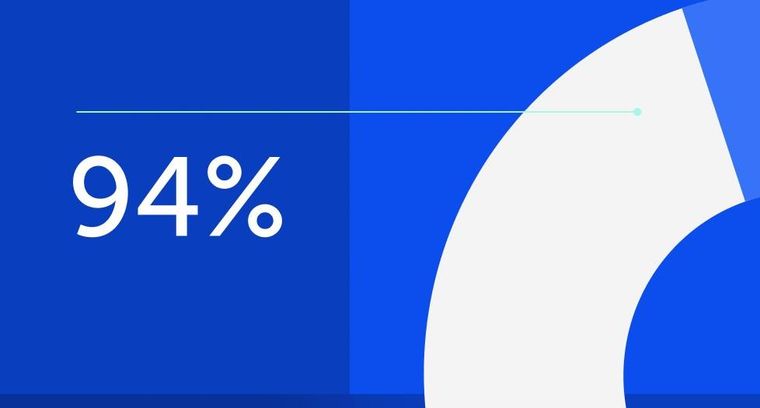
94% of researchers rate our articles as excellent or good
Learn more about the work of our research integrity team to safeguard the quality of each article we publish.
Find out more
ORIGINAL RESEARCH article
Front. Astron. Space Sci., 04 April 2022
Sec. Space Physics
Volume 9 - 2022 | https://doi.org/10.3389/fspas.2022.858300
Small-scale magnetic holes are common magnetic structures with a spatial scale always smaller than one thermal proton gyroradius (
Unlike Earth, Mars is an unmagnetized planet without a global intrinsic magnetic field (Acuña et al., 1998). Therefore, the shock supermagnetosonic solar wind can directly interact with the Martian ionosphere and then both a bow shock and an induced magnetosphere boundary are formed as two important boundaries which can divide the Martian space environment into three parts: the upstream solar wind, the magnetosheath, and the induced magnetosphere (Zhang et al., 1991; Bertucci et al., 2004). The Martian magnetosheath is full of magnetized plasma and strong magnetic fluctuations (Ruhunusiri et al., 2017). Magnetic fluctuations and turbulence can play a key role in the particle acceleration and heating of space plasma (Zimbardo et al., 2010; Bruno and Carbone, 2013), which can deeply affect the interaction between the solar wind and Mars.
The spectral indices are widely used to analyze the properties of magnetic fluctuations and turbulence in the various space plasma environments, such as the solar wind (Alexandrova et al., 2008; Kiyani et al., 2009) and the magnetosphere of Mercury (Uritsky et al., 2011), Venus (Vörös et al., 2008; Xiao et al., 2018), Earth (Vörös et al., 2004; Sahraoui et al., 2006), and Mars (Ruhunusiri et al., 2017). These fluctuations have power–law relationships as
Recently, a sub-proton scale coherent magnetic structure, called small-scale magnetic holes (MHs), has been widely reported in the solar wind and plasma sheet (Ge et al., 2011; Sun et al., 2012; Sundberg et al., 2015; Gershman et al., 2016; Wang et al., 2020a). Meanwhile, these structures are reported in highly turbulent environments like the terrestrial magnetosheath (Huang et al., 2017a; Huang et al., 2017b; Yao et al., 2017; Yao et al., 2020), Venusian magnetosheath (Goodrich et al., 2021), and Martian magnetosheath (Wu et al., 2021). The small-scale MH manifests itself as a depletion in magnetic field strength with a spatial scale less than one thermal proton gyroradius (
Based on the measurements from the Mars Atmosphere and Volatile Evolution (MAVEN) mission, we investigate the kinetic scale magnetic fluctuations in the Martian magnetosheath and focus on the events containing the small-scale magnetic holes. We analyze the effect of the small-scale magnetic holes on the kinetic scale magnetic fluctuations in this study.
