we aim to employ bibliometric analysis to uncover the diverse research aspects within the domain of lunar mass movements research. Based on the literature database, we investigate the overall research pattern by analyzing the annual publication count, and highlight publications that are usually available to acquire articles related to the lunar mass movements through the distribution analysis of affiliated publications. The analysis of the author network and affiliated institutions can help researchers find the prominent scholars and reliable institutions in the field of lunar mass movements, and the research topics and latest research advances of their respective research groups. The objective of discipline analysis is to find the research trends of lunar mass movements across different disciplines, to identify the current hot research fields, and to comprehend the inter-disciplinary application. Lastly, the citation analysis unveils the trend of influence in lunar mass movements research, while co-citation analysis aids in identifying additional points of intersection for mass movements research.
3.1 Publications
There were 1,742 literature retrieved from the 10 keywords, of which 237 were in Scopus, 425 were in WoS, and 1,080 were in ADS. Using the deduplication rule, the final articles database contained 965 items (Figure 1) including 867 unduplicated articles (those found in only one database) and 98 duplicated articles (those found in several databases).
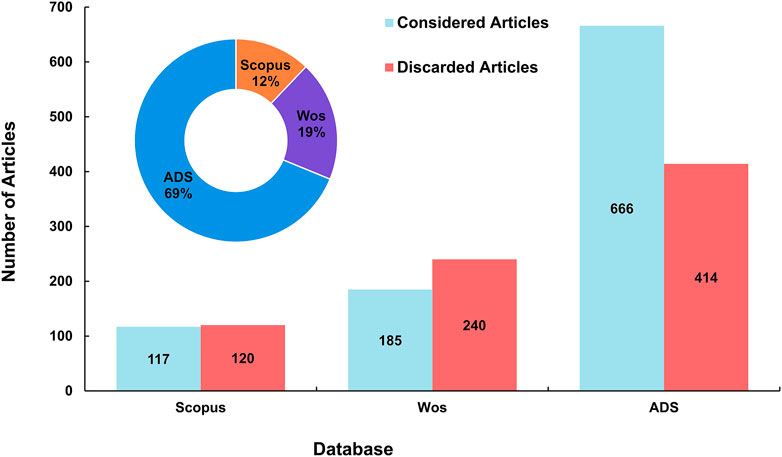
FIGURE 1. Related articles are distributed in three online databases including original search results and deduplicated results.
The number of published papers has grown exponentially since the first article about lunar mass movements appeared in 1872. (R2 = 0.7064) (Figure 2) Taking 1973 and 2009 as key growth nodes, it can be divided into three development periods, with an average number of articles of 0.44, 14, and 29. Taking 10 years as the interval, the total number of articles in each period is calculated, which highlights the boom years of lunar mass movements research (See Figure 2). Researchers have only begun to do a lot of research on the mass movements of the Moon in the last 60 years, and 98 percent of the publications have been published in that time. 53.4 percent of all papers were published in the last 20 years (2003–2022). The high production years (2012, 2016, 2017, 2018, 2019, and 2020) were concentrated over this period, with values that were higher than the production mean (21 articles) reported for the last 30 years (1993–2022).
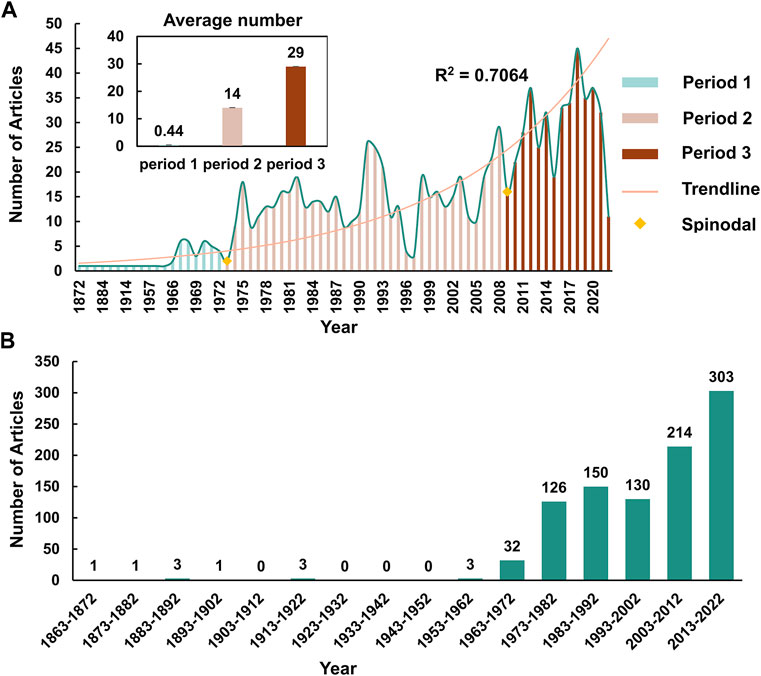
FIGURE 2. Characteristics of annual lunar mass movements literature: (A) Number of publications from 1872–2022 increases exponentially (R2 = 0.7064, remove the years with no publication). The number of articles over years indicates that the evolution of lunar mass movements research could be divided into three stages (1973 and 2009 are the turning points). (B) Numbers of publications each decade. Most publications (98%) related to the lunar mass movements have been published in the recent 60 years.
The articles were published in 175 publications and classified into three categories: Journal article, Conference contributions (talks and posters), and Book chapter, while the majority of them (55.6 percent) were concentrated in 32 publications. The journals with the highest number of publications were Icarus with 96 (9.9%), Journal of Geophysical Research (JGR) with 78 (8.1 percent), Planetary and Space Science (P&SS) with 30 (3.1 percent), Acta Astronautica (AcAau) with 24 (2.5 percent), Advances in Space Research (AdSpR) with 23 (2.4 percent). In addition, some Ph.D. T (Ph.D. thesis) is of great reference value with 27 (2.8 percent) (Figure 3A). The remaining papers (44.4%) were published in 143 different publications. From the data above, we could figure out which journals are usually available to acquire articles related to lunar mass movements.
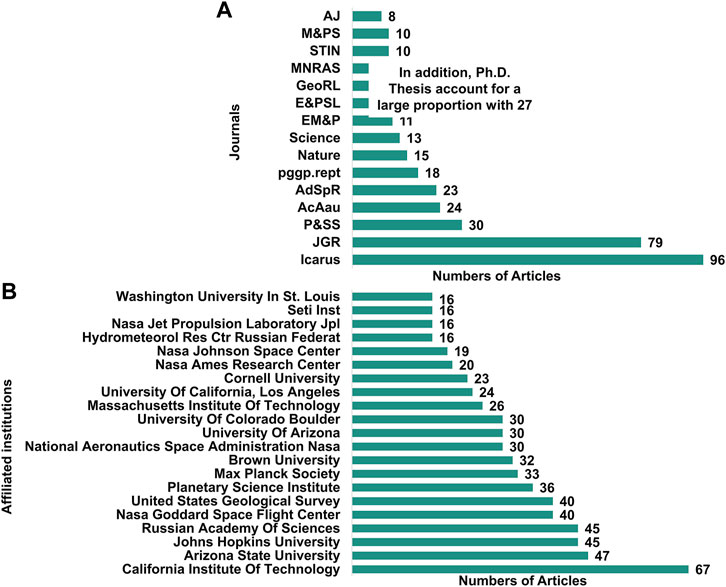
FIGURE 3. Statistics on the sources of literature on lunar mass movements: (A) The 15 productive journals in lunar mass movements research. (B) The 20 productive institutions in lunar mass movements research.
3.2 Authorship
The 965 articles examined included a total of 2,622 authors. We create an author network by taking the top 200 most often appearing writers in the database and measuring the frequency of collaboration between all authors (Figure 4). According to the frequency of collaboration, the authors were split into eight groups, and the lines indicated some collaboration with authors in other groups. The analysis shows that authors in group 1 focused on the erosion of the surface by ejecta on different planets. The second group studied the characteristics, distribution, and age of valleys and landslides on Mars, and the topic of group 3 is geological structures and process on different planets. Group 4 studied the lunar regolith based on image processing. The fifth group investigated the evolution of the planetary surface. Group 6 focuses on the evolution of craters on the Moon, and group 7 is about the thermodynamics of planets. The rest were grouped into the “other” group.
