- Laboratory for Atmospheric and Space Physics, University of Colorado, Boulder, CO, United States
We present the demographics data for the space physics workforce which are compared with other space sciences fields, physics, plus science and engineering in general. We focus on the early stages of college, and draw some lessons from looking beyond the US by discussing this in the context of physics degrees awarded in different countries. We review some of the studies from the National Academies, extracting some relevant recommendations. Studies of the science, technology, engineering and mathematics (STEM) workforce, the physical sciences profession, and specifically the space sciences show that the “pinch point” where the demographics narrow down is at the high school to college stages. We considered the actions that could be made nationally by federal agencies, locally by an institution or individually to enhance and diversify the career pathway through the space sciences.
Introduction
Many studies have shown that multiple forms of diversity in a workforce enhance creativity and productivity (Hong and Page, 2004; Campbell et al., 2013; Freeman and Huang, 2014a; Freeman and Huang, 2014b). NASA Science Plan 2020 states,
“As research has shown, diversity is a key driver of innovation and more diverse organizations are more innovative. . . We will increase support by actively encouraging students and early career researchers. . . . We will also increase partnerships across institutions to provide additional opportunities for engagement and increasing diversity of thought. NASA believes in the importance of diverse and inclusive teams to tackle strategic problems and maximize scientific return.”
A national focus on diversity, equity, inclusivity and accessibility (DEIA) over the past few years has nudged institutions to look at the demographics of their workforce and find quick ways to “fix” discrepancies. However studies of the science, technology, engineering and mathematics (STEM) workforce, the physical sciences profession, and specifically the space sciences show that the “pinch point” where the demographics narrow down is at the high school to college stages (Advancing Diversity, Equity, Inclusion, and Accessibility in the Leadership of Competed Space Missions (2022), hereafter National Academies of Sciences, Engineering, and Medicine, 2022a). This means that the changes need to be made within the education system (i.e., basically, at the state and/or county level in the US) and the impact on the demographics of senior levels of the profession will likely take many years.
The goal of this paper is to pull together the various studies and reports and present a summary for people who are new to the topic but would like to know where to find out more. We are providing the “CliffNotes” for the topic of demographics and career pathways in the field of space physics. In this article we first present the demographics of the space physics profession, addressing gender, race/ethnicity and current trends. We then compare the demographics of space physics with other space sciences (planetary, astrophysics, Earth science), as well as internationally. We next summarize the findings and recommendations of various reports, mostly from the National Research Council (NRC) or the National Academies of Science, Engineering, and Medicine(NASEM). Finally, we summarize some of the research on how STEM education at the college could be improved to retain a more diverse population of STEM degrees. Our conclusions are listed from immediate actions that could be taken locally to longer-term, institutional and/or national policy approaches.
We simplify references to the three Decadal Surveys carried out by NRC or NASEM as follows: Solar and Space Physics: A Science for a Technological Society is called SSP 2013; Pathways to Discovery in Astronomy and Astrophysics for the 2020s becomes Astro 2021; Origins, Worlds, and Life: A Decadal Strategy for Planetary Science and Astrobiology 2023–2032 is shortened to Planet 2023.
First the numbers
Surveying the workforce in solar and space physics is not as straightforward as it might seem at first. We belong to different professional organizations (AGU, AAS, APS, etc.), attend different conferences, get funding from different agencies (NASA, NSF, NOAA, etc.) and work at a range of types of institutions (agency labs/centers, universities, non-profits, industry, etc.). It is a similar story for planetary science and for astrophysics. Yet, to study the state of the profession for a Decadal Survey, each area needs to know the demographics of the appropriate workforce.
Luckily, there is an umbrella organization—the American Institute of Physics (AIP)—that has a Statistical Research Center with staff who are experienced in carrying out surveys. The AIP was sponsored by NSF to carry out the 2011 Survey of Solar, Space and Upper Atmospheric Physicists (White et al., 2011a). Moreover the AIP keeps a tally of physics departments across the US and tracks the number of faculty and degrees (bachelor’s, masters and doctorates) being awarded.
At the same time, the landscape of how demographics are described is changing. Terms to describe gender, race and ethnicity are evolving as society begins to recognize that identity can be more complicated than thought a decade or so ago. Nevertheless, looking at the numbers, albeit simplified, can guide policies and programs to enhance the diversity of the field.
Space physics workforce
In preparation for the 2013 Solar and Space Physics Decadal Survey process, the Education and Workforce Working Group developed a survey of the profession. The survey was implemented by the American Institute of Physics and funded by the National Science Foundation (NSF). The survey request was sent to 2560 unique email addresses gathered from various professional groups: AGU’s Space Physics and Aeronomy Section (SPA, the largest group, with 1,792 unique names); AAS’s Solar Physics Division (SPD); Space Weather Week conference attendee lists, and NSF PI lists. The survey received 1305 responses (51% response rate), of which 1171 indicated that they considered themselves in the field of Solar, Space and Upper Atmospheric Physics (SSUAP) and currently work and reside in the US. If one makes the assumption (probably crude) that the 51% response rate applies across the board, then one can estimate that the profession comprises a total of approximately 1171/0.51–2300 space physicists in the US.
Figure 1 summarizes the basic demographics of this SSUAP population. In 2011 the gender split was 83% men, 17% women. When considering race/ethnicity it is useful to compare the respondents who obtained their doctorate degrees before vs after 2000. The percentage of White respondents drops from 83% with earlier doctorates to 69% with later doctorates. Meanwhile, the percentage of Asian or Asian Americans rose from 12% to 24%. The percentages of Black or African American and Hispanic or Latino respondents made small increases in percentage of doctorates from 1% to 2% and 1%–3% respectively.
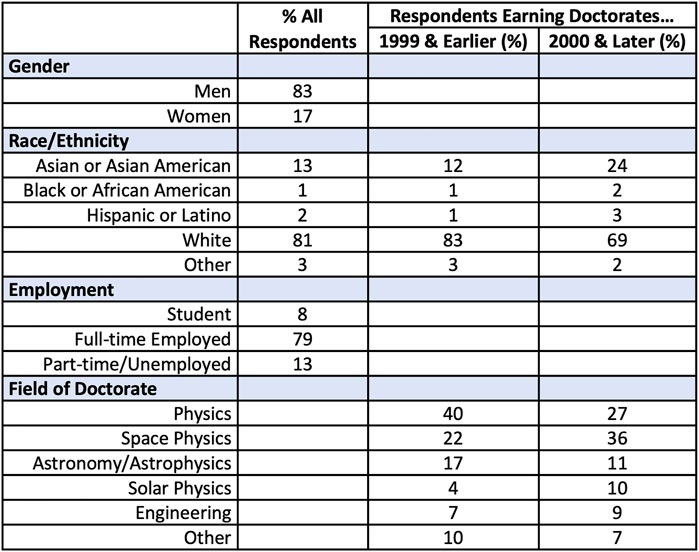
FIGURE 1. Results from the 2011 Survey of Solar, Space and Upper Atmospheric Physicists (White et al., 2011a). Responses were received from 1,171 individuals who resided in the US at the time of their response.
A few decades ago most people in the SSUAP fields came through graduate programs in physics departments. Physics is still the most common (62% of respondents) undergraduate degree. Figure 1 shows that since 2000, more doctorates were in the specialized fields of Space Physics and of Solar Physics. While AIP keeps track of numbers of doctorate degrees in physics, it is hard to keep track of trends in sub-fields unless surveys are repeated to specific areas such as SSUAP. A more effective approach would be to make SandSP a formal dissertation research area or subfield in the NSF Annual Survey of Earned Doctorates. This would provide tracking of PhD production and data to assess the health of graduate programs. (See National Science Foundation, Survey of Earned Doctorates, available at http://www.nsf.gov/statistics/srvydoctorates/).
Education and workforce issues
The SSP2013 Decadal Survey included an Education and Workforce Working Group that evaluated not just the 2011 SSUAP Workforce Survey (White et al., 2011a) but also carried out separate studies of such things as PhD production rate and the job market (via job advertisements) over the previous 10 years (see Appendix D of the SSP2013 report).
