- 1Dipartimento di Fisica, Università degli Studi di Roma “Tor Vergata”, Roma, Italy
- 2INAF-IAPS, Istituto di Astrofisica e Planetologia Spaziali, Roma, Italy
- 3INAF-Osservatorio Astrofisico di Catania, Catania, Italy
We present a new synergic strategy that merges the potential of asteroseismology with solar space weather/climate techniques in order to characterize solar-like stars and their interaction with hosted exoplanets. The method is based on the use of seismic data obtained by the space missions Kepler/K2 and TESS Transiting Exoplanet Survey Satellite, coupled with stellar activity estimates deduced from ground-based campaigns (e.g., Mount Wilson Observatory HK Project). Our investigation allows us to determine not only highly accurate fundamental parameters of the mother star and its orbiting planet, but also to study the stellar magnetic activity and the star-planet interaction: in analogy to the Sun-Earth system, it is possible to infer the mean stellar wind acting on the exoplanet in order to define the conditions of the exoplanetary environment and the erosion of its atmosphere with an impact on the habitability of the planet.
1 Introduction
The high–precision and continuous photometric measurements performed by space missions, such as CoRoT (Baglin et al., 2006), Kepler/K2 (Borucki et al., 2010) and TESS (Ricker et al., 2014), have not only allowed to discover thousands of exoplanets in orbit around solar-like stars, but also to disclose the structural and dynamical properties of many of the detected stars, including their evolutionary state, by applying the methods of asteroseismology. However, stand-alone photometry is a limited tool for the characterization of exoplanetary systems. The combination of different approaches, both theoretical and observational, are needed in order to really progress in this field.
As we have learnt from our Solar system, the main physical parameters of a planet and its equilibrium temperature are not sufficient to characterize the habitability conditions. An accurate knowledge of the fundamental parameters of the host-star is crucial for the interpretation of the detection of an exoplanet, the study of its structure and its evolution. Moreover, a necessary other ingredient for a complete characterization is a detailed assessment of the interaction between host-star and its planets (Airapetian et al., 2020). Indeed, manifestations of stellar activity in the form of coronal mass ejections, energetic particles, stellar flares and winds, as well as an high stellar flux in particular wavelength ranges (e.g., UV, X-ray), can strongly affect the planetary environment. Extreme space weather conditions, such as those created by frequent flares and coronal mass ejections from active stars and young Sun, may profoundly affect the chemistry and climate of terrestrial type exoplanets (see e.g., Cohen et al., 2015; Dong et al., 2018).
The strategy that we propose here brings together asteroseismic tools and space-weather/space-climate techniques, to characterize host Sun-like stars and the way they influence the environment around exoplanets. In particular, by analyzing the photometric measurements provided by space missions and by making use of asteroseismic techniques, we are able to obtain the main stellar parameters with high precision, allowing to have an accurate characterization of host stars. On the other side, stellar activity measurements from ground-based observatory allow us, by making use of a model that we calibrated on the Sun-Earth system, to estimate the stellar wind pressure acting on exoplanets, and hence to evaluate the effects on their magnetosphere.
The stellar targets to which apply this strategy include main-sequence solar-like stars hosting, possibly rocky, exoplanets, for which both asteroseismic and magnetic activity observations are available. Considering the fact that we wish to extend to other stars the techniques developed for the Sun, it is important to clarify that low mass, intermediate age stars of spectral type G, should represent the best targets for our study. The only limit to the application of the present strategy is represented by the fact that presence of enhanced magnetic activity might be responsible for suppression of solar-like oscillations as already found in several targets (e.g., García et al., 2010; Chaplin et al., 2011; Mathur et al., 2019).
2 Stellar Characterization With Asteroseismology
During the last decades striking results have been obtained for several hundreds of cool main-sequence, post main-sequence and red-giant stars which exhibit solar-like pulsations, as in the Sun, excited by near-surface turbulent convection. In fact, solar-like pulsations provide the unique opportunity to study in details the internal structure and rotation of the stars and to put very stringent constraints on the fundamental stellar parameters. Accuracy of the order of few % in the determination of the mass and the radius and less than 10% in the age, much higher than with any other method, have been reached by the use of asteroseismic techniques (see e.g., Metcalfe et al., 2010; Mathur et al., 2012) in combination with measurements of chemical composition, position, distance and velocity obtained by large spectroscopic surveys (e.g., GAIA Gaia Collaboration et al., 2016).
Any asteroseismic study starts from the detection and identification of the pulsations properties of a star, deduced by a careful analysis of the oscillation spectrum obtained by photometric observations. Then, by the application of well developed techniques and the use of state-of-the-art stellar structure models built with update evolutionary codes (e.g., Christensen-Dalsgaard, 2008b), it is possible to obtain the stellar fundamental parameters and other details of a star with detected solar-like pulsations.
