- Space Science Center, University of New Hampshire, Durham, NH, United States
Combined in situ observations of the interstellar wind through the solar system and of its pickup ions (PUIs), implanted after ionization in the solar wind, explain, in comparison with interstellar absorption lines of nearby stars, that the Sun is in an interaction region of the two nearest interstellar clouds. This new finding disrupts the long-held understanding that we are inside the local interstellar cloud (LIC). We discuss how space physics evolved toward such interdisciplinary studies between heliophysics and astrophysics. In 1984, the discovery of interstellar He+ PUIs exposed the very local interstellar medium to in situ diagnostics at 1AU. These PUIs provide the interstellar gas composition and form a stepping stone for the acceleration of ions, especially into anomalous cosmic rays. Using the Sun as a gravitational spectrograph, direct imaging of the neutral interstellar wind, first for He and then for H, O, and Ne, provides the interstellar gas velocity vector and temperature at the heliopause. Combining the interstellar gas flow vectors, those of secondary neutral He and O, and the interstellar magnetic field direction deduced from the interstellar H deflection and termination shock anisotropy seen by the Voyagers provides synergistically the heliosphere’s shape, its interaction with the interstellar medium, and constrains our radiation environment. This ISMF organizes the bright Ribbon seen in all-sky images of energetic neutral atoms with the potential to provide its precision determination. The elemental and isotopic composition from PUI and neutral gas observations constrains the galactic evolution and Big Bang cosmology, opening additional interdisciplinary opportunities.
1 Introduction
For a long time, astronomers have located the Sun inside the local interstellar cloud (LIC) (Bertin et al., 1993; Lallement et al., 1995; Redfield and Linsky, 2008; Frisch et al., 2009), albeit close to its edge. However, a recent study places the Sun in a mixing region between the LIC and the G-Cloud (Swaczyna et al., 2022a). It compares in situ measurements of the interstellar neutral (ISN) gas flow vector through the solar system, based on precision analysis of ISN imaging (Wood et al., 2015; Swaczyna et al., 2022b) and pickup ion (PUI) observations (Taut et al., 2018; Bower et al., 2019), with those obtained from stellar absorption lines for nearby interstellar clouds. PUI observations on New Horizons that the H density in our neighborhood is twice as high (Swaczyna et al., 2020) than that derived from absorption lines in the LIC and G-Cloud (Redfield and Linsky, 2008; Linsky et al., 2022) support this finding. To my knowledge, this is the first time that space physics-based in situ observations have diagnosed the interaction of two interstellar clouds, placing the solar system exactly where the action is. Thus, the time has come to conduct genuine interdisciplinary studies between space and astrophysics, and we will map the road that led us here.
2 Discovery of interstellar pickup ions
The journey started on 11 September 1984 at the German Spacecraft Operations Center in Oberpfaffenhofen. After waiting in suspense, the solar wind (SW) conditions appeared to be on target for the first ion cloud release from the Active Magnetospheric Particle Tracer Explorers (AMPTE) Ion Release Module (IRM) (Haerendel et al., 1985), with the interplanetary magnetic field (IMF) almost perpendicular to the SW. After a quick readiness check, the IRM ejected two Li and CuO canisters. They drifted for 10 mins before the mixture ignited and generated an expanding cloud, which silenced the SW for a few seconds like within a comet coma (Häusler et al., 1986). The nearly perpendicular IMF was supposed to pick up the freshly generated Li+ ions and propel them toward the Earth’s magnetosphere. Capable of identifying these ions, the time-of-flight (TOF) spectrograph AMPTE SULEICA (Möbius et al., 1985a) indeed found only seven Li+ ions before the IMF turned any ions out of its field of view (FOV) (Möbius et al., 1986). However, this disappointing result came with a stunning surprise. He+ ions showed up with the Li+ ions repeatedly. The IMF must have picked them up in the SW, freshly ionized from a gas almost at rest like Li, as shown in Figure 1A, hence called PUIs.
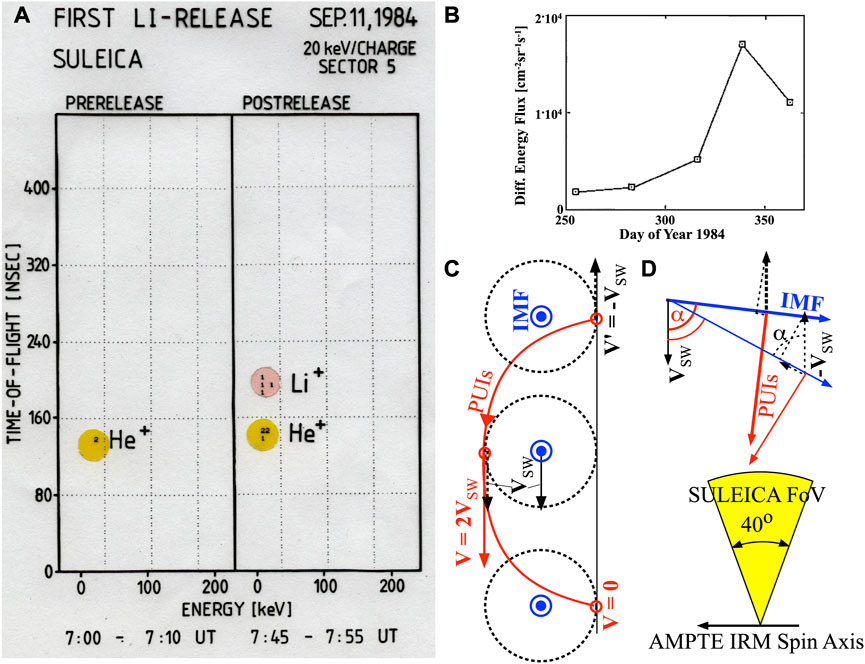
FIGURE 1. (A) Historic TOF versus energy representation of individual He+ and Li+ PUIs detected before and after the first AMPTE Li release. (B) He+ PUI flux obtained with AMPTE SULEICA as a function of day of the year in 1984. The flux reaches a maximum in early December when the Earth is downwind of the Sun relative to the ISN gas flow through the solar system [adapted from (Möbius et al., 1985b)], reproduced with permission from Springer Nature. AMPTE IRM was in the SW only from September through January, hence the clipped coverage of PUIs. (C) PUI motion in the plane perpendicular to the IMF (the blue circular symbol), gyrating about the IMF in the SW frame (the dashed black line), and moving on a cycloid in the spacecraft frame (the solid red line). After ionization from neutral gas at rest, a PUI (the open red circle) starts with V = 0 in the rest frame, while it is injected into the SW with V’ = -VSW in the SW frame. Therefore, the PUI gyrates about the IMF with the speed VSW (the dashed black circle). In the rest frame, this gyration is seen as a cycloid trajectory, which starts with V = 0, reaches a maximum speed of 2VSW or cut-off at the top, where the PUI speed in the SW frame adds to the SW speed, and completes one turn again with V = 0. This motion is similar to that of a valve of a bicycle wheel, as seen by an observer at rest. It distinguishes PUIs from the motion and energy distribution of SW ions. Scattering of PUIs in their pitch angle α (see 1D) at fluctuations in the IMF bring PUIs to the cut-off for all IMF angles. Continuous PUI production from the Sun to the observer and adiabatic cooling in the expanding SW fills the velocity space between 2Vsw and the SW itself. An introduction to PUIs may be found in a recent text book (Hsieh and Möbius, 2022) and review (Zirnstein et al., 2022) and presents a full overview on PUIs in the heliosphere. (D) PUI motion in the SW-IMF plane, along with the AMPTE SULEICA FoV. If the IMF is at an angle α < 90° relative to the SW, PUIs are injected into the SW at pitch angle α, resulting in a velocity component parallel to the IMF, which leads to an overall transport of PUIs in the rest frame at angle α relative to the IMF. Shown are sample directions of the PUI motion for IMF orientations that, after initial pickup, lead the ions into the FoV (solid line, α > 70°) or miss the FoV (dashed line, α < 70°).