Figure 2 (left) shows the trends in PhD production in solar and space physics (in Canada and the US) as well as the number of job advertisements for post-doctoral, research scientist and faculty positions (from Moldwin et al., 2013). The Moldwin et al. (2013) study showed a total of “475 PhDs were produced at 76 different institutions. The top 10 institutions produced 238 (or 50 percent) of the total. Thirty of the 76 institutions produced only 1 solar and space physics PhD during the decade.” Prior to 2007 about ∼5 PhDs per year were produced in the sub-fields of Heliophysics, Space Plasmas and Solar Physics, while ∼12 PhDs per year were produced in Magnetospheres and Ionosphere-Thermosphere-Magnetosphere Coupling (ITM). Between 2007 and 2010 the number of ITM degrees shot up to ∼25 while Solar Physics increased by about a factor of 2.
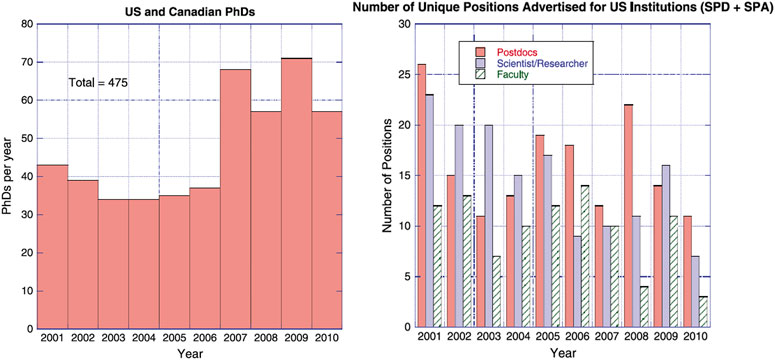
FIGURE 2. Total PhDs in solar and space physics (2001–2010) and number of unique positions advertized (via solar and space physics newsletters) for US institutions at levels of postdoc, research scientist and faculty. (Moldwin et al., 2013).
The right side of Figure 2 shows the number of unique positions advertised for US institutions in the AGU-SPA and AAS-SPD newsletters. These numbers were gathered in a study by Moldwin et al. (2013) who reported “the field—though small—is vibrant and growing. However, there is concern that the number of positions, especially faculty positions, is not keeping track with the growth of the field. Continuation of the NSF Faculty Development in the Space Sciences program would directly address this concern”.
The big question is what has happened in past decade? Have the trends shown in the two plots of Figure 2 persisted or changed? These are questions for the Status of the Profession Panel of the next Decadal Survey.
Comparisons with other space sciences
For the recent NASEM study on Advancing Diversity, Equity, Inclusion, and Accessibility in the Leadership of Competed Space Missions (2022), hereafter National Academies of Sciences, Engineering, and Medicine, 2022a, demographic data were compiled for four of the divisions of NASA’s Science Mission Directorate (SMD): Heliophysics, Astrophysics, Planetary Science and Earth Sciences. (The fifth division, Biological and Physical Sciences, was not included because it does not generate satellite missions, the focus of the study). Figure 3 shows that workforce data are pretty similar across these four science divisions and illustrates how these fields are predominantly white and male. Heliophysics has a notably low percentage of women (17%), half the representation in Earth and in Planetary Sciences. When considering race/ethnicity, all four divisions have similarly very low percentages of non-White populations.
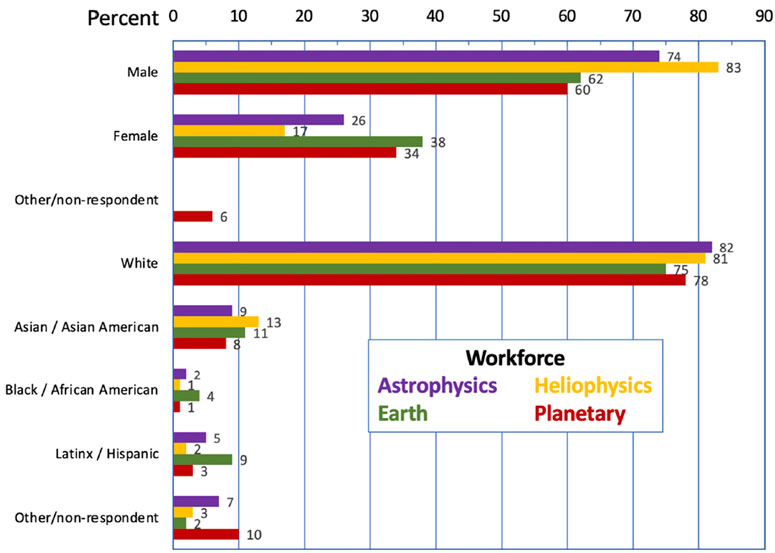
FIGURE 3. Demographics of the space science research workforce (PhD scientists working in the US) derived from workforce surveys. Based on data from Bernard and Cooperdock (2018), National Science Foundation National Center for Science and Engineering Statistics, Pold and Ivie (2018), Porter and Ivie (2019), Porter et al. (2020), and White et al. (2011a).
Figure 4 compares gender demographics data for these four NASA divisions with (left) the percentage of women shown in workforce data across US, for all STEM fields and specifically in physical sciences. On the right the data for the four divisions come from the workforce surveys (Figure 3), all research proposal submissions through NASA’s online NSPIRES platform, and proposed missions with women Principal Investigators (PIs). The involvement of women in Planetary and Earth Sciences is comparable to the overall STEM workforce (39%), while Astrophysics and Heliophysics workforces (perhaps not surprisingly) are comparable to the national workforce in physical science (23%), as are all four percentages of women submitting research proposals to NSPIRES. When it comes to women PIs submitting mission proposals, only Planetary Science has a percentage comparable the national physical science workforce while the other 3 divisions have much lower (5%–8%) representation. Clearly, the workforce of these four NASA SMD divisions have a way to go to reach gender parity.
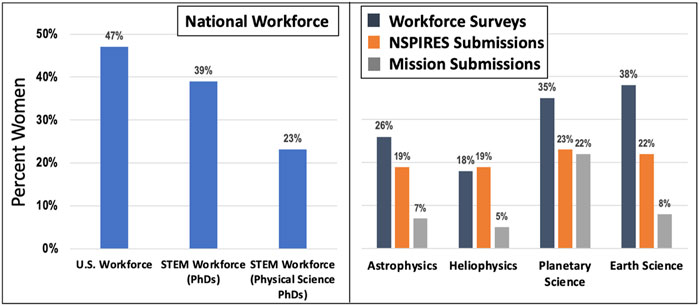
FIGURE 4. Gender statistics of competed mission team leaders compared with the workforce of corresponding NASA SMD divisions and the U.S. STEM workforce overall. Female percentage of populations: PI of submitted mission proposals, PIs and Co-Is of NSPIRES proposals, workforce for SMD science fields, U.S. STEM workforce (Physical Science PhDs), U.S. STEM workforce (PhDs in any field), total U.S. workforce. Based on data from Bernard and Cooperdock (2018), National Science Foundation (2019), Pold and Ivie (2018), Porter and Ivie (2019), Porter et al. (2020), and White et al. (2011a),White et al. (2011b).
Education and career pathway
As discussed in the National Academies of Sciences, Engineering, and Medicine, 2022a Advancing DEIA study, as well as the Decadal Surveys, the “pinch point” in the diversity of careers in the physical sciences comes at the high school to college stages, well before graduate school and doctorate degrees. Focusing on physics (the primary subject underlying most of space sciences), analysis of AIP data by Hodapp and Hazari (2015) shows in Figure 5 how at high school the participation of women in physics classes is close to parity with men. The participation plummets to ∼20% at college entrance. While the total numbers along the career path to professor decrease drastically, the participation of women remains around the ∼20% level, suggesting the career path is not differentially leaky for women.
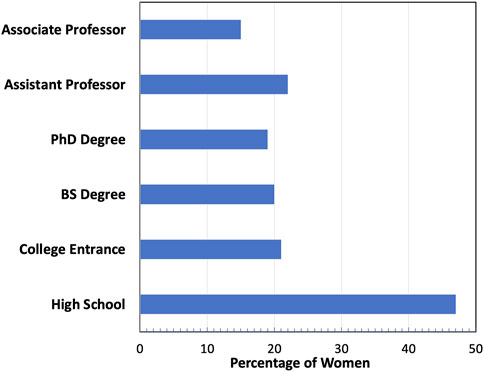
FIGURE 5. Percentage of participating physicists that are women at various academic stages. College entrance refers to first-year student’s intent to major in the field. Based on Hodapp and Hazari (2015).