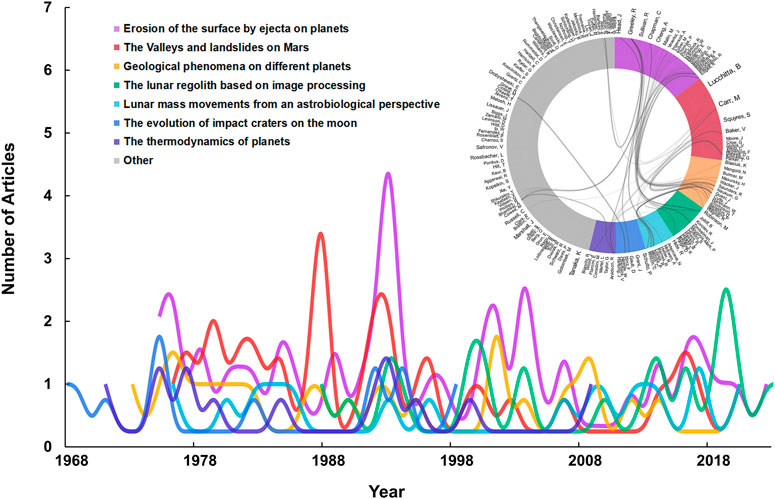
FIGURE 4. Cooperative relationship network of authors. The pie chart displays the authors grouped by the cooperation frequency and named each group by the common research fields, and lines indicate the other collaboration between authors. The line chart reflects the timeline view of the research group activity.
Over time, the research activeness of each group varies. The first group persisted in their research related to lunar mass movements and produced publications on the subject throughout time. Furthermore, group 4 has continued to conduct relevant research in recent years, and added fresh research findings to the database this year. For the past 4 years, groups 2, 3, and 5 have been without a new article about the subject, and group 7 has not published a new research output for approximately 15 years. Finally, the researchers of the sixth group have not updated their articles anymore since 1997.
The affiliations of the authors of the articles were dispersed among 450 institutions, including universities, research institutes, and centers. The country distribution of the articles was counted based on the affiliated institutions of the first author, as shown in Figure 5, which are mainly distributed in the United States (33.49%), Russia (14.10%), China (13.13%), Germany (6.99%), and Italy (6.27%). Coincidentally, among these countries, the United States has successfully launched 30 lunar exploration missions, Russia has successfully launched 27, China has launched 7, and the European Space Agency has launched 1. More than half of the published articles (54.2 percent) were concentrated in 15 institutions, California Institute of Technology (6.9 percent), Arizona State University (4.9 percent), Johns Hopkins University, and Russian Academy Of Sciences (4.6 percent each), NASA Goddard Space Flight Center and United States Geological Survey (4.1 percent each), Planetary Science Institute (3.7 percent), Max Planck Society (3.4 percent), Brown University (3.3 percent), National Aeronautics and Space Administration (3.3 percent) (Figure 3B).
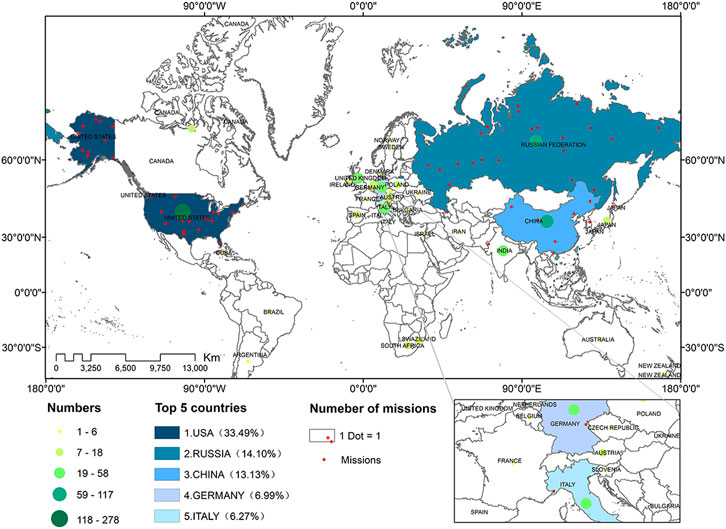
FIGURE 5. Geographical distribution of lunar mass movements publication volume level (Counted based on the affiliated institutions of the first author). The top 5 productive countries in lunar mass movements research are highlighted, the number of scatter points indicates the number of the lunar exploration missions each country launched.
3.3 Interdisciplinarity
The papers in the database involve 35 disciplines, with Astronomy accounting for 686 (57 percent), Physics for 221 (18 percent), Engineering for 92 (8 percent), Earth and Planetary Sciences for 77 (6 percent), Geochemistry Geophysics for 32 (3 percent), and Geology for 29 (2 percent). (Figure 6).
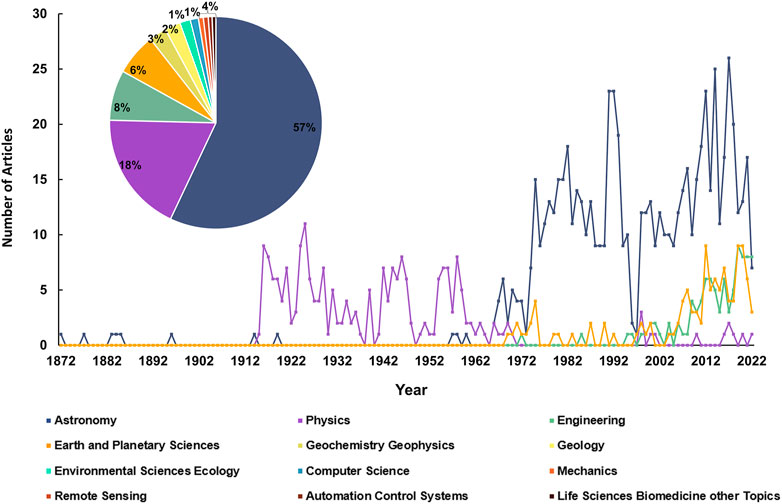
FIGURE 6. Disciplines involved in the research on lunar mass movements. The pie chart is characteristic of the discipline resulting from publication analysis. The line chart is the characteristics of the annual publication of different disciplines.
The changing trend of several disciplines (including a large proportion of articles) from the time dimension was further analyzed. In the direction of physics from 1915 to 1970, a total of 209 publications (94.6 percent) were published, with an average of four papers a year. Papers in Astronomy surged beginning in 1966 and have remained at a high level, averaging 12 publications annually, whereas previously relevant articles were published occasionally. Engineering studies focused mostly on the design and application of spacecraft; the first relevant article we searched was published in 1972, and after that, an increasing number of related publications were published, with an average of six articles per year for nearly 10 years. Earth and Planetary Sciences has been studied since 1969 and has been booming in recent years, with 75.3 percent of publications published in nearly 10 years, averaging 5.8. (See Figure 6).
The retrieved articles are from multiple scientific directions suggesting that the study of lunar mass movements is valuable in different scientific fields. An analysis from the time dimension reveals the development trend of several key disciplines in lunar mass movements research. Most of the articles are in the field of Astronomy, which is partly due to the absence of geology-focused journals in the past, but it can also indicate that the current research on lunar mass movements is mainly focused on this direction The rapid expansion of Earth and Planetary Sciences and many space missions in recent years validates the extensive application of comparative planetary methods in the study of lunar mass movements.
3.4 Quotation
The 965 papers in the database have been cited a total of 20,747 times between 1 January 1964, and 18 May 2022, including 409 self-citations. Each article was cited 21 times on average. Figure 7A demonstrates that the number of citations increases linearly over time. The total number of reads of these publications since 1996 was 109,094, with an average of 113 reads per publication, according to data collected by the system from ADS, Wos, and Scopus about the rate at which articles have been accessed. And 36,959 downloads were made in total, with a 38.3 download average. The data mentioned above illustrates the expansion of the impact of research on lunar mass movements, which is logical given the trend of synchronous rise in the number of articles.
