- International Arctic Research Center, University of Alaska, Fairbanks, AK, United States
All of us encounter our unique difficulties as scientists. Although the kind of difficulty varies by scientist, I describe my own in this program, and explain how I responded, together with a sort of moral. They are: (a) When theorists seemed to encounter a deadlock, (b) When a well-accepted theory is confronted with a seriously contradictory observational fact, (c) When there is a generally believed morphology of a phenomenon that does not agree with my observations, (d) When a single approach or theory prevails, but I have a different idea. Obviously, my responses are just an attempt, which will be judged by future developments in space physics.
Introduction
Space physics began to develop in the 1960s by combining parts of geomagnetism (a study of geomagnetic storms), ionospheric physics, auroral physics, planetary physics and solar physics under the advent of satellite observations. My first paper in the Journal of Geophysical Research, co-authored with Sydney Chapman, was published in 1961. I have witnessed the development of space physics from its early days.
I believe that every young scientist knows how research should be conducted in general terms. Thus, for the purpose of this particular program, I try to describe plainly my humble experiences, when I encountered difficult problems, which had been and have been lasted for a long time in space physics; how long each difficulty had or have lasted will be mentioned in each topic.
Some of the difficulties include: (a) when theorists seemed to encounter a deadlock for a long time; (b) when there is a well-accepted theory, but there seems to exist a seriously contradicting observed fact; (c) when there was a generally accepted morphology of a phenomenon, which does not seem to agree with my observations and (d) when a single theoretical approach or theory has prevailed for a long time, but I have a different idea.
Most readers must have encountered some of these or similar problems. In fact, some of the above problems have been what all space physicists share even at the present time. It is my hope that the readers will get some hints from how I responded, whether it proved to be right or wrong or will be judged by future progress in space physics.
When Theorists Seemed to Encounter a Deadlock
The “Unknown Parameter” in the Solar Wind
Sydney Chapman theorized the interaction between solar plasma flow and the Earth’s dipole magnetic field in 1931; it became the basis of magnetospheric physics and space physics, including planetary physics. Chapman and others established also the concept of the standard geomagnetic storm; it begins with the storm sudden commencement (SSC) as storm onset, which is followed by the initial and main phases; a step function-like increase of SSC indicates the arrival of an intensified solar wind; here, the intensity of the plasma flow is proportional to the kinetic pressure of the solar wind, which is also proportional to the magnitude of the SSC, being typically 25 nT (Chapman’s 1931 theory); Figure 1A.
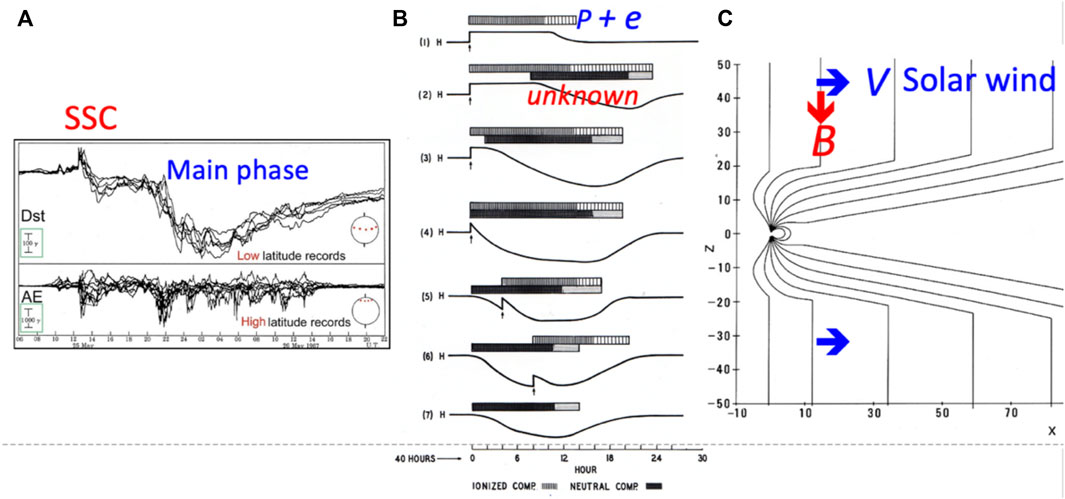
FIGURE 1. (A) Magnetic records of a number of stations from both low latitudes (upper) and low latitudes (lower) of a geomagnetic storm (only the horizontal component); the circular insert show approximate location of the observatories. They are typical geomagnetic records, and it can be considered as a standard type of geomagnetic storm. (B) It illustrates a great variety of geomagnetic storms; (p + e) indicates the case, in which the solar wind consists of only protons and electrons; “unknown” indicates that it was thought that the solar wind had some unknown parameter causing the main phase. (C) The linked magnetic field between IMF and magnetospheric field; this interaction constitutes the solar wind-magnetosphere dynamo, proving the power fo the main phase and the aurora.
