- 1Department of Astrophysics, Centro de Astrobiología (CSIC/INTA), Torrejón de Ardoz, Spain
- 2Departamento de Química Física, Facultad de Ciencia y Tecnología, Universidad del País Vasco, (UPV-EHU), Bilbao, Spain
- 3Biofisika Institute (CSIC, UPV/EHU), Leioa, Spain
- 4SKA Observatory, Jodrell Bank, Macclesfield, United Kingdom
One of the theories for the origin of life proposes that a significant fraction of prebiotic material could have arrived to Earth from outer space between 4.1 and 3.8 billion years ago. This suggests that those prebiotic compounds could have originated in interstellar space, to be later on incorporated to small Solar-system bodies and planetesimals. The recent discovery of prebiotic molecules such as hydroxylamine and ethanolamine in the interstellar medium, strongly supports this hypothesis. However, some species such as sugars, key for the synthesis of ribonucleotides and for metabolic processes, remain to be discovered in space. The unmatched sensitivity of the Square Kilometre Array (SKA) at centimeter wavelengths will be able to detect even more complex and heavier prebiotic molecules than existing instrumentation. In this contribution, we illustrate the potential of the SKA to detect simple sugars with three and four carbon atoms, using a moderate investment of observing time.
1 Introduction
The question of the origin of life has attracted the interest of researchers for centuries. Two main lines of thought have been proposed: i) Life may have emerged endogenously so that the building blocks of life could have formed “in situ” on Earth; or ii) Life could have originated somewhere else in the Universe and been transported to Earth in small Solar-system bodies. Alternatively, an intermediate theory contemplates the possibility that a fraction of the prebiotic material essential for the origin of life could have originated exogenously and been transferred to a young Earth via planetesimal impact on its surface (Anders, 1989; Chyba and Sagan, 1992). Prebiotic molecules such as amino acids, nucleobases and sugars have indeed been detected in meteorites (Cooper et al., 2001; Pizzarello et al., 2006; Callahan et al., 2011; Furukawa et al., 2019; Glavin et al., 2020) and in comets (Altwegg et al., 2016), which supports the latter hypothesis.
In the past decade, it has become clear that the interstellar medium (ISM) is an extraordinary chemical factory. About 250 molecules, including ringed-molecules (see e.g., Cernicharo et al., 2021; McGuire et al., 2021), have so far been reported in the ISM. In addition, the pace at which new molecules are detected not only seems steady but accelerating (McGuire, 2021). In particular, the so-called complex organic molecules (or COMs)1 have attracted great interest in recent years since a subset of them could have been involved in the first biochemical reactions leading to the origin of life. This sub-set of COMs are typically called prebiotic. Some examples of prebiotic COMs include urea and hydroxylamine (Belloche et al., 2019; Jiménez-Serra et al., 2020; Rivilla et al., 2020) since they are possible precursors of ribonucleotides (see e.g., Becker et al., 2019; Menor Salvan et al., 2020); ethanolamine and n-propanol because they could have triggered the formation of phospholipids (Jiménez-Serra et al., 2022; Rivilla et al., 2021); or amino acetonitrile, vynil amine and ethyl amine since they are considered precursors of amino acids (Belloche et al., 2008; Zeng et al., 2021).
One of the most extended theories about the origin of life is the primordial RNA world. In this scenario, early forms of life relied solely on RNA to store genetic information and to catalyze chemical reactions. The basic units of RNA are ribonucleotides, which are composed of a phosphate group, a nitrogenous base, and a ribose sugar (a C5 sugar with five carbon atoms). Interestingly, only small precursors of sugars such as glycolonitrile (HOCH2CN; see Patel et al., 2015) or the simplest C2 sugar molecule, glycolaldehyde (CH2OHCHO), have been reported in the ISM (Hollis et al., 2000; Beltrán et al., 2009; Jørgensen et al., 2012; Zeng et al., 2019). Indeed, searches of C3 sugars such as glyceraldehyde (CHOCHOHCH2OH) or dihydroxyacetone (DHA, with the chemical formula CH2OHCOCH2OH), have not yielded any robust detection (Hollis et al., 2004; Widicus Weaver and Blake, 2005; Apponi et al., 2006; Jiménez-Serra et al., 2020). In contrast, larger sugar-like species such as C3 sugars and ribose have been found in meteorites (de Marcellus et al., 2015; Furukawa et al., 2019), which opens the possibility that these species form in interstellar space.
The search of sugars in the ISM has partly been hindered by the lack of spectroscopic rotational data since these species are thermolabile and hygroscopic. This has triggered the development of new sample preparation techniques as well as the use of ultrafast laser vaporization sources to avoid their decomposition (Cocinero et al., 2012; Calabrese et al., 2020). Recently, the rotational spectroscopy of C4 and C5 sugars such as erythrulose and ribose has been obtained in the laboratory (Cocinero et al., 2012; Insausti et al., 2021), which enables their search in interstellar space.