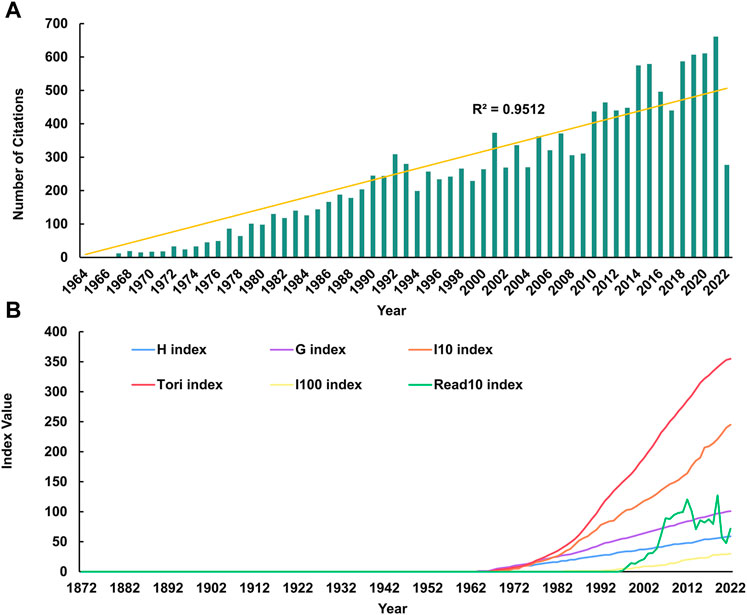
FIGURE 7. Overview of the citation of the literature on lunar mass movements: (A) The citation of related articles increases linearly over time (R2 = 0.9512). (B) Characteristics of the index of citation per year.
We utilize six indexes, including the h-index1, g-index2, i10-Index3, i100-Index, tori-Index4, and read10-Index5, to provide a summary of the citations, usage, and derived indicators of these articles. Because of author disambiguation issues, the result does not remove self-citations based on the author’s name but instead uses a list-based removal of self-citations. Since 1962, the tori-index has increased rapidly, eventually peaking at 354.9, indicating that as time goes on, the research on the mass movements of the Moon and the contribution of earlier work to subsequent research grows. Additionally, the maximum values of the i10-index and i100-index were each 245, while the maximum values of the h-index and g-index, with maximum values of 59 and 101, respectively, indicated a steadily ascending trend. The trend indicates that the number of articles with a high citation rate is increasing, implying growth of the academic impact of articles about the lunar mass movements. The Read10-index has fluctuated since 2005, indicating that the author’s research on the mass movements of the Moon was volatile during the period. The statistics “0” in the chart are due to a data shortage (Figure 7B).
The co-citation network groups articles based on shared references across all publications and names them by searching for common, unique words in their titles. And the lines indicated shared references with papers from other groups, the size of the line reflects the frequency of co-citation (Figure 8). As shown, articles relating to the lunar impact crater and the Martian valley are frequently cited within the same article, implying that lunar mass movements research is commonly carried out by comparing the mass movements of Mars and the Moon. It can also be investigated by comparing the mass movements of other planets. Additionally, surface erosion, slope stability, and magnetosphere effect are some other directions that are researched frequently.
4 Discussion
4.1 Data for lunar mass movements research
The statistical analysis results of publication years and country distribution indicate that as lunar exploration technology matures, there is an increasing number of articles focused on studying lunar mass movements. This observation highlights the necessity of lunar exploration missions in providing fundamental lunar data for conducting research on lunar mass movements. At present, three ongoing lunar missions must be mentioned: the Lunar Reconnaissance Orbiter (LRO) by the National Aeronautics and Space Administrations (NASA, United States), the SELenological and ENgineering Explorer (SELENE-KAGUYA) by the Japan Aerospace Exploration Agency (JAXA, Japan), and the Chang’E missions by the Chinese National Space Administration (CNSA, China) (Scaioni et al., 2018) (Table 1).
The open-source Java Mission Planning and Analysis for Remote Sensing (JMARS) software, a WEB-GIS platform developed by the Arizona State University (Burris et al., 2022), allows for handling planetary remote-sensing datasets. The USGS’s Integrated Software for Imagers and Spectrometers (ISIS; https://isis.astrogeology.usgs.gov//) could use to perform a standard calibration procedure for Lunar Reconnaissance Orbiter Camera (LORC) images (Chin et al., 2007).
The first lunar landslides the researchers encountered were several landslides around the Tsiolkovskiy crater (Boyce et al., 2020). This landslide is the only large, long-runout landslide on the Moon, this makes the Tsiolkovskiy landslide an excellent end-member for the study of the mechanics and conditions that control the emplacement of such rapid natural mass flows and their runout distance. Over the years, the topographic parameters, morphological characteristics, formation mechanism, and surrounding areas of regolith, ejecta, geological age, and rock abundance of the landslides in the Tsiolkovskiy crater have been well studied (Guest and Murray, 1969; Wu et al., 1972; Schultz, 1976b; Greenhagen et al., 2016; Morse et al., 2018; Boyce et al., 2020). Since then, numerous mass wasting features on a local scale have been noticed and reported based on a variety of lunar image data and terrain data (Table 2).
Even though various slope failure types have been examined on the Moon, there is still a lack of a comprehensive inventory of lunar landslides on a global scale. Table 3 lists the existing inventory of mass movements on the moon on a global scale.
From the list of mass movements, we found that researchers have used several morphological characteristic factors to describe the shape of the mass movements quantitatively (see Table 2). Moreover, Brunetti et al. (2015) compiled the inventory of 60 rock slides in the simple crater and using the planimetric area of the mapped failures calculated the probability density distribution of the landslide area. The measuring methods in detail list in Table 4 Below.
4.2 Methods used in lunar mass movements research
Three approaches are most commonly used to study mass movements on the Moon: 1) Image recognition, which is used to extract the boundary information of mass movements, thus studying their morphological, evolutionary, formational, distributional, and erosional characteristics; 2) Model simulation; physical models are usually constructed to simulate the lunar environment to study the development process and formation causes of mass movements; 3) Comparative planetology, by comparing the similar characteristics of different planets, the characteristics, influencing factors and classification standard of lunar mass movements can be well interpreted.
As research advances, image recognition techniques are gradually becoming semiautomatic or automatic from the manual. There are three main approaches: visual interpretation incorporating expert knowledge; recognition based on morphological features; and recognition based on machine learning or deep learning methods (Table 5). The primary method is visual interpretation with expert knowledge at present due to the diversity of types and complex morphological characteristics, which is slow and laborious. Technology for automatic identification is evolving, whereas the method is only capable of detecting a single type of mass movement at present. And recognition methods based on machine learning or deep learning lack sample libraries containing multi-scale morphological information of lunar mass movements. Therefore, more comprehensive automatic recognition methods need to be developed in combination with artificial intelligence technology in the future.
