- Department of Physics, University of Alberta, Edmonton, AB, Canada
Frameworks for describing magnetospheric substorms are as good as the data on which they are based. While disturbances in the ionosphere are well described by existing data bases, the plasma and field data acquired by satellites in the magnetosphere are sparse and unable to lend an element of uniqueness to any model for substorm activity. This paper describes the voyage of discovery experienced by the author from the leadup to his career to the time of his retirement. Perhaps it will provide young scientists with a sense of how space physics developed and what needs to be done before the solar wind-magnetosphere-ionosphere interaction becomes fully understood.
A bit of my history and how I fell into space science
I grew up in Toronto starting my schooling in the mid 1940s. I was not a particularly good student, and I was probably more interested in playing competitive chess than in the subjects I was taught in school. Nonetheless, I believe what I learned playing chess probably was at least as important as what was taught in school. In my work in space physics, the ability to visualize complex scenes in three dimensions and to think ahead several moves was a distinct asset. When I arrived in university, I chose to enter MPC (Math, Physics and Chemistry) at the University of Toronto. It seemed a reasonable thing to try, since my three older brothers all had gone through university obtaining their doctorates in the physical sciences. I was, at best, a mediocre student for the first 3 years. Then a stroke of luck changed everything. I got to work in a scientific lab for the first time during the summer between my third and fourth year, building equipment and getting involved with one of the first brain scanning machines. I finished my last year with a flourish that got me into Graduate School. For my M. Sc., I set up an array of magnetometers to study the subsurface conductivity across southern Ontario. I was supposed to be studying the induced electric currents in the Earth, but I wondered what was inducing those currents. I spent many hours at the Agincourt Magnetic Observatory looking at magnetograms, and after a while I began to see patterns. I was actually looking at the magnetic signatures of what are now called substorms—in those days they were called magnetic bays. When I was persuaded to go the University of British Columbia for my doctorate studying perturbations in the Earth’s magnetic field, I was already hooked. That is how I fell into Space Physics as a career.
After completing my Ph.D. in 1966 studying geomagnetic pulsations, I spent a postdoctoral period at the Royal Institute of Technology in Stockholm, Sweden attracted there by the presence of Hannes Alfvén. There I had the opportunity to work with some of the first interplanetary electric field data, establish a great working relationship with Rolf Boström, and learn another language (Swedish). Little did I know, but the market for university academics was closing down rapidly as I was doing my postdoctoral studies. I was fortunate to be recruited by Professor Jack Jacobs (my department head at UBC who had moved to University of Alberta to assume an endowed Chair). And that is how, in 1968, I started my academic career.
I knew exactly what I wanted to do at that point in time—to set up a line of magnetometers spanning the average position of the auroral oval and monitor the development of the auroral electrojets. What those data did for me, other than allowing me to learn a lot about geomagnetic disturbances, was to provide an entry into the world of scientists who were studying those same disturbances using satellite data. The balance of my career was spent cooperating with colleagues using my ground-based data to help them know what was happening in the magnetosphere when they were looking at their data.
There are two papers that I published during my career which, in retrospect, are a source of pride. The first was co-authored with Rolf Boström (cf. Rostoker and Boström, 1976) stemming from work done during my first sabbatical at the Royal Institute of Technology. It came as a consequence of me sitting in a nearly empty office for some weeks contemplating the three-dimensional structure of the magnetotail as it pertained to the electric field configuration. I was responsible for the geometry of the situation and Rolf Boström was responsible for the mathematical formalisms. One of the referees commented that he didn’t believe it, but he couldn’t find anything wrong with it. You can’t ask for more than that! The second paper was a review of substorm phenomenology along with my framework for the processes that are responsible for substorms (Rostoker, 1996). In Figure 8 of that paper, I showed the development of an auroral substorm as projected on the ionosphere. This differed from the original Akasofu picture (Akasofu, 1964) in that it showed the full development of a substorm in the context of the changes in the interplanetary magnetic field. It emphasized the presence of two distinct regions of activity—the equatorward portion of the auroral oval where substorm activity initiates and the poleward portion where auroral surge forms develop (see Figure 1). It turns out that a substorm expansion phase onset on the equatorward branch of the midnight sector auroral oval looks very much like the development of a surge structure on the poleward branch of the oval in terms of their magnetic signature on the ground—a sharp negative perturbation in the north-south component of the magnetic field. It is all too easy to take the magnetic signature of a surge and misinterpret it as an expansion phase onset.