This major drop of the participation early in college by women-and racial/ethnic minorities-is consistent with studies of when and why students switch out of STEM majors, as discussed at length in the 1997 book Talking About Leaving: Why Undergraduates Leave the Sciences (Seymour and Hewitt, 1997). The TAL study illustrates the “iceberg effect” that many of the issues that caused student to switch out of classes were also experienced by the students who persisted, emphasizing that these issues need to be addressed even if departments are willing to let a significant fraction of students switch their major. Twenty-four years later, the follow-on 2019 book Talking About Leaving Revisited: Persistence, Relocation, and Loss in Undergraduate STEM Education (Seymour and Hunter, 2019) found, through many interviews of students and teachers at six different universities, that the same factors continued to affect students’ decisions to switch majors. Briefly stated, these factors are:
• Poor teaching in introductory math, physics, chemistry classes (e.g., disorganized, disinterested, poor delivery of content (“chalk talk”), unwilling to help, etc.). Across persisting and switching students, 78% expressed frustration with poor teaching.
• “Weed-out” culture (e.g., high workload, fast pace, tough grading on a curve, little support, etc.)
• Loss of interest in STEM major, discovery of interest outside STEM
• Inadequate math preparation at high school
Additional factors emerging in the second book TALR were:
• Competitive class climate
• Financial difficulties
While more women than men switched majors, the difference was much reduced over 24 years (perhaps partly related to changes in culture and parental support). For students of color, given greater emphasis in TALR, the primary issues were poor high school preparation, difficulties making the transition to college, the competitive nature of STEM classes, and being discouraged by getting low grades. We return to these issues and their potential remedies in a later section.
Degree data
Meanwhile, let us consider how the total numbers and demographics of physics bachelor degrees have varied over time. In the top of Figure 6 we show total numbers and below is the percentage awarded to women, Hispanic Americans and African Americans. The total number of bachelor’s degrees (summing all subjects) has risen steadily since the 50 s from ¼ million to just over 2 million. By contrast, the number of physics degrees oscillated around ∼4500 ± 20% for about 50 years and then proceeded to climb, at a faster rate than the All Bachelor’s slope, to a peak of ∼9300 in 2019 (the 2020 and 2021 numbers may be affected by COVID). The lowest curve on the top plot shows the total number of women getting bachelor’s degrees in physics, steadily rising from 1980 to 2020, but not as steeply as the men or total. The net result of the flatter curve for the women means that fraction of physics bachelor’s degrees awarded to women actually dropped between ∼2000 and ∼2013 before kicking back up again. At the same time the percentage of undergraduate physics degrees awarded to Hispanic Americans has been steadily rising over the past 30 years, reaching nearly 10% (still below the overall percentage in the US population of 19%). The number of African Americans decreased over most of the past 30 years (∼5%–3%), perhaps slowly rising back to 4%, well below the 12.5% of the US population.
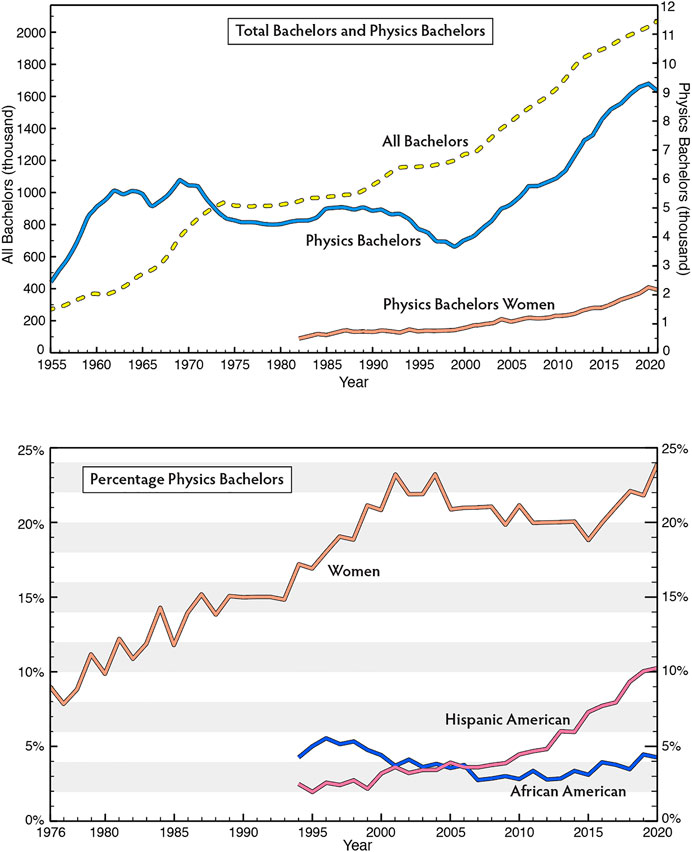
FIGURE 6. Top: Number of bachelor’s degrees (all topics) and physics bachelor’s degrees (1955–2021). Bottom: Percentage of Bachelor’s degrees in physics awarded to women, to Hispanic Americans and African Americans. Produced using data from the Statistical Research Center of the American Institute of Physics, see https://www.aip.org/statistics.
Turning to graduate students, Figure 7 shows the total numbers of physics degrees between 1975 and 2020. The peak number of about 1900 doctorates (in 2018) corresponds to a little under 1 in 5 of the 9300 physics bachelor’s degrees. However Figure 7 also shows that since the mid-90 s about half of the physics doctorates have been awarded to people born outside the US (while only ∼20% of physics bachelor’s degrees were awarded to non-US students). This means that only about 1 in 7440/950 = 7.8 of US students getting physics bachelor’s degrees went on to get PhDs in physics. The total number of doctorates awarded to Black/African Americans has hovered around 10–20 while the number of Hispanic/Latinx physics doctorates has increased over the past decade from ∼15 to over 40. Note that these numbers are far below their representation in the US population as a whole which would currently be 143 (14% in US) for Black/African Americans and 195 (19%) for Hispanic/Latinx.
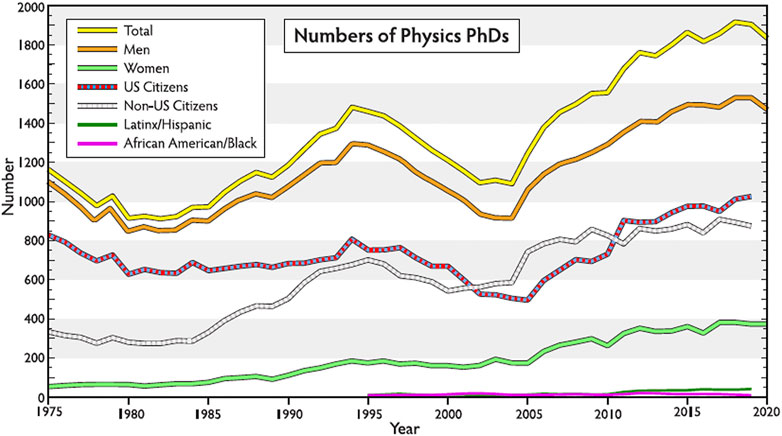
FIGURE 7. Trends in Physics disaggregated by gender and citizenship status as well as race/ethnicity. Produced using data from the Statistical Research Center of the American Institute of Physics, see https://www.aip.org/statistics.
The story with women getting physics doctorates may be more complicated. The total numbers have been increasing steadily over the past 45 years up to an annual rate of ∼400 per year (about 21% of the total). But, as we see in the next section, a disproportionate number of these may be non-US.
International comparisons
Obtaining numbers for physics degrees in the US is hard enough—but getting numbers for other countries is even harder. Ivie and Guo (2006) reported data on degrees awarded to women in about twenty countries for 1999 and 2000 shown in various ways in Figure 8. First (top) we show the total numbers of physics bachelor and doctorate degrees. Readers may not be surprised to see the US produce the highest numbers. But when these numbers are plotted per million people of each country’s total population, the US slips way down the league table of per capita degree production. Even more surprising is the plot of percentage of physics degrees awarded to women. These data are now 2 decades old and the current numbers could be quite different. But the data get one thinking about possible reasons why each country sits where it is in these plots. What are the cultures and policies that drive high/low per capita production of physics degrees or high/low percentage awarded to women? Are a significant number of women getting physics doctorates in the US coming from the countries that award a higher fraction of their bachelor degrees to women?