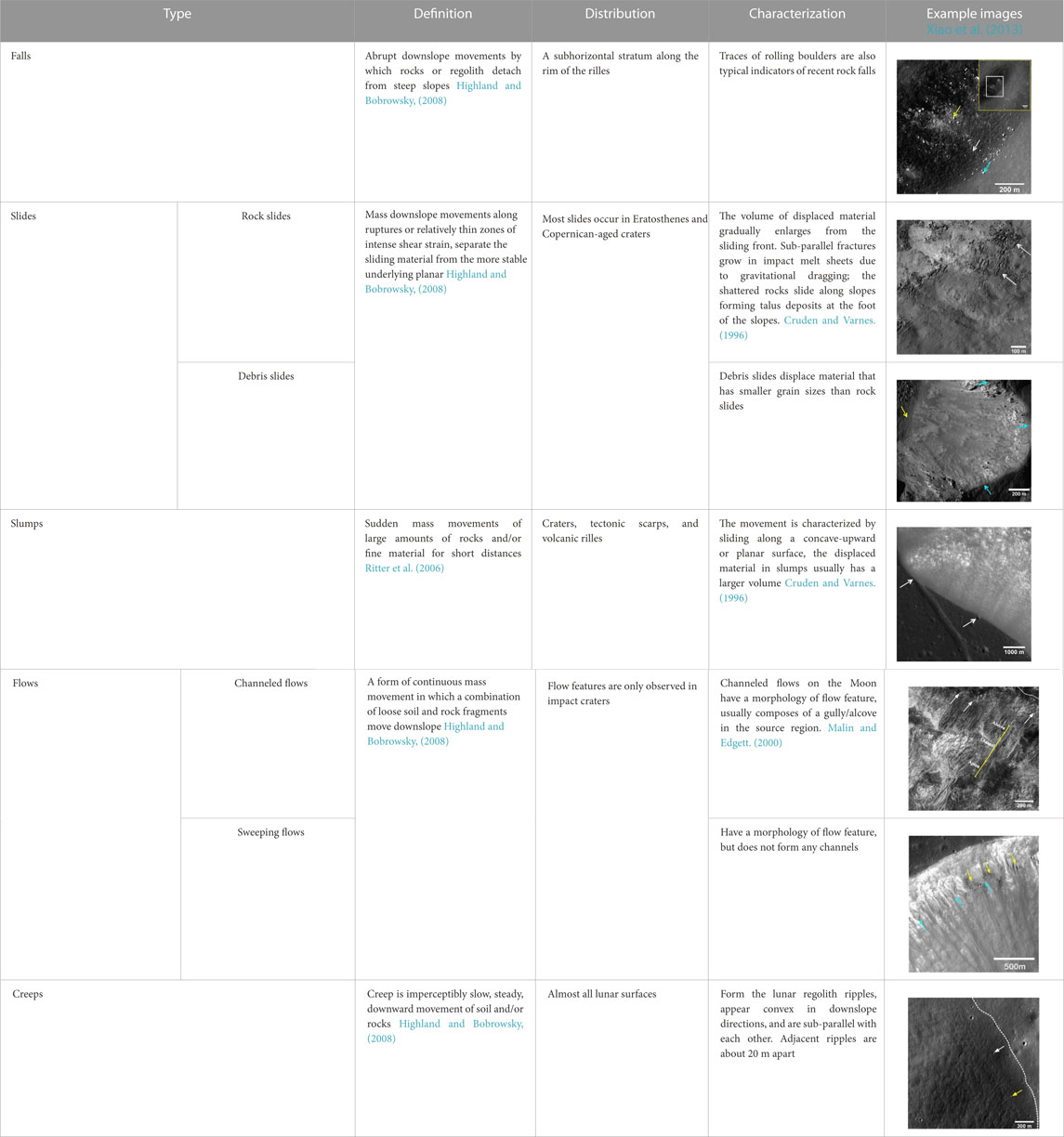
TABLE 6. Characteristics of different types of lunar mass movements [Modified from Xiao et al. (2013)]
Physical model simulation compensates for the fact that researchers could not contact the lunar surface, helps us to imitate the process of movements, and explore the factors that influence mass movements and the effects on geomorphic evolution. By the discrete element method, Bui et al. (2009) introduce the self-locking ability between particles into the particle contact model to study the slope collapse of lunar soil. Since then, the discrete element method has become a common method for simulating lunar soil landslides, analyzing landslide processes and landslide mechanisms, and evaluating the engineering disaster index (Liao et al., 2018; Liever et al., 2018; Wu et al., 2020). To investigate the influence of the low gravity environment on particle fluidity, Huang and Mao (2013) used the drop tower to design a system to simulate a low gravity environment, which captured the configurations and variability in the flow materials by drop-tower tests. Zheng and Huang (2015) used silicon carbide as a lunar regolith simulant to study the flow patterns of lunar regolith by capturing configurations of the flow and calculating the engineering strains under the action of different chute angles.
As mentioned in the introduction, the mass movement process is widely distributed on Earth, Moon, Mars, Mercury, Venus, and some icy satellites and asteroids in the outer Solar System, with some of the same or similar characteristics, meaning that their forming may have similar properties. As a result, when studying the classification, formation, and influencing factors of mass movements on the Moon, researchers frequently refer to mass movements on other planets (Hargitai and Kereszturi, 2015). For example, an alcove, a channel, and a deposition apron are characteristics shared by lunar crater-wall landslides and Martian gullies. Fine granular material without liquid water collapsing results in the formation of Martian gullies. Also, on the Moon, there is no atmosphere and water, these observations suggest that dry landslides can form recess-channel-apron morphology on the planets (Bart, 2007). Mass-wasting such as slumps, slides, and flow-like features are both found in the Rheasilvia crater on Vesta and the Tycho crater on the Moon. Analyzing the similarities and differences of mass-wasting features on the Moon and asteroid Vesta concerning their gravity shows that slumping is triggered when gravity forms a torque on the slumping body which exceeds the frictional forces and slumping develops more efficiently on bodies with higher gravity (Otto et al., 2014). Rock slides on the Moon are, on average, more massive than analogous rock slides on Mercury, according to a comparison of the size of landslides on the simple impact crater-wall on the Moon and Mercury (Brunetti et al., 2015). Therefore, it is hypothesized that authors a result of the properties of the rock material or possibly a result of the lunar surface gravity, which is weaker than those of Mercury’s (Brunetti et al., 2015). Furthermore, the lunar mass movements classification system was updated from the Varnes (1978) classification system for terrestrial landslides based on movement characteristics and material properties (Cruden and Varnes, 1996).
4.3 Parameters of morphological and triggering analysis
As mentioned above, several variables were employed to quantify the morphological characteristics of mass movements including geometric parameters such as length, width, surface area, volume, and average thickness; mobility parameters such as L/H, L/V, and H/V; and other factors such as slope angle, which were typically determined using ArcMap software (Table 4). These morphometric parameters serve as indicators that reflect the effects of mass movements on topographic modification of the lunar surface. They are integrated into topographic analysis for quantitative comparison to highlight the difference of mass wasting within various environments, thus revealing the triggers and modification effects on the terrain of the lunar mass movements. For example, slope angles measurement for the host terrains of the mass wasting features aids in detecting the minimum slopes on which mass wasting can initiate. This provides insights into the slope evolution of lunar surface terrains under the influence of mass wasting (Xiao et al., 2013). Furthermore, comparing the details of topographic features on mass wasting in different craters enables exploration of whether mass wasting in mare settings differs significantly from that in highlands. Comparisons with terrestrial counterparts could also aid in understanding lunar surface processes (Kokelaar et al., 2017).
The modification of the surface topography by lunar mass movements focuses on two aspects: the modification of the sloping topography and the modification of the deposit topography. The lunar gravity gradually changed the slope height difference to a flatter topography by moving the slope material toward a region with a gentler topography. Therefore, mass movements generally occur preferentially over slopes forming lately, with a steeper slope angle (Xiao et al., 2013). Thus, the distribution of mass movements could provide a reference for relative age estimates in lunar topographic units, which was shown in Bickel et al. (2020) using the quantitative of rockfalls. In addition, the type and velocity of mass movements are different on the different side slopes. For example, older surfaces on the Moon generally have slopes much less than the angle of repose of lunar materials, where creeping of regolith is the dominate landslides (Xiao et al., 2013), and the speed at which the debris moves increases with slope steepness (Soderblom, 1970).
Compared to the same volume of landslides on the Earth, the lunar landslide has a much larger scale of mass wasting, resulting in a spectacular accumulation of landforms (Xiao et al., 2013). It is due to the Moon having a sixth gravity of the Earth, which means the material formed is less fragmented under the small gravity force of the Moon for the same material strength. Additionally, the weathered lunar regolith on the Moon’s surface can lubricate coarse particles and reduce sliding friction, lengthening, extending the path of mass movements (Riller and Lieger, 2008), and giving the accumulation landform a slender appearance, as well as the regolith would damp the impact of individual particles, reducing fragmentation (Kokelaar et al., 2014). The density of lunar soil on the lunar slope is lower than that on the flat surface, and the bedrock erosion rate is higher than that on the flat surface because large-scale movement exposes the bedrock by clearing debris on the slope (Lin et al., 2020). In conclusion, the combined effect of small-scale microscopic gardening and mass movements will lessen the slope’s fluctuation while burying the downhill and making it flat or fluctuate gently.
Studying the triggering of mass movements is inextricably linked to investigating its morphological features and spatial distribution traits. On the Moon, endogenic dynamic processes (such as volcanism, tectonism, tides, and body shrinkage) and seismic activity induced by impact cratering, as well as thermal stresses raised by diurnal temperature changes, are among the forces that could result in deformation and failure in slope materials (Watters et al., 2019; Head et al., 2020) (Figure 9).