2.1 Data Analysis
In order to analyze stellar oscillations we require that a power spectral density (PSD) is computed out of the light curve of the star we intend to analyze (e.g., García et al., 2011). Once the PSD is available, the preliminary step is to estimate the overall signal that constitutes each observation. This signal, broadly referred to as background, comprises signal originated from stellar oscillations (which are modeled through a typical Gaussian envelope), granulation activity (often decomposed into multiple components operating at different characteristic timescales, each one reproduced by a Harvey-like profile, Harvey 1985) and possible long-trend variations that could be related to the presence of stellar activity, rotational modulation, and instrumental effects (usually modeled by means of an additional Harvey-like profile). This background fit can be carried out by means of the public Bayesian inference software DIAMONDS (Corsaro and De Ridder, 2014), which has a specific adaptation for dealing with the multiple components that are typically encountered in low- and intermediate-mass stars of spectral types from F to K (Corsaro et al., 2017). Furthermore, one can extract the average (or global) asteroseismic parameters, namely the frequency of maximum oscillation power νmax and the large frequency separation Δν, quantities directly linked to fundamental stellar properties. A typical example of this analysis is shown in panel A of Figure 1 for the solar-type star 16 Cyg A observed by Kepler.
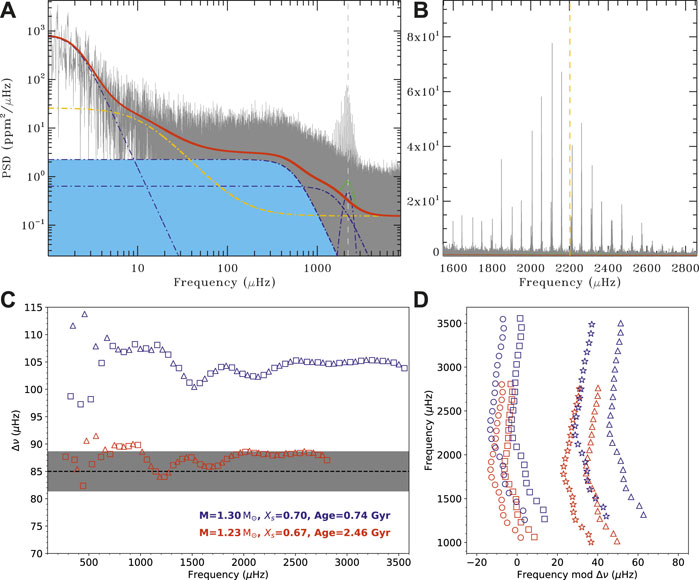
FIGURE 1. (A): An example of power spectral density (gray) for the solar-like star 16 Cyg A observed by Kepler. The solid red curve is a fit to the background as obtained by Corsaro et al. (2020), consisting of three Harvey-like profiles (blue dotted–dashed curves) plus white and colored noise (yellow dotted–dashed line). A global fit to the oscillation power excess is shown by the blue dotted–dashed Gaussian curve and the net effect on the background is visible as a doted green curve. (B): Observed frequency spectrum of 16 Cyg A centered in the frequency range around the frequency of maximum oscillation power (indicated with the yellow dashed line), showing the typical Gaussian-like shape. (C): Large separation Δν as a function of the frequency for two theoretical models (identified by red and blue colors) of the star GJ 504, chosen to fit the spectroscopic parameters. The dashed line indicates the still uncertain observed value Δν =(85.0 ± 3.6) μHz, with the confidence interval shown by the gray shaded area. Different symbols are used to distinguish between the modes l =0 (squares) and l =1 (triangles). (D): Échelle diagram for GJ 504 computed for the two models of panel C, with model’s average large separation values Δν =87.8 μHz (blue color model) and Δν =104.5 μHz (red color model) (Di Mauro et al., 2022). Different symbols are used for modes with different harmonic degrees, squares for l =0, triangles for l =1, circles for l =2 and stars for l =3.
The final step in the data analysis foresees an additional level of detail and it consists in measuring the observational properties of the individual oscillation modes that belong to the region of the oscillation power excess (see panel B of Figure 1). This analysis is of particular complexity as it may involve the fitting of a large number of parameters, moreover it can be accomplished only for stars where the PSD has a signal-to-noise ratio that is sufficiently high, along with the available frequency resolution, such that the individual modes can be resolved. In these cases, we make use of the public pipeline FAMED (Corsaro et al., 2020), which can perform an automated analysis of tens of individual mode properties in a reasonable amount of time.
2.2 Asteroseismic Assessment of Stellar Physical Properties
In the context of stellar asteroseismic characterization a key role is played by empirical relations, found by studying oscillation properties of hundreds of stars, that connect observable quantities with stellar physical parameters. In fact, a first estimate of global stellar parameters can be obtained starting from the main properties of the oscillation spectra, such as the large frequency separation (Δν), namely the difference between frequencies of same harmonic degree, and the frequency of maximum oscillation power (νmax) (Brown et al., 1991; Kjeldsen and Bedding, 1995; Huber et al., 2011). The large frequency separation (Δν), defined as the inverse sound travel time through the stellar radius (Tassoul, 1980), is linearly proportional to the square root of the stellar density (Ulrich, 1986) and hence it is related to the stellar mass and radius. The frequency of maximum oscillation power (νmax) is expected to scale with the acoustic cutoff frequency (νac) (Brown et al., 1991):
where Teff is the effective temperature of the star and g is its surface gravity. Hence by definition, νmax is also related to the mass and the radius of the star. A set of powerful scaling-laws, normalized to solar values, can be used to roughly determine the mass and radius of a star once Δν and νmax are known, as suggested by Huber et al. (2011):
A more precise characterization of the stellar structure, and in particular the age, can be obtained looking at individual pulsation frequencies, instead of using the average oscillation properties of the spectra, and comparing them with frequencies obtained by theoretical models built in order to match all the available photometric and spectroscopic parameters of the star under study or by employing more sophisticated techniques, such as inversion methods (Di Mauro, 2016).
