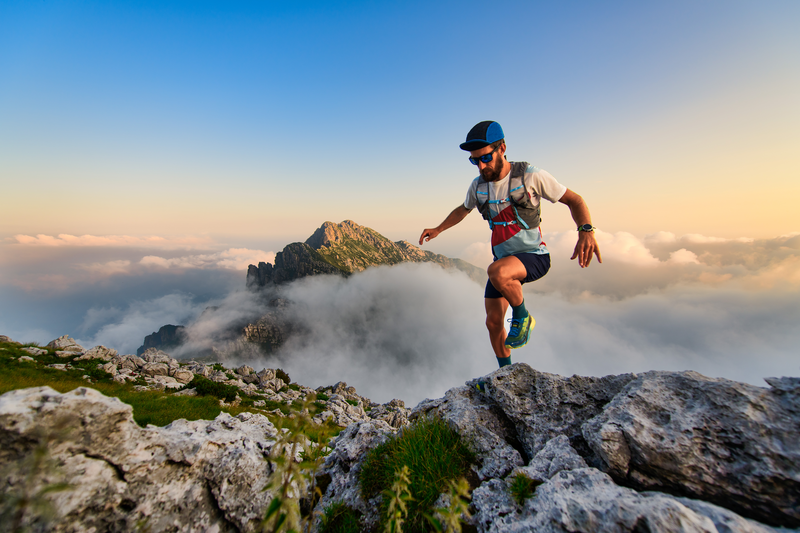
94% of researchers rate our articles as excellent or good
Learn more about the work of our research integrity team to safeguard the quality of each article we publish.
Find out more
ORIGINAL RESEARCH article
Front. Astron. Space Sci. , 31 May 2022
Sec. Space Physics
Volume 9 - 2022 | https://doi.org/10.3389/fspas.2022.879314
This article is part of the Research Topic Magnetosphere and Ionosphere response to the solar wind transients View all 6 articles
The present article aims at a consistent understanding of observation, theoretical model, and simulation with the geomagnetic sudden commencement (SC) observed in the morning and afternoon at high and middle latitudes in the northern and southern hemispheres and at the noontime equator on 12 May 2021. The SC in Bx- and By-components of the geomagnetic field, SCx,y, was composed of the positive/negative preliminary (PI) and main impulses (MI) as SCx (+ -) and SCy (- +) in the morning and SCx (- +) and SCy (+ -) in the afternoon at middle latitudes in the northern hemisphere. SCx in the southern hemisphere is in the same polarity as those in the northern hemisphere, except for SCx (+ +) in the morning. SCy in the southern hemisphere has reverse polarity to those in the northern hemisphere. The PIx in the northern hemisphere matches the well-established two-cell Hall current vortices with anti-clockwise and clockwise directions in the morning and afternoon, respectively, and the MIx matches reverse Hall current vortices. The PIx and MIx in the southern hemisphere meet the Hall currents that are mirror images of those in the northern hemisphere with respect to the equator except for the positive MI in the morning. The PIy in the northern hemisphere is shown to meet the northward and southward Pedersen currents in the morning and afternoon, respectively, and the MIy meets reverse Pedersen currents. The PIy and MIy in the southern hemisphere are found to meet the Pedersen currents that are mirror images of those in the northern hemisphere. At the equator, typical SCx (- +) is observed, meeting the Cowling currents that should be supplied by the Pedersen currents responsible for the observed midlatitude SCy in the northern and southern hemispheres. The electric fields of the PI and MI observed by the HF Doppler sounders at the middle latitudes in the northern hemisphere are westward and eastward, respectively, in both the morning and afternoon, meeting the conventional dusk-to-dawn PI and dawn-to-dusk MI electric fields. The onset of the PI is found to be simultaneous with the resolution of a few seconds from high latitude to the equator in both the northern and southern hemispheres, indicating instantaneous achievement of the Pedersen–Cowling currents from high latitude to the equator. The instantaneous achievement of the energy-consuming Pedersen–Cowling currents is explained by the TM0/TEM mode wave in the Earth-ionosphere waveguide/transmission line rather than the compressional waves in the magnetosphere and F-region ionosphere. REPPU (REProduce Plasma Universe) global simulation model equipped with a potential solver at the inner boundary of the model magnetosphere reproduces the PI and MI electric fields at middle latitudes and SCx (- +) at the dayside equator. The simulation results are found to be consistent with most features of observations, such as the time scale of PI and MI, direction of the midlatitude electric field and generation of the Cowling currents. The simulation proves that the electric fields and FACs are generated in the outer magnetosphere, transmitted to the polar ionosphere and then to the equator in the Pedersen–Cowling current circuit.
The geomagnetic sudden commencement (SC) caused by the solar wind shock/discontinuity is composed of a step-like magnetic increase (DL: disturbance at low latitude), preliminary impulse (PI) and main impulse (MI) (Araki, 1994 and references therein). The SC represents a change in Bx-component of the magnetic field in most cases. In the present article, we use SCx,y, PIx,y and MIx,y to represent those in Bx- and By-components, while DL is used as is. The time scales of PIx and MIx are 1 min and 5–10 min, respectively. The DL is caused by the magnetopause currents, and the PIx and MIx are caused by the ionospheric Hall current vortices driven by the dusk-to-dawn and dawn-to-dusk electric fields, respectively. The PIx currents are in counter-clockwise and clockwise directions in the morning and afternoon, respectively and therefore, the PIx is positive (PPI: Preliminary positive impulse) and negative (PRI: preliminary reverse impulse) in the morning and afternoon, respectively at high and middle latitudes. The MIx currents are in reverse direction to those of the PIx. The two-cell Hall current vortices are estimated from the global magnetometer networks as the equivalent ionospheric currents (Vichare et al., 2014; Kikuchi et al., 2016). Thus, the type of SCx is SCx (+ -) in the morning and SCx (- +) in the afternoon. The SCx is in phase in the northern and southern hemispheres with greater amplitude in summer hemisphere, because the MIx currents flow in the ionosphere with higher conductivity in summer (Yumoto et al., 1996). The PIx and MIx electric field and currents are supplied with field-aligned currents (FACs) generated by dynamos in the outer magnetosphere (Slinker et al., 1999; Fujita et al., 2003a; Fujita et al., 2003b). Therefore, the polarity of the PIx changes from positive/negative to negative/positive as one moves to higher latitude across the foot of the upward/downward FACs (Kikuchi et al., 2016).
