- 1Lockheed Martin Advanced Technology Center, Palo Alto, CA, United States
- 2Southwest Research Institute, San Antonio, TX, United States
- 3Johns Hopkins Applied Physics Laboratory, Laurel, MD, United States
- 4California Institute of Technology, Pasadena, CA, United States
- 5Universidad de Alcalá, Space Research Group, Alcalá de Henares, Spain
- 6School of Earth and Space Sciences, Peking University, Beijing, China
- 7Physics and Astronomy Department, George Mason University, Fairfax, VA, United States
- 8NASA Goddard Space Flight Center, Greenbelt, MD, United States
A series of 3He-rich solar energetic particle (SEP) events was observed by Solar Orbiter in May 2021 at a radial distance of 0.95 AU. An isolated active region AR 12824 was likely the ultimate source of these SEP events. The period of the enhanced flux of 3He was also a period of frequent type III bursts in the decametric-hectometric range, confirming their close relationship. As in past studies, we try to find the solar activities possibly responsible for 3He-rich SEP events, using the type III bursts close to the particle injection times estimated from the velocity dispersion. But this exercise is not as straightforward as in many of the past studies since the region produced many more type III bursts and jet-like eruptions than the SEP injections. We may generalize the solar activities for the 3He-rich SEP events in question as coronal jets, but their appearances do not necessarily conform to classic jets that consist of a footpoint and a spire. Conversely, such jets often did not accompany type III bursts. The areas that produced jet-like eruptions changed within the active region from the first to the second set of 3He-rich SEP events, which may be related to the extended coronal mass ejection that launched stealthily.
1 Introduction
A defining attribute of the so-called impulsive solar energetic particle (SEP) events is anomalies in ionic composition, especially the enhancement of the 3He/4He ratio by up to 104 of the typical values in the corona and solar wind. Since their discovery many decades ago (e.g., Schaeffer and Zähringer, 1962; Hsieh and Simpson, 1970), “3He-rich flares” puzzled many workers because most of them showed either no or very weak flare emissions (Kahler et al., 1987; Reames et al., 1988), even though they were associated with type III radio bursts below 2 MHz (Reames and Stone, 1986) and in situ electron events observed in the 10–100 keV range (Reames et al., 1985)1. To avoid possible confusion that the word “impulsive” may incur, we call this type of events “3He-rich” SEP events throughout the paper. A new insight was obtained by Kahler et al. (2001), who found the association of 3He-rich SEP events with narrow coronal mass ejections (CMEs) observed by the Large Angle Spectroscopic Coronagraph (Brueckner et al., 1995) on board the Solar and Heliospheric Observatory (SOHO). Some of these CMEs started off as coronal jets observed by the Extreme-Ultraviolet Imaging Telescope (Delaboudinière et al., 1995) also on board SOHO, as was found by Wang et al. (2006), who showed for the first time that 3He-rich SEP events were temporally correlated with recurrent jets in coronal hole boundaries.
Since the Wang et al. (2006) paper, it has been proclaimed that coronal jets are responsible for 3He-rich SEP events, even though the original paper primarily discussed the importance of the interaction between the base of an open field line and a nearby bipole. Jets have been emphasized in some of the review articles on 3He-rich SEP events (e.g., Mason, 2007; Bučík, 2020; Reames, 2021). It is not difficult to find clear examples of jets in EUV or soft X-rays temporally correlated with 3He-rich SEP events (Nitta et al., 2006, 2008; 2015; Wang et al., 2006; Bučík et al., 2014, 2018). However, these individual cases may not ensure that jets are a necessary condition for 3He-rich SEP events. Indeed, in ensemble studies, the association rate of 3He-rich SEP events with jets is not necessarily high, only up to ∼50% (Nitta et al., 2006, 2015; Bučík et al., 2021). We may attribute the failure to capture jets by EIT to its limitation in spatial resolution, sensitivity, cadence and temperature coverage. However, we may not necessarily observe jets around the estimated injection times of 3He particles even in data from the Atmospheric Imaging Assembly (Lemen et al., 2012) on board the Solar Dynamics Observatory (SDO). The other forms of solar activities associated with 3He-rich SEP events may include compact brightenings, eruptions more extended than a jet, EUV waves, etc. (e.g., Nitta et al., 2015; Bučík et al., 2016, 2021; Bučík, 2022).
In this paper we study 3He-rich SEP events observed by Solar Orbiter in May 2021 (Ho et al., 2022) to further our understanding of the solar sources of this type of SEP event. Ho et al. (2022) focused on the “dropout” phenomenon (Giacalone et al., 2000; Mazur et al., 2000; Chollet and Giacalone, 2008) that was observed during the period in which six clear ion injections were identified. Our emphasis is on solar activities observed by AIA during the same period. Unlike many previous examples of isolated 3He-rich SEP events, we note multiple type III bursts and jet-like activities around the estimated times of ion injections, and their associations with 3He-rich SEP events may be more complicated. After giving an overview of the series of 3He-rich SEP events in Section 2, we describe in Section 3 solar activities that may be related to 3He-rich SEP events, using snapshots from movies that reveal them in detail. Some of the movies are included in Supplementary Material. In Section 4 we summarize what we learn from this study concerning the origin of 3He-rich SEP events in terms of jets. While we mention solar activities or transients, we specifically look for those that have appearances and properties in common with coronal jets. Coronal jets are ubiquitous phenomena and are actively investigated (see recent reviews by Raouafi et al., 2016; Shen, 2021), irrespective of their possible roles in the production of 3He-rich SEP events. We note, however, that there is no universal definition of coronal jets, quantitatively speaking, and that identifying them is somewhat subjective.
2 Overview of the 21–24 May 2021 period
In late May 2021, Solar Orbiter observed a series of 3He-rich SEP events (Ho et al., 2022). Figure 1 combines a decametric-hectometric (DH) radio dynamic spectrum (Figure 1A) from the Radio and Plasma Wave Investigation on the STEREO Mission (Bougeret et al., 2008) on board STEREO-A (hereafter SWAVES-A) with Solar Orbiter data [Figures 1B–F, reproduced from Ho et al. (2022)]. Figures 1B–E show SEP data from the Energetic Particle Detector (Rodríguez-Pacheco et al., 2020) and (Figure 1F) the interplanetary magnetic field (IMF) in RTN coordinates from the Solar Orbiter magnetometer (Horbury et al., 2020).