Once the planet host has been properly studied, different exoplanetary details can be deduced with a good accuracy (Campante et al., 2018; Lundkvist et al., 2018). Several attempts have been tried to determine, for example, the orbital elements from the parameters of the star, but certainly the estimate of the age is the most important for understanding the history of the planet and its habitability. Generally, the ages of single main-sequence stars are inferred from empirical indicators, such as activity, rotation or from stellar model isochrones that are compared to observed classical parameters. However, the precision and accuracy that can currently be reached with these methods is not good enough (Lebreton and Goupil, 2014), compared to the possibility to use asteroseismic data.
As an example, here we report the case of GJ 504 (HD 115383), a G0-type solar-like star with effective temperature Teff ≃ 6200 K and surface gravity log g = 4.29 ± 0.07 dex (D’Orazi et al., 2017). This target appears to be more massive than the Sun (M = 1.28 ± 0.07 M⊙), with a radius R = 1.38 ± 0.2 R⊙ as predicted by theoretical models (Di Mauro et al., 2022), but its evolutionary stage is still an open question, with consequences for mass estimation of its star’s companion named GJ 504b, which could be a Jovian planet or a brown-dwarf. Panel C of Figure 1 shows the comparison between the average large separation obtained with a very low resolution by the analysis of the photometric TESS data collected in 120-s cadence mode and the theoretical value as a function of the oscillation frequency for modes l = 0 and l = 1. Here the theoretical adiabatic oscillation frequencies have been computed by using the ADIPLS package (Christensen-Dalsgaard, 2008a) on theoretical models built in order to match all the observed spectroscopic parameters. Among all the possible models, we show one model which seems compatible with the unconfirmed value of large frequency separation Δν = (85.0 ± 3.6) μHz (Di Mauro et al., 2022). It is very interesting to notice that models that match the luminosity deduced from the Gaia Parallax, are characterized by a very young age
A more detailed comparison between theoretical and observational frequencies is usually done by employing a so called échelle diagram, like the ones shown in panel D of Figure 1, obtained for two theoretical models of GJ 504 with parameters given in panel C. This diagram shows, for each harmonic degree, vertical ridges of frequencies in which consecutive symbols are equally spaced by the large separations. The distance between two adjacent columns of frequencies represents the small separation. Clearly here, the two theoretical models show a different oscillatory pattern. Please notice that for GJ 504 no individual observational frequencies have been already detected, but a comparison with the theoretical frequencies will have allowed to determine the age with an accuracy of less than 10%. In addition, the échelle diagram shows, for each l, a weak oscillatory signal so that symbols do not form straight columns. The characteristics of such signal are related to the location and thermodynamic properties of discontinuities occurring inside the star. By isolating the oscillatory components from the observed frequencies of oscillations it is possible to determine, for example, the location of the base of the convective envelope. This information is highly important also for testing stellar dynamo theories (Corsaro et al., 2021) and studying the stellar magnetic activity.
3 Magnetic Activity
Characterizing a star does not mean only to determine the main stellar parameters and its spectral type, but it requires also a precise assessment of the activity level, which is a measure of how strong the surface magnetic field is (Schrijver and Zwaan, 2000). In order to quantify the magnetic activity of a star, indices linked to physical quantifies (i.e., physical indices), like the flux in a particular spectral band, are the more appropriate ones. The intensities of emission in the chromospheric H (393.3 nm) and K (396.8 nm) lines of the Ca II represent strong markers of stellar magnetic activity (Hall, 2008) and for this reason many activity indicators are based on them (e.g.,
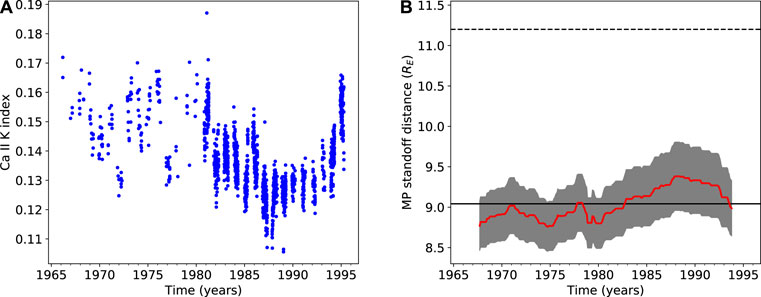
FIGURE 2. (A): Ca II K index of the star HD 76151 for the time interval 1966–1996, obtained from the MWO S-index measurements. (B): Magnetopause standoff distance (red line, with confidence interval represented by the gray shaded area) of a fictitious Earth-twin planet orbiting at 1 AU around the solar-type star HD 76151. The continuous black line indicates the mean value, while the dashed black line shows the mean Earth value as reference.