The SCx at the dayside equator is enhanced with the latitudinal and longitudinal profiles of amplitude similar to those of diurnal variations (Rastogi, 1993; Rastogi et al., 2001) and with amplitude peaks before noon (Nilam et al., 2020). The equatorial enhancement of SCx is caused by the Cowling currents, i.e., Pedersen currents intensified by the Cowling effects (Hirono, 1952; Baker and Martyn, 1953). As a result, the equatorial SCx on the dayside is SCx (- +) composed of the DL superimposed by the negative PIx and positive MIx. The Cowling currents in the night are weak, but the night SCx begins with a two-step increase due to a small PPI (Araki et al., 1985). The nighttime DL is superimposed by magnetic field caused by the Region-1 type FACs of the MI, which results in larger amplitude in the night than in the day and larger amplitude at higher latitudes around the midnight (Araki et al., 2006; Shinbori et al., 2009).
The SC in the Y-component of the geomagnetic field (SCy) is caused by the Pedersen currents at middle latitude, so the MIy is positive/negative in the morning/afternoon (Tsunomura, 1998). The midlatitude Pedersen currents are supposed to achieve a current circuit from FACs at high latitude to the Cowling current at the equator (Kikuchi and Araki, 1979).
The electric fields of the PI and MI cause ionospheric plasma motion at middle latitudes as observed by the HF Doppler sounders with temporal resolution of 10 s (Kikuchi et al., 1985; Sastri, 2002; Kikuchi et al., 2016; Kikuchi et al., 2021). The SC-associated HF Doppler frequency deviations (SCF) are mostly in a bipolar structure as SCF (+ -) and SCF (- +) composed of the preliminary (PFD) and main (MFD) frequency deviations (Huang et al., 1973; Kikuchi et al., 1985). Kikuchi et al. (1985) showed that SCF (+ -) appears in the day and evening, and SCF (- +) in the night. The day-night reversal of PFD and MFD are caused by the dusk-to-dawn and dawn-to-dusk electric fields, respectively. The evening anomaly of the electric fields is due to the dawn-dusk asymmetry in the distribution of electric potential transmitted from the polar ionosphere (Tsunomura, 1999).
The onset of the PIx was found to be simultaneous within the temporal resolution of 10 s at all latitudes (Araki, 1977), and the onset of the PFD observed at middle latitudes has been shown to be simultaneous with the PIx at high and equatorial latitudes on the day- and night-sides (Kikuchi et al., 2021). Araki (1977) showed that the onset of the PIx is earlier than the DL by several 10 s of sec, probably because of difference in the propagation time between along and perpendicular to the magnetic field lines as deduced from earlier onset of the PFD than DL at middle latitude (Kikuchi, 1986). The PI currents are transmitted from the magnetopause dynamo down the magnetic field lines via FACs (Tamao, 1964a; Tamao, 1964b; Slinker et al., 1999; Fujita et al., 2003a), while it takes some more time for the DL to propagate in the equatorial plane of the magnetosphere and plasmasphere via the fast (compressional) mode wave (Chi et al., 2006). In extreme solar wind conditions, however, the DL can arrive earlier than the PIx currents as observed during the SC on 29 October 2003 (Kikuchi et al., 2016). In this extreme event, no PIx appears at the equator because of overwhelming DL, but PI appears in the equatorial Cowling currents and PFD at midlatitude, because both PFD and Cowling current are not associated with DL, but associated with the PI current from the polar to equatorial ionosphere.
As overviewed above, ground magnetometer and HF Doppler sounder observations have shown that the electric field and currents are transmitted from the polar ionosphere to the equator near-instantaneously. Wave modes that allow rapid propagation have been studied using the compressional waves in the magnetosphere and/or in the F-region ionosphere (Tamao, 1964a; Tamao, 1964b; Chi et al., 2006; Lysak et al., 2015; Tu and Song, 2019) or using the TM0/TEM mode waves in the Earth-ionosphere waveguide/transmission line (Kikuchi et al., 1978; Kikuchi and Araki, 1979; Kikuchi, 2014). The Alfven speed of the compressional waves can be applied to near-instantaneous propagation, and the Hall currents responsible for the PIx may be explained by the mode conversion from the compressional waves (Tamao, 1964a; Tamao, 1964b; Lysak et al., 2015). However, we need to explain the overall characteristics of the electric field, including the day-night reversal (Kikuchi et al., 2021) and evening anomaly with the same direction as in the day (Kikuchi et al., 2016). Furthermore, we should explain the instantaneous achievement of the polar to equatorial ionospheric currents and energy consumption in the midlatitude Pedersen and equatorial Cowling currents. The Pedersen/Cowling currents have never been explained by means of the compressional waves. Kikuchi et al. (2016) showed that the midlatitude F-region ionosphere is not compressed by the compressional waves, which indicates no contribution of the compressional waves on the energy input to the midlatitude ionosphere. Kikuchi (2014) showed that the TM0/TEM mode waves transmit the ionospheric electric potential and Pedersen currents to the equator, achieving the Pedersen–Cowling current circuit near-instantaneously. The present article aims at consistent understanding of observations of ionospheric electric fields and ground magnetic fields, waveguide/transmission line model, and global simulations by analyzing the SC event that occurred on 12 May 2021. For this purpose, we examine local time and latitudinal characteristics of the SCx and SCy observed in the northern and southern hemispheres, electric fields observed by HF Doppler sounders at middle latitudes in the northern hemisphere and reproduction of the midlatitude electric fields and equatorial SCx by the global simulation. In particular, SCy is focused on from the viewpoint of the Pedersen–Cowling current circuit as an energy channel from the polar to the equatorial ionosphere. We use magnetometer data from the northern and southern hemispheres in the morning and afternoon and from the equator at noon, and the HF Doppler sounder data from Czechia and Japan in the morning and afternoon, respectively. As shown below, the morning/afternoon and northern/southern hemispheric symmetry/asymmetry of SCx and SCy are consistent with the Hall and Pedersen–Cowling current circuits that are mirror images of each other in the northern and southern hemispheres. Furthermore, the onset of the PIy is found to be simultaneous over the globe within a few seconds even when PIx begins with delays of tens of seconds. The simultaneous onset proves the instantaneous achievement of the global Hall and Pedersen–Cowling current circuits by the TM0/TEM mode waves propagating at the speed of light in the Earth-ionosphere waveguide/transmission line (Kikuchi et al., 1978; Kikuchi and Araki, 1979; Kikuchi, 2014). The HF Doppler sounders operated in the morning (Czechia) and afternoon (Japan) help ensure that the PI and MI electric fields are typical from dusk-to-dawn and dawn-to-dusk, respectively. We discuss that the SC electric fields are potential fields associated with ionospheric currents flowing from the polar to equatorial ionosphere by reproducing midlatitude electric fields and Cowling currents with the REPPU global simulation model. The REPPU model employs the potential solver that solves the current continuity equation with the FACs as an input at the inner boundary of the model magnetosphere (Tanaka, 2007; Ebihara et al., 2014; Tanaka et al., 2020). The physical basis for the instantaneous propagation of the electric potential and currents in the potential solver is provided by the TM0/TEM waveguide/transmission line mode.