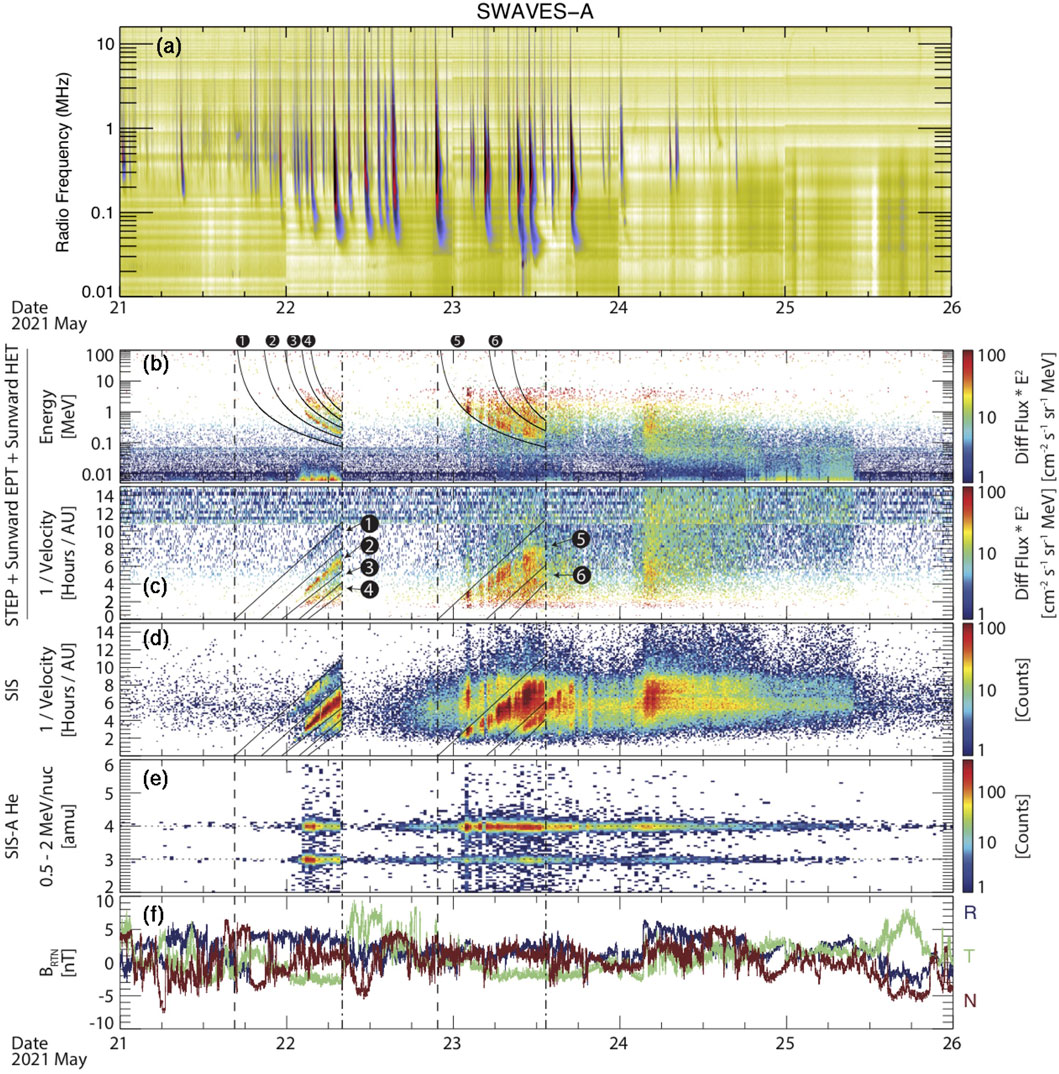
FIGURE 1. Radio, energetic particle and interplanetary magnetic field for the 5-day period starting 21 May 2021. (A) Radio dynamic spectra from SWAVES-A. (B, C) The total ion energy and the total ion inverse velocity from STEP, EPT and HET. (D) The inverse velocity of heavy ions as measured by SIS. (E) The mass spectrogram for helium isotopes from SIS. (F) The interplanetary magnetic field (IMF) in RTN coordinates from MAG. Of the four vertical lines in panels (B–F), which are reproduced from Ho et al. (2022), the first and third (dashed) mark the estimated times of injections 1 and 5, and the second and fourth (dashed-dot) the dropouts that ended the first and second sets of 3He-rich SEP events.
Concerning SEP data, Figures 1B–C show, respectively, the total energy and inverse velocity of ions (mostly protons) in the 10 keV–100 MeV range, as measured by the three Sunward-facing sensors of EPD, i.e., the SupraThermal Electrons and Protons (STEP), the Electron Proton Telescope (EPT), and the High- Energy Telescope (HET). The ion composition measurements Figures 1D–E come from the Suprathermal Ion Spectrograph (SIS), another component of EPD, showing the inverse velocity of heavy ions (Figure 1D) and the mass spectrogram for helium isotopes (Figure 1E). Ho et al. (2022) identified six ion events during 21–23 May, with the first four grouped into the first set, followed by the last two in the second set after a long dropout. They are numbered Figures 1B–C. They are all 3He-rich impulsive SEP events, according to the helium ion masses Figure 1E. They occurred in a period of enhanced type III radio activity, confirming their close relationship.
On 22 May 2021, Solar Orbiter was located at ∼0.95 AU from the Sun and
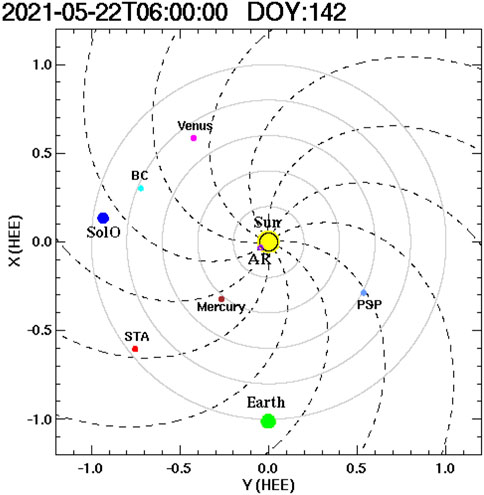
FIGURE 2. The location of Solar Orbiter on 22 May 2021 with respect to the Sun and Earth. Other observatories and planets are also included. The rough position of AR 12824 is indicated as “AR”. Dashed curves are the nominal Parker Spiral for the assumed constant solar wind velocity of 400 km s −1.
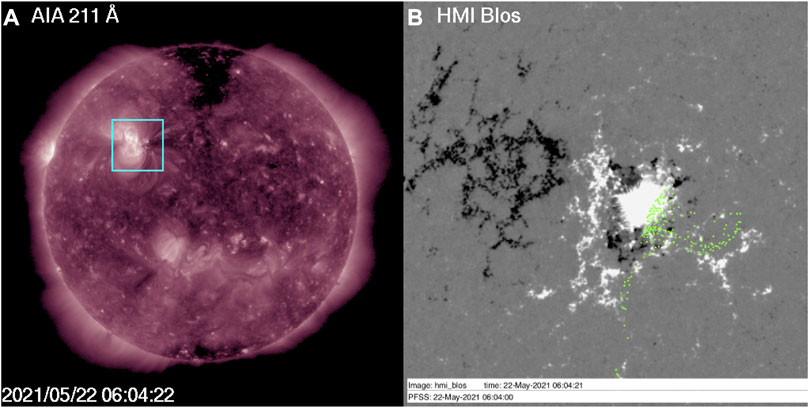
FIGURE 3. (A) AIA 211 Å image on 22 May 2021. AR 12824 was within the cyan box. (B) HMI line-of-sight magnetogram of the region marked by the cyan box in (A). Green dots show the footpoints of open field lines as calculated using the PFSS model.