4 Host Star—Planet Interaction
To date, we have a simplified notion of habitability on extra-solar planets, mainly related to the concept of Habitable Zone (HZ). It is indeed based on the flux of radiation incident on a planet, and is classically defined as the range of distances from the host star in which a planet could potentially maintain liquid water on its surface (Hart, 1979; Kasting et al., 1993; Kopparapu et al., 2013).
Nevertheless, the stellar irradiance is not the only source to consider in the evaluation of the stellar environment from the habitability point of view. The variability of the host star and the extreme events must also be considered, as well as the flux in particular wavelength ranges (e.g., UV) compared to the bolometric one. The increase in UV and X-ray star’s flux generated by flares can strongly affect the near stellar environment. This radiation has enough energy to modify the stratospheric ozone cycle of an Earth-like planet, which plays a central role in the thermal structure of high atmosphere (Lovric et al., 2017). Recent studies have assessed the effects of high flaring activity on exoplanets, showing that ozone shield may be comprehensively destroyed, leaving the planet’s surface unprotected to extreme radiations that can damage complex organic structures (e.g., Tilley et al., 2019). Looking to more general effects on exoplanet’s atmospheres, frequent strong flares on active and young stars are disadvantageous for hosting life, because the atmospheric composition of orbiting planets is constantly altered (Vida et al., 2017). In the worst cases of unmagnetized (unprotected) planets, the atmosphere can even be eroded (Roettenbacher and Kane, 2017; Loyd et al., 2020).
When studying the star-planet interaction, a key point is represented by the evaluation of the stellar winds effects on the planetary environment. In magnetized planets, a main outcome of this interaction is represented by the perturbation and compression of the planetary magnetosphere. A direct measurement of the exoplanet magnetic moment is a difficult task. Different methods have been proposed, involving bow-shock observations during transits (Vidotto et al., 2011), radio signature (Hess and Zarka, 2011) or atmospheric escape structure detections (Carolan et al., 2021). All proposed techniques have a bias towards giant planet magnetospheric detection.
Nevertheless, magnetic field in terrestrial exoplanets can be estimated by dynamo models assuming an internal planetary structure model (see e.g., Driscoll and Olson, 2011). In general, it is interesting to estimate the magnetospheric compression of an Earth-twin exoplanet under the effect of host star stellar wind. Such method has been applied to a sample of solar-mass stars by assuming a Parker stellar wind model and using Ca II H&K lines measurements in See et al. (2014). Indeed, the magnetosphere extension can be estimated by the so called magnetopause standoff distance (RMP), defined as the distance from the planet at which the planetary magnetic field pressure balances the stellar wind dynamic pressure. On the Sun-Earth system such interaction results in a day-side average standoff distance of
Recently, we calibrate on the Sun-Earth system some relations which allow us to relate the solar activity, by means of the Ca II K index, with the solar wind speed and dynamic pressure (Reda et al., 2021, 2022). We also introduce a model which allows to compute the Earth magnetopause standoff distance simply starting from the above activity index (see Reda et al., 2022 for further details). Because of the use of a physical proxy, as the Ca II K index, such relations are very useful and can be employed to estimate the stellar wind properties in solar-like stars for which similar measurements are available. Panel B of Figure 2 shows, as example, the magnetopause standoff distance of an hypothetical Earth-twin planet orbiting at 1 AU around the solar-like star HD 76151, computed with our model. Due to the higher level of chromospheric activity of this star compared to the Sun, an Earth-twin planet orbiting around it would have a mean magnetosphere extension of
5 Discussion
Although the concept of habitable zone has been in the literature for some time, it is only in the last few years that complementary strategies have allowed a more rigorous definition, in order to understand how long an exoplanet may have been able to maintain habitability, so that life has had the opportunity to originate and to develop. Under the hypothesis that the evolution of life requires sufficient long times in a constant environment, it appears clear that a lasting stability of the atmosphere plays the most crucial role, since the planet should have survived not only to the period of heavy bombardment by asteroids and comets, but also during the host stars’ active X-ray, extreme ultraviolet (XUV) and stellar wind exposure (e.g., Wood et al., 2002; Lammer et al., 2003) occurring during the early phases of planetary system formation.
The extreme efficiency of asteroseismology in supporting the exoplanetary program has been demonstrated by several recent works (see e.g., Campante et al., 2018; Lundkvist et al., 2018), but we believe that our synergic strategy has the additional potential of enabling to characterize, not only the main stellar and planetary parameters such as the dimensions, the density and the age, but also to infer the stellar magnetic activity and the interaction between the star and the hosted planets. Knowledge about the magnetic activity level of the host-star and its influence on an orbiting planet will allow for example to get conclusions on the magnetosphere compression which, as known, is directly connected to atmospheric erosion processes induced by stellar winds, and strictly related to planetary habitability conditions. We believe that this method is clearly advantageous since it only requires asteroseismic and chromospheric activity measurements, already available for a large sample of stars and further new measurements will be available in the next years. This will produce results of particular interest once the incoming data from operating space missions and from ground based campaigns will have been fully exploited and understood.