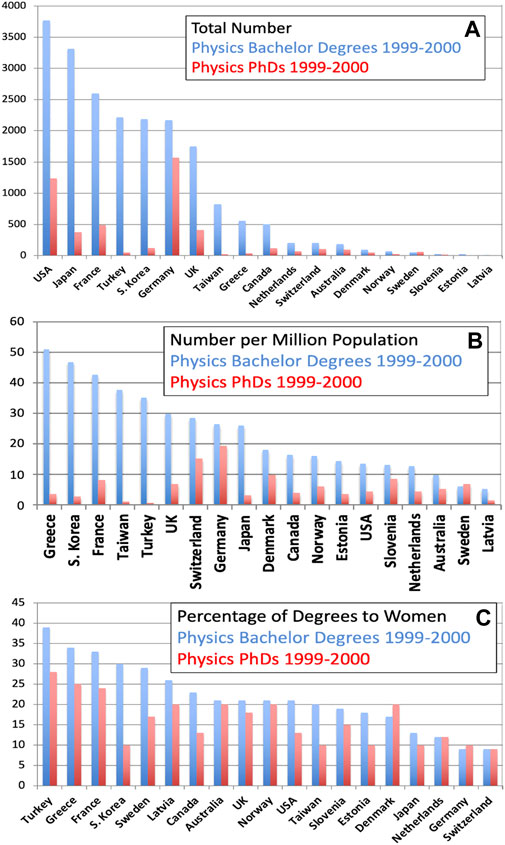
FIGURE 8. Physics degrees awarded (averaged over 2 years 1999–2000) for 19 countries. (A) Total number; (B) per million people in each country’s population; (C) percentage to women. Based on Ivie and Guo (2006) which says “To be included, countries had to provide appropriate data from reliable statistical agencies”.
Comparing the US career paths (Figure 5; Figure 6; Figure 7) with demographics from a United Kingdom survey by the Royal Astronomical Society, 2017 (published in 2017 and summarized by Massey et al., 2017) we see that the participation of women in Solar System science in the United Kingdom is similar to the US while in astronomy there is a steeper drop with rank. Students pursuing PhDs in the United Kingdom have similarly exceptionally poor non-white representation. While the permanent staff and graduate students were largely British (73% and 69% respectively), only 48% of post-docs were from the United Kingdom. The 33% of post-docs from the European Union (EU) may drop following the British withdrawal from the EU.
The international data from Ivie and Guo (2006) are limited in their coverage. Their paper says “To be included, countries had to provide appropriate data from reliable statistical agencies”. A couple countries that are notably absent in Figure 8 are India and China. In fact, over the past 20 years, science and engineering education in China and India have taken off (see top plot in Figure 9). Moreover, China’s expenditure in RandD is also sharply rising (see bottom plot in Figure 9). The three factors of i) US’s low per capita production of bachelor degrees in STEM degrees; ii) the high fraction of US doctorates awarded to students born outside the US; and iii) the steep rise of production of STEM doctorates outside the US, has caught policymakers’ attention. The 2022 report on The State of US Science and Engineering: Science and Engineering Indicators from the National Science Board (2022) notes:
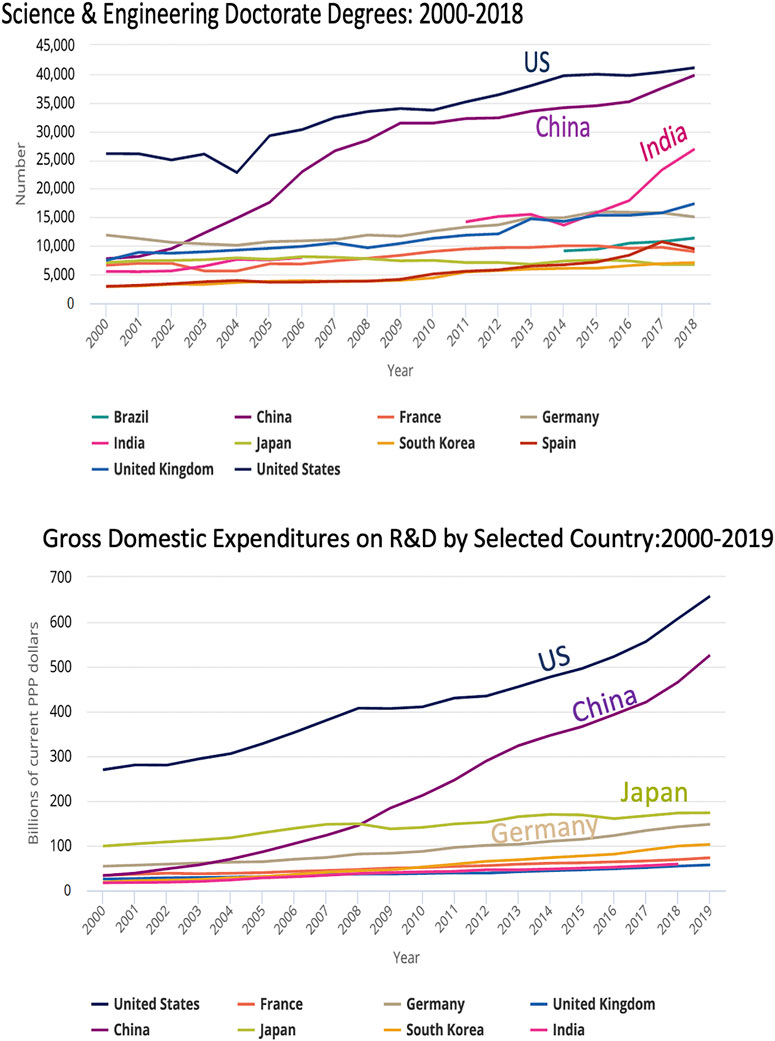
FIGURE 9. Top: Number of science and engineering degrees awarded to 10 countries. Bottom: Gross expenditures on science and engineering RandD. From National Science Board (2022).
“The STEM workforce relies heavily on foreign-born individuals, who account for about one-fifth of the STEM workforce (and higher proportions in certain fields). Among foreign-born STEM workers with an SandE degree, about 50% are from Asia, with most from India or China.”
They also show a chart that illustrates the US share of world-wide RandD expenditure decreasing between 2000 and 2019 from 36% to 28%. Over the same period, the share of China increased from 4% to 22%. Hence, a key takeaway of the report is:
“The global concentration of RandD performance continues to shift from the United States and Europe to countries in East-Southeast Asia and South Asia.”
A report from the American Academy of Arts and Sciences in 2020, The Perils of Complacency: America at a Tipping Point in Science and Engineering is even blunter. One of their key messages is:
“The United States is in severe danger of no longer being the premier destination for SandE talent. An increasingly unwelcome environment for foreign talent, together with a failure to cultivate an adequate domestic SandE workforce, threatens a decline in American health, prosperity, and national security.”
This report (from a committee co-chaired by Norm Augustine and Neal Lane), including many graphs, presents the case that:
“The United States is today at a “tipping point” with regard to its ability to compete globally. Decisions made in the next few years will determine what kind of country America will leave to future generations. A decision to compete will require a renewed commitment to enhancing the four essential elements of American innovation: human capital, knowledge capital, an ecosystem that promotes innovation, and financial capital.”
These reports present the workforce situation. Next we consider what actions they recommend be taken to change things.
Remedies
While the studies discussed above present the issues with the workforce demographics, their recommendations also suggest remedies. Some issues need the attention of the Federal Government (hence could take some time), but there are also local actions that small groups or even individuals could take.
Report recommendations
Each NASEM study presents multiple recommendations in their reports (usually lengthy). We highlight here some of the recommendations related to workforce issues. First we consider the NASEM Decadal surveys in space sciences.
The SSP2013 recommendations were primarily focused on research but they also recommended further hands-on spaceflight experience (through sub-orbital flights and Cubesats), financial support for graduate students and expanding summer schools. The survey committee recommended implementation of a new, integrated, multiagency initiative (DRIVE—Diversify, Realize, Integrate, Venture, Educate) to develop more fully and use more effectively the many experimental and theoretical assets at NASA, NSF, and other agencies. The Educate component entails Educate, Empower, and Inspire the Next-Generation of Space Researchers. To date there have been 9 Step 1 funded DRIVE projects that were down-selected to 3 currently-funded DRIVE projects.
Much of solar and space physics involves physics of plasmas. The community of plasma scientists in the US carried out a decadal survey that primarily addresses issues of funding and organization of research but they also recognize the need for enhancing education and diversity of their workforce (Plasma Science: Enabling Technology, Sustainability, Security, and Exploration, National Academies of Sciences, Engineering, and Medicine, 2021):
Recommendation: Federal agencies (e.g., DOE, NSF, NASA, DoD) should structure funding to support undergraduate and graduate educational, training, and research opportunities—including faculty—and encourage and enable access to plasma physics for diverse populations.