MAVEN (Jakosky et al., 2015) was launched in November 2013 and was designed to explore the Martian upper atmosphere, ionosphere, and interactions between Mars and the solar wind. MAVEN has a tilted elliptical orbit with a perigee of 150 km and an apogee of 6,220 km. The orbital period of MAVEN around Mars is about 4.5 h. MAVEN spends much time in the Martian magnetosheath and collects a vast amount of data to study small-scale MHs in the Martian magnetosheath. In this study, we use 32-Hz magnetic field data from the magnetometer (Connerney et al., 2015). The Mars Solar Orbital (MSO) coordinate system is used unless otherwise specified. The MSO is a right-handed system whose
We identified small-scale MHs by a similar criterion used in Wu et al. (2021). The magnetic field amplitude depression
For the analysis of the magnetic fluctuation and turbulence associated with small-scale MHs in the Martian magnetosheath, the power spectral densities (PSDs) of magnetic fluctuations are first calculated with a continuous magnetic field data series, and then the values of spectral indices in the frequency between 1 and 16 Hz are determined by linear fitting. In the Martian magnetosheath, the median local proton gyrofrequency is lower than 0.6 Hz (Ruhunusiri et al., 2017). So, the frequency range of 1–16 Hz is always above the proton gyrofrequency, which indicates that it is a kinetic frequency range.
Among the 137 small-scale MH events, we first show an example observed in the Martian magnetosheath by MAVEN on 05 February 2016. Figure 1(A1-A2) shows the measured magnitude and three components of the magnetic field vector. The observed MH is located at 16:37:48.600 UT, which has an obvious dip in magnetic field magnitude. The two boundaries of this MH, which are determined by the nearest points with a magnetic field magnitude greater than
FIGURE 1. Small-scale magnetic hole observed in the Martian magnetosheath by MAVEN on 05 February 2016. (A1) Magnetic field magnitude. (A2) Three components of the magnetic field (red, cyan, and blue are the
The presence of small-scale MHs can influence the spectral index because they have high-frequency spectral components. We try to check whether this transient structure can influence turbulence cascade and play an important role in the dissipation of magnetic energy. The wavelet analysis of the magnetic field fluctuation is employed to confirm the effect of this MH. The results of the wavelet analysis are shown in Figure 2A. The color contour shows the wavelet spectrograph of the magnetic field data, and the red line is the time series of the magnetic field magnitude. It can be found that the magnetic field fluctuation with frequencies ranging from 1 to 4 Hz has an obvious enhancement inside this MH, while there is no such enhancement outside this transient structure. If we just exclude this magnetic structure, the spectral index has a value of 2.0 during the 1-min interval before the MH, and 2.1 during the 1-min interval after the MH, which are shown in Figure 2(B1) and (B2), respectively. These values are almost the same as those in Figure 1(C1) and (C3). Thus, the small-scale MHs seem to not affect the properties of the ambient magnetic fluctuation at least in this event, but the MHs can indeed influence the spectral index by their high-frequency spectral components.
FIGURE 2. (A)Wavelet spectrograph of the magnetic field data, and the red curve is the time series of the magnetic field magnitude; (B1) the PSDs computed by the 1-min window marked by the left blue bar in (A); (B2) the PSDs computed by the 1-min window marked by the right blue line in (A).
To obtain the statistical features of spectral indices in the kinetic range 1–16 Hz of magnetic fluctuations in the Martian magnetosheath, a 3-min window used in our example event is employed in this study. The orbit coverage of the Martian magnetosheath during February 2016 is shown in Figure 3. Cells in a cylindrical coordinate system with a size
FIGURE 3. Distribution of the orbit coverage shown in a cylindrical coordinate system. The bin size is 0.1
The spectral indices in the kinetic range 1–16 Hz for each event are calculated with this 3-min magnetic field data series. To determine whether small-scale MHs influence the spectral index value around the magnetosheath environment, we calculated power spectra at the 1–16 Hz frequency range in three 1-min windows like the example shown in Figure 1: a time interval containing the MH, a time interval before the one containing the MH, and a time interval after the one containing the MH. Then, the spectral index values
FIGURE 4. Spectral index value distributions: (A) the median value of the spectral indices computed by 1-min data before each small-scale magnetic hole; (B) the median value of the spectral indices computed by 1-min date containing a small-scale magnetic hole; (C) the median value of the spectral indices computed by 1-min date after each small-scale magnetic hole.