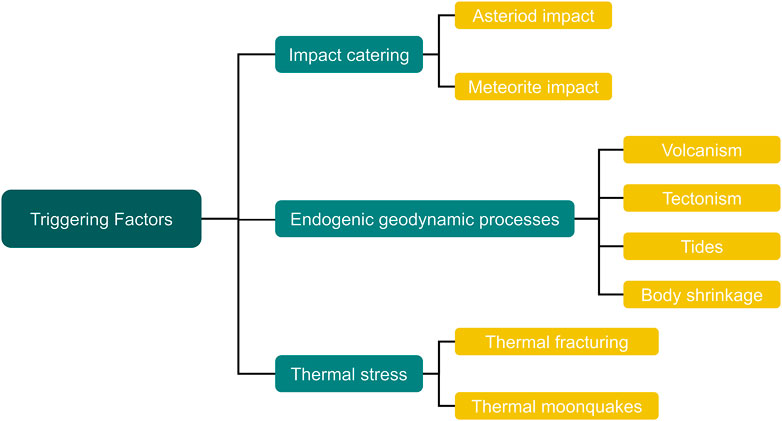
FIGURE 9. Triggering factors for mass movements on the Moon [Modified from Xiao. (2021)].
Impact cratering includes asteroid impact, meteorite impact, etc. Different sized impact bodies have various impact effects, and seismic activity caused by impacts can have an impact on a much larger areas than the original impact craters [(Schultz, 1976a). The impact destroys the composition material’s structure and original topography. The impact destabilizes the slope by causing a mass movement and changing its morphological characteristics, makes the slopes gentler and the surface regolith layer thicker and results in degraded craters (Bickel et al., 2022; Ikeda et al., 2022; Chandnani and Herrick, 2023)]. The impact of micrometeorites (0.1–2 mm) can also degrade the surface of the rock and possibly cause the granule on the surface to separate triggered debris flow and gardening produced agglutinate formation and related cohesion increase (Soderblom, 1970; Hörz et al., 1971; Basilevsky et al., 2013; Bandfield et al., 2014; Bickel et al., 2021; Bickel et al., 2022; Ikeda et al., 2022). Impact-related seismicity is shallow seismic, and tectonic activity can also be the cause of shallow seismic. Boulder falls are not caused by shallow moonquakes along lobate scarps but by ground shaking due to meteorite impacts (Ikeda et al., 2022).
The rate of diurnal temperature change is less than 2 K/min on the Moon ((Molaro and Byrne, 2012), but grain-scale stresses induced by diurnal temperature changes are on the order of 100 MPa (Molaro et al., 2015). This magnitude of stress is comparable to the typical strength of lunar rocks. Thus, the temperature changes lead to the grain reorientation caused by the differential expansion of the matter to induce mass movements. The strength of thermal weathering depends on the size of the rock mass’s particles and albedo and thermal inertia, and some research has found that the thin regolith cover would prevent the initiation of thermal fracturing (Molaro et al., 2017; Ruesch et al., 2020). Besides thermal fracturing, thermal moonquakes, which happen only in extremely dry sand with a smooth surface may be another mechanism driving lunar landslides (Linasay, 1976; Bickel et al., 2021).
There are no affect factors like rainfall, melting snow, and frequent human activities of mass movements on the Moon compared to the mass movements on the Earth. Other factors, such as material composition, terrain conditions, lunar exploration and land missions, and space weathering, may play an important role in the formation and development of the lunar mass movements. In addition, whether the lunar soil contains water ice, what form of water storage, and how much water is still controversial, which will also have an important impact on the cause of the mass movements (Bart, 2007; McCubbin et al., 2015). At the same time, the mechanical properties of the rock composition material will affect the stability of the slope and the formation and development of the mass movements.
As lunar exploration engineering technology, such as the Artemis project (Barzilla et al., 2021) continues to advance, an increasing number of lunar orbiters and manned landers are impacting the lunar surface environment. According to recent news reports, scientists have found an orbiter component that has fallen into a fresh impact crater on the far side of the Moon. This intriguing finding has led us to speculate that external impact events of a similar nature could generate varying degrees of vibration across different scales of lunar surface regions. Such vibrations may potentially contribute to the occurrence of mass movements.
4.4 Future research direction
The research in this field describes a higher increase in the last 14 years (2009–2022), which has benefited from the dozens of successful manned and unmanned exploration Missions. In light of ongoing advancements in lunar exploration technology and an abundance of lunar exploration data, lunar mass movements have attracted the attention of an increasing number of researchers as an ideal window to study the transformation effects of internal and external forces on the morphology of the Moon’s surface. However, the following problems still need to be solved.
At present, the recognition and mapping of mass movements still rely on visual interpretation of integrated expert knowledge, which is a time-consuming task (Nass et al., 2018), while suffering from artificial and technological limitations and biases. This contributes to the lack of catalogs on a global scale including all kinds of mass movements, and the lack of knowledge about the spatial distribution and density of mass movements. Research by Bickel et al. (2021), Bickel et al. (2022) used a machine learning-driven approach to solve this major limitation of human-driven, mapped 28,101 flow features and 136,610 boulder tracks between 60°N and S. These works opened the door to a statistically sound, global-scale analysis of the spatial distribution, density, and drivers of rockfalls and granular flows. However, a comprehensive inventory of other types of mass movements is still lacking. A morphological information sample database of multi-scale mass movements needs to be constructed to complete the global-scale database of mass movements, and machine learning or deep learning methods for identifying more types of lunar mass movements need to be experimented with. Then, a global-scale analysis of the spatial distribution, and density of all kinds of mass movements could be carried out.
Existing classification schemes for mass movements have unclear classification metrics. The current classification of the mass movements modified the classification system adopted by Varnes’s (1978) for terrestrial landslides, which is classified by three indicators: movement process, slope morphology, and deposition characteristics (Kokelaar et al., 2017). Classifying lunar landslides based on the characteristics of terrestrial landslides would be incomprehensible. There are a series of problems, such as environmental simulation costs being high, explaining characteristic differences being difficult, and reference standards being inconsistent. It is urgent to establish a classification index system combining morphology, slip materials, and movement mode according to mass movements’ morphology and spatial distribution characteristics. Rajaneesh et al. (2022) defined 15 critical parameters of the Martian landslide inventory viz., length (L), width (W), relative relief (RR), area, L/W ratio, RR/L ratio, TI of landslide (mean, minimum, and maximum), and slope statistics (mean, minimum, and maximum), TI of the scarp, the slope of the scarp, and geology, which were analyzed using multiple discriminant analysis (MDA), it turns out that the parameters RR/L, L/W, and RR were suggested to be the parameters that primarily discriminates the Martian landslides. And then machine learning algorithms were used to evaluate the classification of landslides. This study demonstrates the potential of using machine learning models to classify extra-terrestrial landslides. Therefore, the main research direction of lunar landslide classification in the future is using machine learning algorithms to design a data-driven classification parameter system based on the spatial distribution characteristics and the morphological characteristics, integrating the environmental and geological information.
Triggers of the mass movements discussed above were short of compelling statistical evidence at present. This is partly limited by the lack of a comprehensive global-scale inventory. With the available data, only one or a few factors of a single type of mass movement could be conducted a quantitative assessment on a small scale by researchers, which cannot meet the demands of multi-scale big data analysis. The differences in the morphological characteristics and spatial distribution of mass movements were significant because its formation process is always influenced by the interaction of environmental and geological factors. In the study of terrestrial landslides, the landslide susceptibility model is usually constructed by multiple discriminant analysis of triggers such as terrain, hydrology, and human activities through algorithm models such as logistic model tree, random forest, support vector machine, and Bayesian (Chen et al., 2017; Pham et al., 2019; Sun et al., 2020; Xie et al., 2021). Thus, driven by machine learning and big data technology, there is a need to construct the mathematical model to illustrate the intersecting response mechanism of triggers for lunar landslides based on the multiple discriminant analysis of environmental, geological, and geomorphic on a multi-scale.
The study on the modification effect of mass movement on the lunar surface morphology is mostly a qualitative analysis, and without quantitative indicators and universality, as discussed above. In future studies, comparing the transformation scale under various types, terrain conditions, composition materials, space environment, and other conditions could be considered to develop a systematic evaluation index system, and to provide parameters for revealing the evolution process and mechanism of the lunar surface morphology.
It is anticipated that the study of lunar mass movements will advance significantly based on novel data sets acquired in the new era of lunar exploration, evolving computational methods, and GIS big data capabilities as a result of growing recognition of the significance of lunar mass movements research and the development of unmanned and manned lunar exploration projects.