3 Observations
In order to identify the solar activities that likely contributed to the production of 3He particles during 21–23 May 2021, we follow the procedure used in previous studies (Nitta et al., 2006, 2015). That is, to find a type III burst in radio dynamic spectra around the estimated time of particle injection and then to search for transient phenomena in EUV images within a few minutes after the start of the type III burst. We base this exercise on the six particle injection times listed in Ho et al. (2022). In addition, since AR 12824 exhibits so many type III bursts and jets, we also address a broader question outside the SEP context about which type IIIs are associated with a jet, and which jets are associated with a type III burst. This may in turn help clarify whether jets are a necessary condition for 3He-rich SEP events.
Most of the findings in this paper come from closely comparing AIA images with radio dynamic spectra from SWAVES-A. We use SWAVES-A 1-min data because they allow us to easily plot DH radio dynamic spectra, on which type III radio bursts are identified by visual inspection. Similar data from WAVES on Wind had a ∼17 h long data gap on 22 May 2021 while the 3He/4He ratio was elevated (Figure 1E). We produced movies of AIA images in 24 s cadence (just excluding those with the automatic exposure control on) over a 3-day period starting at 09 UT on 21 May 2021. This period covers the six particle injections (Ho et al., 2022) with a 0.5–1 day margin before and after. A snapshot from one of the movies is shown in Figure 4. Above the AIA image taken at time t, a SWAVES-A dynamic spectrum is shown over the interval of [t-1.5 h, t+1.5 h]. Viewing frames in this layout as a movie, we can study, in reasonable detail, the association of type III bursts and transient activities including jets over a long period (e.g., 3 days). The upper panel also shows the GOES 1–8 Å light curve (white) and the time profile of 40–102 keV electrons from EPT (cyan) that inform us about how the type III bursts involve solar flares and electron events, respectively. Figure 4 is a snapshot from the movie of 211 Å full-disk running difference images. The red arrow point to a small jet barely noticeable. The purpose of this movie is to find whether a given type III burst is correlated with a transient activity within AR 12824. It turns out that almost all the type III bursts during our 3-day period were from AR 12824.
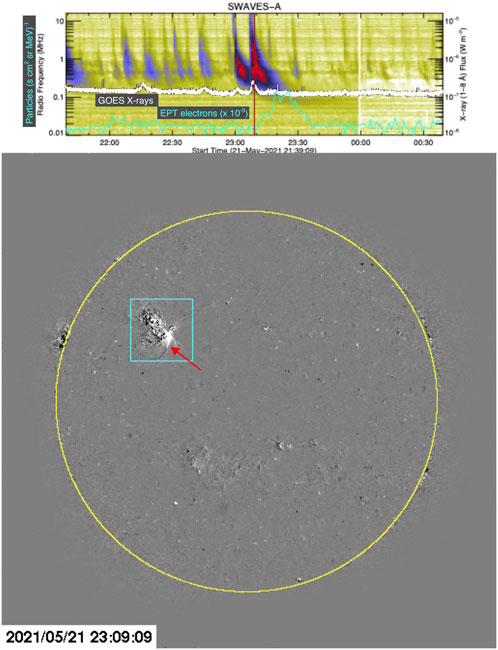
FIGURE 4. Snapshot from a movie of AIA 211 Å full-disk running-difference images taken at the time indicated by a brown line in the upper panel that shows radio dynamic spectra in the ±1.5 h interval. The red arrow points to a jet. The yellow circle indicates the solar disk. The upper panel also includes the GOES soft X-ray (1–8 Å light curve (white) and the 40–102 keV electron time profile as recorded (cyan). The electron flux (particles cm2 sr MeV)−1 is multiplied by 2 × 10−4 to be scaled with the y-axis on the left.
To isolate transient activities more clearly, we zoom in on the active region, bounded by the cyan box in Figures 3A, 4. Moreover, we examine AIA images in multiple channels to avoid missing jets in case they may appear in a narrow temperature range. As in the snapshot shown in Figure 5, however, most jets are observed in all of the AIA 304 Å (0.089 MK), 171 Å (0.79 MK), and 211 Å (1.78 MK) channels, and their appearances are similar across these channels. The full-length movies of these images are included as Supplementary Videos S1–S3. They show that many typical jets consisting of a footpoint brightening and a spire that delineates a collimated outflow (e.g., Mulay et al., 2016; Panesar et al., 2016) are ejected toward south and west. Ejections toward northwest are often more extended than a classic jet and may be classified as surges. In order to inform the magnetic origins of these transients, Figure 6, a snapshot from Supplementary Video S4, shows an AIA 211 Å image over a line-of-sight magnetogram from HMI. The jet-like activities such as the one indicated by the red arrows in Figure 6, are related to small magnetic elements around the leading sunspot of AR 12824, which look like moving magnetic features (MMFs) (see Harvey and Harvey, 1973; Shine and Title, 2000; Innes et al., 2016).
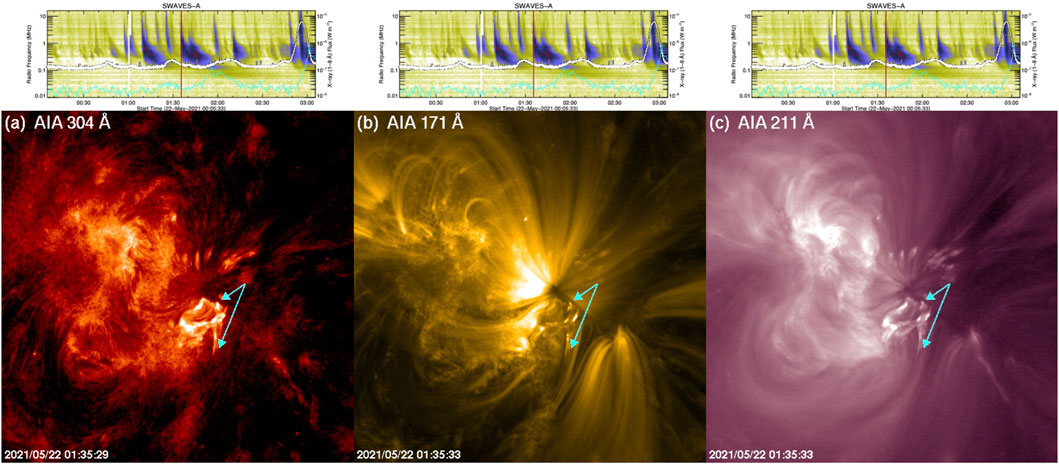
FIGURE 5. A jet captured in AIA images of AR 12824 in 304 Å, 171 Å, and 211 Å channels, respectively, in (A–C). These are snapshots from movies (images and radio dynamic spectra) in the respective AIA channels. The arrows point to the footpoint and spire of the jet. The full-length movies are included as Supplementary Videos S1–S3.