Figures 4A,C give the magnetic fluctuations without small-scale MHs. The distributions of spectral indices at the dayside and the nightside of the Marian magnetosheath are different. At the nightside, the occurrence rate (number of events per hour) is mainly in the range from 2.3 to 2.6, while at the dayside, the occurrence rate mainly ranges from 1.7 to 3.2. Ruhunusiri et al. (2017) have found that the spectral index value can vary from 1.9 to 3.2 for the kinetic range in the Martian magnetosheath. Our results are consistent with theirs. In addition, as shown in Figures 4A,C, it can be found that the spectral index near bow shock is smaller than that near the IMB at the nightside. The value of spectral indices in the kinetic range gradually decreases from the region near the bow shock to the region near the IMB. Since there are not enough data at the dayside, this trend is not obvious. In the future, we would like to use more events to analyze the features at the dayside.
For each MH event, we have computed
FIGURE 5. (A) Histograms of the difference of the spectral index value between the time interval containing the MH and the time interval before the one containing the MH; (B) histograms of the difference of the spectral index value between the time interval containing the MH and the time interval after the one containing the MH.
TABLE 1. Minimum, maximum, median, and mean values and the standard deviation of
The Martian magnetosheath is a turbulent plasma environment (Mazelle et al., 2004; Ruhunusiri et al., 2017). Ruhunusiri et al. (2017) have first investigated the global features of magnetic fluctuations in the Martian space environment and found that magnetic fluctuations in the Martian magnetosheath are dominated by some local structures and processes. In our study, we have studied magnetic fluctuations associated with small-scale MHs in the Martian magnetosheath and aimed to find the effect of these MHs on the spectral indices in the kinetic frequency range 1–16 Hz. The spectral index value is mainly from 2.3 to 2.6 at the nightside of the Martian magnetosheath. At the dayside of the Martian magnetosheath, the spectral indices are around 2.8 and 1.8 for most events. Ruhunusiri et al. (2017) have found that for the kinetic range, the spectral indices of sheath fluctuations are around 2.9 in the central part of the Martian magnetosheath, while the indices have a flatter distribution from 2.0 to 2.9 near the induced magnetosphere boundary. In our study, the value of the spectral index is in a similar range.
In our study, the value of spectral indices has a decreasing tendency from the region near the bow shock to the region near the IMB at the nightside. However, the spectral index values at the dayside have no obvious trend. In Ruhunusiri et al. (2017), the plasma processes near the IMB have an obvious effect on the value of spectral indices. It can be found that the size of the magnetosheath at the dayside is very great. So, magnetic fluctuations at the dayside can be more strongly affected by the plasma processes than those at the IMB. It may lead to the difference in the index value at the dayside and nightside. Additionally, the interplanetary magnetic field mainly is along
Small-scale MHs are typical sub-proton-scale magnetic structures that are always detected in the turbulent Martian, terrestrial, and Venusian magnetosheaths (e.g., Huang et al., 2017a; Yao et al., 2017; Goodrich et al., 2021; Wu et al., 2021). The PIC simulation results have shown that small-scale MHs can arise during the turbulent evolution of the plasma (Haynes et al., 2015; Roytershteyn et al., 2015). Whether small-scale MHs can contribute to the properties of magnetic fluctuations is still an open question. Based on the statistical analysis of 137 small-scale MHs, we have calculated the spectral indices of magnetic fluctuations in the kinetic range (1–16 Hz) for three time intervals associated with an identified MH: a time interval containing the MH, a time interval before the one containing the MH, and a time interval after the one containing the MH. Our results have shown that small-scale MHs have high-frequency spectral components and thus can influence the spectral index in the high-frequency range. For most events, the small-scale MHs lead to a variation in the spectral index. In more than 20% of the events, such a variation can exceed 0.3.