The Astro2021 report contained a substantial component on what they called Foundations of the Profession, including seven recommendations related to education and enhancing workforce diversity and climate, several of which gave suggested funding levels. These recommendations are to fund programs to diversify faculty and the research workforce, train undergraduate, graduate students and post-docs, as well as systematically gather demographic data and ensure that relevant organizations have policies that address harassment and discrimination. We highlight the following recommendation for which they recommend funding of $1M each by NSF, NASA and DOE:
Recommendation: NSF, NASA, and DOE should implement undergraduate and graduate “traineeship” funding…. to incentivize department/institution-level commitment to professional workforce development, and prioritize interdisciplinary training, diversity, and preparation for a variety of career outcomes.
The Planet2023 report included a chapter on the State of the Profession which also gave seven recommendations for how demographics and climate of the profession should be improved. While the Planet2023 recommendations did not include specific funding levels, the report did point out the reduction in NASA funding to the extended community for outreach activities:
While there have been benefits to centralizing public engagement in NASA’s Science Activation Program Initiatives (Erickson, 2021), education and public outreach and engagement activities by members of the community have been left unfunded. Engaging URCs at the pinch point of high school to college and providing support systems (including introductory courses) to encourage and retain them along the path of Planetary Science and Astrobiology is going to be essential to create and grow a diverse community. For example, the opportunity to propose outreach activities as an optional extension to funded RandA grants would allow grantees to make a positive impact on community diversity and inclusion activities.
Recommendation: NASA’s Planetary Science Division should regularly evaluate programs that enhance participation of students and faculty from URC’s; fellowship programs that facilitate engagement of NASA funded planetary scientists and astrobiologists with faculty at URC institutions; and mechanisms for supporting education and outreach as an integral part of research via, e.g., the inclusion of outreach activities as optional add-ons to RandA grants, or as a requirement for missions or cooperative agreements.
The National Academies of Sciences, Engineering, and Medicine, 2022a study was charged with addressing the issue of diversity of the leadership of space missions but realized that considerable effort needs to be put into early career stages. Here are a couple (of 15) recommendations that support early involvement in missions:
RECOMMENDATION 11: To engage and train diverse teams at all stages of professional talent development, NASA should offer mission-related research, mentorship, and training opportunities—ideally, integrated into actual NASA missions—through colleges/universities as well as NASA centers, that should start as early as first-year undergraduates and graduate students (e.g., internships), and extend to the ranks of postdocs (e.g., fellowships), and established scientists (e.g., participating scientists) as well as STEM initiatives centered on DEIA:
RECOMMENDATION 14: In order to ensure a vibrant, next-generation pool of excellent and diverse talent for leadership in competed space missions, NASA Science Mission Directorate, in collaboration with the Office of STEM Engagement, should provide consistent and adequate funding for STEM initiatives that are explicitly centered on diversity, equity, inclusion, and accessibility, address recruitment and retention challenges in the Earth and space sciences, and support and expand opportunities for individuals from underrepresented groups. These investments should reflect a pathways approach spanning the academic and career continuum from post-secondary through post-PhD years in order to establish flexible and robust education-to-career trajectories into the Earth and space sciences workforce, and ultimately into principal investigator–led missions. A systematic process should also be in place to document measurable impacts of these investments.
In parallel with the National Academies of Sciences, Engineering, and Medicine, 2022a study of mission leadership, NASA commissioned a study of Foundations of a Healthy and Vital Research Community for NASA Science (hereafter National Academies of Sciences, Engineering, and Medicine, 2022b) that looked into ways NASA could evaluate the impact of their space science programs on the research community. The National Academies of Sciences, Engineering, and Medicine, 2022b’s first recommendation is to gather key data to track trends in who and where gets funded:
Recommendation: NASA’s Science Mission Directorate should collect key data and trends representative of the research solicitation process and quality of the research produced by the science community. Key indicators and/or efforts.
− Metrics of participation (center, type of institution, specific institutions, partnership);
− Metrics of innovativeness and research pedigree (completeness of topics, novelty). The assessment of the quality of research and science results is likely best handled by periodic peer review;
− Review of peer review effectiveness and data sharing of other division-unique initiatives;
− A dashboard that tracks the multi-objective nature of driving science while incentivizing and supporting change; and
− Trends that capture the ratio of basic to applied research funding and foundational investment as defined in decadal recommendations.
The final recommendation involved supporting mentoring programs working with MSIs as well as with professional organizations:
Recommendation: NASA’s Science Mission Directorate (SMD) should develop a Mentor-Protégé Program for Minority Serving Institutions, including Historically Black Colleges and Universities, Hispanic Serving Institutions, Tribal Colleges and Universities, Asian American and Pacific Islander Serving Institutions, Alaska Native and Native Hawaiian Institutions, Native American Serving Non-Tribal Institutions, Predominantly Black Institutions, etc., to help them train and develop principal investigators and researchers. In addition, SMD should continue to work closely with outside professional societies—for example, the American Physical Society, the American Astronomical Society, the American Geophysical Union, etc., in development and expansion of mentoring programs. This will enable NASA to collect data and engage in longitudinal tracking of its research communities.
The National Academies’ Institute of Medicine 2007 report Rising Above the Gathering Storm: Energizing and Employing America for a Brighter Economic Future presents a bold target for enhancing the US STEM workforce as a whole and recommend these 3 actions:
Action A-1: Annually recruit 10,000 science and mathematics teachers by awarding 4-year scholarships and thereby educating 10 million minds.
Action A-2: Strengthen the skills of 250,000 teachers through training and education programs at summer institutes, in master’s programs, and in Advanced Placement (AP) and International Baccalaureate (IB) training programs.
Action A-3: Enlarge the pipeline of students who are prepared to enter college and graduate with a degree in science, engineering, or mathematics by increasing the number of students who pass AP and IB science and mathematics courses.
While these actions may seem expensive, the report shows that such actions are key for the US economy. The 2020 Perils of Complacency report follows up:
“The recommendations in the 2007 NASEM’s Gathering Storm report pertaining to pre-K–12 education should be implemented, including creating each year 10,000 federally funded four-year scholarships in STEM fields to be competitively awarded to U.S. citizens in exchange for a commitment to teach STEM in a public school for at least five years following graduation.”
Enough of these high-level viewpoints, what can we do to fix the problems locally?
Think global, act local: Undergraduate
To address the main factors that drive students to switch out of STEM majors (listed above), strategies need to be made at the departmental or institutional level. Bradforth et al. (2015) set out in a 3-page Nature some strategies from the bottom-up (for faculty) to top-down (for institutions) to enhance science education. We reproduce in Figure 10 their graphic (called a Sankey diagram) that illustrates for the University of California Davis (UCD) the flow of students from the subjects they choose on entry to their outcomes 6 years later. In contrast to other STEM disciplines like biology or social sciences, physical sciences were loosing ∼90% of students arriving at UCD with an interest in those fields (2007–2013). Drawing on Bradforth et al. (2015), the TALR book, and personal experience, here are a some strategies.
• Regularly repeat to class and individual students “You can do this. We will help you pass the class—that’s the most important thing—so you can do what you want in your career”.
• In the classroom, focus on the students—what are they thinking? This might draw attention away from “How can I deliver as much as possible as quickly as possible”.
• Training in effective teaching techniques:
1) Plug into teacher training provided by the local institution;
2) Develop a group of fellow teachers to share experiences and ideas;
3) bring in experts in Discipline-Based Education Research to give seminars;
4) Apply data tools to track when students switch vs persist (like Figure 10). Work with education or sociology departments to survey students on why they persist vs switch.
• Get the department to do a self-study to address:
1) Do we have introductory “weed-out” classes? If so, what is the goal? How do we change?
2) Could we address the impact of “grading on a curve” with other methods? Make introductory classes pass-fail?
3) Discuss how teaching is evaluated in faculty careers and ways to improve;
4) Consider hiring teaching faculty or instructors to focus on bringing evidence-based techniques to teaching the introductory classes (leaving research-focused faculty to teach the upper division classes);
5) Team with education department to make combined science + education degrees.
• Set up study areas that are friendly, safe and open in the evening (perhaps even 24–7) near the student housing, with comfortable chairs, whiteboards, coffee and music. Pay upper-division majors to be informal mentors, helping students persist through their introductory classes.