Their fluxes exceeded those for He from the Earth’s exosphere at IRM’s distance by three orders of magnitude. Memories arose from an astronomy seminar as a student at Bochum about background radiation. My assignment focused on Lyman-α background, which, to my disappointment, originated in our backyard, ISN gas illuminated by the Sun (Bertaux and Blamont, 1971). Contrary to earlier astronomical wisdom, the solar system is not (Fahr, 1968) within a Strömgren sphere (Strömgren, 1939). The ISN gas blows through the solar system as an interstellar wind, too fast for ionization before reaching 1 AU. Interstellar He forms a unique pattern in the inner heliosphere, focused downwind of the Sun (Weller and Meier, 1974; Fahr et al., 1976). I thought, “Wouldn’t it not be cool if the He+ ions were of interstellar origin?” Indeed, they exhibited the predicted behavior with a substantial flux enhancement in early December when the Earth is downwind of the flow (Möbius et al., 1985b) (Figure 1B). The literature from a student seminar proved invaluable for identifying a fundamental discovery, PUIs from the interstellar wind, and so did the humble explorer AMPTE (Krimigis et al., 1982), initiated by Tom Krimigis and Gerhard Haerendel.
The He+ ions were visible continuously up to a cut-off at 2Vsw or 4Esw, as previously predicted (Vasyliunas and Siscoe, 1976) (Figure 1C), contrary to locally injected Li+ PUIs that entered SULEICA only during favorable IMF orientations (Figure 1D). Continuous He+ PUI injection into the SW affords its effective scattering in pitch angle α at IMF fluctuations and adiabatic cooling in the expanding SW (Isenberg, 1986; Isenberg, 1987; Möbius et al., 1988), filling the observed spectra up to 4Esw, as explained in a recent text book (Hsieh and Möbius, 2022) and PUI review (Zirnstein et al., 2022).
3 Diagnostic opportunities with pickup ions and their challenges
This first in situ diagnostic method for ISN gas expanded the horizon of space plasma physics into the Sun’s galactic neighborhood. It allowed the sampling of interstellar He, H (Gloeckler et al., 1993), N, O, and Ne (Gloeckler and Geiss, 1998), the calculation of their abundance ratios (Gloeckler and Geiss, 2001), and an estimate of the ISN flow velocity and temperature (Möbius et al., 1995).
However, PUIs presented formidable challenges in determining the dynamic ISN parameters precisely. Slower than anticipated pitch-angle scattering made the PUI distributions asymmetric in the SW frame, softened the otherwise sharp cut-off at 2Vsw, and lowered the most easily accessible part of the PUI fluxes above the SW energy (Gloeckler et al., 1995; Möbius et al., 1998). PUI distributions and fluxes varied substantially in response to SW structures, such as stream interaction regions (SIRs) and coronal mass ejections (CMEs) (Möbius et al., 2010). Non-radial PUI transport in the SW affects the shape and location of the focusing cone (Möbius et al., 1996; Chalov and Fahr, 2006; Quinn et al., 2016). Effective acceleration into a suprathermal tail smoothens the PUI cut-off further (Gloeckler et al., 2000; Möbius et al., 2019).
However, one person’s trash may be another’s treasure. When interstellar PUIs came up as a tentative explanation for the He+ by AMPTE IRM, Dieter Hovestadt exclaimed “SULEICA has detected the seed particles for the anomalous cosmic rays (ACR)” (Garcia-Munoz et al., 1973; Hovestadt et al., 1973; Klecker, 1995; Jokipii, 1998). ACRs are substantially overabundant in O and Ne over galactic cosmic rays. Dieter pointed to a model that implicated the ISN gas (Fisk et al., 1974) and opened another essential connection for PUIs. They form a particle distribution that enables preferential injection into acceleration to higher energies. The enormous injection efficiency compared to underlying bulk plasma was visible in SIRs (Gloeckler et al., 1994; Morris et al., 2001; Möbius et al., 2002) and CMEs (Kucharek et al., 2003), with remarkable He+ overabundances over SW He2+ in the respective energetic particles. Identifying He+ PUIs as the source solved a previous mystery: He+/He2+ ratios in energetic interplanetary particles that substantially exceeded the SW ratio (Hovestadt et al., 1984).
Another aspect of the PUI distribution took a surprising turn much later. During a seminar on the PUI discovery, Martin Lee mused whether Alfvén waves that lag behind the SW could reduce the He+ PUI cut-off energy by ≈ 10–15% from the nominal 4Esw as the observations seemed to suggest. However, this conclusion would have been a stretch with SULEICA’s 10% energy width, data points spaced by 20% in energy, and integration over a 40 × 45° FOV. Only 15 years later, when analyzing PUI data from CELIAS (Hovestadt et al., 1995) on the SOlar and Heliospheric Observatory (SOHO), centered around June, or upwind of the Sun relative to the ISN flow, the original question entered daylight again but with a twist. The cut-off was ≈15% above the nominal value. SOHO observing upwind and AMPTE IRM downwind suggested an explanation. Because in the SW frame, PUI injection occurs with the vector sum of its local ISN flow and SW velocities, and the cut-off was at a noticeably higher PUI speed on the upwind side than the downwind side (Möbius et al., 1999).
The advent of PLASTIC (Galvin et al., 2008) on the Solar Terrestrial RElations Observatory (STEREO), with superior energy and angle resolution in the PUI regime, enabled a more detailed study of the PUI evolution after their initial injection (Drews et al., 2015). It also turned this earlier discovery into a precision tool to obtain at least the ISN flow direction with much higher fidelity. When increasing ionization losses of the ISN flow from the upwind to the downwind side could not produce the He+ PUI crescent (Sokól et al., 2016), as proposed earlier (Drews et al., 2012), flux modulation due to the shifting PUI cut-off within the fixed energy window in the analysis became the focal point. This explanation suggested using symmetry in the PUI cut-off shift in the flow axis to determine the ISN flow longitude precisely (Möbius et al., 2015a; Taut et al., 2018; Bower et al., 2019). This measurement has now become a linchpin in obtaining the complete set of dynamic ISN gas parameters in the very local interstellar medium (VLISM) just outside the solar system from local observations the inner heliosphere, complementary to the direct ISN flow observations at 1 AU (Möbius et al., 2009a) with the Interstellar Boundary Explorer (IBEX) (McComas et al., 2009a). These neutral gas measurements became possible after the pioneering observations of the He ISN flow with Ulysses GAS (Witte et al., 1996).
4 Catching the neutral interstellar wind directly
The discovery of the interstellar PUIs triggered the invitation by Hans Fahr in 1986 to a series of workshops focused on the interaction between the heliosphere and the surrounding interstellar medium that involved German, Polish, and Soviet groups. The workshops revolved around observational and modeling efforts to understand our galactic neighborhood. During one meeting, Helmut Rosenbauer jokingly regretted that the PUI observations would steal the thunder of Ulysses GAS (Witte et al., 1992), whose launch was still in the future. However, this tiny sensor that measured the ISN He distribution via sputtering Li+ ions off a LiF surface obtained images of the He ISN flow during the transit of Ulysses to Jupiter and during the three fast latitude scans when Ulysses scoped out the 3D structure of the SW and energetic particles. Following the He atoms along their hyperbolic trajectories in the Sun’s gravitational field (Fahr, 1974; Wu and Judge, 1979; Lee et al., 2012; Lee et al., 2015) with a tailored fitting technique (Banaszkiewicz et al., 1996) translates the neutral He images into the velocity distribution function outside the heliosphere. The GAS observations of the He ISN flow enabled the most detailed and accurate determination of the ISN flow vector and temperature (Witte, 2004). These values were validated and placed into context with PUI and solar ultraviolet backscattering observations of ISN He within a scientific team at the International Space Science Institute (ISSI). The team effort consolidated the ISN gas parameters (Möbius et al., 2004) and reemphasized the complementary nature of the three in situ observation techniques, each affected by different systematic uncertainties.