Since then, Chapman and many researchers had tried to find the way for the solar plasma particles to enter into the magnetosphere in order to explain the main phase of geomagnetic storms (the ring current around the Earth and the aurora). When I joined Chapman in 1959 as his graduate student, he asked me to pursue this problem further.
Since I did not know much about geomagnetic storms at that time, I decided to examine, first of all, a large number of geomagnetic storm records (magnetograms) from many stations. It was a great surprise to me that many geomagnetic storms began with a large SSC, indicating the arrival of an intensified (high kinetic pressure) of the solar wind, but were not followed by the main phase; further, many storms developed an intense main phase without SSC; there are many between the two, so that the concept of the standard geomagnetic storm is not appropriate.
Thus, I concluded that there must be an “unknown parameter” in the solar wind, which determines the development of the main phase, other than an intensified solar wind; Figure 1B. I recall that in one conference, I was told by a person that I was not qualified as a graduate student in space physics by not knowing that the solar wind consists of only protons and electrons. However, Chapman agreed with me about my finding, and published a joint paper (Akasofu and Chapman, 1963).
Chapman presented our result during the First International Conference on the Solar Wind, which was held at the Jet Propulsion Laboratory (JPL) in 1964. After Chapman’s talk, some people expressed their doubt about our finding, but Jim Dungey suggested that our “unknown parameter” may be the southward-oriented interplanetary magnetic field (IMF) component. His suggestion was confirmed by a satellite observation a few years later.
The importance of the interplanetary magnetic field has now been well recognized in terms of the interaction between the solar wind and the magnetosphere. The solar wind blows across the linked field lines between the IMF and the magnetospheric field, constituting the solar wind-magnetosphere dynamo, generating the power for geomagnetic storms and the aurora; Figure 1C.
A kind of moral here is that even a new graduate student can find a clue for solving a long-standing problem.
Causes of the Solar Wind
Gene Parker published the first paper on the solar wind in 1958 (he coined the term “solar wind”). However, in spite of the fact that so much has been observed and studied on details of the solar wind, its cause is still very controversial at best even after more than 60 years (cf. Viall and Borovsky, 2020).
In such a situation, one way of approaching the problem is not to pursue the problem under the basic assumptions everyone shares. In this case, many theoretical researchers’ work have been based on the high temperature of the corona in order to overcome the powerful solar gravitational force; in fact, I used to say: “the corona is so hot that it blows away by itself”.
The solar wind is a heliospheric phenomenon, so that a very large-scale force throughout the heliosphere is needed to propel the whole heliospheric plasma outward. Further, the needed force must be a very powerful (J x B) force, which can propel the whole heliospheric plasma with a speed of up to 800 km/s. The question was then what kind of current system might be able to produce such a (J x B) force, rather than low level processes in the corona. In 1950, Alfven (1981) worked on the solar unipolar induction current system, which can be generated by a rotating dipolar magnetic body like the sun surrounded by plasma. It has the needed configuration and the requirements. An estimate indicated that the (J x B) force can propel the heliospheric plasma with a speed of 200 km/s (Lee and Akasofu, 2021). We are currently trying to search for processes to provide additional 500 km/s (Figure 2).