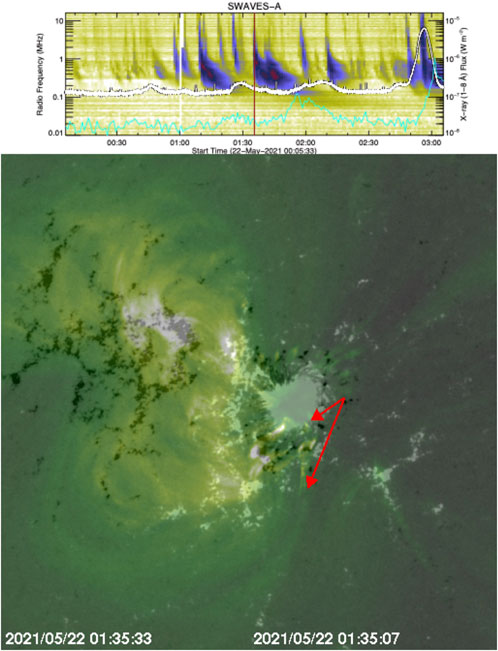
FIGURE 6. Snapshot from a movie of AIA 211 Å images of AR 12824 on top of HMI line-of-sight magnetograms. The red arrows point to the footpoint and spire of the jet. The full-length movie is included as Supplementary Video S4.
Now we discuss the candidates of solar activity that may be related to the series of 3He-rich SEP events during 21–23 May 2021. See Table 1 in Ho et al. (2022) for their estimated injection times and other important properties such as 3He/4He ratios. We hereafter refer to these individual SEP events as Event 1–Event 6, whose candidate sources are illustrated in Figures 7A–F. For Event 1, we cannot readily find a candidate that accounts for the estimated injection time of 21 May 16:05. However, the first major type III burst at 18:52 should be the best option despite the long delay. It appears that the velocity dispersion for this injection may not be approximated by a straight line, and that the estimated injection time could be off by a few hours. This type III burst was correlated with a jet-like eruption toward south with a marginal spire (Figure 7A). For event 2, the estimated injection time is 21 May 22:00. Two successive jets and type III bursts at 21 May 22:59 and 23:08 seem to be a good candidate. As seen in Figure 7B, their locations are close to that of the earlier (18:52) event. Moreover, the 23:08 event is accompanied by an electron event. The estimated injection times for Event 3 and Event 4 are 22 May 00:40 and 02:00, respectively. We find two type III bursts that are not too much apart from these times and are accompanied by electron events. They occur at 22 May 01:09 and 01:35 (Figures 7C, D). They again correspond to jets emanating from the southwestern periphery of the leading sunspot of AR 12824 and directed south. Another type III burst at 22 May 02:55 (also a C6.1 flare) may be in the time range to be considered for Event 4, especially since it is accompanied by an electron event. But it is linked to a global EUV wave, which is usually not associated with 3He-rich SEP events (but see, for example, Nitta et al., 2015; Bučík et al., 2015, 2016; Bučík, 2022). Events 5 and 6 occur after a long dropout. Their estimated injection times are 22 May 20:50 and 23 May 04:20. The most promising type III bursts, especially because of the accompanying electron events, are the ones at 22 May 21:32 and 23 May 04:36, respectively (Figures 7E, F). They occur at the northwestern to western perimeter of the leading sunspot. Note that Event 5 appears to be more spatially extended and it may be classified as a surge. It is also an M1.4 flare. Incidentally, the baseline of the electron time profile shifted upward by an order of magnitude after the type III burst and C1.3 flare on 22 May around 06:50 UT. Further discussion of this phenomenon is beyond the scope of this work and will be explored in future papers.
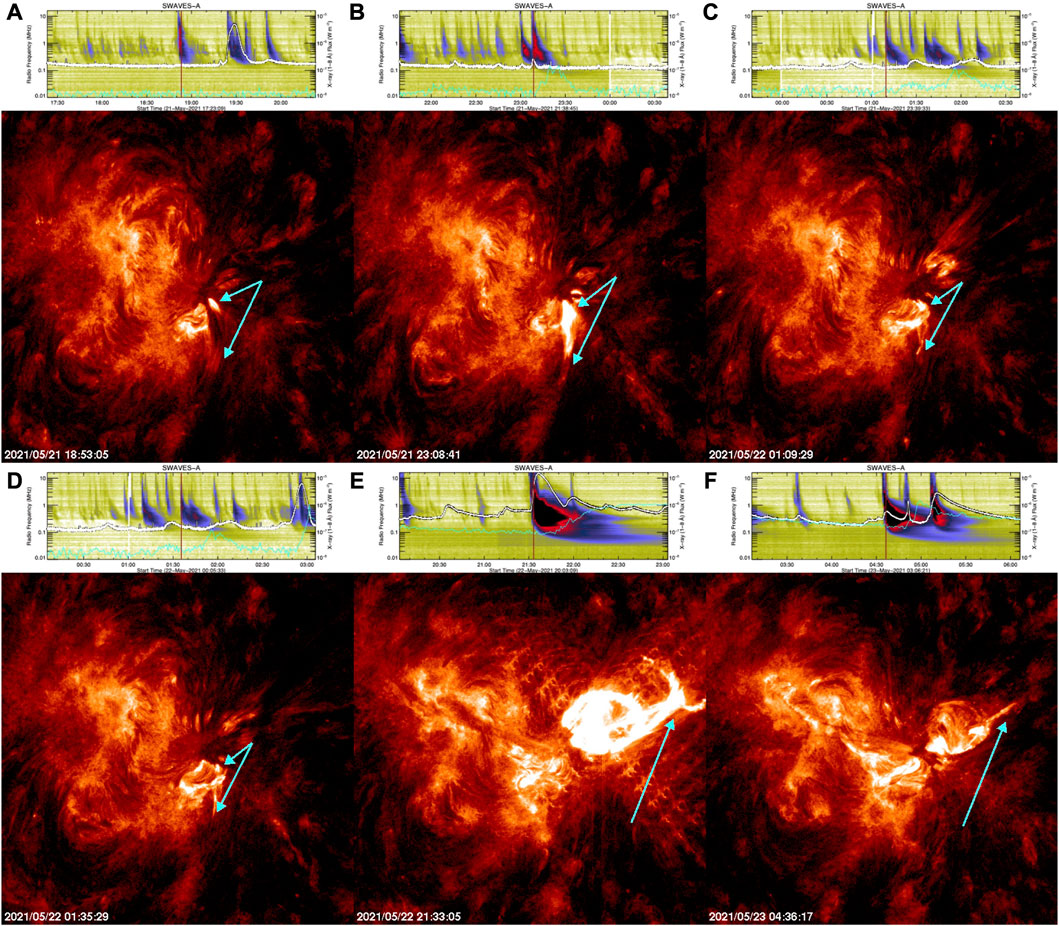
FIGURE 7. Jet-like eruptions around the times of type III bursts. In (A–D), two arrows point to the footpoint and spire. In (E,F), the arrow points to the spire or the upper part of the ejections. These eruptions likely contributed to the six 3He-rich SEP events.