This collaborative work on the physical state of the interstellar medium dovetailed into the proposal of a potential explorer mission to study the VLISM and its interaction with the heliosphere, the Interstellar Pathfinder, which, in two attempts, almost made it into a Phase B study, but only “almost”. The studies proposed sounded like the perfect interdisciplinary endeavor that could engage two scientific communities, heliophysics and astrophysics, and thus garner multiple support. Instead, the advice was to root the proposal firmly in heliophysics; otherwise, it may fall between all chairs. In a third attempt, the concentration on two neutral atom cameras with the capability to image the boundary of the heliosphere in energetic neutral atoms (ENA) and to simultaneously capture the interstellar wind of He, O, and possibly H under the constraints of a Small Explorer kicked the proposal above the threshold and led to the successful IBEX mission (McComas et al., 2009a).
The combination of mechanical collimation, surface conversion of neutral atoms into negative ions, electrostatic energy analysis, post-acceleration, and a triple time-of-flight measurement in IBEX-Lo (Fuselier et al., 2009) enabled the observation of the He, H, and O ISN flow (Möbius et al., 2009b) and even Ne (Bochsler et al., 2012) (Figures 2A,C). Thus, IBEX went substantially beyond the GAS capabilities, increasing the signal-to-background ratio by orders of magnitude for He and expanding to other species. However, with its observations limited severely in ecliptic longitude to less than 2 months in early spring when the IBEX FOV points to the oncoming flow, approximately parallel to the Earth’s orbit, the observations and analysis are subject to degeneracy in the ISN parameter space. It is obvious for an idealized ISN trajectory that passes IBEX precisely perpendicular to the IBEX-Sun line, i.e., reaching its perihelion at the point of observation (Möbius et al., 2012) (Figures 2B,D). Trajectories that start at infinity over a wide range of ISN speeds VISN∞ and inflow longitudes λISN∞ fulfill this condition when coupled with the hyperbolic trajectory equation:
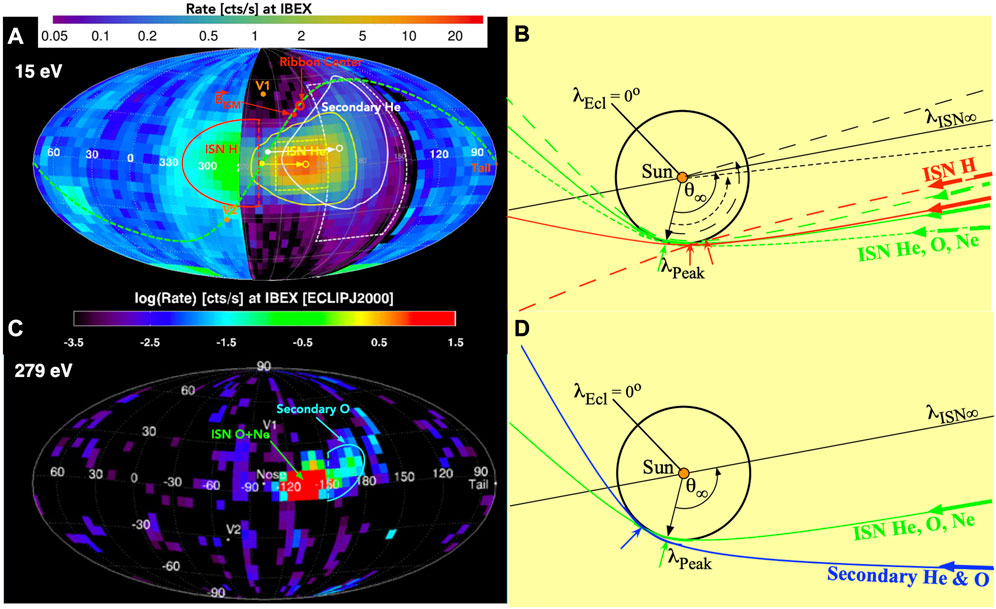
FIGURE 2. Left: Combination of IBEX ENA maps in Mollweide projection that illustrate the interstellar medium information collected at 1 AU. Right: Schematic view of the ISN and ENA trajectories from their source to the observer at 1 AU. (A) ISN He and H flow based on H count rates at 15 eV, along with secondary He neutrals [adapted from (Park et al., 2016; Swaczyna et al., 2018)], reproduced with permission from AAS. (B) Schematic representation of ISN He, O, and Ne (green), as well as H (red) trajectories in the plane that contains
θ∞ is the angle swept out by the position vector of the atom from infinity to perihelion or the true anomaly of the trajectory; λPeak is the ecliptic longitude of the observer when seeing the peak ISN flow; Ms is the Sun’s mass; G is the gravitational constant; and rE = 1 AU (Lee et al., 2012). Because the inflow latitude βISN∞ and temperature TISN∞ connect these quantities dynamically, the analysis of IBEX observations leads to a four-dimensional tube in the parameter space (McComas et al., 2012). Observations of the full He distribution over a range in ecliptic longitude constrain the tube in length (Schwadron et al., 2015a; Möbius et al., 2015b; Swaczyna et al., 2015; Swaczyna et al., 2018), and the PUI analysis previously discussed provides a complementary value for λISN (Möbius et al., 2015a; Taut et al., 2018; Bower et al., 2019).
5 Synergism between PUI, ISN flow, and ENA observations for the heliosphere and beyond
When IBEX-Lo caught the interstellar wind, IBEX-Hi (Funsten et al., 2009) and Lo (Fuselier et al., 2009) combined took the first all-sky images of the heliospheric boundary in the light of ENAs, thus expanding the emerging field of neutral-atom astronomy to its horizon (Hsieh and Möbius, 2022). At low ENA energies, the IBEX maps reveal secondary He (Kubiak et al., 2014; Kubiak et al., 2016) (Figure 2A) and O (Park et al., 2015; Park et al., 2016) (Figure 2C) interstellar neutral flows, which originate in the region outside the heliopause from the charge exchange of interstellar ions with the ISN gas flow. The secondary neutral flow appears slower and hotter than the pristine ISN flow, hence also referred to as the “warm breeze” (Kubiak et al., 2014). This is because the secondary neutrals mimic the distribution of the interstellar plasma, which slows down and heats in response to the presence of the heliosphere. As the secondary neutral signal is at a level of a few percent of the pristine ISN flow for He and O, its prior analysis is necessary before the velocity distribution of secondary neutrals can be extracted from the observations. Both secondary populations appear substantially deflected relative to the pristine ISN flow in the same direction as ISN H (Lallement et al., 2005) in a plane, dubbed the H-deflection plane, which, based on global heliospheric simulations, contains the interstellar flow velocity
Interestingly, the first IBEX ENA sky maps revealed a bright unanticipated Ribbon (McComas et al., 2009b) that traces out a circle in the sky, which conforms with
However, the magnetic field direction measured by Voyager 1 and 2 outside the heliopause, starting in line with the heliospheric field, and the Ribbon-derived direction are still far from each other. After first seemingly approaching the IBEX direction (Schwadron et al., 2015c), it turned back again in response to heliospheric disturbances (Schwadron et al., 2018). Also, the ENA belt, discovered by Cassini INCA at higher energies (Krimigis et al., 2009), appears oriented differently in the sky than the Ribbon, and the heliosheath thickness and heliosphere shapes differ as derived from the ENAs in different energy regimes (Dialynas et al., 2017; Schwadron and Bzowski, 2018). Whether and how the remote and in situ observations of
Yet, combining the O, He, and H ISN flow arrival directions at the heliopause with the He and O secondary neutrals and the ISMF direction arranges them along an arc that represents the plane, which organizes the deflection of the interstellar plasma flow around the heliosphere (Pogorelov et al., 2009b; Izmodenov et al., 2009). Thus, together with
6 Foray into the VLISM, a truly interdisciplinary endeavor between space plasma and astrophysics
With the fidelity of the ISN flow and secondary neutral observations and analysis achieved to date, catching the interstellar wind in the inner heliosphere now connects to several aspects of astrophysics in our galactic neighborhood. For example, a detailed analysis of the secondary He flow provides the He+ density, and thus, the ratio of ionized and neutral He and H in the VLISM (Bzowski et al., 2019), which, in turn, constrains the radiation environment in the Sun’s neighborhood (Slavin and Frisch, 2008). Improvements in the precision of the locally obtained interstellar flow parameters (Swaczyna et al., 2022b) and densities (Swaczyna et al., 2020) now strongly support an earlier suspicion, i.e., that the Sun is neither inside the LIC nor the G-cloud proper (Redfield and Linsky, 2008). Most likely, the solar system traverses an interaction region between these two adjacent interstellar clouds in our immediate galactic neighborhood (Swaczyna et al., 2022a). A recently discovered anisotropy in the ISN He distribution (Wood et al., 2019) may even be a sign of incomplete mixing of the two cloud populations and thus provide insights into the kinetics of the interstellar cloud interaction. Furthermore, obtaining abundances of the ISN species that make it inside the heliosphere through PUI (Gloeckler and Geiss, 2001) and ISN sampling, in particular, for O and Ne (Park et al., 2014), provides a window on the processing of matter in the Milky Way over time (Prantzos et al., 1998). With the interstellar 3He/4He ratio from PUIs (Gloeckler and Geiss, 1996) and the D/H ratio from ISN observations (Rodriguez-Moreno et al., 2013), we even touch upon cosmology and Big Bang nucleosynthesis (Schramm et al., 1998). In summary, combining space physics-based in situ diagnostics and astronomy-based spectroscopy finally enables genuine interdisciplinary research opportunities.