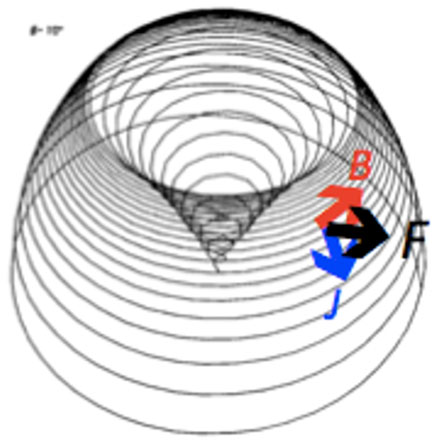
FIGURE 2. One of the magnetic field lines generated by the solar unipolar induction current system. The current J, magnetic field direction B and the direction of the outward force F by the (J x B) force are indicated.
After Parker introduced his theory of the solar wind in 1958, we have not yet solved the problem. It is taking more than 60 years. It may be that a radically different approach is needed in such a situation, for example introducing the (J x B) force and others.
When a Well-Accepted theory was Confronted With a Seriously Contradictory Observational Fact
In space physics, there is hardly a perfect theory. There is always an observed fact, which disagrees or contradicts with well-accepted theory. Unfortunately, many researchers disregard such a fact by considering that it is just an exception or anomaly.
In solar physics, there is a well-accepted theory on sunspots (Babcock, 1961), in which a thin magnetic tube emerges from below the photospheric surface by magnetic buoyancy, and the two emerging points can be identified as a pair (positive and negative, N or S) of spots, Figures 3A,B. So far, such a tube of magnetic flux has not been detected yet.
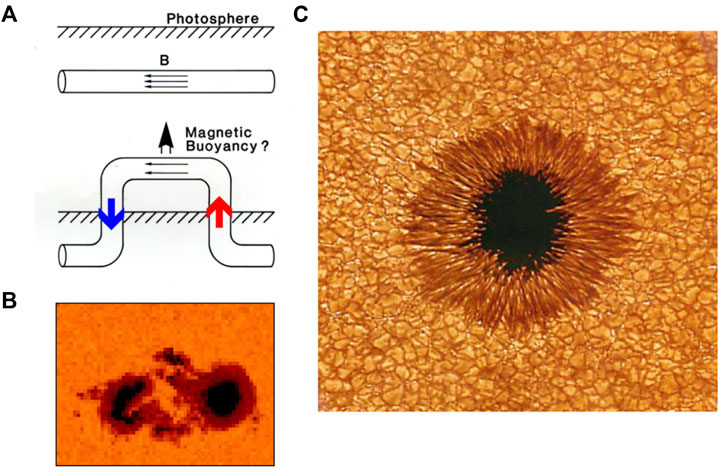
FIGURE 3. (A) A schematic illustration of Babcock’s theory of a pair of sunspots. (B) A typical example of a pair of sunspots (Courtesy of the Kitt Peak Solar Observatory). (C) A typical example of single spots (Courtesy of The Kitt Peak Solar Observatory and the NASA Sunspot Collection).
On the other hand, there exist single spots. When I took up single sunspots, I was told by two prominent solar physicists and many others that a single spot may be a “broken pipe”, so that it is wasting time to even consider them. A typical example of single spots is shown in Figure 3C.
It is puzzling why single spots have hardly been studied in the past, in spite of the fact that most standard textbooks and monographs on solar physics carry images of single spots. This may be because it has been believed from the earliest days that spots are like a magnet (which has both the N and S poles together) and because Babcock’s theory can so intuitively be understood. Another reason may be that ‘magnetic monopoles’ are not supposed to exist, so that single spots are avoided to be considered. Thus, single sunspots have almost been disregarded in the past as a “broken pipe” at best.