Small-scale MHs could affect the spectral index value in the kinetic range by the following mechanism. The small-scale MH is an isolated magnetic dip always with a duration from 0.1 to 1 s (Wu et al., 2021). It can lead an extra power enhancement around several hertz. When we calculated the spectral index in the range [1, 16] Hz, the extra power enhancement can lead to the change of the spectral index value. For example, as shown in Figure 1(C2), the MH is an isolated peak with a duration of 0.34 s, which can lead to an extra power enhancement around 3 Hz. The enhancement of power spectral density at the low-frequency part and the unchanged power spectral density at the high-frequency part would lead to an increase in the spectral index value. As a quasi-stable structure, the small-scale MH only enhances the power spectral density at a specific frequency, instead of changing the broadband spectrum. So during the calculation of the spectral index in the kinetic range, these MHs seem to cause some calculation errors. Therefore, during the analysis of the cascade of magnetic turbulence, using the spectral index alone as a diagnostic may not be convincing, especially in the presence of some transient structures.
Until now, it is unclear how small-scale MHs affect their plasma environment. Recently, Franco et al. (2019) have estimated correlation lengths of ultralow-frequency (ULF) waves around Mars and pointed out that wave fluctuations at the Martian magnetosheath may be correlated with the upper ionosphere. Their work renders a new way to understand the microphysical processes that occur within the Martian environment. We will work on the topic of the importance of small-scale MHs to Martian microphysics in the future.
In this study, we have analyzed magnetic fluctuations in the Martian magnetosheath using the MAVEN magnetic field data in February 2016. We have calculated the spectral indices in kinetic regimes for the 1-min window before and after the small-scale MHs and the 1-min window containing the small-scale MH. By the comparisons of the three intervals, we try to investigate whether small-scale MHs can influence the features of magnetic fluctuations and turbulence in the Martian magnetosheath. The main results are as follows:
1) The small-scale MHs can influence the spectral indices in the kinetic range of magnetic fluctuations by their high-frequency spectral components.
2) The small-scale MH lead to a variation in the spectral index in the kinetic range for most events.
3) For more than 20% of events, the value of the spectral index in the kinetic range can change by 0.3 due to the effect of small-scale MHs.
Our results have already confirmed that small-scale MHs can lead to a change in the spectral index in this kinetic range by the enhancement of the power spectral density at the frequency range [1, 16] Hz. Therefore, using the spectral index alone as a diagnostic sometimes is not enough convincing during the analysis of turbulence in planetary environments.
Publicly available datasets were analyzed in this study. These data can be found here: https://pds-ppi.igpp.ucla.edu/mission/MAVEN.
YC analyzed the MAVEN data and drew all the figures. MW conceptualized the study and produced the published results based on MAVEN physical data products. This study was on the leading of TZ. AD, MW, and TZ contributed substantially to the scientific interpretation of the results. All authors discussed the results and conclusions of the manuscript.
The work in China was supported by NSFC (grants 41774171, 41974205, 41774167, 41804157, and 41904156), and the pre-research project on Civil Aerospace Technologies (No. D020103) was funded by CNSA. The authors also acknowledge the Macau Foundation. MW was supported by a grant from the Key Laboratory of Geospace Environment, CAS. TZ was supported by the CAS Center for Excellence in Comparative Planetology. The MAVEN data are publicly available through the Planetary Plasma Interactions Node of the Planetary Data System (https://pds-ppi.igpp.ucla.edu/mission/MAVEN).
The authors declare that the research was conducted in the absence of any commercial or financial relationships that could be construed as a potential conflict of interest.
All claims expressed in this article are solely those of the authors and do not necessarily represent those of their affiliated organizations, or those of the publisher, the editors, and the reviewers. Any product that may be evaluated in this article, or claim that may be made by its manufacturer, is not guaranteed or endorsed by the publisher.