The outcome will be of relevance especially in the context of the future PLATO (PLAnetary Transits & Oscillations of stars) (Rauer et al., 2014) space mission, whose primary goal is to detect terrestrial exoplanets in the habitable zone of Sun-like stars. Since our model to estimate the mean stellar wind is calibrated on the Sun, the best targets to which to extend our procedure are represented by intermediate age (≳ 2.6 Gyr) G spectral type stars, but we do not exclude the possibility to consider for the future also early K- o late F-type stars. A further requirement is a stellar Rossby number ≳ 1 which, as recently pointed out by Reinhold et al. (2019), is related to a faculae activity regime, the dominant source of activity in the Sun. In this respect of great help is the calibration from asteroseismology recently made by Corsaro et al. (2021), which allows to determine the Rossby number simply knowing the stellar (B − V) color index and the rotation period. At present, we are preparing a catalogue of solar-like targets observed by the Kepler and TESS satellites on which presence of one or more exoplanets has been confirmed. Moreover, for these targets measurements in the Ca II H&K lines have to be available from ground observatories in order to estimate the chromospheric activity and the mean stellar wind acting on the orbiting planets.
Data Availability Statement
The dataset of the HK Project at the Mount Wilson Observatory are available from the National Solar Observatory (NSO) website (https://nso.edu/data/historical-data/mount-wilson-observatory-hk-project/). The TESS asteroseismic data can be found on the TASOC (TESS Asteroseismic Science Operations Center) website (https://tasoc.dk). The Kepler asteroseismic data can be found on the KASOC (Kepler Asteroseismic Science Operations Center) website (https://kasoc.phys.au.dk).
Author Contributions
RR assembled the synergic strategy, made the analysis on the magnetic data and the computation of the theoretical adiabatic oscillation frequencies; EC was responsible for the analysis of oscillations data; MPDM provided the theoretical framework to interpret the asteroseismic data; LG and FB took care of the synergies between stellar and solar activity; TA designed the model of star-planet interaction. All authors contributed to manuscript revision, read and approved the submitted version.
Funding
Funding for the TESS mission is provided by the NASA Explorer Program.
Conflict of Interest
The authors declare that the research was conducted in the absence of any commercial or financial relationships that could be construed as a potential conflict of interest.
The handling editor TC declared a past co-authorship with the authors MM, EC.
Publisher’s Note
All claims expressed in this article are solely those of the authors and do not necessarily represent those of their affiliated organizations, or those of the publisher, the editors and the reviewers. Any product that may be evaluated in this article, or claim that may be made by its manufacturer, is not guaranteed or endorsed by the publisher.
Acknowledgments
RR is a PhD student of the PhD course in Astronomy, Astrophysics and Space Science, a joint research program between the University of Rome “Tor Vergata,” the Sapienza University of Rome and the National Institute of Astrophysics (INAF). The work presented here is based on data from the HK Project at the Mount Wilson Observatory. This paper includes data collected with the TESS mission, obtained from the MAST data archive at the Space Telescope Science Institute (STScI). The work presented here is also based on NASA Kepler mission. We thank the entire Kepler and TESS teams for the development and operations of these NASA outstanding missions.
References
Airapetian, V. S., Barnes, R., Cohen, O., Collinson, G. A., Danchi, W. C., Dong, C. F., et al. (2020). Impact of Space Weather on Climate and Habitability of Terrestrial-type Exoplanets. Int. J. Astrobiol. 19, 136–194. doi:10.1017/S1473550419000132
Baglin, A., Auvergne, M., Boisnard, L., Lam-Trong, T., Barge, P., Catala, C., et al. (2006). CoRoT: a High Precision Photometer for Stellar Ecolution and Exoplanet Finding. 36th Cospar Sci. Assem. 36, 3749.