The SC was recorded at middle latitudes in the northern hemisphere; Uppsala (UPS, 58.50°N geomagnetic latitude (GML)) in the morning (8.4 MLT) and Memambetsu (MMB, 35.72°N GML) in the afternoon (16.3 MLT). In the southern hemisphere, the SC was recorded at Hermanus (HER, 33.95°S GML) in the morning (7.5 MLT) and Learmonth (LRM, 32.51°S GML) in the afternoon (14.3 MLT). We used low latitude and equatorial stations; Alibag (ABG, 10.76°N GML) and Tirunelveli (TIR, 0.52°N GML) at noon (12 MLT) to confirm the Pedersen–Cowling current circuit. We also used the conjugate stations at auroral latitudes in the northern and southern hemispheres; Tjornes (TJO, 66.53°N GML) and Syowa (SYO, 70.46°S GML), to detect ionospheric currents near the FACs in the morning (6.5 MLT). The magnetometer data were sampled every 1 s at TJO, SYO, MMB, HER, and LRM, and 10 s at UPS. The magnetometer stations are listed in Table 1.
TABLE 1. List of the magnetometer stations of INTERMAGNET, Indian Institute of Geomagnetism (IIG), and National Institute of Polar Research (NIPR).
The HF Doppler sounders were operated at Kasperk, Czechia (48.67°N GML), and Iitate, Sugadaira, Fujisawa, in Japan (27–29.5°N GML), as listed in Table 2, to detect the vertical motion of the ionosphere due to the electric fields of SC in the morning (0730 MLT) and afternoon (1530 MLT), respectively. The HF Doppler sounder system is composed of the transmitter (Tx) and receiver (Rx) separated at distances listed in Table 2. The HF Doppler sounder system in Czechia is composed of three transmitters (Tx1, Tx2, and Tx3) with a frequency of 4.65 MHz and one receiver (Rx) at distances 118–171 km, as shown in Table 2, where the elevation angle is for reflection height of 300 km. Details of the system and the principle of Doppler sounding are described by Chum et al. (2010). The HF Doppler sounder system in Japan is composed of Tx in Tokyo with frequencies of 5.006 and 8.006 MHz and several receivers deployed from the northernmost part (Hokkaido) to the southernmost part (Okinawa) of Japan. Details of the system in Japan are described by Nakata et al. (2021). The receiver sites and frequency used in the present analyses are listed in Table 2. The data are sampled every 6 s in Czechia and 10 s in Japan. The time resolutions of the HF Doppler data are high enough to detect the PI and MI electric fields (Kikuchi et al., 2016; Kikuchi et al., 2021).
TABLE 2. List of HF Doppler sounders in Japan [HF Doppler Sounding Experiment in Japan (uec.ac.jp)] and Czechia [Doppler sounding of the Ionosphere (cas.cz)].
Figures 1A and B show SCx (+ -) and SCy (- +) at UPS in the morning (Figure 1A) and SCx (- +) and SCy (+ -) at MMB in the afternoon (Figure 1B), showing morning/afternoon asymmetry both in SCx and SCy in the northern hemisphere. SCx agrees with Araki (1994)’s model composed of the PIx and MIx superimposed on the step-like DL. The morning/afternoon asymmetry in PIx agrees with the two-cell Hall current vortices with counter-clockwise and clockwise directions in the morning and afternoon, respectively (see the schematic current circuit in Figure 6). The morning/afternoon asymmetry in PIy agrees with the northward and southward Pedersen currents in the morning and afternoon, respectively. The morning/afternoon asymmetry in MIx,y is explained by similar current circuits with reverse direction.
FIGURE 1. Geomagnetic sudden commencement recorded in the morning (AM) and afternoon (PM) at middle latitudes in the northern (A and B) and southern hemispheres (C and D). UPS, MMB, HER, and LRM are abbreviations of the magnetometer station names, Uppsala, Memambetsu, Hermanas, and Learmonth, respectively (Table 1).
Figures 1C and D show SCx (+ +) and SCy (+ -) at HER in the morning (Figure 1C) and SCx (- +) and SCy (- +) at LRM in the afternoon (Figure 1D), showing the morning/afternoon asymmetry both in SCx and SCy in the southern hemisphere, although Bx at HER is not sensitive to the PI/MI currents. The SCx at HER is almost DL, but SCy is clearly identified as SCy (+ -).
It is observed that the SCx at LRM is in the same polarity as SCx at MMB, while SCy in the southern hemisphere is in opposite polarity to SCy in the northern hemisphere in both the morning and afternoon. The SCx in the southern hemisphere except for SCx (+ +) at HER matches the two-cell Hall current vortices that are mirror images of the Hall current vortices in the northern hemisphere. The SCy in the southern hemisphere matches the Pedersen currents that are mirror images of those in the northern hemisphere (Figure 6).
Figure 2 shows SCx at low latitude, Alibag (ABG, 10.76° GML) and the equator, Tirunelveli (TIR, 0.52° GML), located at noon (11.9 MLT) together with the EEJ defined as the difference in Bx between TIR and ABG. The SCx at ABG is a step-like increase, which is a typical DL caused by the magnetopause current. The SCx at TIR is SCx (- +), which is typical at the equator on the dayside (Araki, 1994). The Pedersen currents responsible for the midlatitude PIy connect to the Cowling currents responsible for the PIx at TIR, completing a Pedersen–Cowling current circuit between high latitude and equator (Figure 6). Likewise, midlatitude MIy is caused by the Pedersen current circuit between the MI FACs and the Cowling currents with opposite directions to those of PI.
FIGURE 2. SCx recorded at low latitude, Alibag (ABG) and equator, Tirunelveli (TIR). EEJ refers to SCx associated with the equatorial electrojet derived from the difference between SCx at TIR and SCx at ABG.