We thank the entire MAVEN team and instrument leaders for high-quality data and convenient access.
Acuña, M. H., Connerney, J. E. P., Wasilewski, P., Lin, R. P., Anderson, K. A., Carlson, C. W., et al. (1998). Magnetic Field and Plasma Observations at Mars: Initial Results of the Mars Global Surveyor mission. Science 279 (5357), 1676–1680. doi:10.1126/science.279.5357.1676
Alexandrova, O., Carbone, V., Veltri, P., and Sorriso‐Valvo, L. (2008). Small‐Scale Energy Cascade of the Solar Wind Turbulence. ApJ 674 (2), 1153–1157. doi:10.1086/524056
Alexandrova, O. (2008). Solar Wind vs Magnetosheath Turbulence and Alfvén Vortices. Nonlin. Process. Geophys. 15, 95–108. doi:10.5194/npg-15-95-2008
Balikhin, M. A., Sibeck, D. G., Runov, A., and Walker, S. N. (2012). Magnetic Holes in the Vicinity of Dipolarization Fronts: Mirror or Tearing Structures? J. Geophys. Res. 117 (8), 1–14. doi:10.1029/2012JA017552
Bertucci, C., Mazelle, C., Crider, D. H., Mitchell, D. L., Sauer, K., Acuña, M. H., et al. (2004). MGS MAG/ER Observations at the Magnetic Pileup Boundary of Mars: Draping Enhancement and Low Frequency Waves. Adv. Space Res. 33 (11), 1938–1944. doi:10.1016/j.asr.2003.04.054
Bowen, T. A., Bale, S. D., Bandyopadhyay, R., Bonnell, J. W., Case, A., Chasapis, A., et al. (2021). Kinetic‐Scale Turbulence in the Venusian Magnetosheath. Geophys. Res. Lett. 48, e2020GL090783. doi:10.1029/2020GL090783
Bruno, R., and Carbone, V. (2013). The Solar Wind as a Turbulence Laboratory. Living Rev. Solar Phys. 10, 2. doi:10.12942/lrsp-2013-2
Chen, C. H. K., Klein, K. G., and Howes, G. G. (2019). Evidence for Electron Landau Damping in Space Plasma Turbulence. Nat. Commun. 10, 1–8. doi:10.1038/s41467-019-08435-3
Connerney, J. E. P., Espley, J., Lawton, P., Murphy, S., Odom, J., Oliversen, R., et al. (2015). The MAVEN Magnetic Field Investigation. Space Sci. Rev. 195 (1–4), 257–291. doi:10.1007/s11214-015-0169-4
Franco, A. M. S., Fränz, M., Echer, E., and Bolzan, M. J. A. (2019). Correlation Length Around Mars: A Statistical Study with MEX and MAVEN Observations. Earth Planet. Phys. 3 (6), 560–569. doi:10.26464/epp20190510.26464/epp2019051
Ge, Y. S., McFadden, J. P., Raeder, J., Angelopoulos, V., Larson, D., and Constantinescu, O. D. (2011). Case Studies of Mirror-Mode Structures Observed by THEMIS in the Near-Earth Tail during Substorms. J. Geophys. Res. 116 (1), 1–12. doi:10.1029/2010JA015546
Gershman, D. J., Dorelli, J. C., Viñas, A. F., Avanov, L. A., Gliese, U., Barrie, A. C., et al. (2016). Electron Dynamics in a Subproton-Gyroscale Magnetic Hole. Geophys. Res. Lett. 43 (9), 4112–4118. doi:10.1002/2016GL068545
Goldstein, M. L., Roberts, D. A., and Matthaeus, W. H. (1995). Magnetohydrodynamic Turbulence in the Solar Wind. Annu. Rev. Astron. Astrophys. 33, 283–325. doi:10.1146/annurev.aa.33.090195.001435
Goodrich, K. A., Bonnell, J. W., Curry, S., Livi, R., Whittlesey, P., Mozer, F., et al. (2021). Evidence of Subproton‐Scale Magnetic Holes in the Venusian Magnetosheath. Geophys. Res. Lett. 48 (5), 1–9. doi:10.1029/2020GL090329