5 Conclusion
The paper generates a database of articles about the lunar mass movements that reflects general information (e.g., data, tool, and method) related to the research of lunar mass movements and highlights the recent advances and the interest areas.
This study shows that the main and frontier research topics of lunar mass movements include the erosion of the surface by ejecta, geological phenomena, the lunar regolith, the evolution of planetary surface, the evolution of lunar impact craters, and thermodynamics. Icarus, JGR, and P&SS are the top three productive publishing journals. And research on lunar mass movements is dominated by a few countries, institutions, and scholars. California Institute of Technology, Arizona State University, and Johns Hopkins University are active institutions leading the lunar mass movements research, and the related institutions are mainly located in the United States, Russia, and China, considering their successful launch of lunar exploration missions as well as investments in research and development, it is not surprising. Frequent collaboration is carried out between scholars on the same or similar subjects to promote the development of research on the lunar mass movements.
Astronomy and physics are the primary disciplines, with research on the modification effect and degree of mass movements on the lunar surface being crucial to the exploration of the geological structure and evolution of the Moon. The citation index analysis indicates the growth of the academic impact of articles about the lunar mass movements. Moreover, co-citation network analysis, which provides important information about the research direction, revealed that comparative planetology was commonly used in the research on the characterization of lunar mass movements, and surface erosion, slope stability, and magnetosphere effects are some directions that are researched frequently.
Reviewing existing studies of lunar mass movements, we propose four unsolved issues. 1) The comprehensive database of lunar mass movements that records all types of landslides incidents on a global-scale has not been compiled. 2) The classification indicators to quantify the classification criteria for the lunar mass movement have not been definite. 3) The mathematical model to explain the triggering mechanism of mass movements on the Moon has not been built. 4) The quantitative indexes to evaluate the modification effect of mass movements on the lunar surface morphology have not been identified. Under the background of continuous innovation in lunar exploration technology and continuous enrichment of lunar exploration data, more types of mass movements should be identified and mapped on a global-scale with artificial intelligence technology to expand lunar landslide database, Multiple discriminant analysis and machine learning tools could be used to construct a lunar landslide classification system, and combined with feature datasets like crater size-frequency distribution, moonquake, impact flux, and topographic, driven by lunar landslide database, the causes and modification effects of mass movements could be clarified finally.
To sum up, this review provides a longitudinal survey of the published literature about the lunar mass movement in the last 151 years. It is hoped that these studies can inspire researchers from the field of lunar surface processes, especially new researchers, to understand the lunar mass movements.
Author contributions
JW conceived the idea of the study. KL wrote the paper. KL and JW interpreted the results. YS analyzed the data. All authors contributed to the article and approved the submitted version.
Funding
This work was supported in part by National Key Research and Development project (No. 2019YFE0123300), National Natural Science Foundation of China (No. 41801361), and the Fundamental Research Funds for the Central Universities (No. 2652019027).
Acknowledgments
The authors wish to give thanks to the valuable comments and suggestions given by two referees.
Conflict of interest
The authors declare that the research was conducted in the absence of any commercial or financial relationships that could be construed as a potential conflict of interest.
Publisher’s note
All claims expressed in this article are solely those of the authors and do not necessarily represent those of their affiliated organizations, or those of the publisher, the editors and the reviewers. Any product that may be evaluated in this article, or claim that may be made by its manufacturer, is not guaranteed or endorsed by the publisher.
Footnotes
1Hirsch’s h-index is the largest number H such that H publications have at least H citations. It attempts to measure the productivity and impact of a researcher in a single number.
2Given a set of articles ranked in decreasing order of the number of citations that they received, the g-index is the (unique) largest number such that the top g articles received (together) at least g2 citations.
3The iN-index is the number of publications with at least N citations.
4The total research impact of a scholar (tori) is calculated using the reference lists of the citing papers, where self-citations are removed. The contribution of each citing paper is then normalized by the number of remaining references in the citing papers and the number of authors in the cited paper. The tori-index is defined as the amount of work that others have devoted to his/her research, measured in research papers.
5The Read-10 index represents the present readership rate for an individual’s papers published within the last 10 years, normalized according to the number of authors.
6Image source: Xiao et al., 2013.
References
Bandfield, J. L., Song, E., Hayne, P. O., Brand, B. D., Ghent, R. R., Vasavada, A. R., et al. (2014). Lunar cold spots: Granular flow features and extensive insulating materials surrounding young craters. Icarus 231, 221–231. doi:10.1016/j.icarus.2013.12.017
Bart, G. D. (2007). Comparison of small lunar landslides and martian gullies. Icarus 187, 417–421. doi:10.1016/j.icarus.2006.11.004
Barzilla, J., Semones, E., Lee, K., Johnson, A. S., Quinn, P., Whitman, K., et al. (2021). “Concept of operations and requirements for the Artemis mission,” in AGU fall meeting abstracts (New Orleans, LA: AGU).
Basilevsky, A. T., Head, J. W., and Horz, F. (2013). Survival times of meter-sized boulders on the surface of the Moon. Planet. Space. Sci. 89, 118–126. doi:10.1016/j.pss.2013.07.011
Bhardwaj, A., and Sam, L. (2022). Reconstruction and characterisation of past and the most recent slope failure events at the 2021 rock-ice avalanche site in chamoli, Indian himalaya. Remote Sens. 14, 949. doi:10.3390/rs14040949
Bickel, V. T., Aaron, J., Manconi, A., and Loew, S. (2021). Global drivers and transport mechanisms of lunar rockfalls. J. Geophys. Res. Planet. 126 (10), 6824. doi:10.1029/2021JE006824
Bickel, V. T., Aaron, J., Manconi, A., Loew, S., and Mall, U. (2020). Impacts drive lunar rockfalls over billions of years. Nat. Commun. 11, 2862. doi:10.1038/s41467-020-16653-3
Bickel, V. T., Loew, S., Aaron, J., and Goedhart, N. (2022). A global perspective on lunar granular flows. Geophys. Res. Lett. 49 (12), 98812. doi:10.1029/2022GL098812
Boyce, J. M., Mouginis-Mark, P., and Robinson, M. (2020). The Tsiolkovskiy crater landslide, the moon: An LROC view. Icarus 337, 113464. doi:10.1016/j.icarus.2019.113464
Bray, V. J., Tornabene, L. L., Keszthelyi, L. P., McEwen, A. S., Hawke, B. R., Giguere, T. A., et al. (2010). New insight into lunar impact melt mobility from the LRO camera. Geophys. Res. Lett. 37, 4666. doi:10.1029/2010gl044666
Brunetti, M. T., Guzzetti, F., Cardinali, M., Fiorucci, F., Santangelo, M., Mancinelli, P., et al. (2014). Analysis of a new geomorphological inventory of landslides in Valles Marineris, Mars. Earth Planet Sc. Lett. 405, 156–168. doi:10.1016/j.epsl.2014.08.025
Brunetti, M. T., Xiao, Z., Komatsu, G., Peruccacci, S., and Guzzetti, F. (2015). Large rock slides in impact craters on the Moon and Mercury. Icarus 260, 289–300. doi:10.1016/j.icarus.2015.07.014
Bui, H. H., Kobayashi, T., Fukagawa, R., and Wells, J. C. (2009). Numerical and experimental studies of gravity effect on the mechanism of lunar excavations. J. Terramechanics 46, 115–124. doi:10.1016/j.jterra.2009.02.006
Burris, M., Dickenshied, S., Anwar, S., Anderson, Z., Hagee, W., Noss, D., et al. (2022). “Jmars 5: A modernized interface for planetary exploration analysis,” in AGU fall meeting abstracts (Chicago: AGU).