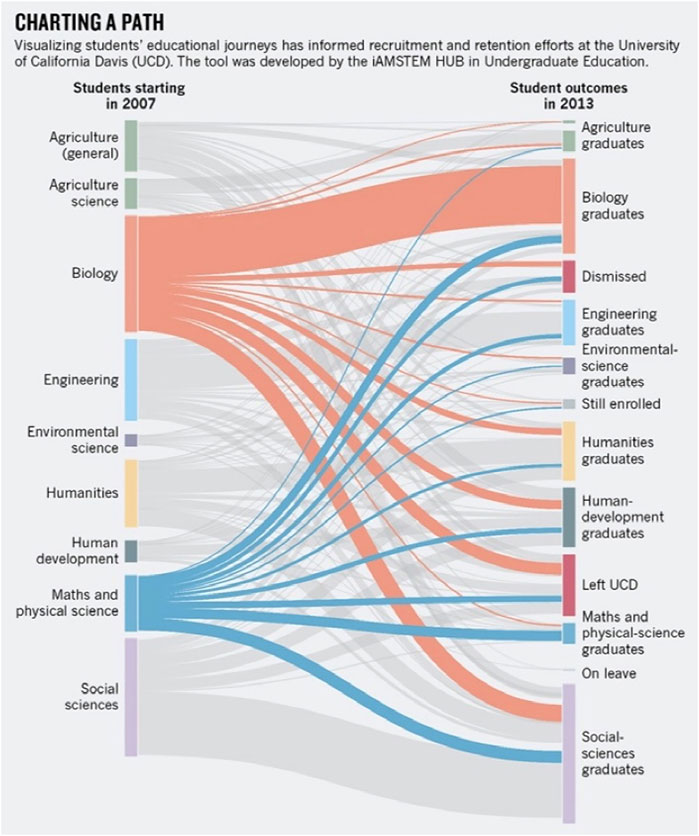
FIGURE 10. Results from a study of undergraduate degree outcomes versus incoming student interests, by field. From Bradforth et al. (2015).
If you are interested in researching how to improve undergraduate education in STEM, there’s an NSF program called Improving Undergraduate STEM Education (IUSE) under the Directorate for STEM Education which
“Supports projects to improve STEM teaching and learning for undergraduate students, including studying what works and for whom and how to transform institutions to adopt successful practices in STEM education.”
This could be an opportunity to collaborate with a local group at an education or science department with faculty and/or researchers who are involved in education research.
Undergraduate research opportunities
The 2011 USSAP workforce survey showed “a steady increase in the proportion of undergraduate students participating in scientific research outside class assignments, with over three-fourths of the recent (2000+) bachelor’s degree recipients report having done so” (White et al., 2011a). Undergraduate research experiences comprise traditional summer REUs (for groups of students) as well as individual projects, and are shown to enhance retention of STEM majors, particularly URM students (e.g., Russell et al., 2007). The National Academies of Sciences, Engineering, and Medicine, 2022a report made a finding that:
“Decades of educational research suggest that early and ongoing experiences with authentic research—experiences that engage students not only in learning about but actually doing research—is key to retaining students generally and URM students specifically.”
All three Decadal Surveys made similar findings. While NASA and NSF do fund summer REU programs (and institutions are encouraged to apply, perhaps teaming with nearby MSIs or community colleges), there is also great value in part-time research projects during the semester. Solar and Space Physics researchers are encouraged, either individually or as a coalition of colleagues, to seek support (e.g., institutional or a grant supplement) to involve undergraduates in their research, perhaps forming a collaboration with a local college. Indeed, such efforts do take time away from one’s own research, and institutions need to recognize the value of researchers getting involved in mentoring students doing authentic research.
Think global, act local: Graduate
Much less attention has been paid to graduate education, maybe because it is perceived to be less formal and the nature of a student’s graduate work is “up to the adviser”. But some relatively recent studies are worth reading. First, there are a couple books by Julie Posselt (2016, 2020) about the graduate admissions process. A major issue is that it is not straightforward to determine what are best predictors of success in graduate school. Several studies (including the Decadal Surveys; Posselt, 2014; Posselt, 2016; Posselt et al., 2017) have described how GRE scores are not reliable, particularly in developing a diverse population where other metrics are more effective. Quoting from Posselt’s 2016 book:
“For example, the Fisk-Vanderbilt Master’s-to-PhD Bridge Program, designed to provide underrepresented minority (URM) students a pathway to doctoral studies, added a question to its selection process to assess the applicants’ understanding of their own grit and resilience. Since 2004, the program has demonstrated positive results, with 81 percent of those entering the program having gone on to enter doctoral programs.”
Posselt’s 2020 book summarizes the message on graduate programs in this succinct list of actions.
Recruitment
• Ensure online presence communicates commitment to diversity, equity, inclusion
• Engage with MSIs
• Attend to cues sent at campus-visit weekend
• Timely responses to email inquiries from prospective students
• Develop a Bridge Program
• Recruit undergraduates directly from their institution as appropriate
• Pursue diversity fellowships
• Coordinate with graduate school outreach and recruitment
• Create a climate in which current students gladly serve as ambassadors
Admissions
• Downplay or eliminate GRE
• Fine-grained read of transcript (not use of cumulative GPA)
• Value research experience
• Intentionally assess non-cognitive competencies
• Emphasize potential over absolute achievements
• Contextualize achievements
• Revisit committee composition
• Discuss meanings of criteria to check misperceptions
• Develop, refine, and use rubric to systematize valuation
• Define merit in relation to what makes program distinctive
Mentoring and Support
• Individual development plans
• Develop a lab manual
• Attend to quality of relationships
• Empower staff
• Same-identity faculty available for mentoring, even if not formal advising.
• Support student organizations
• Include students in faculty decision-making
• Appoint ombudsperson or means to report harassment, bias, and/or assault
• Encourage multiple mentors
• Make support and resources for mental health explicit
Creating Conditions Conducive to Equity
• Attend to social relevance of science
• Coordinate with university and disciplinary leaders, to leverage resources
• Create processes that support reform
• Shift from diversity champions to collective engagement
• Critical mass of women faculty and faculty of color
• Track program-level data, disaggregated by race/ethnicity and gender
• Empower a department diversity, equity, or inclusion committee
A second useful reference is the National Academies of Sciences, Engineering, and Medicine, 2018 study of Graduate STEM Education for the 21st Century which describes an ideal path through graduate school. The report then calls for systematic change from the top level of federal agencies down through institutions, departments, faculty, to the graduate students. Here are a couple notable recommendations, first addressing the need to regularly evaluate and modify programs:
RECOMMENDATION 3.6—A Dynamic Graduate STEM Education System: The STEM education system should develop the capabilities to adjust dynamically to continuing changes in the nature of science and engineering activity and of STEM careers. This includes mechanisms to detect and anticipate such changes, experiment with innovative approaches, implement appropriate educational methods, and support institutional mechanisms on a larger scale.
• Faculty and graduate departments and programs should periodically review and modify curricula, dissertation requirements, and capstone projects to ensure timeliness and alignment with the ways relevant work is conducted and provide students with opportunities to work in teams that promote multidisciplinary learning.
• Professional societies and non-profit organizations should convene and lead discussions with graduate programs, employers, and other stakeholders and disseminate innovative approaches.
• Federal and state funding agencies, professional societies, and private foundations that support or conduct education research should support studies on how different STEM disciplines can integrate the changing scientific enterprise into graduate education programs and curricula.
• Graduate students should learn how to apply their expertise in a variety of professional contexts and seek guidance from faculty, research mentors, and advisors on strategies to gain work-related experience while enrolled in graduate school.
Another recommendation is to consider how graduate programs could improve the research process:
RECOMMENDATION 5.3—Structure of Doctoral Research Activities: Curricula and research projects, team projects, and dissertations should be designed to reflect the state of the art in the ways STEM research and education are conducted.
• Universities, professional societies, and higher education associations should take the lead in establishing criteria and updating characteristics of the doctoral research project and dissertation preparation and format.
• Students should seek opportunities to work in cross-disciplinary and cross-sector teams during their graduate education and via extracurricular activities and be incentivized by their departments and faculty advisors to do so.
• Graduate programs and faculty should encourage and facilitate the development of student teams within and across disciplines.
Three of the suggested actions for graduate students seem particularly valuable.
• Seek multiple mentors to meet their varied academic and career needs, such as information about potential career paths and employers.