Figure 3 shows zoomed-in plots of the PI at high latitude-equatorial stations in a 3-min time frame where one increment is 10 s. To show the clear onset of the PI, we plotted Bx at TJO and EEJ with solid curves and By at UPS, LRM, and SYO with dashed curves, where LRM is magnified five times for better visibility. We also plotted Bx at UPS to show that the onset of PIx can be delayed, as will be discussed later. The PIx/PIy starts at 0637:30 s UT (vertical dotted line) at all the stations within the resolution of a few seconds. It is remarkable that the onset of the PI is simultaneous at the conjugate auroral stations (TJO, SYO) and at the midlatitude and equatorial stations. The observations meet the instantaneous development of ionospheric Hall and Pedersen–Cowling currents from high latitude to the equator, which is achieved by the TM0/TEM mode waves propagating at the speed of light in the Earth-ionosphere waveguide/transmission line (Kikuchi et al., 1978; Kikuchi and Araki, 1979; Kikuchi, 2014).
FIGURE 3. Zoomed-in plots of PIx (solid curve) and PIy (dashed curve). LRM is magnified five times for better visibility. The horizontal dotted lines refer to the pre-event level for better visibility of the onset.
Figure 4 shows the SC-associated HF Doppler frequencies (SCF) observed at Kasperk, Czechia, in the morning and at Iitate, Sugadaira, Fujisawa, Japan, in the afternoon. Three SCFs at Kasperk were obtained from HF radio signals transmitted by three transmitters, Tx1, Tx2, and Tx3, as received at one receiver (Table 2). The SCF is SCF (+ -) in both the morning and afternoon, composed of the positive PFD with a duration of 1 min and negative MFD with a duration of 4 min. The PFD and MFD are caused by the westward and eastward electric fields, that is, the dayside part of the dusk-to-dawn and dawn-to-dusk electric fields of the PI and MI, respectively. These electric fields drive the Hall current vortices responsible for the PIx and MIx at UPS and MMB. It should be stressed that the PFD is caused by the potential electric field transmitted with the Pedersen currents responsible for the equatorial PI (Kikuchi et al., 2016). The onset of the PFD at Kasperk is 0637:30UT, the same as the PI of global currents (Figure 3). The onset of the PFD cannot be identified very clearly at stations in the afternoon, which may be due to ionospheric conditions over Japan.
FIGURE 4. SC-associated HF Doppler frequencies (SCF) observed at Kasperk, Czechia, in the morning (AM) and at Iitate (IIT), Sugadaira (SGD) and Fujisawa (FJS), Japan, in the afternoon (PM). SCF at Kasperk is observed using three transmitters (Tx1, Tx2, and Tx3) and one receiver. Horizontal dotted lines are given to all the Doppler data to indicate zero Doppler frequency. The SCF is composed of PFD and MFD caused by the PI and MI electric fields, respectively, in both morning and afternoon.
We reproduced SC electric fields at the middle latitude and magnetic field due to the Cowling currents using the REPPU (REProduce Plasma Universe) global simulation model, where the current continuity equation is solved in the anisotropic and inhomogeneous conducting ionosphere at the inner boundary (2.6 Re) of the magnetosphere (Tanaka et al., 2020). At the simulation boundary upstream in the solar wind, the IMF Bz changes from +3 nT to −5 nT and solar wind speed from 300 to 500 km/s in the form of a step function. The solar wind density and IMF By are constant at 5/cc and 2.5 nT, respectively (Ebihara et al., 2014). Figures 5A and B show the electric fields for Kasperk (Figure 5A) and Japanese stations (Figure 5B). The reproduced electric fields in the morning and afternoon are in good agreement with the observed SCF (Figure 4) in terms of time scale and polarity (note that Doppler frequency is positive for westward electric field). The good agreement between the simulation and observations suggests that the PI and MI electric fields are potential fields associated with ionospheric currents.
FIGURE 5. SC electric fields composed of the westward PI and eastward MI electric fields are reproduced by the REPPU global simulation model for latitudes and local times applicable to Kasperk, Czechia, in (A) and Japanese stations in (B). SC(- +) is reproduced by the same model for Tirunelveli at the geomagnetic equator at noon in (C).
Figure 5C shows the reproduced equatorial SCx (- +) caused by the Cowling currents at 12 MLT, which is consistent with the SCx (- +) observed at TIR (Figure 2). In the model calculation where the current continuity equation is employed, instantaneous propagation of the electric field and currents is apriori assumed. The speed of light propagation of the TM0/TEM mode waves provides a physical basis for the instantaneous propagation in the model ionosphere.
Figure 6 shows a schematic diagram of the ionospheric current circuits that explain the global characteristics of the observed PI in the northern and southern hemispheres and at the equator on the dayside. The ionospheric current circuits are composed of two-cell Hall current vortices at high and middle latitudes (orange circles) and the Pedersen currents (blue semicircles) extending from the foot of FACs (thick blue arrows) to the equatorial Cowling currents (black arrow). The two-cell current vortices of the PI were described as the equivalent currents estimated from ground magnetic variations (Nagata and Abe, 1955). The ionospheric current pattern is supposed to be affected by the ionospheric conductivities, particularly at auroral latitudes, where the foot of FACs is located and ionospheric conductivities are enhanced by precipitating auroral particles. However, the SC most often occurs during quiet periods prior to stormtime disturbances, as is the case on 12 May 2021, which would allow us to use the simplified two-cell Hall current vortices as shown in Figure 6. The current circuits in the northern and southern hemispheres are mirror images of each other, so the following discussion applies to the northern hemisphere.
FIGURE 6. Schematic diagram of the Hall (orange circles) and Pedersen–Cowling (blue semi-circles and black arrow at the equator) current circuits for the PI of SC. Hall currents are anti-clockwise in the morning and clockwise in the afternoon, surrounding the FACs (thick blue arrows). The Pedersen currents flow from the foot of FACs to the Cowling currents via the midlatitude ionosphere. HF Doppler frequency, △f, is shown with an electric field (green arrow) parallel to the Pedersen currents.