Haynes, C. T., Burgess, D., Camporeale, E., and Sundberg, T. (2015). Electron Vortex Magnetic Holes: A Nonlinear Coherent Plasma Structure. Phys. Plasmas 22 (1), 012309. doi:10.1063/1.4906356
Hollweg, J. V., and Markovskii, S. A. (2002). Cyclotron Resonances of Ions with Obliquely Propagating Waves in Coronal Holes and the Fast Solar Wind. J. Geophys. Res. 107, 1–7. doi:10.1029/2001JA000205
Huang, S. Y., Du, J. W., Sahraoui, F., Yuan, Z. G., He, J. S., Zhao, J. S., et al. (2017a). A Statistical Study of Kinetic-Size Magnetic Holes in Turbulent Magnetosheath: MMS Observations. J. Geophys. Res. Space Phys. 122 (8), 8577–8588. doi:10.1002/2017JA024415
Huang, S. Y., Sahraoui, F., Yuan, Z. G., He, J. S., Zhao, J. S., Contel, O. L., et al. (2017b). Magnetospheric Multiscale Observations of Electron Vortex Magnetic Hole in the Turbulent Magnetosheath Plasma. ApJ 836 (2), L27. doi:10.3847/2041-8213/aa5f50
Jakosky, B. M., Lin, R. P., Grebowsky, J. M., Luhmann, J. G., Mitchell, D. F., Beutelschies, G., et al. (2015). The Mars Atmosphere and Volatile Evolution (MAVEN) Mission. Space Sci. Rev. 195, 3–48. doi:10.1007/s11214-015-0139-x
Kiyani, K. H., Chapman, S. C., Khotyaintsev, Y. V., Dunlop, M. W., and Sahraoui, F. (2009). Global Scale-Invariant Dissipation in Collisionless Plasma Turbulence. Phys. Rev. Lett. 103 (7), 2007–2010. doi:10.1103/PhysRevLett.103.075006
Mazelle, C., Winterhalter, D., Sauer, K., Trotignon, J. G., Acuña, M. H., Baumgärtel, K., et al. (2004). Bow Shock and Upstream Phenomena at Mars. Space Sci. Rev. 111 (1–2), 115–181. doi:10.1007/978-0-306-48604-3_3
Roberts, O. W., Li, X., and Jeska, L. (2015). A Statistical Study of the Solar Wind Turbulence at Ion Kinetic Scales Using Thek-Filtering Technique and Cluster Data. ApJ 802, 2. doi:10.1088/0004-637X/802/1/2
Roytershteyn, V., Karimabadi, H., and Roberts, A. (2015). Generation of Magnetic Holes in Fully Kinetic Simulations of Collisionless Turbulence. Phil. Trans. R. Soc. A. 373, 20140151. doi:10.1098/rsta.2014.0151
Ruhunusiri, S., Halekas, J. S., Espley, J. R., Mazelle, C., Brain, D., Harada, Y., et al. (2017). Characterization of Turbulence in the Mars Plasma Environment with MAVEN Observations. J. Geophys. Res. Space Phys. 122 (1), 656–674. doi:10.1002/2016JA023456
Sahraoui, F., Belmont, G., Rezeau, L., Cornilleau-Wehrlin, N., Pinçon, J. L., and Balogh, A. (2006). Anisotropic Turbulent Spectra in the Terrestrial Magnetosheath as Seen by the Cluster Spacecraft. Phys. Rev. Lett. 96 (7), 075002. doi:10.1103/PhysRevLett.96.075002
Sun, W. J., Shi, Q. Q., Fu, S. Y., Pu, Z. Y., Dunlop, M. W., Walsh, A. P., et al. (2012). Cluster and TC-1 Observation of Magnetic Holes in the Plasma Sheet. Ann. Geophys. 30 (3), 583–595. doi:10.5194/angeo-30-583-2012
Sundberg, T., Burgess, D., and Haynes, C. T. (2015). Properties and Origin of Subproton-Scale Magnetic Holes in the Terrestrial Plasma Sheet. J. Geophys. Res. Space Phys. 120, 2600–2615. doi:10.1002/2014JA020856