By placing a powerful suite of sensors for ENAs, PUIs, energetic particles, interstellar dust, and Lyman-α radiation at the Lagrangian point L1, while monitoring the interplanetary environment, the Interstellar Mapping and Acceleration Probe (IMAP) (McComas et al., 2018) will bring our understanding of the heliosphere and its place in the interstellar medium to the next level. A future dream of an Interstellar Probe that will venture into the VLISM proper and unravel thus far inaccessible ion populations and related interaction processes has recently moved closer to a realization after a detailed scientific and technical feasibility study (McNutt et al., 2021), (Brandt et al., 2023).
Data availability statement
Publicly available datasets were analyzed in this study. These data can be found at: http://ibex.swri.edu/researchers/publicdata.shtml.
Author contributions
The author confirms being the sole contributor of this work and has approved it for publication.
Acknowledgments
The author is very grateful to the many individuals at various institutions that made the many spacecraft missions successful whose observations contributed substantially to the results compiled in this paper. He also gratefully acknowledges the many fruitful collaborations with wonderful colleagues that triggered new insights in many ways. Support for the work toward this manuscript by the IBEX and IMAP projects and by NASA Grant 80NSSC18K1212 is thankfully acknowledged.
Conflict of interest
The author declares that the research was conducted in the absence of any commercial or financial relationships that could be construed as a potential conflict of interest.
Publisher’s note
All claims expressed in this article are solely those of the authors and do not necessarily represent those of their affiliated organizations, or those of the publisher, the editors, and the reviewers. Any product that may be evaluated in this article, or claim that may be made by its manufacturer, is not guaranteed or endorsed by the publisher.
References
Abbasi, R., Abdou, Y., Abu-Zayyad, T., Adams, J., Aguilar, J. A., Ahlers, M., et al. (2011). Observation of anisotropy in the arrival directions of galactic cosmic rays at multiple angular scales with IceCube. Astrophys. J. 740, 16.
Abdo, A. A., Allen, B., Aune, T., Berley, D., Blaufuss, E., Casanova, S., et al. (2008). Discovery of localized regions of excess 10-TeV cosmic rays. Phys. Rev. Lett. 101, 221101. doi:10.1103/physrevlett.101.221101
Abdo, A. A., Allen, B., Aune, T., Berley, D., Casanova, S., Chen, C., et al. (2009). The large-scale cosmic-ray anisotropy as observed with Milagro. Astrophys. J. 698, 2121–2130. doi:10.1088/0004-637x/698/2/2121
Banaszkiewicz, M., Witte, M., and Rosenbauer, H. (1996). Determination of interstellar helium parameters from theULYSSES-NEUTRALGAS experiment: Method of data analysis. Astron. Astrophys. Suppl. 120, 587–602. doi:10.1051/aas:1996311
Bertaux, J. L., and Blamont, J. E. (1971). Evidence for a source of an extraterrestrial hydrogen lyman-alpha emission. Astron. Astrophys. 11, 200–217.
Bertin, P., Lallement, R., Ferlet, R., and Vidal-Madjar, A. (1993). Detection of the local interstellar cloud from high-resolution spectroscopy of nearby stars: Inferences on the heliospheric interface. J. Geophys. Res. 98, 15193–15198. doi:10.1029/93ja01179
Bochsler, P., Petersen, L., Möbius, E., Schwadron, N. A., Wurz, P., Scheer, J. A., et al. (2012). Estimation of the neon/oxygen abundance ratio at the heliospheric termination shock and in the local interstellar medium from IBEX observations. Astrophys. J. Suppl. 198, 13. doi:10.1088/0067-0049/198/2/13
Bower, J., Moebius, E., Berger, L., Farrugia, C., Keilbach, D., Lee, M. A., et al. (2019). Effect of rapid changes of solar wind conditions on the pickup ion velocity distribution. J. Geophys. Res. 124, 6418–6437. doi:10.1029/2019ja026781
Brandt, P. C., Provornikova, E., Bale, S. D., Cocoros, A., DeMajistre, R., Dialynas, K., et al. (2023). Future exploration of the outer heliosphere and very local interstellar medium by interstellar Probe. Space Sci. Rev. 219, 18. doi:10.1007/s11214-022-00943-x
Burlaga, L. F., and Ness, N. F. (2014). Voyager 1 observations of the interstellar magnetic field and the transition from the heliosheath. Astrophys. J. 784, 146. doi:10.1088/0004-637x/784/2/146
Bzowski, M., Czechowski, A., Frisch, P. C., Fuselier, S. A., Galli, A., Grygorczuk, J., et al. (2019). Interstellar neutral helium in the heliosphere from IBEX observations. VI. The He+ density and the ionization state in the Very Local Interstellar Matter. Astrophys. J. 882, 60. doi:10.3847/1538-4357/ab3462
Chalov, S. V., and Fahr, H-J. (2006). Pickup interstellar helium ions in the region of the solar gravitational cone. Astron. Lett. 32, 487–494. doi:10.1134/s1063773706070061
Dialynas, K., Krimigis, S. M., Mitchell, D. G., Decker, R. B., and Roelof, E. C. (2017). The bubble-like shape of the heliosphere observed by Voyager and Cassini. Nat. Astron. 1, 0115. doi:10.1038/s41550-017-0115
Drews, C., Berger, L., Taut, A., Peleikis, T., and Wimmer-Schweingruber, R. F. (2015). 2D He+pickup ion velocity distribution functions: STEREO PLASTIC observations. Astron. Astrophys. 575, A97. doi:10.1051/0004-6361/201425271
Drews, C., Berger, L., Wimmer-Schweingruber, R. F., Bochsler, P., Galvin, A. B., Klecker, B., et al. (2012). Inflow direction of interstellar neutrals deduced from pickup ion measurements at 1 AU. J. Geophys. Res. 117, A09106. doi:10.1029/2012ja017746
Fahr, H-J. (1968). Charge-transfer interactions between solar wind protons and neutral particles in the vicinity of the Sun. Nature 219, 473–474. doi:10.1038/219473a0
Fahr, H-J., Lay, G., and Blum, P. W. (1976). Planetary cones of focused interstellar gases. Astron. Astrophys. 52, 363–372.