However, since I was convinced that single spots do exist, I tried to examine them. After I began to study magnetic fields on the photosphere, I found other contradictions. For example, there are weak, but large-scale fields, both positive and negative fields, which line up alternatively in longitude. They are called unipolar regions. They have generally been considered as old active regions stretched out by the non-uniform rotation of the sun. However, by examining unipolar magnetic fields, I found they grow and decay with the sunspot cycle.
Furthermore, the most important finding is that positive single spots are born in a positive unipolar region (vice versa), and a pair of spots is born only at the boundary (positive and negative) of neighboring unipolar regions, not in the middle of unipolar regions; these findings encouraged me to pursue further the problem. However, one of the reviewers on an early version of my paper rejected it by mentioning “a noble idea, but heretic”.
After synthesizing a number of observed facts on sunspots, I suggested how positive single spots are born in positive unipolar region and how a pair of spots are formed at the boundary of neighboring (positive and negative) unipolar regions. On the basis of these findings, I suggested that a single spot is the basic unit of sunspots, rather than a pair of spots.
The point is that under my idea, I can assemble many other features of sunspots compared with the well-accepted theory, regardless of whether my attempt is right or wrong.
This is a very important point in proposing a new idea against a well-accepted theory. For example, I can now conclude why single spots can exist [positive single spots are born in a positive unipolar region (vice versa)]; unipolar regions grow and decay with the sunspot cycle (not decaying old active regions) as generally considered (Akasofu, 2020a).
Solar physicists who are specifically working on sunspots would perhaps agree with me that the formation of sunspots is not yet solved. Babcock’s theory was published in 1961 and is well accepted in general, but it may be said that we do not really know what sunspots are.
When There is a Generally Believed Morphology of a Phenomenon Which Does Not agree With my Observations
Auroral Oval
The concept of the auroral zone was established by E. Loomis as early as in 1860. Since then, the auroral zone had long been believed to be the belt of the aurora. When I began to observe the aurora, I noticed that the aurora appeared always in the northern sky in the evening and shifted southward as the evening progressed; after midnight hours, the aurora shifted back to the northern sky. Since Fairbanks is located in the auroral zone, I thought that the aurora should appear always overhead any time; Figure 4A.
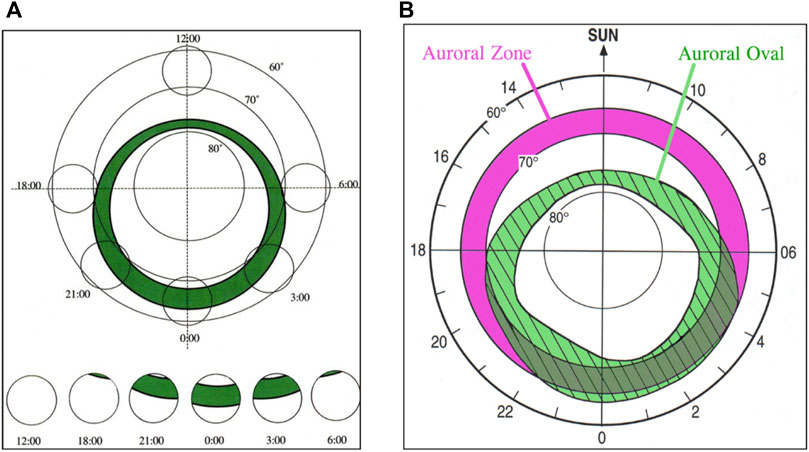
FIGURE 4. (A) Auroral belt imagined by the author. The aurora appeared almost always near the northern and reached overhead and receded in the northern sky in morning hours. The circles indicate the field of view in Fairbanks, Alaska. (B) Feldstein’s auroral oval (green) and the auroral zone (pink) in MLT coordinate system.
I asked my colleagues why my observation did not agree with Loomis’ auroral zone. They said that it was believed that the aurora was formed at the center line of the auroral zone, 67° in geomagnetic latitude and then shifted southward after it was formed. Thus, I examined all-sky images at Fort Yukon (67°, the center line of the auroral zone), and found that the aurora also appeared first in the northern sky. Then, I found also the same even at Barrow (70°, the northern edge of the auroral zone). At that time, I was busy in preparing my Ph.D. thesis on geomagnetic storms, and thus had forgotten about my observation.