Tsurutani, B. T., Lakhina, G. S., Verkhoglyadova, O. P., Echer, E., and Guarnieri, F. L. (2010). Magnetic Decreases (MDs) and Mirror Modes: Two Different Plasma β Changing Mechanisms. Nonlin. Process. Geophys. 17, 467–479. doi:10.5194/npg-17-467-2010
Tsurutani, B. T., Lakhina, G. S., Verkhoglyadova, O. P., Echer, E., Guarnieri, F. L., Narita, Y., et al. (2011). Magnetosheath and Heliosheath Mirror Mode Structures, Interplanetary Magnetic Decreases, and Linear Magnetic Decreases: Differences and Distinguishing Features. J. Geophys. Res. 116, A02103. doi:10.1029/2010JA015913
Uritsky, V. M., Slavin, J. A., Khazanov, G. V., Donovan, E. F., Boardsen, S. A., Anderson, B. J., et al. (2011). Kinetic-scale Magnetic Turbulence and Finite Larmor Radius Effects at Mercury. J. Geophys. Res. 116 (9), 1–14. doi:10.1029/2011JA016744
Vignes, D., Mazelle, C., Rme, H., Acuña, M. H., Connerney, J. E. P., Lin, R. P., et al. (2000). The Solar Wind Interaction with mars: Locations and Shapes of the bow Shock and the Magnetic Pile-Up Boundary from the Observations of the MAG/ER experiment Onboard Mars Global Surveyor. Geophys. Res. Lett. 27 (1), 49–52. doi:10.1029/1999GL010703
Vörös, Z., Baumjohann, W., Nakamura, R., Volwerk, M., Runov, A., Zhang, T. L., et al. (2004). Magnetic Turbulence in the Plasma Sheet. J. Geophys. Res. Space Phy. 109 (A11), 1–16. doi:10.1029/2004JA010404
Vörös, Z., Yordanova, E., Khotyaintsev, Y. V., Varsani, A., and Narita, Y. (2019). Energy Conversion at Kinetic Scales in the Turbulent Magnetosheath. Front. Astron. Space Sci. 6, 60. doi:10.3389/fspas.2019
Vörös, Z., Zhang, T. L., Leubner, M. P., Volwerk, M., Delva, M., Baumjohann, W., et al. (2008). Magnetic Fluctuations and Turbulence in the Venus Magnetosheath and Wake. Geophys. Res. Lett. 35 (11), 1–5. doi:10.1029/2008GL033879
Wang, G. Q., Volwerk, M., Xiao, S. D., Wu, M. Y., Hao, Y. F., Liu, L. J., et al. (2020a). Three-dimensional Geometry of the Electron-Scale Magnetic Hole in the Solar Wind. ApJL 904 (1), L11. doi:10.3847/2041-8213/abc553
Wang, G. Q., Zhang, T. L., Xiao, S. D., Wu, M. Y., Wang, G., Liu, L. J., et al. (2020b). Statistical Properties of Sub‐Ion Magnetic Holes in the Solar Wind at 1 AU. J. Geophys. Res. Space Phys. 125 (10), A028320. doi:10.1029/2020JA028320
Wu, M., Chen, Y., Du, A., Wang, G., Xiao, S., Peng, E., et al. (2021). Statistical Properties of Small-Scale Linear Magnetic Holes in the Martian Magnetosheath. ApJ 916 (2), 104. doi:10.3847/1538-4357/ac090b
Xiao, S. D., Wu, M. Y., Wang, G. Q., Chen, Y. Q., and Zhang, T. L. (2021). The Spectral Scalings of Magnetic Fluctuations Upstream and Downstream of the Venusian bow Shock. Earth Planets Space 73 (1), 13. doi:10.1186/s40623-020-01343-7
Xiao, S. D., Zhang, T. L., and Vörös, Z. (2018). Magnetic Fluctuations and Turbulence in the Venusian Magnetosheath Downstream of Different Types of bow Shock. J. Geophys. Res. Space Phys. 123 (10), 8219–8226. doi:10.1029/2018JA025250