AR 12824 produced a number of EUV waves, starting on 17 May 2021 as documented in https://aia.lmsal.com/AIA_Waves. Several events were observed on 17 and 18 May, but not on 19–20 May. They almost always followed the pattern of starting as a jet-like narrow ejection, followed by a large wave-like feature once the jet material hit a remote quiescent region that was apparently magnetically connected. During the 3-day period starting 21 May 2021 09:00, there were at least 12 EUV waves from AR 12824 as shown in Figure 8. There were two types. One type started as a jet or a surge and gave rise to an EUV wave only at large distances, similar to those observed during 17–18 May. Several of the fat jets or surges belong to this type (Figures 8D,F,G,I–L). They are always associated with strong type III bursts and could also be with 3He-rich SEP events. The event Figure 8I is the same event shown in Figure 7E. Another type started as an outward motion of large-scale closed loops. These events may or may not be associated with a type III burst and probably not related to 3He-rich SEP events. Note that none of these EUV waves are associated with a clear CME observed by LASCO. This may be partly because of the location of AR 12824, which may prevent a narrow CME from being observed. Interestingly, the most prominent CME from this region (Figure 9) occurred without obvious low-coronal signatures like a stealth CME (Robbrecht et al., 2009; D’Huys et al., 2014; Nitta and Mulligan, 2017) on 22 May, shortly before the diffuse EUV wave shown in Figure 8E. There was no clear eruption, but the GOES X-ray flux slowly built up. In Figure 9B we note emission like a two-ribbon flare on the eastern side of the leading sunspot and the plage region in the following polarity. This CME may be related to the dropout even though its direct cause appears to be variations of IMF (∼08 UT on 22 May, see Figure 1F). It may have altered the magnetic field conneciton to Solar Orbiter.
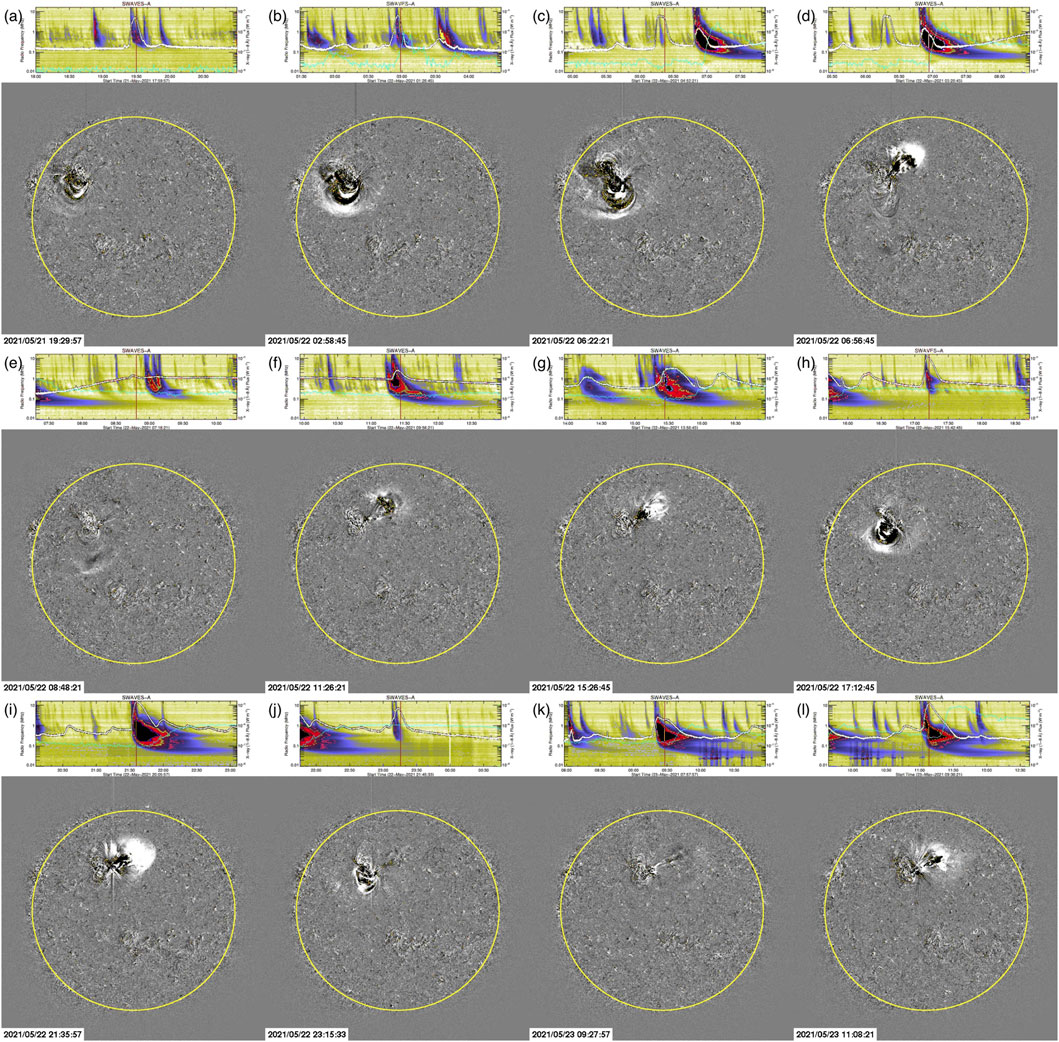
FIGURE 8. AIA 211 Å running difference images showing EUV waves that occurred in AR 12824 during 21–23 May 2021. The yellow circles indicate the solar disk. In (D,F,G,I–L), the EUV wave started as a jet. In others, the wave started as an outward motion of large-scale closed loops (see text).