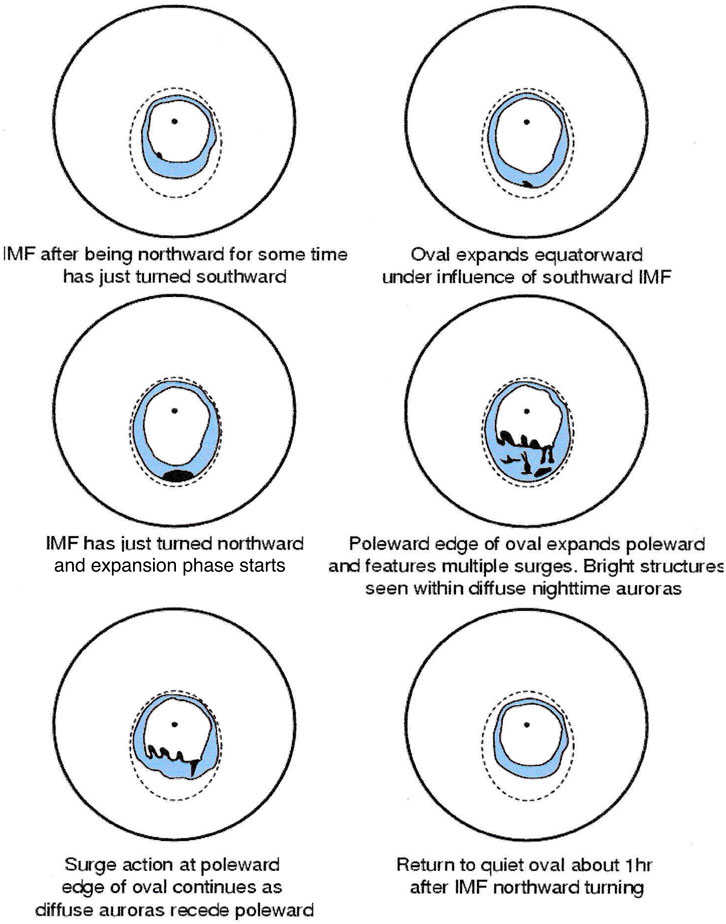
FIGURE 1. Evolution of the auroral signatures during a magnetospheric substorm. Note that this differs from the classic Akasofu auroral substorm in that his substorm features equatorward drifting auroral arcs during his recovery phase whereas this picture features a poleward contracting oval during recovery. Note also that one can have localized auroral forms develop on the poleward branch of the oval during quiet times and pseudo breakups develop on the equatorward branch of the oval in advance of the expansion phase onset. The poleward expansion of the region of discrete auroral arcs during the development of the expansion phase occurs in steps rather than smoothly (Kisabeth and Rostoker, 1974). [modified after Rostoker, 2007].
What I learned during my career
After entering the field of space physics, I watched battles develop in which more than one possible explanation presented itself for observed phenomena. In all these cases, the available data were inadequate to allow one to distinguish between competing models. Over the course of my career, I saw three of these dilemmas resolved when key data became available.
The first of these was a battle between Sidney Chapman (cf. Vestine and Chapman, 1938), who believed that magnetic field disturbances detected at Earth’s surface were caused by ionospheric currents alone, and Hannes Alfvén (cf. Alfven, 1940) who believed that those magnetic field disturbances were caused by a combination of field-aligned and ionospheric currents after the idea of Kristian Birkeland (c.f. Birkeland, 1913). Chapman based his claim on the fact that, in the collisionless plasma above the ionosphere, the conductivity along field lines was infinite and hence any electric field would drive an infinite current. When Alfred Zmuda and others (c.f. Zmuda et al., 1966) flew a magnetometer aboard a polar orbiting satellite hundreds of kilometers above the ionosphere, it detected the unmistakable magnetic signatures of field-aligned currents and Alfvén was proved to have been correct thanks to the right data becoming available.