Then, I found a paper by Yasha Feldstein (1963), in which he showed the belt of the aurora is actually the auroral oval, which is deformed poleward side in daylight hours; its midday location higher than 75° in latitude. His oval could explain my early auroral observation.
Thus, I thought that one way to prove the auroral oval and Feldstein’s finding was to set up a chain of all-sky cameras along the magnetic meridian line between Alaska and the northwestern tip of Greenland (located near the geomagnetic pole), which could scan the whole polar sky as the earth rotates once a day like an azimuth scan radar at an airport. This became my first grant from the National Science Foundation. This observation proved the accuracy of the auroral oval, but the result could not convince many. I found that the oval nearly coincides with the outer boundary of the outer radiation belt on the ionosphere, which was just worked out by James Van Allen of the University of Iowa; this was more convincing than the meridian scanning observation. However, the final proof had to wait until the first image of the oval by the Canadian satellite ISIS II became available in 1972; the controversy faded away after the first oval image taken from the Canadian ISIS II satellite in 1972 became available. Later, I asked the NASA plane Galileo to fly under the midday oval over the Norwegian Sea (the best location to see the midday oval in the northern hemisphere); everyone on board was excited to see the red auroral arcs.
The auroral oval is very crucial in studying polar and magnetospheric phenomena, because it provides the natural frame of reference; for example, the auroral oval surround the “roots”, of magnetic field lines which are linked with IMF field lines. Many auroral phenomena are different, depending on whether they are within, in or outside the oval.
After all, the aurora and auroral oval are fixed in the MLT-MLAT frame, but we observers rotate under them. Since the controversy on the oval was so intense, I thought that it was somewhat like the ancient situation, whether the Sun or the earth rotated.
A New Morphology of Auroral Activities: Auroral Substorms
Another example of well-established observation in the 1930s is that it had been very firmly believed that the aurora was quiet in evening hours, active in midnight hours and patchy in morning hours and that the Earth and its observers witnessed such a pattern once a night as the Earth rotated. When I found such a pattern occurred twice or three times in one very active night, I wondered instantaneously whether the Earth rotated three times in that night, since I was told that the believed pattern had so firmly been established for long time.
Thus, I decided to disregard what had been believed and analyzed simultaneous auroral activities in Siberia (evening sky), Alaska (midnight sky) and Canada (morning sky). It was my finding that such a pattern over the whole polar sky repeats several times in 24 h during active periods (once or twice a night) under which the earth rotates. The concept of auroral substorms was established on the basis of such a study based on a number of all-sky cameras located in the Arctic region (Akasofu, 1964); Figure 5. However, it took some time to convince many colleagues on my results, since they had firmly believed in the old concept. One way to observe two auroral substorms in 6 h was to fly from the East Coast to Alaska in midnight hours against the Earth’s rotation. This flight was conducted several times by the NASA Galileo and the Air Force’s flying ionospheric laboratory (KC135) to record two substorms. In 1982, the Explorer satellite imaged an auroral substorm high above the north polar region (Figure 5B); its images resembled the schematic illustrations made by the author, becoming another convincing fact.