Fahr, H-J. (1974). The extraterrestrial UV-background and the nearby interstellar medium. Space Sci. Rev. 15, 483–540. doi:10.1007/bf00178217
Fisk, L. A., Kozlovsky, B., and Ramaty, R. (1974). An interpretation of the observed oxygen and nitrogen enhancements in low-energy cosmic rays. Astrophys. J. 190, L35. doi:10.1086/181498
Florinski, V., Zank, G. P., Heerikhuisen, J., Hu, Q., and Khazanov, I. (2010). Stability of a pickup ion ring-beam population in the outer heliosheath: Implications for the IBEX Ribbon. Astrophys. J. 719, 1097–1103. doi:10.1088/0004-637x/719/2/1097
Frisch, P. C., Bzowski, M., Grün, E., Izmodenov, V., Kruger, H., Linsky, J. L., et al. (2009). The galactic environment of the Sun: Interstellar material inside and outside of the heliosphere. Space Sci. Rev. 146, 235–273. doi:10.1007/s11214-009-9502-0
Frisch, P. C., Piirola, V., Berdyugin, A. B., Heiles, C., Cole, A., Hill, K., et al. (2022). Whence the interstellar magnetic field shaping the heliosphere? Astrophys. J. Suppl. 259, 48. doi:10.3847/1538-4365/ac5750
Funsten, H. O., Guthrie, A. A., Harper, R. W., Dunn, G., Ellis, S., Everett, D., et al. (2009). The interstellar boundary explorer high energy (IBEX-Hi) neutral atom imager. Space Sci. Rev. 146, 75–103. doi:10.1007/s11214-009-9504-y
Fuselier, S. A., Ghielmetti, A. G., Hertzberg, E., Clark, G., Crew, G. B., Dunn, G., et al. (2009). The IBEX-lo sensor. Space Sci. Rev. 146, 117–147. doi:10.1007/s11214-009-9495-8
Galvin, A. B., Kistler, L., Popecki, M. A., Farrugia, C. J., Simunac, K. D. C., Ellis, L., et al. (2008). The plasma and suprathermal ion composition (plastic) investigation on the STEREO observatories. Space Sci. Rev. 136, 437–486. doi:10.1007/s11214-007-9296-x
Garcia-Munoz, M., Mason, G. M., and Simpson, J. A. (1973). A new test for solar modulation theory: The 1972 may-july low-energy galactic cosmic-ray proton and helium spectra. Astrophys. J. 182, L81–L84. doi:10.1086/181224
Gary, S. P., Hinata, S., Madland, C. D., and Winske, D. (1986). The development of shell-like distributions from newborn cometary ions. Geophys. Res. Lett. 13, 1364–1367. doi:10.1029/gl013i013p01364
Gloeckler, G., Fisk, L. A., and Geiss, J. (1997). Anomalously small magnetic field in the local interstellar cloud. Nature 386, 374–377. doi:10.1038/386374a0
Gloeckler, G., Fisk, L. A., Zurbuchen, T. H., and Schwadron, N. A. (2000). “Sources, injection and acceleration of heliospheric ion populations,” in ACE 2000 Symposium (Melville: AIP), 221–228.
Gloeckler, G., and Geiss, J. (1996). Abundance of 3He in the local interstellar cloud. Nature 381, 210–212. doi:10.1038/381210a0
Gloeckler, G., Galvin, A. B., Geiss, J., Balsiger, H., Balsiger, H., von Steiger, R., et al. (1993). Detection of interstellar pick-up hydrogen in the solar system. Science 261, 70–73. doi:10.1126/science.261.5117.70
Gloeckler, G., and Geiss, J. (2001). Heliospheric and interstellar phenomena deduced from pickup ion observations. Space Sci. Rev. 97, 169–181. doi:10.1023/a:1011867320416
Gloeckler, G., and Geiss, J. (1998). Interstellar and inner source pickup ions observed with SWICS on ULYSSES. Space Sci. Rev. 86, 127–159. doi:10.1023/a:1005019628054
Gloeckler, G., Geiss, J., Roelof, E. C., Fisk, L. A., Ipavich, F. M., Ogilvie, K. W., et al. (1994). Acceleration of interstellar pickup ions in the disturbed solar wind observed on Ulysses. J. Geophys. Res. 99, 17637–17644. doi:10.1029/94ja01509
Gloeckler, G., Schwadron, N. A., Fisk, L. A., and Geiss, J. (1995). Weak pitch angle scattering of few MV rigidity ions from measurements of anisotropies in the distribution function of interstellar pickup H+. Geophys. Res. Lett. 22, 2665–2668. doi:10.1029/95gl02480
Haerendel, G., Bauer, O. H., Ertl, M., Foppl, H., Kaiser, K. h., Lieb, W., et al. (1985). The Li/Ba release experiments of the lon release Module. IEEE Trans. Geosci. Remote Sens. GE-23, 253–258. doi:10.1109/tgrs.1985.289523
Häusler, B., Woolliscroft, L. J., Anderson, R. R., Gurnett, D. A., Holzworth, R. H., Koons, H. C., et al. (1986). Plasma waves observed by the IRM and UKS spacecraft during the AMPTE solar wind lithium releases: Overview. J. Geophys. Res. 91, 1283–1300. doi:10.1029/ja091ia02p01283
Heerikhuisen, J., Pogorelov, N. V., Zank, G. P., Crew, G. B., Frisch, P. C., Funsten, H. O., et al. (2010). Pick-up ions in the outer heliosheath: A possible mechanism for the interstellar boundary EXplorer Ribbon. Astrophys. J. 708, L126–L130. doi:10.1088/2041-8205/708/2/l126
Hovestadt, D., Gloeckler, G., Klecker, B., Scholer, M., and Ipavich, F. M. (1984). Survey of He+/He2+ abundance ratios in energetic particle events. Astrophys. J. 282, L39–L42. doi:10.1086/184300
Hovestadt, D., Hilchenbach, M., Bürgi, A., Klecker, B., Laeverenz, P., Scholer, M., et al. (1995). CELIAS - charge, element and isotope analysis system for SOHO. Sol. Phys. 162, 441–481. doi:10.1007/bf00733436
Hovestadt, D., Vollmer, O., Gloeckler, G., and Fan, C. Y. (1973). Differential energy spectra of low-energy (<8.5 MeV per nucleon) heavy cosmic rays during solar quiet times. Phys. Rev. Lett. 31, 650–653. doi:10.1103/physrevlett.31.650
Hsieh, K. C., and Möbius, E. (2022). Neutral-atom astronomy – plasma diagnostics from the aurora to the interstellar medium. Hackensack, NJ: World Scientific Publishing Co.
Isenberg, P. A. (1987). Evolution of interstellar pickup ions in the solar wind. J. Geophys. Res. 92, 1067–1074. doi:10.1029/ja092ia02p01067
Isenberg, P. A. (1986). Interaction of the solar wind with interstellar neutral hydrogen: Three-fluid model. J. Geophys. Res. 91, 9965–9972. doi:10.1029/ja091ia09p09965
Izmodenov, V. V., and Alexashov, D. B. (2020). Magnitude and direction of the local interstellar magnetic field inferred from Voyager 1 and 2 interstellar data and global heliospheric model. Astron. Astrophys. 633, L12. doi:10.1051/0004-6361/201937058
Izmodenov, V. V., Alexashov, D. B., and Myasnikov, A. V. (2005). Direction of the interstellar H atom inflow in the heliosphere: Role of the interstellar magnetic field. Astron. Astrophys. 437, L35–L38. doi:10.1051/0004-6361:200500132
Izmodenov, V. V., Malama, Y. G., Ruderman, M. S., Chalov, S. V., Alexashov, D. B., Katushkina, O. A., et al. (2009). Kinetic-gasdynamic modeling of the heliospheric interface. Space Sci. Rev. 146, 329–351. doi:10.1007/s11214-009-9528-3
Jokipii, J. R. (1998). Insights into cosmic-ray acceleration from the study of anomalous cosmic rays. Space Sci. Rev. 86, 161–178.