Borucki, W. J., Koch, D., Basri, G., Batalha, N., Brown, T., Caldwell, D., et al. (2010). Kepler Planet-Detection Mission: Introduction and First Results. Science 327, 977–980. doi:10.1126/science.1185402
Brown, T. M., Gilliland, R. L., Noyes, R. W., and Ramsey, L. W. (1991). Detection of Possible P-Mode Oscillations on Procyon. ApJ 368, 599. doi:10.1086/169725
Campante, T. L., Barros, S. C. C., Demangeon, O., da Nóbrega, H. J., Kuszlewicz, J. S., Pereira, F., et al. (2018). “Synergy between Asteroseismology and Exoplanet Science: an Outlook,” in PHysics of Oscillating STars. Proceedings from the PHOST (PHysics of Oscillating STars) symposium hosted by the Oceanographic Observatory in Banyuls-sur-mer (France) from 2-7 September 2018. This conference honours the life work of Professor Hiromoto Shibahashi. doi:10.5281/zenodo.246321050
Carolan, S., Vidotto, A. A., Hazra, G., Villarreal D’Angelo, C., and Kubyshkina, D. (2021). The Effects of Magnetic Fields on Observational Signatures of Atmospheric Escape in Exoplanets: Double Tail Structures. Mon. Not. R. Astron. Soc. 508, 6001–6012. doi:10.1093/mnras/stab2947
Chaplin, W. J., Bedding, T. R., Bonanno, A., Broomhall, A.-M., García, R. A., Hekker, S., et al. (2011). Evidence for the Impact of Stellar Activity on the Detectability of Solar-like Oscillations Observed by Kepler. ApJ 732, L5. doi:10.1088/2041-8205/732/1/L5
Christensen-Dalsgaard, J. (2008a). ADIPLS-the Aarhus Adiabatic Oscillation Package. Astrophys. Space Sci. 316, 113–120. doi:10.1007/s10509-007-9689-z
Christensen-Dalsgaard, J. (2008b). ASTEC-the Aarhus STellar Evolution Code. Astrophys. Space Sci. 316, 13–24. doi:10.1007/s10509-007-9675-5
Cohen, O., Ma, Y., Drake, J. J., Glocer, A., Garraffo, C., Bell, J. M., et al. (2015). The Interaction of Venus-like, M-Dwarf Planets with the Stellar Wind of Their Host Star. ApJ 806, 41. doi:10.1088/0004-637x/806/1/41
Corsaro, E., Bonanno, A., Mathur, S., García, R. A., Santos, A. R. G., Breton, S. N., et al. (2021). A Calibration of the Rossby Number from Asteroseismology. A&A 652, L2. doi:10.1051/0004-6361/202141395
Corsaro, E., and De Ridder, J. (2014). DIAMONDS: A New Bayesian Nested Sampling Tool. A&A 571, A71. doi:10.1051/0004-6361/201424181
Corsaro, E., Mathur, S., García, R. A., Gaulme, P., Pinsonneault, M., Stassun, K., et al. (2017). Metallicity Effect on Stellar Granulation Detected from Oscillating Red Giants in Open Clusters. A&A 605, A3. doi:10.1051/0004-6361/201731094
Corsaro, E., McKeever, J. M., and Kuszlewicz, J. S. (2020). Fast and Automated Peak Bagging with DIAMONDS (FAMED). A&A 640, A130. doi:10.1051/0004-6361/202037930
Di Mauro, M. P. (2016). “A Review on Asteroseismology,” in Frontier Research in Astrophysics-II (FRAPWS2016) (Trieste, Italy: PoS) 269, 29. doi:10.22323/1.269.0029
Di Mauro, M. P., Reda, R., Mathur, S., García, R. A., Buzasi, D. L., Corsaro, E., et al. (2022). On the Non-detection of Solar-like Pulsations in the Host Star GJ 504 Observed by TESS. submitted to ApJ
Dong, C., Jin, M., Lingam, M., Airapetian, V. S., Ma, Y., and van der Holst, B. (2018). Atmospheric Escape from the TRAPPIST-1 Planets and Implications for Habitability. Proc. Natl. Acad. Sci. U.S.A. 115, 260–265. doi:10.1073/pnas.1708010115
D’Orazi, V., Desidera, S., Gratton, R. G., Lanza, A. F., Messina, S., Andrievsky, S. M., et al. (2017). A Critical Reassessment of the Fundamental Properties of GJ 504: Chemical Composition and Age. A&A 598, A19. doi:10.1051/0004-6361/201629283
Driscoll, P., and Olson, P. (2011). Optimal Dynamos in the Cores of Terrestrial Exoplanets: Magnetic Field Generation and Detectability. Icarus 213, 12–23. doi:10.1016/j.icarus.2011.02.010
Egeland, R., Soon, W., Baliunas, S., Hall, J. C., Pevtsov, A. A., and Bertello, L. (2017). The Mount Wilson Observatorys-Index of the Sun. ApJ 835, 25. doi:10.3847/1538-4357/835/1/25
García, R. A., Hekker, S., Stello, D., Gutiérrez-Soto, J., Handberg, R., Huber, D., et al. (2011). Preparation of Kepler Light Curves for Asteroseismic Analyses. Mon. Not. R. Astron. Soc. 414, L6–L10. doi:10.1111/j.1745-3933.2011.01042.x
García, R. A., Mathur, S., Salabert, D., Ballot, J., Régulo, C., Metcalfe, T. S., et al. (2010). CoRoT Reveals a Magnetic Activity Cycle in a Sun-like Star. Science 329, 1032. doi:10.1126/science.1191064
Hall, J. C. (2008). Stellar Chromospheric Activity. Living Rev. Sol. Phys. 5, 2. doi:10.12942/lrsp-2008-2
Hart, M. H. (1979). Habitable Zones about Main Sequence Stars. Icarus 37, 351–357. doi:10.1016/0019-1035(79)90141-6
Harvey, J. (1985). High-resolution Helioseismology. In Future Missions in Solar, Heliospheric & Space Plasma Physics, eds. E. Rolfe, and B. Battrick. 235 (Noordwijk, Netherlands: ESA Special Publication)