• Learn how to apply their expertise in a variety of professional contexts and seek guidance from faculty, research mentors, and advisors on strategies to gain work-related experience while enrolled in graduate school.
• Engage in group activities and experiences outside of traditional academic settings to increase feelings of inclusion and to normalize feelings associated with negative phenomena, such as imposter syndrome, that can reduce productivity and success in the training experience and extend time to degree.
Finally, we encourage departments to develop online resources for both current students as well as recruitment of future graduate students. An excellent example is found at the University of Iowa (incidentally, the department of space physics pioneer James Van Allen) where the website has several links to resources providing advice to students (https://physics.uiowa.edu/graduate/advising).
Career development along braided pathways
Careers in STEM—and Solar and Space Physics is no exception—are complicated. Contrary to common folklore, few take a direct path from high school to university UG to grad school to post-doc to faculty or permanent jobs. Most weave a path that involves bends, turns, side loops—as illustrated in Figure 11 (from Batchelor et al., 2021). Each person must find what works for themself. Institutions need to recognize the damage of stereotyping career paths and assist people along their different pathways.
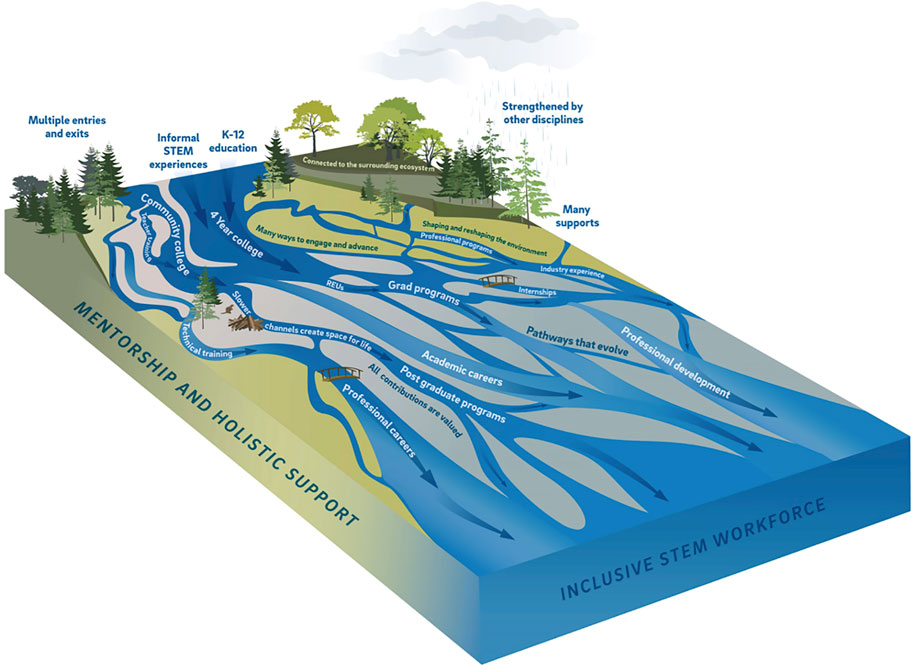
FIGURE 11. A braided river system illustrates a new, holistic STEM workforce career development model. Credit: Jennifer Matthews. From Batchelor et al. (2021).
The need for training and mentoring all along the braided pathways of careers in the space sciences was emphasized in the National Academies of Sciences, Engineering, and Medicine, 2022a report. The National Academies of Sciences, Engineering, and Medicine, 2022a focuses on training of potential PIs of competed missions:
RECOMMENDATION 12: Principal investigator (PI)-led missions present opportunities for aspiring PIs to gain invaluable experiences. NASA should expand resources (e.g., instructional materials, seminars, workshops) for aspiring PIs to gain leadership experience and connect with individuals with mission experience for mentorship opportunities. This may include.
• Integrating aspiring PIs as mentees in roles on mission teams, including the higher leadership positions. This could be achieved by including developmental positions in all missions (i.e., competed, non-competed, and instrument investigations), which may require increasing the PI-managed costs.
• Encouraging aspiring PIs to pursue entry points to mission leadership, such as proposing to smaller, low-cost mission opportunities (e.g., suborbital, SmallSats and CubeSats, instrument development, and hosted payloads).
• Expanding structured networking opportunities at relevant disciplinary conferences such as meet-and-greets where aspiring PIs can connect with collaborators and meet existing PIs, and participate in presentations and question and answer sessions led by NASA personnel about the proposal process.
… but similar advice applies throughout the space sciences—in research areas (e.g., data analysis, modeling, theory) as we as the more operational side (e.g., space weather prediction). Summer schools, workshops and conferences are vital opportunities to meet broader communities of scientists with various backgrounds, to learn about different fields, approaches and careers, and to tap into resources provided by professional organizations (such as APS, AGU, AAS). The larger conferences usually have booths where funding agencies display their programs, where you can ask the staff about opportunities such as post-doc, early-career, guest-investigator or participating scientist grants as well as find out about career opportunities at these agencies. Perhaps the simplest advice is to keep an open mind about potential careers, seek advice broadly, explore options, assess what extra skills are needed, and persist over bumps in the path.
Conclusions
We have presented the demographics data for the space physics workforce which we compared with other space sciences fields. Since the biggest change in demographics occurs in the high-school to college period, we focused on the early stages of college, and drew some lessons from looking beyond the US. We reviewed some of the studies from the National Academies, pulling out some relevant recommendations. Finally, we considered the actions that could be made locally by an institution or individually to enhance and diversify the career pathway through the space sciences. Our conclusions are summarized.
1) Space physics is pretty white and male. The percentage of women has been slowly improving but racial/ethnic diversity remains very small. Such demographics are similar to the physics profession overall.
2. The “pinch point” seems to be between high school and first year of college—when the percentage involvement of women in physics courses drops from 47% to ∼20%. The percentage of physics degrees awarded to Hispanic Americans has been rising for the past decade to 10% (still below the national population) while the percentage of physics degrees awarded to Black/African Americans remains ∼4%.
3. Studies of STEM entry-level college/university courses show common issues (e.g., poor teaching, “weed-out” culture, lack of support) with freshman math and physics classes for both those who switch and those who persist, across gender and race/ethnicity.
4. Concerted efforts have been made at various institutions across the country to improve retention of students in freshman STEM courses. These range from small, local efforts to improve teaching (e.g., more interactive classes, transparent and fair grading systems, student support, socially-relevant applications, etc.) to national sponsorship of internships, REU programs, bridge programs, teacher-training programs, etc. It is important to learn which programs are the most effective at promoting diversity and retention of STEM students.
5. Research suggests that early and ongoing experience with authentic research is key to retaining students generally and URM students specifically. Institutions are encouraged to develop research experience for undergraduates, during the semester as well as over the summer months.
6. For the past ∼30 years roughly half of Physics PhDs in the US have been awarded to people who are born outside the US. While this has boosted the percentage of women getting Physics PhDs (since many other countries award a larger percentage of their physics bachelor’s degrees to women) it means the STEM research and industry relies on imported talent which may not be such a secure source in the future. Besides, the US should provide education and career opportunities for all Americans.
7. In a national effort to enhance STEM careers, federal agencies and academic institutions need to a) gather data on the evolving STEM workforce; b) improve freshman math and physics classes; c) train more physics high-school teachers by providing combined physics + education degrees. Better pay for high school teachers would likely also help.
8. Attention is needed in improving and evolving graduate programs (with non-academic career advice, mentorship, multi-disciplinary experiences, involvement in missions, etc.), continued through post-doc and early-career support.
Marcia McNutt, President of the National Academy of Sciences, recently issued an editorial in Science magazine entitled “Higher Education For All”, urging the nation to provide higher education for everyone in the US (McNutt, 2022):
“It is time for educators to ask which innovations can be introduced and, importantly, sustained, to expand the accessibility of higher education to meet the needs of the 21st century.”
Author contributions
The author confirms being the sole contributor of this work and has approved it for publication.
Acknowledgments
We thank Margy Kivelson, Julie Rathbun, Noah Finkelstein, Mark Moldwin and Cherilyn Morrow for discussions. The NASEM staff are thanked for their assistance with studies. Steve Bartlett is thanked for assistance with graphics.
Conflict of interest
The author declares that the research was conducted in the absence of any commercial or financial relationships that could be construed as a potential conflict of interest.
Publisher’s note
All claims expressed in this article are solely those of the authors and do not necessarily represent those of their affiliated organizations, or those of the publisher, the editors and the reviewers. Any product that may be evaluated in this article, or claim that may be made by its manufacturer, is not guaranteed or endorsed by the publisher.