Yao, S. T., Hamrin, M., Shi, Q. Q., Yao, Z. H., Degeling, A. W., Zong, Q. G., et al. (2020). Propagating and Dynamic Properties of Magnetic Dips in the Dayside Magnetosheath: MMS Observations. J. Geophys. Res. Space Phys. 125 (6), 1–16. doi:10.1029/2019JA026736
Yao, S. T., Wang, X. G., Shi, Q. Q., Pitkänen, T., Hamrin, M., Yao, Z. H., et al. (2017). Observations of Kinetic‐size Magnetic Holes in the Magnetosheath. J. Geophys. Res. Space Phys. 122 (2), 1990–2000. doi:10.1002/2016JA023858
Zhang, T. L., Baumjohann, W., Russell, C. T., Jian, L. K., Wang, C., Cao, J. B., et al. (2009). Mirror Mode Structures in the Solar Wind at 0.72 AU. J. Geophys. Res. 114 (10), 1–5. doi:10.1029/2009JA014103
Zhang, T. L., Russell, C. T., Baumjohann, W., Jian, L. K., Balikhin, M. A., Cao, J. B., et al. (2008). Characteristic Size and Shape of the Mirror Mode Structures in the Solar Wind at 0.72 AU. Geophys. Res. Lett. 35 (10), 2–5. doi:10.1029/2008GL033793
Zhang, T. L., Schwingenschuh, K., Lichtenegger, H., Riedler, W., Russell, C. T., and Luhmann, J. G. (1991). Interplanetary Magnetic Field Control of the Mars bow Shock: Evidence for Venuslike Interaction. J. Geophys. Res. 96 (A7), 11265–11269. doi:10.1029/91JA01099
Keywords: small-scale magnetic holes, Mars, magnetic fluctuations, spectral indices, magnetosheath
Citation: Chen Y, Wu M, Xiao S, Du A, Wang G, Chen Y, Pan Z and Zhang T (2022) Magnetic Fluctuations Associated With Small-Scale Magnetic Holes in the Martian Magnetosheath. Front. Astron. Space Sci. 9:858300. doi: 10.3389/fspas.2022.858300
Received: 19 January 2022; Accepted: 07 March 2022;
Published: 04 April 2022.
Edited by:
Olga V. Khabarova, Institute of Terrestrial Magnetism ionosphere and Radio Wave Propagation (RAS), RussiaReviewed by:
Ezequiel Echer, National Institute of Space Research (INPE), BrazilCopyright © 2022 Chen, Wu, Xiao, Du, Wang, Chen, Pan and Zhang. This is an open-access article distributed under the terms of the Creative Commons Attribution License (CC BY). The use, distribution or reproduction in other forums is permitted, provided the original author(s) and the copyright owner(s) are credited and that the original publication in this journal is cited, in accordance with accepted academic practice. No use, distribution or reproduction is permitted which does not comply with these terms.
*Correspondence: Mingyu Wu, d3VtaW5neXVAaGl0LmVkdS5jbg==; Tielong Zhang, dGllbG9uZy56aGFuZ0BvZWF3LmFjLmF0
Disclaimer: All claims expressed in this article are solely those of the authors and do not necessarily represent those of their affiliated organizations, or those of the publisher, the editors and the reviewers. Any product that may be evaluated in this article or claim that may be made by its manufacturer is not guaranteed or endorsed by the publisher.
Research integrity at Frontiers
Learn more about the work of our research integrity team to safeguard the quality of each article we publish.