Klecker, B. (1995). The anomalous component of cosmic rays in the 3-D heliosphere. Space Sci. Rev. 72, 419–430. doi:10.1007/bf00768815
Krimigis, S . M., Haerendel, G., McEntire, R . W., Paschmann, G ., and Bryant, D. A. (1982). The active magnetospheric particle tracer Explorers (AMPTE) program. Eos Trans. AGU 63, 843. doi:10.1029/eo063i045p00843
Krimigis, S. M., Decker, R. B., Roelof, E. C., Hill, M. E., Armstrong, T. P., Gloeckler, G., et al. (2013). Search for the exit: Voyager 1 at heliosphere’s border with the galaxy. Science 341, 144–147. doi:10.1126/science.1235721
Krimigis, S. M., Mitchell, D. G., Roelof, E. C., Hsieh, K. C., and McComas, D. J. (2009). Imaging the interaction of the heliosphere with the interstellar medium from Saturn with Cassini. Science 326, 971–973. doi:10.1126/science.1181079
Kubiak, M. A., Bzowski, M., Sokòl, J. B., Swaczyna, P., Grzedzielski, S., Alexashov, D. B., et al. (2014). Warm breeze from the starboard bow: A new population of neutral helium in the heliosphere. Astrophys. J. Suppl. 213, 29. doi:10.1088/0067-0049/213/2/29
Kubiak, M. A., Swaczyna, P., Bzowski, M., Sokol, J. M., Fuselier, S. A., Galli, A., et al. (2016). Interstellar neutral helium in the heliosphere from IBEX observations. IV. Flow vector, Mach number, and abundance of the Warm Breeze. Astrophys. J. Suppl. 223, 25. doi:10.3847/0067-0049/223/2/25
Kucharek, H., Möbius, E., and Li, W. (2003). On the source and acceleration of energetic He+: A long-term observation with ACE/SEPICA. J. Geophys. Res. 108, 8040. doi:10.1029/2003ja009938
Lallement, R., Ferlet, R., Lagrange, A. M., Lemoine, M., and Vidal-Madjar, A. (1995). Local cloud structure from HST-GHRS. Astron. Astrophys. 304, 461–474.
Lallement, R., Quemerais, E., Bertaux, J. L., Ferron, S., Koutroumpa, D., and Pellinen, R. (2005). Deflection of the interstellar neutral hydrogen flow across the heliospheric interface. Science 307, 1447–1449. doi:10.1126/science.1107953
Lee, M. A., Kucharek, H., Möbius, E., Wu, X., Bzowski, M., and McComas, D. J. (2012). AN analytical MODEL of interstellar gas in the heliosphere tailored to interstellar boundary explorer observations. Astrophys. J. Suppl. 198, 10. doi:10.1088/0067-0049/198/2/10
Lee, M. A., Möbius, E., and Leonard, T. (2015). The analytical structure of the primary interstellar helium distribution function in the heliosphere. Astrophys. J. Suppl. 220, 23. doi:10.1088/0067-0049/220/2/23
Linsky, J. L., Redfield, S., Ryder, D., and Chasan-Taber, A. (2022). Inhomogeneity within local interstellar clouds. Astron. J. 164, 106. doi:10.3847/1538-3881/ac816b
McComas, D. J., Alexashov, D., Bzowski, M., Fahr, H., Heerikhuisen, J., Izmodenov, V., et al. (2012). The heliosphere’s interstellar interaction: No bow shock. Science 336, 1291–1293. doi:10.1126/science.1221054
McComas, D. J., Allegrini, F., Bochsler, B., Bzowski, M., Christian, E. R., Crew, G. B., et al. (2009). Global observations of the interstellar interaction from the interstellar boundary explorer (IBEX). Science 326, 959–962. doi:10.1126/science.1180906
McComas, D. J., Allegrini, F., Bochsler, P., Bzowski, M., Collier, M., Fahr, H., et al. (2009). IBEX - interstellar boundary explorer. Space Sci. Rev. 146, 11–33. doi:10.1007/s11214-009-9499-4
McComas, D. J., Christian, E. R., Schwadron, N. A., Fox, N., Westlake, J., Allegrini, F., et al. (2018). Interstellar mapping and acceleration Probe (IMAP): A new NASA mission. Space Sci. Rev. 214, 116. doi:10.1007/s11214-018-0550-1
McComas, D. J., Lewis, W. S., and Schwadron, N. A. (2014). IBEX’s Enigmatic Ribbon in the sky and its many possible sources. Rev. Geophys. 52, 118–155. doi:10.1002/2013rg000438
McNutt, R. L., Paul, M. V., and Brandt, P. C. (2021). Interstellar Probe – humanity’s journey to interstellar space. NASA Solar and Space Physics Mission Concept Study Report. Laurel, MD: Johns Hopkins Applied Physics Laboratory.
Möbius, E., Bochsler, P., Bzowski, M., Crew, G. B., Funsten, H. O., Fuselier, S. A., et al. (2009). Direct observations of interstellar H, He, and O by the interstellar boundary explorer. Science 326, 969–971. doi:10.1126/science.1180971
Möbius, E., Bochsler, P., Bzowski, M., Heirtzler, D., Kubiak, M. A., Kucharek, H., et al. (2012). Interstellar gas flow parameters derived from IBEX-Lo observations in 2009 and 2010: Analytical analysis. Astrophys. J. Suppl. 198, 11. doi:10.1088/0067-0049/198/2/11
Möbius, E., Bower, J., Aly, A., Berger, L., Farrugia, C., Galvin, A. B., et al. (2019). Observation of suprathermal tails of He+ pickup ions across solar wind compression regions with STEREO PLASTIC. J. Phys. Conf. Ser. 1332, 012011. doi:10.1088/1742-6596/1332/1/012011
Möbius, E., Bzowski, B., Chalov, S., Fahr, H. J., Gloeckler, G., Izmodenov, V., et al. (2004). Synopsis of the interstellar He parameters from combined neutral gas, pickup ion and UV scattering observations and related consequences. Astron. Astrophys. 426, 897–907. doi:10.1051/0004-6361:20035834
Möbius, E., Bzowski, M., Frisch, P. C., Fuselier, S. A., Heirtzler, D., Kubiak, M. A., et al. (2015). Interstellar flow and temperature determination with IBEX: Robustness and sensitivity to systematic effects. Astrophys. J. Suppl. 220, 24. doi:10.1088/0067-0049/220/2/24
Möbius, E., Gloeckler, G., Hovestadt, D., Ipavich, F. M., Klecker, B., Scholer, M., et al. (1985). The time-of-flight spectrometer SULEICA for ions of the energy range 5-270 keV/charge on AMPTE IRM. IEEE Trans. Geoscience Remote Sens. 23, 274–279. doi:10.1109/tgrs.1985.289527
Möbius, E., Hovestadt, D., Klecker, B., Scholer, M., Gloeckler, G., and Ipavich, F. M. (1985). Direct observation of He+ pick-up ions of interstellar origin in the solar wind. Nature 318, 426–429. doi:10.1038/318426a0
Möbius, E., Hovestadt, D., Klecker, B., Scholer, M., Gloeckler, G., Ipavich, F. M., et al. (1986). Observation of lithium pickup ions in the 5 to 20 keV energy range following the AMPTE solar wind releases. J. Geophys. Res. 91, 1325–1332. doi:10.1029/ja091ia02p01325
Möbius, E., Klecker, B., Bochsler, P., Gloeckler, G., Kucharek, K., Simunac, K., et al. (2010). “He pickup ions in the inner heliosphere-diagnostics of the local interstellar gas and of interplanetary conditions,” in Pickup ions throughout the heliosphere and beyond: Proc. of the 9th Ann. Int. Astrophys. Conf., AIP Conf. Proc., 37–43.