The pair of FACs of the PI provides electric potentials to the polar ionosphere from the dynamo created by the compression of the magnetosphere (Tamao, 1964a; Tamao, 1964b; Slinker et al., 1999; Fujita et al., 2003a). The electric potentials are then transmitted with the Pedersen currents to the global ionosphere by the TM0/TEM mode wave in the Earth-ionosphere waveguide/transmission line (Kikuchi, 2014). The magnetic field of the TM0 mode propagating equatorward is negative/positive By, and the Pedersen currents are northward/southward in the morning/afternoon. The disseminated potential electric fields drive counterclockwise/clockwise Hall current vortices closing around the upward/downward FACs in the morning/afternoon, as shown in Figure 6. The Hall current vortices cause positive/negative PIx as observed at middle latitudes in the morning/afternoon (Figure 1). The upward/downward FACs are connected to the equatorial ionosphere in the morning/afternoon via the northward/southward Pedersen currents, which cause the negative/positive PIy as observed at middle latitudes (Figure 1). The Pedersen–Cowling current circuit is achieved near-instantaneously, causing the negative PIx at the dayside equator (Figure 2).
We found that the onset of PIy is simultaneous with the temporal resolution of a few seconds in both the northern and southern hemispheres, even when PIx is delayed by tens of seconds, as seen in PIx at UPS in Figure 3. The delay could be due to the fact that the Pedersen current had a westward component and canceled the Hall current effect. The instantaneous transmission of the electric potentials is achieved by the TM0/TEM mode waves in the Earth-ionosphere waveguide/transmission line, which carries the Pedersen currents together with ground surface currents connected by the wave front currents (Kikuchi, 2014). We stress that the By component is associated with the TM0/TEM mode propagating southward, which helps confirm the simultaneous onset of the global PIy.
Concerning the instantaneous propagation from high latitude to low latitude of the electric field/magnetic field, lots of studies have used the fast (compressional) mode waves propagating at the Alfven speed perpendicular to the ambient magnetic field in the upper ionosphere (Tu and Song, 2019) or the Alfven waves converted from the compressional mode (Tamao, 1964a; Tamao, 1964b; Chi et al., 2006). However, no theory/model based on the fast mode waves has succeeded to explain the Hall and Pedersen–Cowling current circuits responsible for the PI and MI on the ground. Tamao (1964b) attempted to explain the Hall current vortices by means of the converted transverse mode (CT mode) converted from the compressional mode at its wave front. It should be recalled that the Hall current is a result of ion-neutral collisions in the ionospheric E-layer, which decouple the ion motion from that of the collision-free electrons and create the Pedersen currents as well. Therefore, a theory/model that aims for explaining the Hall current circuits should also explain the Pedersen current circuits. The CT mode never carries FACs, since the electric field of the CT mode is solenoidal (rotational) (Tamao, 1964b), so the Pedersen currents are not supplied by the CT mode or by the compressional mode. Kikuchi (2014) showed that the TM0/TEM mode transports electric potentials and the Pedersen currents from the foot of the FACs to the Cowling currents via the midlatitude. The TM0/TEM mode does not have the cutoff frequency of the waveguide, which allows any period of disturbances such as the SC, DP2 and so on. It should be noted that the Schumann resonance frequency at about 8 Hz is misunderstood as the lower cutoff frequency of the Earth-ionosphere waveguide (Lysak et al., 2015). The Schuman resonance is a global cavity resonance created by the propagating TM0 mode waves in the Earth-ionosphere waveguide (Wait, 1960). Wait (1960) describes that “Schumann [1956] has carried out an extensive set of calculations of waveforms for the zero mode in the idealized Earth-ionosphere waveguide”. The TM0 waveguide mode is the TEM transmission line mode that is unattenuated at all frequencies (Budden, 1961, p33). It should also be noted that the plasma motion observed by HF Doppler sounders is upward on the dayside during the MI phase of an SC event which is well correlated with the eastward Cowling currents (Kikuchi et al., 2016). This fact indicates that the ionospheric plasma is never compressed by the compressional mode wave but is moved by the electric field transmitted with the Pedersen currents. It should be stressed that the theory/model designed to explain the fast propagation of electric and magnetic fields to low latitude should also explain the energy supply to the Pedersen and Cowling currents.
The HF Doppler sounders detected downward motion of the ionospheric plasma for the first 1 min, followed by the upward motion for 4 min in both the morning and afternoon (Figure 4). The initial downward motion was interpreted as caused by the compressional wave (Huang, 1976; Pilipenko et al., 2010). The magnetospheric plasma is compressed earthward during the SC, as observed by the Akebono satellite (Shinbori et al., 2004). However, Kikuchi et al. (2016) showed that the dayside ionosphere never moves earthward (downward) but moves upward as the eastward Cowling current grows during the MI phase when the magnetosphere continues to be compressed. The electric field responsible for the upward motion is not the electric field of the compressional wave but is the potential field associated with the ionospheric Pedersen/Cowling current. The initial downward motion in Figure 4 occurred during the period of the equatorial PI caused by the westward Cowling current (Figure 2), which indicates that the PI electric field at middle latitude is transmitted by the TM0/TEM mode in the Earth-ionosphere waveguide/transmission line.
Tsunomura (1998) showed that the midlatitude MIy is positive in the morning and negative in the afternoon, and its amplitude is larger at higher latitudes. These observations meet the Pedersen current circuit, as shown in Figure 6. The latitudinal variation of MIx, on the other hand, is not so significant, implying that MIx is superimposed by DL. A similar difference in the latitudinal profiles between Bx and By was found for Pi2 magnetic pulsation, of which period (1 min) is the same as the time scale of PI (Yumoto et al., 1994). Yumoto et al. (1994) showed that Pi2 in the By component is in an anti-phase relationship in the northern and southern hemispheres, and the amplitude increases exponentially at higher latitudes. Imajo et al. (2015), Imajo et al. (2017) made detailed analyses of Pi2 in By and suggested that the anti-phase relationship is caused by ionospheric currents that are symmetric with respect to the equator. The latitudinal and hemispheric characteristics of Pi2 are similar to those of PIy and MIy reported in the present article, consistent with the Pedersen–Cowling current circuit as depicted in Figure 6.
We analyzed the geomagnetic sudden commencement (SC) on 12 May 2021 with magnetometer data recorded in the morning and afternoon at high and middle latitudes in the northern and southern hemispheres and at the noontime equator. The electric field of the SC was observed by the HF Doppler sounders at middle latitudes in the morning and afternoon in the northern hemisphere. Local time, latitude, and hemispheric characteristics are obtained as follows.
1) SC in Bx- and By-components are SCx (+ -) and SCy (- +) in the morning and SCx (- +) and SCy (+ -) in the afternoon at middle latitudes in the northern hemisphere. SCx in the southern hemisphere has the same polarity as those in the northern hemisphere except for SCx (+ +) in the morning, while SCy in the southern hemisphere has reverse polarity to those in the northern hemisphere.