4 Discussion
A series of 3He-rich SEP events occurred in late May 2021 and were observed by Solar Orbiter (Ho et al., 2022). They provide us an opportunity to reevaluate the widely proclaimed link between 3He-rich SEP events and coronal jets (Wang et al., 2006; Mason, 2007; Bučík, 2020; Reames, 2021). In this period there was only one active region (AR 12824) that could have been connected to Solar Orbiter, which in principle makes the correlative study easier because solar transients from this region would have ultimately been responsible for the production of 3He. The six 3He-rich SEP events generally show clear velocity dispersions, suggestive of their separate injections in the solar corona. This is somewhat less clear in Event 1. As in previous studies, type III bursts are used to limit the time range in which we look for solar transients in AIA images. This may be justified because the period of elevated 3He/4He ratio coincides with frequent type III bursts, except for the long “dropout” period (roughly 08–22 UT on 22 May, see Figure 1). The challenge pertaining to this particular period is to single out the solar transient for each of the 3He-rich SEP events because multiple jet-like eruptions are seen within one or 2 minutes from the type III burst, coming from different areas around the sunspot, in the range of 180° (south)–30° (northwest) from solar north. Even if we find the candidate events as in Figure 7, we cannot rule out the possibility that other jets close to the SEP injection time, irrespective of their type III association, may have contributed to the observed 3He. The videos of images in 24 s cadence, included in Supplementary Material, capture many instances of jet-like eruptions within a minute or two, which would have been not possible in images taken with poorer temporal resolution.
The solar transients temporally correlated with type III bursts show various appearances, as found in Supplementary Videos S1–S3, whose snapshots are shown in Figure 5. Specifically, it is hard to isolate jets from other forms of activity including compact brightenings, wider eruptions, and EUV waves. Out of the 118 type III bursts during the 3-day period starting at 21 May 2021 09:00 UT (which include the six that are associated with 3He-rich SEP events), only 63 (∼53%) may be classified as classic jets. But brightenings (∼19%) may be generalized to be jets, as some unspecified observational conditions may make it hard to find the spire. Whether to identify wider eruptions (∼28%) with jets may be a matter of subjective decision. Despite extensive studies of jets (see recent reviews by Raouafi et al., 2016; Shen, 2021), there seems to be no universal definition of jets that can be used for their automated detection. Indeed, SDO initially had a plan to automatically detect and characterize jets (Martens et al., 2012), but it has not been implemented in the AIA data pipeline. Jets (and many other phenomena) included in the Heliophysics Event Knowledgebase (Hurlburt et al., 2012)2 have been detected manually by scientists via visual inspection.
Conversely, many classic jets that consist of a footpoint and spire do not appear correlated with a type III burst. This may simply reflect the limited sensitivity of the radio instruments, but SWAVES-A data show many marginal type III bursts, including those that appear repeatedly in limited time intervals and in limited frequency ranges. A more likely reason may be the lack of non-thermal electron beams escaping along open field lines. Following the detection of hard X-ray emission from the base of a jet by Yohkoh (Nitta, 1997), RHESSI observed many examples of jets with hard X-ray emission even from the corona, some of which was determined to be of non-thermal origin (Bain and Fletcher, 2009; Krucker et al., 2011; Glesener et al., 2012; Musset et al., 2020). But these may be a small subset of jets. We also point out that type III bursts are characteristically missing from jets that come from polar coronal holes, such as those studied by Sterling et al. (2015, 2022).
It is important to know which jet-like solar transients are more likely associated with 3He-rich SEP events and how they may affect the properties of the latter. Unfortunately, it is hard to distinguish the jets associated with 3He-rich SEP events in terms of their basic properties including size, speed, etc. In our examples, Events 1 – 4 are associated with small jets from the southern perimeter of the leading spot, whereas Events 5–6 with eruptions larger than typical jets that are ejected from an area northwest of the spot. The projected lengths of the jets excluding the footpoints are
Data availability statement
SDO data are available at JSOC (http://jsoc.stanford.edu/). STEREO/WAVES data are downloaded from the STEREO Science Center (https://stereo-ssc.nascom.nasa.gov/). The Solar Orbiter data are available at https://soar.esac.esa.int/soar/.
Author contributions
NN processed AIA and SWAVES-A data. RB and LW encouraged the lead author to write a paper for the topical volume. GM first notified the lead author of the SEP events in question, and further updated SEP analysis. GH provided SEP information including his paper on the same events before official publication. CC helped with interpretation of SEP data. RG-H provided electron data. LB discussed with the lead author about the effect of image cadence on the appearance of transient phenomena.
Funding
The work of NN was supported by NASA grants 80NSSC18K1126 and 80NSSC20K0287. RB acknowledges support from a NASA grant 80NSSC22K0757. CC was supported by NASA grants 80NSSC18K0223 and 80NSSC21K1512. RG-H acknowledges the financial support of the Spanish MINCIN Project PID2019-104863RBI00/AEI/10.13039/501100011033.
Acknowledgments
We acknowledge the International Space Science Institute (ISSI) for their support of International Team “Origins of 3He-Rich Solar Energetic Particles” (https://www.issibern.ch/teams/origin3henrgpart/).
Conflict of interest
Author NN was employed by Lockheed Martin Advanced Technology Center.
The remaining authors declare that the research was conducted in the absence of any commercial or financial relationships that could be construed as a potential conflict of interest.
Publisher’s note
All claims expressed in this article are solely those of the authors and do not necessarily represent those of their affiliated organizations, or those of the publisher, the editors and the reviewers. Any product that may be evaluated in this article, or claim that may be made by its manufacturer, is not guaranteed or endorsed by the publisher.