Möbius, E., Klecker, B., Hovestadt, D., and Scholer, M. (1988). Interaction of interstellar pick-up ions with the solar wind. Astrophys. Space Sci. 144, 487–505. doi:10.1007/bf00793200
Möbius, E., Kucharek, H., Clark, G., O’Neill, M., Petersen, L., Bzowski, M., et al. (2009). Diagnosing the neutral interstellar gas flow at 1 AU with IBEX-lo. Space Sci. Rev. 146, 149–172. doi:10.1007/s11214-009-9498-5
Möbius, E., Lee, M. A., and Drews, C. (2015). Interstellar flow longitude from the symmetry of the pickup ion cut-off at 1 AU. Astrophys. J. 815, 20. doi:10.1088/0004-637x/815/1/20
Möbius, E., Litvinenko, Y., Grünwaldt, H., Aellig, M. R., Bogdanov, A., Ipavich, F. M., et al. (1999). Direct evidence of the interstellar gas flow velocity in the pickup ion cut-off as observed with SOHO CELIAS CTOF. Geophys. Res. Lett. 26, 3181–3184. doi:10.1029/1999gl003644
Möbius, E., Morris, D., Popecki, M. A., Klecker, B., Kistler, L. M., and Galvin, A. B. (2002). Charge states of energetic (≈0.5 MeV/n) ions in corotating interaction regions at 1 AU and implications on source populations. Geophys. Res. Lett. 29, 1016. doi:10.1029/2001gl013410
Möbius, E., Rucinski, D., Hovestadt, D., and Klecker, B. (1995). The helium parameters of the very local interstellar medium as derived from the distribution of He+ pickup ions in the solar wind. Astron. Astrophys. 304, 505–519.
Möbius, E., Rucinski, D., Isenberg, P. A., and Lee, M. A. (1996). Determination of interstellar pickup ion distributions in the solar wind with SOHO and CLUSTER. Ann. Geophys 14, 492–496. doi:10.1007/s00585-996-0492-x
Möbius, E., Rucinski, D., Lee, M. A., and Isenberg, P. A. (1998). Decreases in the anti-sunward flux of interstellar pickup He+ associated with radial interplanetary magnetic field. J. Geophys. Res. 103, 257–265. doi:10.1029/97ja02771
Morris, D., Möbius, E., Lee, M. A., Popecki, M. A., Klecker, B., Kistler, L. M., et al. (2001). Implications for source populations of energetic ions in Co-rotating interaction regions from ionic charge states. In: Solar and galactic composition. AIP Conf. Proc. 598, 201.
Opher, M., Loeb, A., Drake, J., and Toth, G. (2020). A small and round heliosphere suggested by magnetohydrodynamic modelling of pick-up ions. Nat. Astron. 4, 675–683. doi:10.1038/s41550-020-1036-0
Opher, M., Richardson, J. D., Toth, G., and Gombosi, T. I. (2009). Confronting observations and modeling: The role of the interstellar magnetic field in voyager 1 and 2 asymmetries. Space Sci. Rev. 143, 43–55. doi:10.1007/s11214-008-9453-x
Opher, M., Stone, E. C., and Liewer, P. C. (2006). The effects of a local interstellar magnetic field on voyager 1 and 2 observations. Astrophys. J. 640, L71–L74. doi:10.1086/503251
Park, J., Kucharek, H., Möbius, E., Galli, A., Kubiak, M. A., Bzowski, M., et al. (2016). IBEX observations of secondary interstellar helium and oxygen distributions. Astrophys. J. 833, 130. doi:10.3847/1538-4357/833/2/130
Park, J., Kucharek, H., Möbius, E., Galli, A., Livadiotis, G., Fuselier, S. A., et al. (2015). Statistical analysis of the heavy neutral atoms measured by IBEX. Astrophys. J. Suppl. 220, 34. doi:10.1088/0067-0049/220/2/34
Park, J., Kucharek, H., Möbius, E., Leonard, T., Bzowski, M., Sokol, J. M., et al. (2014). The Ne to O abundance ratio of the interstellar medium from IBEX-Lo observations. Astrophys. J. 795, 97. doi:10.1088/0004-637x/795/1/97
Park, J., Kucharek, H., Paschalidis, J., Szabo, A., Heirtzler, D., Mobius, E., et al. (2019). The characterization of secondary interstellar neutral oxygen beyond the heliopause: A detailed analysis of the IBEX-lo oxygen observations. Astrophys. J. 880, 4. doi:10.3847/1538-4357/ab264a
Pogorelov, N. V., Borikov, S. N., Heerikhuisen, J., and Zhang, M. (2015). Heliotail. Astrophys. J. Lett. 812, L6. doi:10.1088/2041-8205/812/1/l6
Pogorelov, N. V., Borovikov, S. N., Zank, G. P., and Ogino, T. (2009). Three-dimensional features of the outer heliosphere due to coupling between the interstellar and interplanetary magnetic fields. III. The effects of solar rotation and activity cycle. Astrophys. J. 696, 1478–1490. doi:10.1088/0004-637x/696/2/1478
Pogorelov, N. V., Heerikhuisen, J., Zank, G. P., and Borikov, S. N. (2009). Influence of the interstellar magnetic field and neutrals on the shape of the outer heliosphere. Space Sci. Rev. 143, 31–42. doi:10.1007/s11214-008-9429-x
Prantzos, N. (1998). “The chemical evolution of the Milky Way disk,” in Primordial nuclei and their galactic evolution. Editors N. Prantzos, M. Tosi, and R. von Steiger (Bern, Switzerland: Space Science Series of ISSI), 225.
Quinn, P., Schwadron, N. A., Möbius, E., Gorbi, M., and McComas, D. J. (2016). Transport of helium pickup ions within the focusing cone: Reconciling STEREO observations with IBEX. Astrophys. J. 824, 142. doi:10.3847/0004-637x/824/2/142
Rahmanifard, F., Möbius, E., Schwadron, N. A., Galli, A., Richards, N., Kucharek, H., et al. (2019). Radiation pressure from interstellar hydrogen observed by IBEX through solar cycle 24. Astrophys. J. 887, 217. doi:10.3847/1538-4357/ab58ce
Redfield, S., and Linsky, J. (2008). The structure of the local interstellar medium. IV. Dynamics, morphology, physical properties, and implications of cloud-cloud interactions. Astrophys. J. 673, 283–314. doi:10.1086/524002
Rodriguez-Moreno, D. F., Wurz, P., Saul, L., Bzowski, M., Kubiak, M. A., Sokol, J. M., et al. (2013). Evidence of direct detection of interstellar deuterium in the local interstellar medium by IBEX. Astron. & Astrophys. 557, A125. doi:10.1051/0004-6361/201321420
Schramm, D. N. (1998). “Big Bang nucleosynthesis and the density of baryons in the universe,” in Primordial nuclei and their galactic evolution. Editors N. Prantzos, M. Tosi, and R. von Steiger (Bern, Switzerland: Space Science Series of ISSI), 3.