2) Preliminary impulse in Bx (PIx) in the northern hemisphere matches the conventional two-cell Hall current vortices with anti-clockwise and clockwise directions in the morning and afternoon, respectively, and the MIx matches reverse Hall current vortices. PIx and MIx in the southern hemisphere meet the Hall current vortices that are mirror images of those in the northern hemisphere with respect to the equator.
3) PIy in the northern hemisphere is found to meet the northward and southward Pedersen currents in the morning and afternoon, respectively, and the MIy meets reverse Pedersen currents. The PIy and MIy in the southern hemisphere are found to meet the Pedersen currents that are mirror images of those in the northern hemisphere.
4) At the equator, typical SCx (- +) is observed, meeting the Cowling currents supplied by the Pedersen currents from the northern and southern hemispheres.
5) The electric fields of the PI and MI observed by the HF Doppler sounders at the middle latitudes in the northern hemisphere are westward and eastward, respectively, in both the morning and afternoon, meeting the conventional model composed of dusk-to-dawn PI and dawn-to-dusk MI potential electric fields.
6) The onset of the PIx/PIy is found to be simultaneous with the resolution of a few seconds in both the northern and southern hemispheres, indicating instantaneous achievement of the Hall and Pedersen–Cowling current circuits by the TM0/TEM mode wave propagating at the speed of light in the Earth-ionosphere waveguide/transmission line.
7) REPPU global simulation model reproduces the PI and MI electric fields at middle latitudes and SCx (- +) at the dayside equator. The simulation results are found to be consistent with most features of observations, such as the time scale of PI and MI, direction of the midlatitude electric field, and generation of equatorial Cowling currents. The simulation proves that the ionospheric electric fields are potential fields transmitted in the Pedersen–Cowling current circuit.
Publicly available datasets were analyzed in this study. This data can be found at 1. HF Doppler: HF Doppler Sounding Experiment in Japan-HFDOPE, The University of Electro-Communications (http://gwave.cei.uec.ac.jp/∼hfd/) 2. HF Doppler. The Institute of Atmospheric Physics of the Czech Academy of Science, (http://datacenter.ufa.cas.cz/) 3. Magnetometer data: World Data Center for Geomagnetism, Kyoto (http://wdc.kugi.kyoto-u.ac.jp/wdc/Sec3.html) 4. Magnetometer data: Kakioka Magnetic Observatory (http://www.kakioka-jma.go.jp/obsdata/metadata/en) 5. Magnetometer data: World Data Centre for Geomagnetism, Mumbai (http://wdciig.res.in/WebUI/Home.aspx) Us | Indian Institute of Geomagnetism (IIG) (iigm.res.in) 6. Magnetometer data: Data Center for Aurora, National Institute of Polar Research polaris.nipr.ac.jp/∼uap-mon/uapm/uapm_new_top.html 7. Magnetometer data: INTERMAGNET website (http://www.intermagnet.org).
TK and KKH analyzed the magnetometer and HF Doppler data for the SC event. TA and YN provided ideas about the global current circuits. YE and TT performed global simulations of SC electric field and currents. GV and AS performed magnetometer observations in India and provided suggestions on the onset of PI at the equator. JC performed HF Doppler observations in Czechia. KH and IT performed HF Doppler observations in Japan. YT and AK performed magnetometer observations at TJO and SYO.
This study is supported by the grants-in-aid for Scientific Research (15H05815, 20H01960) of Japan Society for the Promotion of Science (JSPS) and by JSPS KAKENHI Grant Number JP22540461 and JP26400481. The works of TK are supported by the joint research program of the Institute for Space-Earth Environmental Research, Nagoya University, the KDK of the Research Institute for Sustainable Humanosphere, Kyoto University, and National Institute of Polar Research, Tokyo. The work of JC was supported under the grant 18-01969S by the Czech Science Foundation. The works of IT, KKH, KH, and TK are supported by Hoso Bunka Foundation, the Takahashi Industrial and Economic Research Foundation and the Murata Science Foundation. The works of YN is supported by NASA Grant 80NSSC18K0657, 80NSSC20K0604, 80NSSC20K0725, 80NSSC21K1321, and 80NSSC20K1788, NSF Grant AGS-1907698 and AGS-2100975, and AFOSR Grant FA9559-16-1-0364.
The authors declare that the research was conducted in the absence of any commercial or financial relationships that could be construed as a potential conflict of interest.
All claims expressed in this article are solely those of the authors and do not necessarily represent those of their affiliated organizations, or those of the publisher, the editors, and the reviewers. Any product that may be evaluated in this article, or claim that may be made by its manufacturer, is not guaranteed or endorsed by the publisher.
The results presented in this article rely on the data collected at Hermanus, Uppsala, Learmonth and Memambetsu. The authors thank the South African National Space Agency (SANSA) for data from Hermanus, Uppsala Geological Survey of Sweden (SGU) for Uppsala, Geoscience Australia (GA) for Learmonth and Kakioka Magnetic Observatory, and Japan Meteorological Agency (JMA) for Memambetsu. The authors thank INTERMAGNET for promoting high standards of magnetic observatory practice (www.intermagnet.org). The authors also thank the National Institute of Polar Research for data from Tjornes and Syowa and the Indian Institute of Geomagnetism for Alibag and Tirunelveli.
DL, stepwise low latitude magnetic disturbance; EEJ, equatorial electrojet; GML, geomagnetic latitude; HF, high frequency; MFD, main frequency deviation; MHD, magnetohydrodynamic; MI, main impulse; MLT, magnetic local time; PFD, preliminary frequency deviation; PI, preliminary impulse; SC, geomagnetic sudden commencement; SCF, SC-associated Doppler frequency; TM0, zeroth-order transverse magnetic.
Araki, T., Allen, J. H., and Araki, Y. (1985). Extension of a Polar Ionospheric Current to the Nightside Equator. Planet. Space Sci. 33, 11–16. doi:10.1016/0032-0633(85)90137-0
Araki, T., Keika, K., Kamei, T., Yang, H., and Alex, S. (2006). Nighttime Enhancement of the Amplitude of Geomagnetic Sudden Commencements and its Dependence on IMF-Bz. Earth Planet Sp. 58, 45–50. doi:10.1186/bf03351912
Araki, T. (1977). Global Structure of Geomagnetic Sudden Commencements. Planet. Space Sci. 25, 373–384. doi:10.1016/0032-0633(77)90053-8
Araki, T. (1994). A Physical Model of the Geomagnetic Sudden Commencement, Solar Wind Sources of Magnetospheric Ultra-low-frequency Waves. Geophys. Monogr. 81, 183–200.