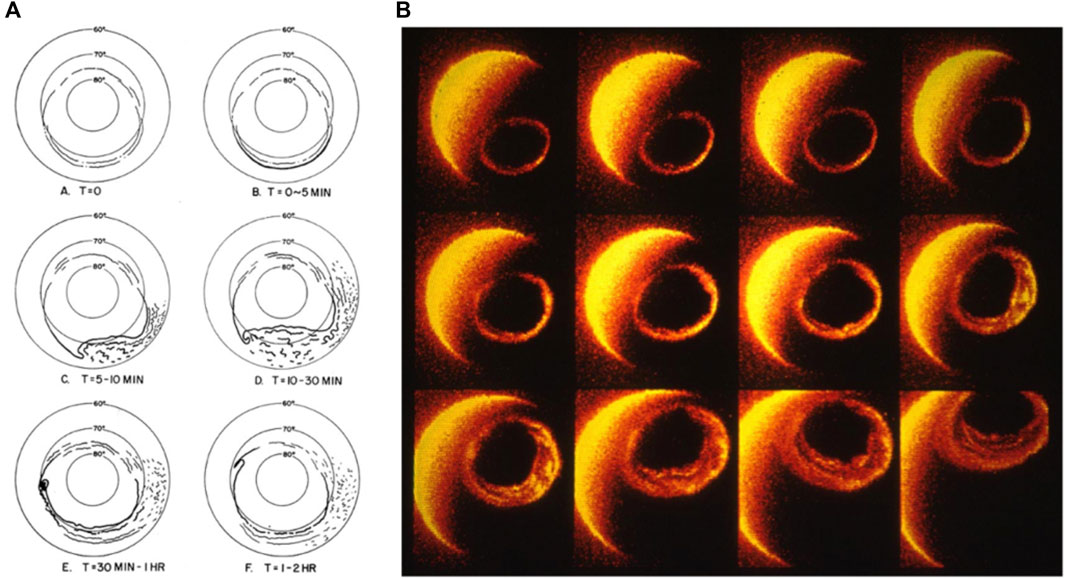
FIGURE 5. (A) The schematic illustration of the development of auroral substorms (Akasofu, 1964). The first indication of substorm onset is a sudden brightening of an auroal arc (T = 0), followed by a rapid poleward advance; this movement causes westward traveling surge (WTS), which propagates toward the evening sky; In the morning sky, the auroral arcs disintegrate into “patches” and drift eastward. It takes about 2 h (B) A series of images of an auroral substorm obtained by the Explorer satellite in 1982 (Courtesy of L. Frank).
It was fortunate that many magnetospheric phenomena observed by satellites occur also simultaneously with auroral substorms, so that the field of magnetospheric substorms had also been established soon afterward by the whole community.
On the other hand, it should be mentioned that some of my colleagues pointed out that the concept of auroral substorms has unfortunately become a “well-established concept”. It is my sincere hope that a better concept will emerge soon in order to make a new progress in auroral physics, since many fundamental issues of the aurora have not been explored yet. Even the cause of a thin curtain-like structures of the aurora, one of the basic features of the aurora, is not well understood.
When a Single Approach or theory Prevails, but I Have a Different Idea
When I met Hannes Alfven in 1966 for the first time at a conference held in Norway, he almost insisted to me that in studying space physics, I should concentrate on the electric currents, instead of magnetic field lines. This method is called the electric current approach by him.
Since he was the originator of the MHD (magnetohydrodynamic) theory (Alfven, 1950), this was a great surprise to me; I had just begun to learn MHD at that time; in the theory, a magnetic field line can be identified by plasma particles attached to it, namely the “frozen-in field” concept. This approach is called the magnetic field line approach.
By then (1966), Alfven must have already realized the limitation of the concept of “frozen-in field” in rarefied plasma in solar and magnetospheric phenomena. On the other hand, it seems that the magnetic field line approach has been the main or only one approach in space physics for a long time.
I recall that Alfven was unhappy by the fact that people did not listen to his advice, although he repeated his warning whenever possible (cf. Alfven, 1981; Alfven, 1986; even in his Nobel Prize acceptance speech). Thus, by taking this opportunity, I would like to remind the reader of his warning.
Both solar flares and auroral substorms are electromagnetic phenomena in rarefied plasma. By taking the electric current approach, it is necessary to consider, first of all, a dynamo as the power supply, and secondly transmission of the power to the location where the power is finally dissipated (observed phenomena are mostly dissipation results) in understanding the whole processes of a phenomenon. I believe that this is the standard approach to study electromagnetic phenomena in nature. Even if one wants to devote on one part of the series of this electromagnetic process, it is important to keep in mind the whole current system (Akasofu, 2020b).