Schwadron, N. A., Bzowski, M., Crew, G. B., Gruntman, M., Fahr, H., Fichtner, H., et al. (2009). Comparison of interstellar boundary explorer observations with 3D global heliospheric models. Science 326, 966–968. doi:10.1126/science.1180986
Schwadron, N. A., Bzowski, M., McComas, D. J., and Moebius, E. (2018). The local interstellar magnetic field observed by voyager 1 and IBEX. J. Phys. Conf. Ser. 1100, 012021. doi:10.1088/1742-6596/1100/1/012021
Schwadron, N. A., and Bzowski, M. (2018). The heliosphere is not round. Astrophys. J. 862, 11. doi:10.3847/1538-4357/aacbcf
Schwadron, N. A., Frisch, P. C., Adams, F. R., Christian, E. R., Desiati, P., Funsten, H. O., et al. (2015). A consistent scenario for the IBEX Ribbon, anisotropies in TeV cosmic rays, and the local interstellar medium. ASTRA Proc. 2, 9–16. doi:10.5194/ap-2-9-2015
Schwadron, N. A., Möbius, E., Lee, M. A., Bochsler, P., Bzowski, M., Fuselier, S. A., et al. (2016). Determination of interstellar O parameters using the first two years of data from theinterstellar boundary explorer. Astrophys. J. 828, 81. doi:10.3847/0004-637x/828/2/81
Schwadron, N. A., Möbius, E., Leonard, T., Fuselier, S. A., McComas, D. J., Heirtzler, D., et al. (2015). Determination of interstellar He parameters using 5 years of data from the Interstellar Boundary Explorer–Beyond closed form approximations. Astrophys. J. Suppl. 220, 25. doi:10.1088/0067-0049/220/2/25
Schwadron, N. A., Richardson, J., Burlaga, L., McComas, D. J., and Moebius, E. (2015). Triangulation of the interstellar magnetic field using voyager 1, SOHO/SWAN and IBEX. Astrophys. J. Lett. 813, L20. doi:10.1088/2041-8205/813/1/l20
Slavin, J. D., and Frisch, P. C. (2008). The boundary conditions of the heliosphere: Photoionization models constrained by interstellar and in situ data. Astron. Astrophys. 491, 53–68. doi:10.1051/0004-6361:20078101
Sokól, J. M., Bzowski, M., Kubiak, M. A., and Möbius, E. (2016). Solar cycle variation of interstellar neutral He, Ne, O density and pickup ions along the Earth’s orbit. MNRAS 458, 3691–3704. doi:10.1093/mnras/stw515
Stone, E. C., Cummings, A. C., McDonald, F. B., Heikkila, B. C., Lal, N., and Webber, W. R. (2008). An asymmetric solar wind termination shock. Nature 454, 71–74. doi:10.1038/nature07022
Stone, E. C., Cummings, A. C., McDonald, F. B., Heikkila, B. C., Lal, N., and Webber, W. R. (2013). Voyager 1 observes low-energy galactic cosmic rays in a region depleted of heliospheric ions. Science 341, 150–153. doi:10.1126/science.1236408
Strömgren, B. (1939). The physical state of interstellar hydrogen. Astrophys. J. 89, 526–547. doi:10.1086/144074
Swaczyna, P., Bzowski, M., Kubiak, M. A., Sokol, J. M., Fuselier, S. A., Galli, A., et al. (2018). Interstellar neutral helium in the heliosphere from IBEX observations. V. Observations in IBEX-lo ESA steps 1, 2, and 3. Astrophys. J. 854, 119. doi:10.3847/1538-4357/aaabbf
Swaczyna, P., Bzowski, M., Kubiak, M. A., Sokol, J. M., Fuselier, S. A., Heirtzler, D., et al. (2015). Interstellar neutral helium in the heliosphere from IBEX observations. I. Uncertainties and backgrounds in the data and parameter determination method. Astrophys. J. Suppl. 220, 26. doi:10.1088/0067-0049/220/2/26
Swaczyna, P., Kubiak, M. A., Bzowski, M., Bower, J., Fuselier, S. A., Galli, A., et al. (2022). Very local interstellar medium revealed by a complete solar cycle of interstellar neutral helium observations with IBEX. Astrophys. J. Suppl. 259, 42. doi:10.3847/1538-4365/ac4bde
Swaczyna, P., McComas, D. J., Zirnstein, E. J., Sokol, J. M., Elliott, H. A., Bzowski, M., et al. (2020). Density of neutral hydrogen in the Sunʼs interstellar neighborhood. Astrophys. J. 903, 48. doi:10.3847/1538-4357/abb80a
Swaczyna, P., Schwadron, N. A., Möbius, E., Bzowski, M., Frisch, P. C., Linsky, J. L., et al. (2022). Mixing interstellar clouds surrounding the Sun. Astrophys. J. Lett. 937, L32. doi:10.3847/2041-8213/ac9120
Taut, A., Berger, L., Drews, C., Morris, E., Heidrich-Meisner, V., Keilbach, D., et al. (2018). Challenges in the determination of the interstellar flow longitude from the pickup ion cutoff. Astron. Astrophys. 611, A61. doi:10.1051/0004-6361/201731796
Vasyliunas, V. M., and Siscoe, G. L. (1976). On the flux and the energy spectrum of interstellar ions in the solar system. J. Geophys. Res. 81, 1247–1252. doi:10.1029/ja081i007p01247
Weller, C. S., and Meier, R. R. (1974). Observations of helium in the interplanetary/interstellar wind: The solar-wake effect. Astrophys. J. 193, 471–476. doi:10.1086/153182
Witte, M., Banaszkiewicz, M., and Rosenbauer, M. (1996). Recent results on the parameters of the interstellar helium from the ULYSSES/GAS experiment. Space Sci. Rev. 78, 289–296. doi:10.1007/bf00170815
Witte, M. (2004). Kinetic parameters of interstellar neutral helium. Review of results obtained during one solar cycle with the Ulysses/GAS-instrument. Astron. Astrophys. 426, 835–844. doi:10.1051/0004-6361:20035956
Witte, M., Rosenbauer, M., Keppler, E., Keppler, E., Fahr, H., Hemmerich, P., et al. (1992). The interstellar neutral-gas experiment on Ulysses. Astron. Astrophys. Suppl. Ser. 92, 333–348.
Wood, B., Müller, H-R., and Witte, M. (2015). Revisiting Ulysses observations of interstellar helium. Astrophys. J. 801, 62. doi:10.1088/0004-637x/801/1/62
Wood, B. E., Müller, H. R., and Möbius, E. (2019). Evidence for asymmetry in the velocity distribution of the interstellar neutral helium flow observed by IBEX and Ulysses. Astrophys. J. 881, 55. doi:10.3847/1538-4357/ab2e74
Wu, F. M., and Judge, D. L. (1979). Temperature and flow velocity of the interplanetary gases along solar radii. Astrophys. J. 231, 594–605. doi:10.1086/157221
Zirnstein, E. J., Heerikhuisen, J., Funsten, H. O., Livadiotis, G., McComas, D. J., and Pogorelov, N. V. (2016). Local interstellar magnetic field determined from the interstellar boundary explorer Ribbon. Astrophys. J. Lett. 818, L18. doi:10.3847/2041-8205/818/1/l18
Keywords: pickup ions, interstellar gas flow, interstellar magnetic field, interstellar gas composition, energetic neutral atoms, heliosphere boundary
Citation: Möbius E (2023) How catching the interstellar wind in the inner solar system led the way on a road to interdisciplinary research between heliophysics and astrophysics. Front. Astron. Space Sci. 10:1020921. doi: 10.3389/fspas.2023.1020921
Received: 16 August 2022; Accepted: 23 March 2023;
Published: 12 April 2023.
Edited by:
Joseph E. Borovsky, Space Science Institute, United StatesReviewed by:
Merav Opher, Boston University, United StatesCopyright © 2023 Möbius. This is an open-access article distributed under the terms of the Creative Commons Attribution License (CC BY). The use, distribution or reproduction in other forums is permitted, provided the original author(s) and the copyright owner(s) are credited and that the original publication in this journal is cited, in accordance with accepted academic practice. No use, distribution or reproduction is permitted which does not comply with these terms.
*Correspondence: Eberhard Möbius, eberhard.moebius@unh.edu