A second such dilemma arose when the first satellite to sample the interplanetary medium (IMP-1) in the late 1960s detected magnetic field pointing towards the Sun for some days, then away from the Sun for some days, then towards the Sun for some days and then away from the Sun for some days. The pattern had an ∼ 27- day periodicity, so it was suggested by John Wilcox (cf. Wilcox and Ness, 1965) that there were sectors on the Sun in which the field pointed alternately away from or towards the solar surface. He called this the sector structure and initially this was believed to be the explanation of the observations. A few years later Michael Schulz (cf. Schulz, 1973) proposed that the observations could be explained if there was a wavy current sheet near the ecliptic plane, and sometimes the satellite was above the current sheet and sometimes it was below. The Wilcox viewpoint prevailed until serendipity intervened when the Pioneer 11 satellite, after reaching Jupiter, was slung out of the ecliptic plane on its way to Saturn. When Pioneer got to about 16° above the ecliptic plane, the sector structure disappeared and until the satellite moved back towards the ecliptic plane, the magnetic field pointed in one direction (cf. Smith et al., 1978). It was immediately apparent that Schulz had been correct, but it took the right data to distinguish between the two explanations.
A third such dilemma centered on the cause of geomagnetic storms. From the time of the observation of a giant solar flare in 1869 by Carrington, it was believed that geomagnetic storms were caused by solar flares. Until the early 1990s, efforts were made to clearly establish this causal relationship. At NOAA, Jo Anne Joselyn and her group worked intensely on this problem but were frustrated by the fact that sometimes geomagnetic storms occurred with no apparent flare to blame, and sometimes there were major flares but no ensuing geomagnetic storms (cf. Joselyn, 1995). It was not until Jack Gosling at Los Alamos National Laboratory used Skylab data to reveal that coronal mass ejections (CME’s) were to blame for geomagnetic storms that flares were abandoned as the causal agent (cf. Gosling, 1993). Until that time, the presence of coronal mass ejections could not easily be established because the observations from the ground were simply not up to the task of detecting CME’s.
My career was dominated over the years by the study of magnetospheric substorms, which involved working with ground-based magnetometer data on which the definition of the substorm was based in part. To understand the physical processes responsible for substorms, it was necessary to have observations in space of magnetic fields, plasmas and electric fields. While such measurements were essential to follow the development of substorms in space, the number of observation points at any one time was woefully inadequate to provide unique solutions to the physical phenomena involved with the substorm process. In the early stages of my career, space-based data were sparse. By the time I retired, the vastly increased amount of data was surprisingly still inadequate in terms of a multipoint set of observations that would provide a clear physical insight to the problem. Two issues were paramount. The first involved the ability to know whether a disturbance was temporal in nature or reflected the passage of plasma and field structures past the observation points. To this day, researchers assume they are dealing with temporal changes despite the fact that evidence dating back to the beginning of the 1970s indicated that plasma structures could sweep past a spacecraft leading to measurements that could incorrectly be attributed to a temporal change. The second issue centered around being able to map along magnetic field lines from the ionosphere to points in the magnetosphere; this involves being able to model volume filling electric currents whose perturbation magnetic fields add to the background geomagnetic field (Donovan, 1993). Between the antiparallel current sheets, the magnetic field lines are skewed towards the flanks of the magnetosphere (see Figure 2). While ionospheric disturbances nowadays can often have their locations precisely defined, if one does not know where in the magnetosphere these disturbances (e.g., auroral forms) map to, one cannot be confident about the physics of the processes that led to these disturbances. It remains for future space scientists to address these issues and to not accept, without question, existing paradigms in this field of research.