Baker, W. G., and Martyn, D. F. (1953). Electric Currents in the Ionosphere I. The Conductivity. Phil. Trans. R. Soc. Lond. Ser. A 246, 281–294.
Budden, K. G. (1961). The Wave-Guide Mode Theory of Wave Propagation. Academic Press Inc. London, 33–34.
Chi, P. J., Lee, D.-H., and Russell, C. T. (2006). Tamao Travel Time of Sudden Impulses and its Relationship to Ionospheric Convection Vortices. J. Geophys. Res. 111, A08205. doi:10.1029/2005JA011578
Chum, J., Šindelářová, T., Laštovička, J., Hruška, F., Burešová, D., and Baše, J. (2010). Horizontal Velocities and Propagation Directions of Gravity Waves in the Ionosphere over the Czech Republic. J. Geophys. Res. 115. doi:10.1029/2010JA015821
Ebihara, Y., Tanaka, T., and Kikuchi, T. (2014). Counter Equatorial Electrojet and Overshielding after Substorm Onset: Global MHD Simulation Study. J. Geophys. Res. Space Phys. 119, 7281–7296. doi:10.1002/2014JA020065
Fujita, S., Tanaka, T., Kikuchi, T., Fujimoto, K., Hosokawa, K., and Itonaga, M. (2003a). A Numerical Simulation of the Geomagnetic Sudden Commencement: 1. Generation of the Field-Aligned Current Associated with the Preliminary Impulse. J. Geophys. Res. 108 (A12), 1416. doi:10.1029/2002JA009407
Fujita, S., Tanaka, T., Kikuchi, T., Fujimoto, K., and Itonaga, M. (2003b). A Numerical Simulation of the Geomagnetic Sudden Commencement: 2. Plasma Processes in the Main Impulse. J. Geophys. Res. 108 (A12), 1417. doi:10.1029/2002JA009763
Hirono, M. (1952). A Theory of Diurnal Magnetic Variations in Equatorial Regions and Conductivity of the Ionosphere E Region. J. Geomagn. Geoelec. 4, 7–21. doi:10.5636/jgg.4.7
Huang, Y.-N., Najita, K., and Yuen, P. (1973). The Ionospheric Effects of Geomagnetic Sudden Commencements as Measured with an HF Doppler Sounder at Hawaii. J. Atmos. Terr. Phys. 35, 173–181. doi:10.1016/0021-9169(73)90225-0
Huang, Y.-N. (1976). Modeling HF Doppler Effects of Geomagnetic Sudden Commencements. J. Geophys. Res. 81, 175–182. doi:10.1029/ja081i001p00175
Imajo, S., Yoshikawa, A., Uozumi, T., Ohtani, S., Nakamizo, A., Marshall, R., et al. (2015). Pi2 Pulsations Observed Around the Dawn Terminator. J. Geophys. Res. Space Phys. 120, 2088–2098. doi:10.1002/2013JA019691
Imajo, S., Yoshikawa, A., Uozumi, T., Ohtani, S., Nakamizo, A., and Chi, P. J. (2017). Application of a Global Magnetospheric‐ionospheric Current Model for Dayside and Terminator Pi2 Pulsations. J. Geophys. Res. Space Phys. 122, 8589–8603. doi:10.1002/2017JA024246
Kikuchi, T., and Araki, T. (1979). Horizontal Transmission of the Polar Electric Field to the Equator. J. Atmos. Terr. Phys. 41, 927–936. doi:10.1016/0021-9169(79)90094-1
Kikuchi, T., Araki, T., Maeda, H., and Maekawa, K. (1978). Transmission of Polar Electric Fields to the Equator. Nature 273, 650–651. doi:10.1038/273650a0
Kikuchi, T., Ishimine, T., and Sugiuchi, H. (1985). Local Time Distribution of HF Doppler Frequency Deviations Associated with Storm Sudden Commencements. J. Geophys. Res. 90, 4389–4393. doi:10.1029/ja090ia05p04389
Kikuchi, T., Hashimoto, K. K., Tomizawa, I., Ebihara, Y., Nishimura, Y., Araki, T., et al. (2016). Response of the Incompressible Ionosphere to the Compression of the Magnetosphere during the Geomagnetic Sudden Commencements. J. Geophys. Res. Space Phys. 121, 1536–1556. doi:10.1002/2015ja022166
Kikuchi, T., Chum, J., Tomizawa, I., Hashimoto, K. K., Hosokawa, K., Ebihara, Y., et al. (2021). Penetration of the Electric Fields of the Geomagnetic Sudden Commencement over the Globe as Observed with the HF Doppler Sounders and Magnetometers. Earth Planets Space 73. doi:10.1186/s40623-020-01350-8
Kikuchi, T. (1986). Evidence of Transmission of Polar Electric Fields to the Low Latitude at Times of Geomagnetic Sudden Commencements. J. Geophys. Res. 91, 3101–3105. doi:10.1029/ja091ia03p03101
Kikuchi, T. (2014). Transmission Line Model for the Near-Instantaneous Transmission of the Ionospheric Electric Field and Currents to the Equator. J. Geophys. Res. Space Phys. 119, 1131–1156. doi:10.1002/2013JA019515
Lysak, R. L., Song, Y., Sciffer, M. D., and Waters, C. L. (2015). Propagation of Pi2 Pulsations in a Dipole Model of the Magnetosphere. J. Geophys. Res. Space Phys. 120, 355–367. doi:10.1002/2014JA020625
Nagata, T., and Abe, S. (1955). Notes on the Distribution of SC* in High Latitudes. Rept. Ionos. Res. Jpn. 9, 39–44.