2 Searching for Sugars in Sources With Low Excitation Temperatures (Tex)
The first searches in the ISM of glyceraldehyde and dihydroxyacetone (DHA) targeted the massive star-forming region SgrB2 N-LMH. While an upper limit to the abundance of glyceraldehyde of ≤(2.4–5.7) × 10–11 was obtained by Hollis et al. (2004), Widicus Weaver and Blake (2005) reported a tentative detection of DHA toward this source with an abundance of 1.2 × 10–9. The latter detection, however, was never confirmed (Apponi et al., 2006). For low-mass star-forming regions, Jiménez-Serra et al. (2020) also reported upper limits to the abundance of both species in the range ≤(0.6–4)×10–10 toward the low-mass hot corino IRAS16293-2422 B.
Massive hot cores and low-mass hot corinos are among the most chemically rich sources in the Galaxy and, traditionally, they have been the selected targets for searches of new prebiotic COMs in the ISM (see e.g., Jørgensen et al., 2012; Belloche et al., 2014; Jørgensen et al., 2016; Belloche et al., 2019). Their disadvantage, however, lies in the fact that their observed rotational spectra present hundreds (even thousands) of different molecular rotational transitions as a result of the high excitation temperatures (of Tex = 100–300 K). These high temperatures populate a wide number of energy levels of a COM since its partition function is large. In addition, at temperatures of 100–300 K COM rotational spectra present their peak emission shifted towards millimeter and sub-millimeter wavelengths, which are heavily contaminated by the emission from smaller and lighter species such as CO. Consequently, the COM spectra observed in massive hot cores and low-mass hot corinos not only largely suffer from line blending and line confusion but also present weak lines due to the large partition functions expected at high excitation temperatures.
Alternatively, sources where COMs show low excitation temperatures (Tex) represent better targets for the search and discovery of new large prebiotic species in the ISM (Jiménez-Serra et al., 2014)2. As a result of the low Tex, the emission peak of the COM observed spectra is shifted towards lower frequencies, which are cleaner from the contribution from lighter molecules. In addition, the line intensities increase since only the lowest energy levels of the COMs can be populated at such low Tex and so, the number of rotational transitions present in the measured spectra is significantly smaller than in hotter sources. The frequency span for the transitions between the lowest energy levels is also much larger than for those between the higher energy levels, which helps reducing significantly line blending and line confusion in the observed spectra (Jiménez-Serra et al., 2014).
Giant Molecular Clouds (GMCs) located in the Galactic Center such as the molecular cloud G+0.693-0.027 (hereafter G+0.693) have proven to be not only efficient chemical factories for the formation of complex organics (Requena-Torres et al., 2006; Requena-Torres et al., 2008; Zeng et al., 2018), but also excellent targets for the discovery of new prebiotic species (see e.g., Jiménez-Serra et al., 2020; Rivilla et al., 2020; Rivilla et al., 2021; Zeng et al., 2021). These clouds show low H2 gas densities of ∼104 cm−3 and their gas and dust temperatures are decoupled (while Tdust ≤ 20K, Tgas ∼70–150 K; see Rodríguez-Fernández et al., 2000; Zeng et al., 2018). The low H2 gas densities also imply that for high-dipole moment molecules (such as COMs), and despite the high gas kinetic temperatures and broad linewidths of their emission (of ∼ 20 km s−1), their Tex is low (between 5 and 20 K) and thus their observed spectra will be less affected by line blending and line confusion. Recent searches of prebiotic molecules toward the Galactic Center molecular cloud G+0.693 have yielded the discovery of several of these species for the first time in the ISM such as hydroxylamine (NH2OH; Rivilla et al., 2020), ethanolamine (NH2CH2CH2OH; Rivilla et al., 2021), thioformic acid (HC(O)SH; Rodríguez-Almeida et al., 2021) or n-propanol (n-C3H7OH; Jiménez-Serra et al., 2022). As a result, Galactic Center GMCs are prime targets for the search and discovery of large C3 and C4 sugars in the ISM.