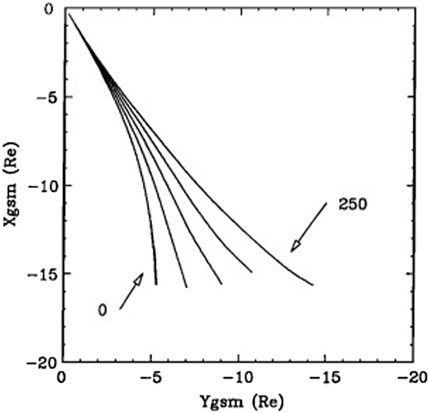
FIGURE 2. Projections in the XYgsm plane of field lines traced using the T87 Kp = 2 model modified to include the magnetic effects of field-aligned and closure currents. The volume filling field-aligned currents stretch across 5 degrees of latitude with downward field aligned current equatorward of 69.45° and upward field-aligned current poleward of that latitude. The five field lines shown here were traced with amounts of field-aligned current added that would produce eastward perturbations of the magnetic field at 800 km altitude of 0 nT, 62.5 nT, 125 nT, 187.5 and 250 nT (0 nT corresponds to no field-aligned current added.) The field lines all have an ionospheric footprint 2 hours from local midnight midway between the current sheets and terminate in the equatorial plane (after Donovan, 1993).
What you can look forward to during a career in space science (or any science, for that matter)
While I was spending 2 years on Faculty at the Solar-Terrestrial Environment Laboratory of Nagoya University in Japan a couple of years after my retirement from the University of Alberta in 1997, I was asked to give a talk on what it takes to become a successful scientist. What I told them can be summarized in three points:
1 Successful scientists are persistent in doing what they want to do regardless of what others think.
2 Successful scientists must be creative and believe in themselves. What they discover will likely disagree with what is the current belief, and a scientist must be prepared to disagree publicly with famous people with gray hair who have written the “bible” in their area of research.
3 A successful scientist must be able to communicate very effectively in both the spoken and written language. Some researchers find it hard to write up what they have done, although they love doing the research. Some researchers give talks in a manner which puts the audience to sleep in a manner of minutes. Successful scientists are usually very animated speakers who know just how much information they can provide in the time allotted.
Some final thoughts
I was fortunate to have the opportunity to work in a real laboratory prior to my final year of undergraduate studies. That provided the motivation to propel me into graduate school. That I ended up in space science was a matter of luck—it was either biophysics or geophysics and, as it turned out, the head of Geophysics in Toronto (J. Tuzo Wilson) admitted me to graduate studies. It was good fortune that put me in front of years of magnetograms from Agincourt Magnetic Observatory; that allowed me to recognize patterns in disturbances which turned out to be the signatures of the substorms that I ended up studying for the rest of my career. If you are a young scientist reading this, keep in mind how much luck is involved in finding your final area of research. The rest is hard work, although it can be a lot of fun and very rewarding!
I learned during my career that, to provide a unique solution to aspects of the physical processes that take place in the solar wind-magnetosphere-ionosphere system, one must have adequate data. The human mind is very creative and can think of more than one mechanism that satisfies the available data. As I have pointed out earlier, even during my time in space science, I could identify three instances in which more than one explanation existed to explain a set of observations which was resolved when new data became available that allowed one to identify the correct explanation.
Despite the vast amount of data that has been accumulated in situ observations of the solar wind-magnetosphere-ionosphere system, it has still been grossly inadequate to resolve issues that are still outstanding. In fact, the present paradigm for explaining how magnetic field line reconnection in the magnetotail leads to auroras and electric current flows in the ionosphere and magnetosphere is still very fragile and does not account for the fact that there are two separate regions of space in which auroral brightenings take place—the equatorward edge of the midnight sector auroral oval where substorm expansion phases are initiated and the poleward edge where auroral surge phenomena are initiated. When mapping from the ionosphere to the magnetotail along magnetic field lines during significant levels of activity becomes possible, that will be an important step towards answering many of the questions that were left unanswered when I retired from the field.
Data availability statement
The original contributions presented in the study are included in the article/supplementary material, further inquiries can be directed to the corresponding author.
Author contributions
The author performed all research on this perspective and wrote the manuscript.
Acknowledgments
The author is forever grateful to W. Kerr who gave me an opportunity to work in a science lab as an undergraduate student, to Professor Jack Jacobs who found a faculty position for me at the University of Alberta and helped me launch my career, and to the graduate students and postdoctoral associates who helped me in my exploration of magnetic pulsations and magnetospheric substorms. The author is grateful to the Natural Sciences and Engineering Research Council of Canada for support of his research over the years.