Supplementary material
The Supplementary Material for this article can be found online at: https://www.frontiersin.org/articles/10.3389/fspas.2023.1148467/full#supplementary-material
Footnotes
1Note that, although near-relativistic electron events are statistically correlated with the periods of 3He enhancement (Wang et al., 2012), individual electron events may not be (e.g., Agueda et al., 2014)
References
Agueda, N., Klein, K. L., Vilmer, N., Rodríguez-Gasén, R., Malandraki, O. E., Papaioannou, A., et al. (2014). Release timescales of solar energetic particles in the low corona. Astron. Astrophys. 570, A5. doi:10.1051/0004-6361/201423549
Bain, H. M., and Fletcher, L. (2009). Hard X-ray emission from a flare-related jet. Astron. Astrophys. 508, 1443–1452. doi:10.1051/0004-6361/200911876
Bougeret, J. L., Goetz, K., Kaiser, M. L., Bale, S. D., Kellogg, P. J., Maksimovic, M., et al. (2008). S/WAVES: The radio and Plasma wave investigation on the STEREO mission. Space Sci. Rev. 136, 487–528. doi:10.1007/s11214-007-9298-8
Brueckner, G. E., Howard, R. A., Koomen, M. J., Korendyke, C. M., Michels, D. J., Moses, J. D., et al. (1995). The large Angle spectroscopic Coronagraph (LASCO). Sol. Phys. 162, 357–402. doi:10.1007/BF00733434
Bučík, R. (2020). 3He-Rich solar energetic particles: Solar sources. Space Sci. Rev. 216, 24. doi:10.1007/s11214-020-00650-5
Bučík, R. (2022). Impulsive solar energetic particle events: Extreme-ultraviolet waves and jets. Front. Astronomy Space Sci. 8, 248. doi:10.3389/fspas.2021.807961
Bučík, R., Innes, D. E., Guo, L., Mason, G. M., and Wiedenbeck, M. E. (2015). Observations of EUV waves in 3He-rich solar energetic particle events. Astrophys. J. 812, 53. doi:10.1088/0004-637X/812/1/53
Bučík, R., Innes, D. E., Mall, U., Korth, A., Mason, G. M., and Gómez-Herrero, R. (2014). Multi-spacecraft observations of recurrent 3He-rich solar energetic particles. Astrophys. J. 786, 71. doi:10.1088/0004-637X/786/1/71
Bučík, R., Innes, D. E., Mason, G. M., and Wiedenbeck, M. E. (2016). Association of 3He-rich solar energetic particles with large-scale coronal waves. Astrophys. J. 833, 63. doi:10.3847/1538-4357/833/1/63
Bučík, R., Innes, D. E., Mason, G. M., Wiedenbeck, M. E., Gómez-Herrero, R., and Nitta, N. V. (2018). 3He-rich solar energetic particles in helical jets on the sun. Astrophys. J. 852, 76. doi:10.3847/1538-4357/aa9d8f
Bučík, R., Mason, G. M., Gómez-Herrero, R., Lario, D., Balmaceda, L., Nitta, N. V., et al. (2021). The long period of 3He-rich solar energetic particles measured by Solar Orbiter 2020 November 17-23. Astron. Astrophys. 656, L11. doi:10.1051/0004-6361/202141009
Chollet, E. E., and Giacalone, J. (2008). Multispacecraft analysis of energetic ion flux dropouts. Astrophys. J. 688, 1368–1373. doi:10.1086/592378
Delaboudinière, J. P., Artzner, G. E., Brunaud, J., Gabriel, A. H., Hochedez, J. F., Millier, F., et al. (1995). Eit: Extreme-ultraviolet imaging telescope for the SOHO mission. Sol. Phys. 162, 291–312. doi:10.1007/BF00733432
D’Huys, E., Seaton, D. B., Poedts, S., and Berghmans, D. (2014). Observational characteristics of coronal mass ejections without low-coronal signatures. Astrophys. J. 795, 49. doi:10.1088/0004-637X/795/1/49
Giacalone, J., Jokipii, J. R., and Mazur, J. E. (2000). Small-scale gradients and large-scale diffusion of charged particles in the heliospheric magnetic field. Astrophys. J. Lett. 532, L75–L78. doi:10.1086/312564
Glesener, L., Krucker, S., and Lin, R. P. (2012). Hard X-ray observations of a jet and accelerated electrons in the corona. Astrophys. J. 754, 9. doi:10.1088/0004-637X/754/1/9
Harvey, K., and Harvey, J. (1973). Observations of moving magnetic features near sunspots. Sol. Phys. 28, 61–71. doi:10.1007/BF00152912
Ho, G. C., Mason, G. M., Allen, R. C., Wimmer-Schweingruber, R. F., Rodríguez-Pacheco, J., and Gómez-Herrero, R. (2022). Interplanetary ion flux dropouts across multiple 3He-rich events. Front. Astronomy Space Sci. 9, 939799. doi:10.3389/fspas.2022.939799
Horbury, T. S., O’Brien, H., Carrasco Blazquez, I., Bendyk, M., Brown, P., Hudson, R., et al. (2020). The solar orbiter magnetometer. Astron. Astrophys. 642, A9. doi:10.1051/0004-6361/201937257
Hsieh, K. C., and Simpson, J. A. (1970). The relative abundances and energy spectra of 3He and 4He from solar flares. Astrophys. J. Lett. 162, L191. doi:10.1086/180652
Hurlburt, N., Cheung, M., Schrijver, C., Chang, L., Freeland, S., Green, S., et al. (2012). Heliophysics event Knowledgebase for the solar dynamics observatory (SDO) and beyond. Sol. Phys. 275, 67–78. doi:10.1007/s11207-010-9624-2
Innes, D. E., Bučík, R., Guo, L. J., and Nitta, N. (2016). Observations of solar X-ray and EUV jets and their related phenomena. Astron. Nachrichten 337, 1024–1032. doi:10.1002/asna.201612428
Kahler, S. W., Lin, R. P., Reames, D. V., Stone, R. G., and Liggett, M. (1987). Characteristics of solar coronal source regions producing 3He-rich particle events. Sol. Phys. 107, 385–394. doi:10.1007/BF00152032
Kahler, S. W., Reames, D. V., and Sheeley, N. R. (2001). Coronal mass ejections associated with impulsive solar energetic particle events. Astrophys. J. 562, 558–565. doi:10.1086/323847
Krucker, S., Kontar, E. P., Christe, S., Glesener, L., and Lin, R. P. (2011). Electron acceleration associated with solar jets. Astrophys. J. 742, 82. doi:10.1088/0004-637X/742/2/82
Lemen, J. R., Title, A. M., Akin, D. J., Boerner, P. F., Chou, C., Drake, J. F., et al. (2012). The atmospheric imaging assembly (AIA) on the solar dynamics observatory (SDO). Sol. Phys. 275, 17–40. doi:10.1007/s11207-011-9776-8
Madjarska, M. S. (2019). Coronal bright points. Living Rev. Sol. Phys. 16, 2. doi:10.1007/s41116-019-0018-8
Martens, P. C. H., Attrill, G. D. R., Davey, A. R., Engell, A., Farid, S., Grigis, P. C., et al. (2012). Computer vision for the solar dynamics observatory (SDO). Sol. Phys. 275, 79–113. doi:10.1007/s11207-010-9697-y
Mason, G. M. (2007). 3He-Rich solar energetic particle events. Space Sci. Rev. 130, 231–242. doi:10.1007/s11214-007-9156-8
Masson, S., Antiochos, S. K., and DeVore, C. R. (2013). A model for the escape of solar-flare-accelerated particles. Astrophys. J. 771, 82. doi:10.1088/0004-637X/771/2/82