References
American Academy of Arts and Sciences (2020). The Perils of complacency: America at a tipping point in science and engineering. Cambridge, MA. Available at https://www.amacad.org/publication/perils-of-complacency.
Batchelor, R. L., Ali, H., Gardner-Vandy, K. G., Gold, A. U., MacKinnon, J. A., and Asher, P. M. (2021). Reimagining STEM workforce development as a braided river. Eos 102. doi:10.1029/2021EO157277
Bernard, R. E., and Cooperdock, E. H. G. (2018). No progress on diversity in 40 years. Nat. Geosci. 11, 292–295. doi:10.1038/s41561-018-0116-6
Bradforth, S. E., Miller, E. R., Dichtel, W. R., Leibovich, A. K., Feig, A. L., Martin, J. D., et al. (2015). University learning: Improve undergraduate science education. Nature 523, 282–284. doi:10.1038/523282a
Campbell, L., Mehtani, S., Dozier, M., and Rinehart, J. (2013). Gender-heterogeneous working groups produce higher quality science. PLOS ONE 8 (10), e79147. doi:10.1371/journal.pone.0079147
Erickson, K. J. (2021). NASA’s science activation program, achievements and opportunities. Presentation to the decadal survey. Washington, DC: National Academy of Sciences. Available at https://www.nap.edu/read/25569/chapter/12.
Freeman, R. B., and Huang, W. (2014b). Collaborating with people like me: Ethnic Co-authorship within the US. NBER working paper series 19905. Cambridge, MA: National Bureau of Economic Research. Available at https://www.nber.org/papers/w19905.
Freeman, R. B., and Huang, W. (2014a). Collaboration: Strength in diversity. Nature 513 (305), 305. doi:10.1038/513305a
Hodapp, T., and Hazari, Z. (2015). Women in physics: Why so few?. APS news. 24. Available at https://www.aps.org/publications/apsnews/201511/backpage.cfm.
Hong, S., and Page, S. E. (2004). Groups of diverse problem solvers can outperform groups of high-ability problem solvers. Proc. Natl. Acad. Sci. 101 (46), 16385–16389. doi:10.1073/pnas.0403723101
Institute of Medicine (2007). Rising above the gathering Storm: Energizing and employing America for a brighter economic future. Washington, DC: The National Academies Press. doi:10.17226/11463
Ivie, R., and Guo, S. (2006). Women physicists speak again. College Park, MD: American Institute of Physics Statistical Research Center. Available at https://www.aip.org/statistics/reports/women-physicists-speak-again.
Massey, R., Drake, D., Kanani, S., and McWhinnie, S. (2017). Our scientific community in 2016. Astron. Geophys 58, 6.14–6.17. doi:10.1093/astrogeo/atx211
Moldwin, M. B., Torrence, J., Moldwin, L. A., and Morrow, C. (2013). Is there an appropriate balance between the number of solar and space physics PhDs and the jobs available? Space weather. 11, 445–448. doi:10.1002/swe.20075
NASA Science Plan (2020). Science 2020-2024: A vision for scientific excellence. Washington, D.C: NASA Headquarters. Available at https://science.nasa.gov/science-red/s3fs-public/atoms/files/2020-2024_Science.pdf.
National Academies of Sciences, Engineering, and Medicine (2022a). Advancing diversity, equity, inclusion, and accessibility in the leadership of competed space missions. Washington, DC: The National Academies Press. doi:10.17226/26385
National Academies of Sciences, Engineering, and Medicine (2022b). Foundations of a Healthy and vital research community for NASA science. Washington, DC: The National Academies Press. doi:10.17226/26575
National Academies of Sciences, Engineering, and Medicine (2018). Graduate STEM education for the 21st century. Washington, DC: The National Academies Press. doi:10.17226/25038
National Academies of Sciences, Engineering, and Medicine (2021). Plasma science: Enabling technology, sustainability, security, and exploration. Washington, DC: The National Academies Press. doi:10.17226/25802
National Research Council (2013). Solar and space physics: A science for a technological society. Washington, DC: The National Academies Press. doi:10.17226/13060
National Science Board (2022). Science and engineering indicators 2022: The state of U.S. Science and engineering. NSB-2022-1. Alexandria, VA. Available at https://ncses.nsf.gov/pubs/nsb20221.
National Science Foundation (2019). Doctorate recipients from U.S. Universities: 2019. Report NSF 21-308. Alexandria, VA: National Center for Science and Engineering Statistics. Available at https://ncses.nsf.gov/pubs/nsf21308.
Pold, J., and Ivie, R. (2018). Workforce survey of 2018 US AAS members: Summary results. College Park, MD: American Institute of Physics Statistical Research Center. Available at https://aas.org/sites/default/files/2019-10/AAS-Members-Workforce-Survey-final.pdf.
Porter, A. M., and Ivie, R. (2019). Women in physics and astronomy, 2019. Focus on report march 2019. College Park, MD: American Institute of Physics Statistical Research Center. Available at https://www.aip.org/statistics/reports/women-physics-and-astronomy-2019.
Porter, A. M., White, S., and Dollison, J. (2020). 2020 survey of the planetary science workforce. College Park, MD: American Institute of Physics Statistical Research Center. Available at https://dps.aas.org/sites/dps.aas.org/files/reports/2020/Results_from_the_2020_Survey_of_the_Planetary_Science_Workforce.pdf.
Posselt, J. R. (2020). Equity in Science: Representation, culture, and the dynamics of change in graduate education. Stanford, CA: Stanford University Press.
Posselt, J. R. (2016). Inside graduate admissions: Merit, diversity, and faculty gatekeeping. Cambridge, MA: Harvard University Press.
Posselt, J. R., Reyes, K. A., Slay, K. E., Kamimura, A., and Porter, K. B. (2017). Equity efforts as boundary work: How symbolic and social boundaries shape access and inclusion in graduate education. Teach. Coll. Rec. 119, 1–38. doi:10.1177/016146811711901003
Posselt, J. R. (2014). Toward inclusive excellence in graduate education: Constructing merit and diversity in PhD admissions. Am. J. Educ. 120 (4), 481–514. doi:10.1086/676910
Royal Astronomical Society (2017). The demographics and research interests of the UK astronomy and geophysics communities. Available at https://ras.ac.uk/ras-policy/community-demographics/demographic-survey-2017.
Russell, S. H., Hanckock, M. P., and McCullough, J. (2007). Benefits of undergraduate research experiences. Science 316, 548–549. doi:10.1126/science.1140384
E. S. Seymour, and N. M. Hewitt (Editors) (1997). Talking about leaving: Why undergraduates leave the sciences (Boulder, CO: Westview Press).
E. S. Seymour, and A-B. Hunter (Editors) (2019). Talking about leaving revisited: Persistence, relocation, and Loss in undergraduate STEM education (Switzerland: Springer Nature). doi:10.1007/978-3-030-25304-2
White, S., Anderson, G., and Ivie, R. (2011a). Results from the 2011 survey of solar. College Park, MD: Space and Upper Atmospheric Physicists, American Institute of Physics, Statistical Research Center. doi:10.7302/25
White, S., Chu, R. Y., and Ivie, R. (2011b). Survey of the planetary science workforce. College Park, MD: American Institute of Physics, Statistical Research Center. Available at https://lasp.colorado.edu/home/mop/resources/planetary-scienceworkforce-survey/2011-planetary-science-survey.
Keywords: stem education, workforce, diversity, demographics, space physics research
Citation: Bagenal F (2023) Enhancing demographics and career pathways of the space physics workforce in the US. Front. Astron. Space Sci. 10:1130803. doi: 10.3389/fspas.2023.1130803
Received: 23 December 2022; Accepted: 06 February 2023;
Published: 17 February 2023.
Edited by:
Michael W Liemohn, University of Michigan, United StatesReviewed by:
Amy Keesee, University of New Hampshire, United StatesMaria-Theresia Walach, Lancaster University, United Kingdom
Copyright © 2023 Bagenal. This is an open-access article distributed under the terms of the Creative Commons Attribution License (CC BY). The use, distribution or reproduction in other forums is permitted, provided the original author(s) and the copyright owner(s) are credited and that the original publication in this journal is cited, in accordance with accepted academic practice. No use, distribution or reproduction is permitted which does not comply with these terms.
*Correspondence: Fran Bagenal, bagenal@colorado.edu