Conflict of interest
The author declares that the research was conducted in the absence of any commercial or financial relationships that could be construed as a potential conflict of interest.
Publisher’s note
All claims expressed in this article are solely those of the authors and do not necessarily represent those of their affiliated organizations, or those of the publisher, the editors and the reviewers. Any product that may be evaluated in this article, or claim that may be made by its manufacturer, is not guaranteed or endorsed by the publisher.
References
Akasofu, S.-I. (1964). The development of the auroral substorm. Planet. Space Sci. 12, 273–282. doi:10.1016/0032-0633(64)90151-5
Alfven, H. (1940). A theory of magnetic storms and of the aurorae; 2. The aurorae; 3. The magnetic disturbances. Stockholm: Almqvist & Wiksell. Kungl. Sv. Vetensk.-Akad. Handlingar III, 18, No. 9, Stockholm.
Birkeland, K. (1913). The Norwegian aurora polaris expedition, 1902-1903, 1. Christiania: H. Aschelhoug & Company. Second Section.
Donovan, E. (1993). Modeling the magnetic effects of field-aligned currents. J. Geophys. Res. 98 (13), 13529–13543. doi:10.1029/93ja00603
Gosling, J. T. (1993). The solar flare myth. J. Geophys. Res. 98 (18), 18937–18949. doi:10.1029/93ja01896
Joselyn, Jo Ann (1995). Geomagnetic activity forecasting: The state of the art. Rev. Geophys. 33 (3), 383. doi:10.1029/95rg01304
Kisabeth, J. L., and Rostoker, G. (1974). The expansive phase of magnetospheric substorms: 1. Development of the auroral electrojets and auroral arc configuration during a substorm. J. Geophys. Res. 79, 972–984. doi:10.1029/ja079i007p00972
Rostoker, G., and Boström, R. (1976). A mechanism for driving the gross Birkeland current configuration in the auroral oval. J. Geophys. Res. 81, 235–244. doi:10.1029/ja081i001p00235
Rostoker, G. (1996). Phenomenology and physics of magnetospheric substorms. J. Geophys. Res. 101 (12), 12955–12973. doi:10.1029/96ja00127
Rostoker, G. (2007). “Substorms,” in Handbook of the solar-terrestrial environment. Editors By Y. Kamide, and A. Chian (Springer-Verlag Berlin Heidelberg), 375–395.
Schulz, M. (1973). Interplanetary sector structure and the helliomagnetic equator. Astrophys. Space Sci. 24, 371–383. doi:10.1007/bf02637162
Smith, E. J., T Tsurutani, B., and Rosenberg, R. L. (1978). Observations of the interplanetary sector structure up to heliographic latitudes of 16o: Pioneer 11. J. Geophys. Res. 83, 717. doi:10.1029/ja083ia02p00717
Vestine, E. H., and Chapman, S. (1938). The electric current system of geomagnetic disturbance. J. Geophys. Res. 43, 351. doi:10.1029/te043i004p00351
Wilcox, J. M., and Ness, N. F. (1965). Quasi-stationary corotating structure in the interplanetary medium. J. Geophys. Res. 70, 5793–5805. doi:10.1029/jz070i023p05793
Keywords: substorms, aurora, magnetic field, interplanetary magnetic field, magnetotail
Citation: Rostoker G (2022) Lessons from a career in space physics. Front. Astron. Space Sci. 9:912036. doi: 10.3389/fspas.2022.912036
Received: 03 April 2022; Accepted: 26 July 2022;
Published: 17 August 2022.
Edited by:
Joseph E. Borovsky, Space Science Institute, United StatesReviewed by:
Christine Gabrielse, The Aerospace Corporation, United StatesRobert McPherron, California State University, Los Angeles, United States
Copyright © 2022 Rostoker. This is an open-access article distributed under the terms of the Creative Commons Attribution License (CC BY). The use, distribution or reproduction in other forums is permitted, provided the original author(s) and the copyright owner(s) are credited and that the original publication in this journal is cited, in accordance with accepted academic practice. No use, distribution or reproduction is permitted which does not comply with these terms.
*Correspondence: Gordon Rostoker, Z3JAdWFsYmVydGEuY2E=