Hess, S. L. G., and Zarka, P. (2011). Modeling the Radio Signature of the Orbital Parameters, Rotation, and Magnetic Field of Exoplanets. A&A 531, A29. doi:10.1051/0004-6361/201116510
Huber, D., Bedding, T. R., Stello, D., Hekker, S., Mathur, S., Mosser, B., et al. (2011). Testing Scaling Relations for Solar-like Oscillations from the Main Sequence to Red Giants Usingkeplerdata. ApJ 743, 143. doi:10.1088/0004-637X/743/2/143
Kasting, J. F., Whitmire, D. P., and Reynolds, R. T. (1993). Habitable Zones Around Main Sequence Stars. Icarus 101, 108–128. doi:10.1006/icar.1993.1010
Kjeldsen, H., and Bedding, T. R. (1995). Amplitudes of Stellar Oscillations: the Implications for Asteroseismology. Astronomy Astrophysics 293, 87–106. doi:10.48550/ARXIV.ASTRO-PH/9403015
Kopparapu, R. K., Ramirez, R., Kasting, J. F., Eymet, V., Robinson, T. D., Mahadevan, S., et al. (2013). Habitable Zones Around Main-Sequence Stars: New Estimates. ApJ 765, 131. doi:10.1088/0004-637x/765/2/131
Lammer, H., Selsis, F., Ribas, I., Guinan, E. F., Bauer, S. J., and Weiss, W. W. (2003). Atmospheric Loss of Exoplanets Resulting from Stellar X-Ray and Extreme-Ultraviolet Heating. ApJ 598, L121–L124. doi:10.1086/380815
Lebreton, Y., and Goupil, M. J. (2014). Asteroseismology for "à la carte" stellar age-dating and weighing. A&A 569, A21. doi:10.1051/0004-6361/201423797
Lovric, M., Tosone, F., Pietropaolo, E., Del Moro, D., Giovannelli, L., Cagnazzo, C., et al. (2017). The Dependence of the [FUV-MUV] Colour on Solar Cycle. J. Space Weather Space Clim. 7, A6. doi:10.1051/swsc/2017001
Loyd, R. O. P., Shkolnik, E. L., France, K., Wood, B. E., and Youngblood, A. (2020). When “Boring” Stars Flare: The Ultraviolet Activity of GJ 887, a Bright M Star Hosting Newly Discovered Planets. Res. Notes AAS 4, 119. doi:10.3847/2515-5172/aba94a
Lundkvist, M. S., Huber, D., Silva Aguirre, V., and Chaplin, W. J. (2018). Using Asteroseismology to Characterise Exoplanet Host Stars. arXiv e-prints , arXiv:1804.02214
Marsden, S. C., Petit, P., Jeffers, S. V., Morin, J., Fares, R., Reiners, A., et al. (2014). A BCool Magnetic Snapshot Survey of Solar-type Stars. Mon. Not. R. Astron. Soc. 444, 3517–3536. doi:10.1093/mnras/stu1663
Mathur, S., García, R. A., Bugnet, L., Santos, Â. R. G., Santiago, N., and Beck, P. G. (2019). Revisiting the Impact of Stellar Magnetic Activity on the Detectability of Solar-like Oscillations by Kepler. Front. Astron. Space Sci. 6, 46. doi:10.3389/fspas.2019.00046
Mathur, S., Metcalfe, T. S., Woitaszek, M., Bruntt, H., Verner, G. A., Christensen-Dalsgaard, J., et al. (2012). A Uniform Asteroseismic Analysis of 22 Solar-type Stars Observed Bykepler. ApJ 749, 152. doi:10.1088/0004-637X/749/2/152
Metcalfe, T. S., Monteiro, M. J. P. F. G., Thompson, M. J., Molenda-Żakowicz, J., Appourchaux, T., Chaplin, W. J., et al. (2010). A Precise Asteroseismic Age and Radius for the Evolved Sun-like Star KIC 11026764. ApJ 723, 1583–1598. doi:10.1088/0004-637x/723/2/1583
Gaia Collaboration Prusti, T., de Bruijne, J. H. J., Brown, A. G. A., Vallenari, A., Babusiaux, C., et al. (2016). The Gaia Mission. Astronomy Astrophysics 595, A1. doi:10.1051/0004-6361/201629272
Rauer, H., Catala, C., Aerts, C., Appourchaux, T., Benz, W., Brandeker, A., et al. (2014). The PLATO 2.0 Mission. Exp. Astron. 38, 249–330. doi:10.1007/s10686-014-9383-4
Reda, R., Giovannelli, L., Alberti, T., Berrilli, F., Bertello, L., Del Moro, D., et al. (2022). The Exoplanetary Magnetosphere Extension in Sun-like Stars Based on the Solar Wind - Solar UV Relation. arXiv e-prints , arXiv:2203.01554
Reda, R., Giovannelli, L., Alberti, T., Berrilli, F., Giobbi, P., and Penza, V. (2021). Correlation of Solar Activity Proxy with Solar Wind Dynamic Pressure in the Last Five Solar Cycles. Il Nuovo Cimento della Soc. Ital. Fis. 44 C, 120. doi:10.1393/ncc/i2021-21121-7
Reinhold, T., Bell, K. J., Kuszlewicz, J., Hekker, S., and Shapiro, A. I. (2019). Transition from Spot to Faculae Domination. A&A 621, A21. doi:10.1051/0004-6361/201833754
Ricker, G. R., Winn, J. N., Vanderspek, R., Latham, D. W., Bakos, G. Á., Bean, J. L., et al. (2014). “Transiting Exoplanet Survey Satellite (TESS),” in Space Telescopes and Instrumentation 2014: Optical, Infrared, and Millimeter Wave. Editors J. Oschmann, M. Jacobus, M. Clampin, G. G. Fazio, and H. A. MacEwen, 914320. 9143 of Society of Photo-Optical Instrumentation Engineers (SPIE) Conference Series. doi:10.1117/12.2063489
Roettenbacher, R. M., and Kane, S. R. (2017). The Stellar Activity of TRAPPIST-1 and Consequences for the Planetary Atmospheres. ApJ 851, 77. doi:10.3847/1538-4357/aa991e
Schrijver, C. J., and Zwaan, C. (2000). Solar and Stellar Magnetic Activity. Cambridge University Press.