Nakata, H., Nozaki, K., Oki, Y., Hosokawa, K., Hashimoto, K. K., Kikuchi, T., et al. (2021). Software-defined Radio-Based HF Doppler Receiving System. Earth Planets Space 73. doi:10.1186/s40623-021-01547-5
Nilam, B., Ram, S. T., Shiokawa, K., Balan, N., and Zhang, Q. (2020). The Solar Wind Density Control on the Prompt Penetration Electric Field and Equatorial Electrojet. J. Geophys. Res. Space Phys. 125, e2020JA027869. doi:10.1029/2020JA027869
Pilipenko, V., Fedorov, E., Yumoto, K., Ikeda, A., and Sun, T. R. (2010). An Analytical Model for Doppler Frequency Variations of Ionospheric HF Sounding Caused by SSC. J. Geophys. Res. 115. doi:10.1029/2010JA015403
Rastogi, R. G., Pathan, B. M., Rao, D. R. K., Sastry, T. S., and Sastri, J. H. (2001). On Latitudinal Profile of Storm Sudden Commencement in H, Y and Z at Indian Geomagnetic Observatory Chain. Earth Planet Sp. 53, 121–127. doi:10.1186/BF03352369
Rastogi, R. G. (1993). Longitudinal Variation of Sudden Commencement of Geomagnetic Storm at Equatorial Stations. J. Geophys. Res. 98, 15,411–15,416. doi:10.1029/92ja02971
Sastri, J. H. (2002). Penetration Electric Fields at the Nightside Dip Equator Associated with the Main Impulse of the Storm Sudden Commencement of 8 July 1991. J. Geophys. Res. 107 (A12), 9–1. doi:10.1029/2002JA009453
Shinbori, A., Ono, T., Iizima, M., and Kumamoto, A. (2004). SC Related Electric and Magnetic Field Phenomena Observed by the Akebono Satellite inside the Plasmasphere. Earth Planet Sp. 56, 269–282. doi:10.1186/bf03353409
Shinbori, A., Tsuji, Y., Kikuchi, T., Araki, T., and Watari, S. (2009). Magnetic Latitude and Local Time Dependence of the Amplitude of Geomagnetic Sudden Commencements. J. Geophys. Res. 114. doi:10.1029/2008JA013871
Slinker, S. P., Fedder, J. A., Hughes, W. J., and Lyon, J. G. (1999). Response of the Ionosphere to a Density Pulse in the Solar Wind: Simulation of Traveling Convection Vortices. Geophys. Res. Lett. 26, 3549–3552. doi:10.1029/1999gl010688
Tamao, T. (1964a). The Structure of Three-Dimensional Hydromagnetic Waves in a Uniform Cold Plasma. J. Geomagn. Geoelec. 16, 89–114. doi:10.5636/jgg.16.89
Tamao, T. (1964b). Hydromagnetic Interpretation of Geomagnetic SSC. Rep. Ionos. Space Res. Jpn. 18, 16–31.
Tanaka, T., Ebihara, Y., Watanabe, M., Den, M., Fujita, S., Kikuchi, T., et al. (2020). Reproduction of Ground Magnetic Variations during the SC and the Substorm from the Global Simulation and Biot‐Savart's Law. J. Geophys. Res. Space Phys. 125, e2019JA027172. doi:10.1029/2019ja027172
Tanaka, T. (2007). Magnetosphere-Ionosphere Convection as a Compound System. Space Sci. Rev. 133, 1–72. doi:10.1007/s11214-007-9168-4
Tsunomura, S. (1998). Characteristics of Geomagnetic Sudden Commencement Observed in Middle and Low Latitudes. Earth Planet Sp. 50, 755–772. doi:10.1186/bf03352168
Tsunomura, S. (1999). Numerical Analysis of Global Ionospheric Current System Including the Effect of Equatorial Enhancement. Ann. Geophys. 17, 692–706. doi:10.1007/s00585-999-0692-2
Tu, J., and Song, P. (2019). On the Momentum Transfer from Polar to Equatorial Ionosphere. J. Geophys. Res. Space Phys. 124, 6064–6073. doi:10.1029/2019JA026760
Vichare, G., Rawat, R., Bhaskar, A., and Pathan, B. M. (2014). Ionospheric Current Contribution to the Main Impulse of a Negative Sudden Impulse. Earth Planets Space 66, 92. doi:10.1186/1880-5981-66-92
Wait, J. R. (1960). On the Theory of the Slow-Tail Portion of Atmospheric Waveforms. J. Geophys. Res. 65, 1939–1946. doi:10.1029/jz065i007p01939
Yumoto, K., Osaki, H., Fukao, K., Shiokawa, K., Tanaka, Y., Solovyev, S. I., et al. (1994). Correlation of High- and Low-Latitude Pi 2 Magnetic Pulsations Observed at 210° Magnetic Meridian Chain Stations. J. Geomag. Geoelectr. 46, 925–935. doi:10.5636/jgg.46.925
Keywords: geomagnetic sudden commencement, Hall current circuit, Pedersen–Cowling current circuit, simultaneous onset of preliminary impulse, morning/afternoon symmetry/asymmetry, northern and southern hemispheric symmetry/asymmetry, HF Doppler sounder, global simulation
Citation: Kikuchi T, Araki T, Hashimoto KK, Ebihara Y, Tanaka T, Nishimura Y, Vichare G, Sinha AK, Chum J, Hosokawa K, Tomizawa I, Tanaka Y and Kadokura A (2022) Instantaneous Achievement of the Hall and Pedersen–Cowling Current Circuits in Northern and Southern Hemispheres During the Geomagnetic Sudden Commencement on 12 May 2021. Front. Astron. Space Sci. 9:879314. doi: 10.3389/fspas.2022.879314
Received: 19 February 2022; Accepted: 12 April 2022;
Published: 31 May 2022.
Edited by:
David Gary Sibeck, National Aeronautics and Space Administration, United StatesReviewed by:
Arnaud Masson, European Space Astronomy Centre (ESAC), SpainCopyright © 2022 Kikuchi, Araki, Hashimoto, Ebihara, Tanaka, Nishimura, Vichare, Sinha, Chum, Hosokawa, Tomizawa, Tanaka and Kadokura. This is an open-access article distributed under the terms of the Creative Commons Attribution License (CC BY). The use, distribution or reproduction in other forums is permitted, provided the original author(s) and the copyright owner(s) are credited and that the original publication in this journal is cited, in accordance with accepted academic practice. No use, distribution or reproduction is permitted which does not comply with these terms.
*Correspondence: Takashi Kikuchi, a2lrdWNoaUBpc2VlLm5hZ295YS11LmFjLmpw
Disclaimer: All claims expressed in this article are solely those of the authors and do not necessarily represent those of their affiliated organizations, or those of the publisher, the editors and the reviewers. Any product that may be evaluated in this article or claim that may be made by its manufacturer is not guaranteed or endorsed by the publisher.
Research integrity at Frontiers
Learn more about the work of our research integrity team to safeguard the quality of each article we publish.