Chandnani, M., and Herrick, R. R. (2023). Influence of target properties on wall slumping in lunar impact craters within the simple-to-complex transition. J. Geophys. Res. Planets. 128 (1), 7369. doi:10.1029/2022JE007369
Chen, W., Xie, X., Wang, J., Pradhan, B., Hong, H., Bui, D. T., et al. (2017). A comparative study of logistic model tree, random forest, and classification and regression tree models for spatial prediction of landslide susceptibility. Catena 151, 147–160. doi:10.1016/j.catena.2016.11.032
Chin, G., Brylow, S., Foote, M., Garvin, J., Kasper, J., Keller, J., et al. (2007). Lunar reconnaissance orbiter overview: The Instrument suite and mission. Space Sci. Rev. 129, 391–419. doi:10.1007/s11214-007-9153-y
Corominas, J., van Westen, C., Frattini, P., Cascini, L., Malet, J. P., Fotopoulou, S., et al. (2014). Recommendations for the quantitative analysis of landslide risk. B Eng. Geol. Environ. 73, 209–263. doi:10.1007/s10064-013-0538-8
Corsini, A., Borgatti, L., Cervi, F., Dahne, A., Ronchetti, F., and Sterzai, P. (2009). Estimating mass-wasting processes in active Earth slides - Earth flows with time-series of High-Resolution DEMs from photogrammetry and airborne LiDAR. Nat. Hazard Earth Sys. 9, 433–439. doi:10.5194/nhess-9-433-2009
Craddock, R. A., and Howard, A. D. (2000). Simulated degradation of lunar impact craters and a new method for age dating farside mare deposits. J. Geophys. Res. Planet. 105, 20387–20401. doi:10.1029/1999je001099
Cruden, D. M., and Varnes, D. J. (1996). in Landslide types and processes. Editors A. K. Turner, and R. L. Schuster (Washington, DC: National Academy Press), 36–75.
Deplus, C., Friant, A. L., Boudon, G., Komorowski, J. C., Villemant, B., Harford, C., et al. (2001). Submarine evidence for large-scale debris avalanches in the Lesser Antilles Arc. Earth Planet Sc. Lett. 192, 145–157. doi:10.1016/s0012-821x(01)00444-7
Greenhagen, B. T., Neish, C. D., Williams, J. P., Cahill, J. T., Ghent, R. R., Hayne, P. O., et al. (2016). Origin of the anomalously rocky appearance of Tsiolkovskiy crater. Icarus 273, 237–247. doi:10.1016/j.icarus.2016.02.041
Guest, J. E., and Murray, J. B. (1969). Nature and origin of Tsiolkovsky crater, lunar farside. Planet Space Sci. 17, 121–141. doi:10.1016/0032-0633(69)90128-7
Hargitai, H., and Kereszturi, Á. (2015). Encyclopedia of planetary landforms. New York, NY, USA: Springer, 1–2460.
Head, J. W., Wilson, L., Deutsch, A. N., Rutherford, M. J., and Saal, A. E. (2020). Volcanically induced transient atmospheres on the moon: Assessment of duration, significance, and contributions to polar volatile traps. Geophys. Res. Lett. 47, 89509. doi:10.1029/2020gl089509
Highland, L. M., and Bobrowsky, P. (2008). The landslide handbook: A guide to understanding landslides. U. S. Geol. Surv. Circ. 1325, 3–25. doi:10.3133/cir1325
Hörz, F., Hartung, J. B., and Gault, D. E. (1971). Micrometeorite craters on lunar rock surfaces. J. Geophys. Res. 76, 5770–5798. doi:10.1029/jb076i023p05770
Huang, Y., and Mao, W. (2013). First results derived from a drop-tower testing system for granular flow in a microgravity environment. Landslides 10, 493–501. doi:10.1007/s10346-013-0403-7
Ikeda, A., Kumagai, H., and Morota, T. (2022). Topographic degradation processes of lunar crater walls inferred from boulder falls. J. Geophys. Res. Planets. 127 (10), 7176. doi:10.1029/2021JE007176
Kereszturi, A. (2023). Polar ice on the moon. Encycl. Lunar Sci. 1, 9. doi:10.1007/978-3-319-05546-6_216-1
Kereszturi, A. (2014). Surface processes in microgravity for landing and sampling site selection of asteroid missions—suggestions for MarcoPolo-R. Planet. Space. Sci. 101, 65–76. doi:10.1016/j.pss.2014.06.005
Kereszturi, A., Tomka, R., Gläser, P. A., Pal, B. D., Steinmann, V., and Warren, T. (2022). Characteristics of de Gerlache crater, site of girlands and slope exposed ice in a lunar polar depression. Icarus 388, 115231. doi:10.1016/j.icarus.2022.115231
Kokelaar, B. P., Bahia, R. S., Joy, K. H., Viroulet, S., and Gray, J. M. (2017). Granular avalanches on the Moon: Mass-wasting conditions, processes, and features. J. Geophys. Res. Planet. 122, 1893–1925. doi:10.1002/2017je005320
Kokelaar, B. P., Graham, R. L., Gray, J. M., and Vallance, J. W. (2014). Fine-grained linings of leveed channels facilitate runout of granular flows. Earth Planet Sc. Lett. 385, 172–180. doi:10.1016/j.epsl.2013.10.043
Kumar, P. S., Sruthi, U., Krishna, N., Lakshmi, K. J. P., Menon, R., Amitabh, G., et al. (2016). Recent shallow moonquake and impact-triggered boulder falls on the Moon: New insights from the Schrodinger basin. J. Geophys. Res. Planet. 121, 147–179. doi:10.1002/2015je004850
Li, B., Ling, Z., Zhang, J., Chen, J., Liu, C., and Bi, X. (2018). Geological mapping of lunar highland crater Lalande: Topographic configuration, morphology and cratering process. Planet Space Sci. 151, 85–96. doi:10.1016/j.pss.2017.11.007
Liao, Y., Jiang, M., Chen, Y., Xi, B., and Tan, Y. (2018). Discrete element analysis of landslide mechanism on lunar soil induced by different slope angles. J. Water Resour. Water Eng. 29, 194–200. doi:10.11705/j.issn.1672-643X.2018.02.32
Liever, P. A., Gale, M. P., Mehta, R. S., and West, J. S. (2018). Gas-granular simulation framework for spacecraft landing plume-surface interaction and debris transport analysis, in Granular Materials in Space Exploration. Ohio: ASCE Earth & Space, 39–48. doi:10.1061/9780784481899.005
Lin, Q., Cheng, Q., Li, K., Xie, Y., and Wang, Y. (2020). Contributions of rock mass structure to the emplacement of fragmenting rockfalls and rockslides: Insights from laboratory experiments. J. Geophys. Res. Sol. Ea. 125, 19296. doi:10.1029/2019JB019296
Lindsay, J. (1976). “Energy at the lunar surfaces,” in Lunar stratigraphy and sedimentology. Editors Z. Kopal, and A. G. Cameron (Amsterdam: Developments in Solar System and Space Science), 45–55.
Mahanti, P., Robinson, M. S., Humm, D. C., and Stopar, J. D. (2014). A standardized approach for quantitative characterization of impact crater topography. Icarus 241, 114–129. doi:10.1016/j.icarus.2014.06.023
Malin, M. C., and Edgett, K. S. (2000). Evidence for recent groundwater seepage and surface runoff on Mars. Science 288, 2330–2335. doi:10.1126/science.288.5475.2330
McCubbin, F. M., Vander Kaaden, K. E., Tartèse, R., Klima, R. L., Liu, Y., Mortimer, J., et al. (2015). Magmatic volatiles (H, C, N, F, S, Cl) in the lunar mantle, crust, and regolith: Abundances, distributions, processes, and reservoirs. Am. Mineral. 100, 1668–1707. doi:10.2138/am-2015-4934ccbyncnd
McEwen, A. S. (1989). Mobility of large rock avalanches - evidence from valles marineris, Mars. Geology 17, 1111–1114. doi:10.1130/0091-7613(1989)017<1111:molrae>2.3.co;2
Molaro, J., and Byrne, S. (2012). Rates of temperature change of airless landscapes and implications for thermal stress weathering. J. Geophys. Res. Plane 117, 413. doi:10.1029/2012JE004138
Molaro, J. L., Byrne, S., and Langer, S. A. (2015). Grain-scale thermoelastic stresses and spatiotemporal temperature gradients on airless bodies, implications for rock breakdown. J. Geophys. Res. Planet. 120, 255–277. doi:10.1002/2014je004729
Molaro, J. L., Byrne, S., and Le, J. L. (2017). Thermally induced stresses in boulders on airless body surfaces, and implications for rock breakdown. Icarus 294, 247–261. doi:10.1016/j.icarus.2017.03.008
Moore, J. G., Normark, W. R., and Holcomb, R. T. (1994). Giant Hawaiian landslides. Annu. Rev. Earth Pl. Sc. 22, 119–144. doi:10.1146/annurev.ea.22.050194.001003
Morse, Z. R., Osinski, G. R., and Tornabene, L. L. (2018). Morphologic mapping and analysis of Tsiolkovsky crater ejecta. Lunar Plant Sci. 56, 13650. doi:10.1111/maps.13650
Murdoch, N., Sánchez, P., Schwartz, S. R., and Miyamoto, H. (2015). Asteroid surface geophysics. arXiv preprint arXiv:1503.01931.