See, V., Jardine, M., Vidotto, A. A., Petit, P., Marsden, S. C., Jeffers, S. V., et al. (2014). The Effects of Stellar Winds on the Magnetospheres and Potential Habitability of Exoplanets. A&A 570, A99. doi:10.1051/0004-6361/201424323
Shue, J.-H., Chao, J. K., Fu, H. C., Russell, C. T., Song, P., Khurana, K. K., et al. (1997). A New Functional Form to Study the Solar Wind Control of the Magnetopause Size and Shape. J. Geophys. Res. 102, 9497–9511. doi:10.1029/97ja00196
Skumanich, A. (1972). Time Scales for Ca II Emission Decay, Rotational Braking, and Lithium Depletion. ApJ 171, 565. doi:10.1086/151310
Tassoul, M. (1980). Asymptotic Approximations for Stellar Nonradial Pulsations. ApJS 43, 469–490. doi:10.1086/190678
Tilley, M. A., Segura, A., Meadows, V., Hawley, S., and Davenport, J. (2019). Modeling Repeated M Dwarf Flaring at an Earth-like Planet in the Habitable Zone: Atmospheric Effects for an Unmagnetized Planet. Astrobiology 19, 64–86. doi:10.1089/ast.2017.1794
Ulrich, R. K. (1986). Determination of Stellar Ages from Asteroseismology. ApJ 306, L37. doi:10.1086/184700
Vaughan, A. H., Preston, G. W., and Wilson, O. C. (1978). Flux Measurements of Ca II H and K Emission. Publ. Astronomical Soc. Pac. 90, 267–274. doi:10.1086/130324
Vida, K., Kővári, Z., Pál, A., Oláh, K., and Kriskovics, L. (2017). Frequent Flaring in the TRAPPIST-1 System-Unsuited for Life? ApJ 841, 124. doi:10.3847/1538-4357/aa6f05
Vidotto, A. A., Jardine, M., and Helling, C. (2011). Prospects for Detection of Exoplanet Magnetic Fields through Bow-Shock Observations during Transits. Mon. Not. R. Astron. Soc. 411, L46–L50. doi:10.1111/j.1745-3933.2010.00991.x
Wilson, O. C. (1978). Chromospheric Variations in Main-Sequence Stars. ApJ 226, 379–396. doi:10.1086/156618
Keywords: exoplanets, solar-type stars, asteroseismology, stellar magnetic activity, habitability, stellar wind, stellar pulsations, star-planet interaction
Citation: Reda R, Di Mauro MP, Giovannelli L, Alberti T, Berrilli F and Corsaro E (2022) A Synergic Strategy to Characterize the Habitability Conditions of Exoplanets Hosted by Solar-Type Stars. Front. Astron. Space Sci. 9:909268. doi: 10.3389/fspas.2022.909268
Received: 31 March 2022; Accepted: 03 June 2022;
Published: 06 July 2022.
Edited by:
Tiago Campante, Instituto de Astrofísica e Ciências do Espaço (IA), PortugalReviewed by:
R. Gafeira, University of Coimbra, PortugalCopyright © 2022 Reda, Di Mauro, Giovannelli, Alberti, Berrilli and Corsaro. This is an open-access article distributed under the terms of the Creative Commons Attribution License (CC BY). The use, distribution or reproduction in other forums is permitted, provided the original author(s) and the copyright owner(s) are credited and that the original publication in this journal is cited, in accordance with accepted academic practice. No use, distribution or reproduction is permitted which does not comply with these terms.
*Correspondence: Raffaele Reda, raffaele.reda@roma2.infn.it