Data Availability Statement
The original contributions presented in the study are included in the article/Supplementary Material, further inquiries can be directed to the corresponding author.
Author Contributions
The author confirms being the sole contributor of this work and has approved it for publication.
Conflict of Interest
The author declares that the research was conducted in the absence of any commercial or financial relationships that could be construed as a potential conflict of interest.
Publisher’s Note
All claims expressed in this article are solely those of the authors and do not necessarily represent those of their affiliated organizations, or those of the publisher, the editors and the reviewers. Any product that may be evaluated in this article, or claim that may be made by its manufacturer, is not guaranteed or endorsed by the publisher.
Acknowledgments
Looking back my research carrier, first of all, I owe so much to both Sydney Chapman and Hannes Alfven in building up my independent mind. I owe greatly so many of my colleagues for their discussions, regardless they agreed or disagreed. I am very happy that I am still corresponding with Tony Lui at Applied Physics Lab of the Johns Hopkins University, Bruce Tsurutani at the JPL (Jet Propulsion’ Laboratory) and Lou Lee at Academia Sinica in Taiwan after three or four decades.
References
Akasofu, S.-I. (1964). The Development of the Auroral Substorm. Planet. Space Sci. 12, 273–282. doi:10.1016/0032-0633(64)90151-5
Akasofu, S. I. (2020a). A Morphological Study of Unipolar Magnetic fields and the Relationship with Sunspots. J. Atmos. Solar-Terr. Phys. 218 (2021), 105625. doi:10.1016/j.jastp.2021.105625
Akasofu, S. I. (2020b). Electric Current Approach Studying Both Auroral Substorms and Solar Flares Together. Frontiers Astronom Space Sci. 7, 2–20. doi:10.3389./fspas.202000004
Akasofu, S. I., and Chapman, S. (1963). The Development of the Main Phase of Magnetic Storms. J. Geophys. Res. 68, 125–129. doi:10.1029/jz068i001p00125
Alfvén, H. (1981). “Cosmic Plasma,” in Astrophysics and Space Science Library (Berlin/Heidelberg, Germany: Springer-Verlag). 90-277-1151-8.
Alfven, H. (1986). Double Layers and Circuits in Astrophysics. IEEE Trans. Plasma Sci. 14 (6), 779–793. doi:10.1109/tps.1986.4316626
Babcock, H. W. (1961). The Topology of the Sun's Magnetic Field and the 22-YEAR Cycle. ApJ 133, 572–587. doi:10.1086/147060
Feldstein, Y. (1963). Some Problems Concerning the Morphology of Auroras and Magnetic Disturbances at High Latitudes. Geomagnetism and Aeronomy 3, 183–192.
Lee, L. C., and Akasofu, S. I. (2021). On the Causes of the Solar Wind: Part 1. Unipolar Solar Induction Currents. J. Geophys. Space Phys. 126, 1–8. doi:10.1029/2021ja029358
Keywords: geomagnetic storm, solar wind, sunspot, aurora, solar flare
Citation: Akasofu S-I (2022) When I Encountered Difficult Problems. Front. Astron. Space Sci. 9:879290. doi: 10.3389/fspas.2022.879290
Received: 19 February 2022; Accepted: 15 March 2022;
Published: 19 April 2022.
Edited by:
Noora Partamies, The University Centre in Svalbard, NorwayReviewed by:
Maxime Grandin, University of Helsinki, FinlandCopyright © 2022 Akasofu. This is an open-access article distributed under the terms of the Creative Commons Attribution License (CC BY). The use, distribution or reproduction in other forums is permitted, provided the original author(s) and the copyright owner(s) are credited and that the original publication in this journal is cited, in accordance with accepted academic practice. No use, distribution or reproduction is permitted which does not comply with these terms.
*Correspondence: Syun-Ichi Akasofu, c2FrYXNvZnVAYWxhc2thLmVkdQ==