Nass, A., Di, K., Elgner, S., van Gasselt, S., Hare, T., Hargitai, H., et al. (2018). Planetary cartography–activities and current challenges. Proc. ICA. 1, 81–10. doi:10.5194/ica-proc-1-81-2018
Otto, K. A., Krohn, K., Balzer, J., Hoffmann, H., and Jaumann, R. (2014). Comparison of mass-wasting features in craters on asteroid 4 Vesta and the moon. (London: European Lunar Symposium), 15–16.
Parker, R. N., Densmore, A. L., Rosser, N. J., de Michele, M., Li, Y., Huang, R., et al. (2011). Mass wasting triggered by the 2008 Wenchuan earthquake is greater than orogenic growth. Nat. Geosci. 4, 449–452. doi:10.1038/ngeo1154
Perumal, S. K. (2012). “Gullies and landslides on the Moon: An example from Schrodinger basin,” in 39th COSPAR scientific assembly (Mysore, India: COSPAR), 1487.
Pham, B. T., Jaafari, A., Prakash, I., and Bui, D. T. (2019). A novel hybrid intelligent model of support vector machines and the MultiBoost ensemble for landslide susceptibility modeling. Bull. Eng. Geol. Environ. 78, 2865–2886. doi:10.1007/s10064-018-1281-y
Pike, R. J. (1971). Some preliminary interpretations of lunar mass-wasting process from Apollo 10 photography, analysis of Apollo 10 photography and visual observations. Washington, D. C: Work of the US Gov. Public Use Permitted, 14–20.
Pradhan, S. P., and Siddique, T. (2019). “Mass wasting: An overview,” in Landslides: Theory, practice and modelling. Editors S. P. Pradhan, V. Vishal, and T. Singh (Cham: OpenGeology). doi:10.1007/978-3-319-77377-3_1
Rajaneesh, A., Vishnu, C. L., Oommen, T., Rajesh, V. J., and Sajinkumar, K. S. (2022). Machine learning as a tool to classify extra-terrestrial landslides: A dossier from valles marineris, Mars. Icarus 376, 114886. doi:10.1016/j.icarus.2022.114886
Riller, U., and Lieger, D. (2008). Geological evidence for acoustic fluidization in large impact structures. Large Meteor. Impacts Planet. Evol. IV 1423, 3025.
Ritter, D. F., Kochel, C. R., and Miller, J. R. (2006). Process geomorphology. 4th edn. Long Grove: Waveland Press, 79–133.
Ruesch, O., Sefton-Nash, E., Vago, J. L., Kuppers, M., Pasckert, J. H., Khron, K., et al. (2020). In situ fragmentation of lunar blocks and implications for impacts and solar-induced thermal stresses. Icarus 336, 113431. doi:10.1016/j.icarus.2019.113431
Sam, L., and Bhardwaj, A. (2022). A remote sensing perspective on mass wasting in contrasting planetary environments: Cases of the moon and ceres. Remote Sens. 14, 1049. doi:10.3390/rs14041049
Scaioni, M., Yordanov, V., Brunetti, M. T., Melis, M. T., Zinzi, A., Kang, Z., et al. (2018). Recognition of landslides in lunar impact craters. Eur. J. Remote Sens. 51, 47–61. doi:10.1080/22797254.2017.1401908
Schenk, P. M., and Bulmer, M. H. (1998). Origin of mountains on io by thrust faulting and large-scale mass movements. Science 279, 1514–1517. doi:10.1126/science.279.5356.1514
Schultz, P. H. (1976b). Moon morphology: Interpretations based on lunar orbiter photography. Austin: University of Texas Press.
Simon, J., and de Bruyn, J. R. (2007). Shape of impact craters in granular media. Phys. Rev. E. 76, 041306. doi:10.1103/physreve.76.041306
Soderblom, L. A. (1970). A model for small-impact erosion applied to the lunar surface. J. Geophys. Res. 75, 2655–2661. doi:10.1029/jb075i014p02655
Sun, D., Wen, H., Wang, D., and Xu, J. (2020). A random forest model of landslide susceptibility mapping based on hyperparameter optimization using Bayes algorithm. Geomorphology 362, 107201. doi:10.1016/j.geomorph.2020.107201
Varnes, D. J. (1978). in Slope movement types and processes. Editors R. L. Schuster, and R. J. Krizek (Washington, D. C: National Academy of Sciences),11–33.
Waltham, D., Pickering, K. T., and Bray, V. J. (2008). Particulate gravity currents on Venus. J. Geophys. Res. Planet. 113, E02012. doi:10.1029/2007JE002913
Watters, T. R., Weber, R. C., Collins, G. C., Howley, I. J., Schmerr, N. C., and Johnson, C. L. (2019). Shallow seismic activity and young thrust faults on the Moon. Nat. Geosci. 12, 411–417. doi:10.1038/s41561-019-0362-2
Wolman, M. G., and Gerson, R. (1978). Relative scales of time and effectiveness of climate in watershed geomorphology. Earth Surf. Proc. 3, 189–208. doi:10.1002/esp.3290030207
Wu, S. S., Schafer, F. J., Jordan, R., Nakata, G. M., and Derick, J. L. (1972). Photogrammetry of Apollo 15 photography. Apollo Prelim. Sci. rept. 289, 36.
Wu, S., Wang, Y., Hou, X., Xue, P., and Long, L. (2020). Research on theoretical model of vertical impact of foot pad on lunar soil. Manned Spacefl. 26, 135–141. doi:10.3969/j.issn.1674-5825.2020.02.001
Xiao, Z., and Komatsu, G. (2013). Impact craters with ejecta flows and central pits on Mercury. Planet Space Sci. 83, 62–78. doi:10.1016/j.pss.2013.03.015
Xiao, Z. (2021). “Mass-movements on the moon,” in Treatise on geomorphology. Editor F. John (Jack), and Shroder (Academic Press: Elsevier, Oxford), 458–476. doi:10.1016/B978-0-12-818234-5.00047-X
Xiao, Z., Zeng, Z., Ding, N., and Molaro, J. (2013). Mass wasting features on the Moon - how active is the lunar surface? Earth Planet Sc. Lett. 376, 1–11. doi:10.1016/j.epsl.2013.06.015
Xie, W., Nie, W., Saffari, P., Robledo, L. F., Descote, P. Y., and Jian, W. (2021). Landslide hazard assessment based on Bayesian optimization–support vector machine in Nanping City, China. Nat. Hazards. 109 (1), 931–948. doi:10.1007/s11069-021-04862-y
Yordanov, V., Scaioni, M., Brunetti, M. T., Melis, M. T., Zinzi, A., and Giommi, P. (2016). Mapping landslides in lunar impact craters using Chebyshev polynomials and DEM’S. Comm. VI 41, 17–24. doi:10.5194/isprsarchives-XLI-B6-17-2016
Keywords: mass-movements, landslide, Moon, literature review, surface erosion
Citation: Li K, Wang J and Shi Y (2023) A review of studies on mass-movements on the Moon. Front. Astron. Space Sci. 10:1223642. doi: 10.3389/fspas.2023.1223642
Received: 16 May 2023; Accepted: 30 June 2023;
Published: 21 July 2023.
Edited by:
Josep M. Trigo-Rodríguez, Spanish National Research Council (CSIC), SpainReviewed by:
Akos Kereszturi, Hungarian Academy of Sciences (MTA), HungaryJianguo Yan, Wuhan University, China
Copyright © 2023 Li, Wang and Shi. This is an open-access article distributed under the terms of the Creative Commons Attribution License (CC BY). The use, distribution or reproduction in other forums is permitted, provided the original author(s) and the copyright owner(s) are credited and that the original publication in this journal is cited, in accordance with accepted academic practice. No use, distribution or reproduction is permitted which does not comply with these terms.
*Correspondence: Jiao Wang, amlhb3dhbmdAY3VnYi5lZHUuY24=