Mazur, J. E., Mason, G. M., Dwyer, J. R., Giacalone, J., Jokipii, J. R., and Stone, E. C. (2000). Interplanetary magnetic field line mixing deduced from impulsive solar flare particles. Astrophys. J. Lett. 532, L79–L82. doi:10.1086/312561
Mulay, S. M., Tripathi, D., Del Zanna, G., and Mason, H. (2016). Multiwavelength study of 20 jets that emanate from the periphery of active regions. Astron. Astrophys. 589, A79. doi:10.1051/0004-6361/201527473
Musset, S., Jeunon, M., and Glesener, L. (2020). Statistical study of hard X-ray emitting electrons associated with flare-related coronal jets. Astrophys. J. 889, 183. doi:10.3847/1538-4357/ab6222
Nitta, N. (1997). Hard X-ray emission from active region transient brightenings. Astrophys. J. 491, 402–408. doi:10.1086/304938
Nitta, N. V., Mason, G. M., Wang, L., Cohen, C. M. S., and Wiedenbeck, M. E. (2015). Solar sources of 3He-rich solar energetic particle events in solar cycle 24. Astrophys. J. 806, 235. doi:10.1088/0004-637X/806/2/235
Nitta, N. V., Mason, G. M., Wiedenbeck, M. E., Cohen, C. M. S., Krucker, S., Hannah, I. G., et al. (2008). Coronal jet observed by hinode as the source of a3He-rich solar energetic particle event. Astrophys. J. Lett. 675, L125–L128. doi:10.1086/533438
Nitta, N. V., and Mulligan, T. (2017). Earth-affecting coronal mass ejections without obvious low coronal signatures. Sol. Phys. 292, 125. doi:10.1007/s11207-017-1147-7
Nitta, N. V., Reames, D. V., De Rosa, M. L., Liu, Y., Yashiro, S., and Gopalswamy, N. (2006). Solar sources of impulsive solar energetic particle events and their magnetic field connection to the Earth. Astrophys. J. 650, 438–450. doi:10.1086/507442
Panesar, N. K., Sterling, A. C., Moore, R. L., and Chakrapani, P. (2016). Magnetic flux cancelation as the trigger of solar quiet-region coronal jets. Astrophys. J. Lett. 832, L7. doi:10.3847/2041-8205/832/1/L7
Raouafi, N. E., Patsourakos, S., Pariat, E., Young, P. R., Sterling, A. C., Savcheva, A., et al. (2016). Solar coronal jets: Observations, theory, and modeling. Space Sci. Rev. 201, 1–53. doi:10.1007/s11214-016-0260-5
Reames, D. V., Dennis, B. R., Stone, R. G., and Lin, R. P. (1988). X-ray and radio properties of solar (He-3) rich events. Astrophys. J. 327, 998. doi:10.1086/166257
Reames, D. V. (2021). Fifty years of 3 He-rich events. Front. Astronomy Space Sci. 8, 164. doi:10.3389/fspas.2021.760261
Reames, D. V., and Stone, R. G. (1986). The identification of solar He-3-rich events and the study of particle acceleration at the sun. Astrophys. J. 308, 902. doi:10.1086/164560
Reames, D. V., von Rosenvinge, T. T., and Lin, R. P. (1985). Solar He-3-rich events and nonrelativistic electron events - a new association. Astrophys. J. 292, 716–724. doi:10.1086/163203
Robbrecht, E., Patsourakos, S., and Vourlidas, A. (2009). No trace left behind: STEREO observation of a coronal mass ejection without low coronal signatures. Astrophys. J. 701, 283–291. doi:10.1088/0004-637X/701/1/283
Rodríguez-Pacheco, J., Wimmer-Schweingruber, R. F., Mason, G. M., Ho, G. C., Sánchez-Prieto, S., Prieto, M., et al. (2020). The energetic particle detector. Energetic particle instrument suite for the solar orbiter mission. Astron. Astrophys. 642, A7. doi:10.1051/0004-6361/201935287
Rouillard, A. P., Pinto, R. F., Vourlidas, A., De Groof, A., Thompson, W. T., Bemporad, A., et al. (2020). Models and data analysis tools for the Solar Orbiter mission. Astron. Astrophys. 642, A2. doi:10.1051/0004-6361/201935305
Schaeffer, O. A., and Zähringer, J. (1962). Solar flare helium in satellite materials. Phys. Rev. Lett. 8, 389–390. doi:10.1103/PhysRevLett.8.389
Scherrer, P. H., Schou, J., Bush, R. I., Kosovichev, A. G., Bogart, R. S., Hoeksema, J. T., et al. (2012). The helioseismic and magnetic imager (HMI) investigation for the solar dynamics observatory (SDO). Sol. Phys. 275, 207–227. doi:10.1007/s11207-011-9834-2
Schrijver, C. J., and De Rosa, M. L. (2003). Photospheric and heliospheric magnetic fields. Sol. Phys. 212, 165–200. doi:10.1023/A:1022908504100
Shen, Y. (2021). Observation and modelling of solar jets. Proc. R. Soc. Lond. Ser. A 477, 20200217. doi:10.1098/rspa.2020.0217
Shine, R., and Title, A. (2000). “Sunspots: Moving magnetic features and moat flow,” in Encyclopedia of Astronomy and astrophysics. Editor P. Murdin (UK: Nature Physics).
Sterling, A. C., Moore, R. L., Falconer, D. A., and Adams, M. (2015). Small-scale filament eruptions as the driver of X-ray jets in solar coronal holes. Nature 523, 437–440. doi:10.1038/nature14556
Sterling, A. C., Moore, R. L., and Panesar, N. K. (2022). Another look at erupting minifilaments at the base of solar X-ray polar coronal “standard” and “blowout” jets. Astrophys. J. 927, 127. doi:10.3847/1538-4357/ac473f
Wang, L., Lin, R. P., Krucker, S., and Mason, G. M. (2012). A statistical study of solar electron events over one solar cycle. Astrophys. J. 759, 69. doi:10.1088/0004-637X/759/1/69
Wang, Y. M., Pick, M., and Mason, G. M. (2006). Coronal holes, jets, and the origin of 3He-rich particle events. Astrophys. J. 639, 495–509. doi:10.1086/499355
Keywords: Sun, solar energetic particles, radio emission, UV radiation, solar jets, elemental abundances
Citation: Nitta NV, Bučík R, Mason GM, Ho GC, Cohen CMS, Gómez-Herrero R, Wang L and Balmaceda LA (2023) Solar activities associated with 3He-rich solar energetic particle events observed by Solar Orbiter. Front. Astron. Space Sci. 10:1148467. doi: 10.3389/fspas.2023.1148467
Received: 20 January 2023; Accepted: 14 February 2023;
Published: 14 March 2023.
Edited by:
Olga V. Khabarova, Tel Aviv University, IsraelReviewed by:
Alexander Nindos, University of Ioannina, GreeceSusan Samwel, National Research Institute of Astronomy and Geophysics, Egypt
Copyright © 2023 Nitta, Bučík, Mason, Ho, Cohen, Gómez-Herrero, Wang and Balmaceda. This is an open-access article distributed under the terms of the Creative Commons Attribution License (CC BY). The use, distribution or reproduction in other forums is permitted, provided the original author(s) and the copyright owner(s) are credited and that the original publication in this journal is cited, in accordance with accepted academic practice. No use, distribution or reproduction is permitted which does not comply with these terms.
*Correspondence: Nariaki V. Nitta , bml0dGFAbG1zYWwuY29t