3 The Potential of the SKA to Detect New Prebiotic Species in the ISM: The Case of Sugars
The Square Kilometre Array (SKA) will be the largest radio telescope in the world operating at centimeter and meter wavelengths. The Phase 1 of this observatory will consist of two radio interferometers located at two different sites: SKA1 LOW in Western Australia and SKA1 MID in South Africa (Braun et al., 2019). SKA1 LOW will have 512 stations of 256 log periodic dipole antennas each operating within the frequency range between 50 and 350 MHz, while SKA1 MID will have a total of 197 antennas (that include the 64 antennas of the MeerKAT array) operating at frequencies between 350 MHz and 15 GHz (Braun et al., 2019). Given the frequency coverage and how dramatically the strength of the rotational transitions of high-dipole molecules drops for frequencies
3.1 SKA1 Simulations for C3 and C4 Sugars in Galactic Center GMCs: Glyceraldehyde, Dihydroxyacetone and Erythrulose
The emission from COMs in the Galactic Center GMCs is known to be extended (Martín-Pintado et al., 2001; Li et al., 2017; Li et al., 2020). This is particularly interesting for absorption studies in which COMs can be searched for against a bright continuum background source. This technique may allow the detection of low-abundance COMs whose emission spectra is expected to be very weak. Indeed, in the presence of a bright background continuum source, the predicted absorption line intensity (TL) is given by (see e.g., Martín et al., 2019):
where Tc is the temperature of a continuum source and Tbg is the temperature of the comic microwave background radiation (Tbg = 2.73 K). If Tc ≫Tex, this will largely enhance the observed absorption line intensity over the emission for the Tex expected to be close to Tbg.
Therefore, we simulate the case of absorption spectra of glyceraldehyde, dihydroxyacetone and erythrulose (C4H8O4) using the SLIM tool within the MADCUBA software package3 (see Martín et al., 2019). We focus our simulations on C3 and C4 sugars because, as discussed in Section 3.2, C5 sugars such as ribose are not expected to be abundant enough to be detected with SKA1 in a reasonable amount of observing time. We assume the physical conditions of the G+0.693 molecular cloud with a typical Tex of
As column densities, we have assumed that glyceraldehyde and dihydroxyacetone have column densities of 1013 cm−2 and 6.8 × 1012 cm−2, respectively. These column densities correspond to the upper limits obtained toward G+0.693 in previous spectral surveys (see Table2 in Jiménez-Serra et al., 2020). For erythrulose, we consider that this C4 sugar is a factor of 10 less abundant than the C3 sugar glyceraldehyde, with an assumed column density of 1012 cm−2 for erythrulose. We note that this decrease by one order of magnitude in the column density of molecules from the same family when one carbon atom is added to the molecular structure, has been reported for alcohols (Jiménez-Serra et al., 2022) and thiols (Rodríguez-Almeida et al., 2021). The assumed spectral resolution considered in our simulations is 79.3 kHz (equivalent to a velocity resolution between ∼1.6 and 5.0 km s−1) across the full Band 5 frequency coverage.
Figure 1 presents the predicted absorption spectra of glyceraldehyde, dihydroxyacetone and erythrulose obtained for the extended GMC G+0.693 against the background source L. Depending on the structure of the molecule, the transitions that show the larger absorption depths lie in the range between 4.8 and 7.0 GHz for glyceraldehyde and erythrulose, and 8–14 GHz for dihydroxyacetone. The maximum bandwidth that will be covered simultaneously by the Band 5 receivers of SKA1 MID in its Phase 1 is 4 GHz at frequencies between 4.6 and 8.5 GHz, and 5 GHz for frequencies between 8.3 and 15.3 GHz. Given that the deepest absorption lines appear clustered within frequency ranges of ≤ 4-5 GHz-width, future detection experiments of these lines with SKA1 MID could be carried out in just two observing runs: one to simultaneously cover all the deepest features of glyceraldehyde and erythrulose, and a second one to simultaneously cover all the transitions of dihydroxyacetone. We stress that Figure 1 includes all possible transitions of these C3 and C4 sugars available within the frequency range covered by the Band 5 receivers of SKA1 MID. The intensities of the deepest absorption features in our simulated spectra reach values of −0.47 mJy for glyceraldehyde, of −0.17 mJy for dihydroxyacetone, and −0.01 mJy for erythrulose. Table1 lists the spectroscopic information of the deepest features found in our predicted spectra for each molecule. In Section 3.2, we evaluate the observing time required to perform these detection experiments with SKA1 MID during its Phase 1 of operations, which will have only 133 antennas equipped with Band 5 receivers.
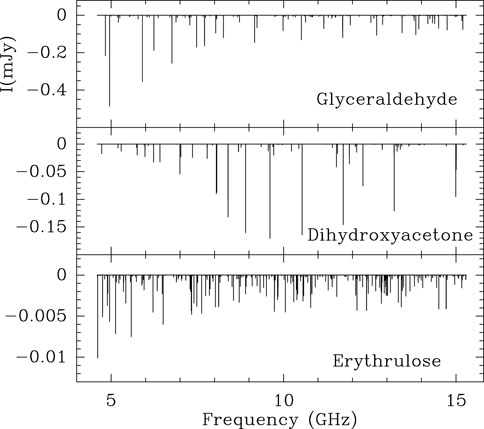
FIGURE 1. Predicted absorption spectra of the C3 and C4 sugars glyceraldehyde, dihydroxyacetone and erythrulose obtained with the SLIM tool of the MADCUBA package considering the physical conditions of the G+0.693 molecular cloud (Tex = 10K, linewidths of Δv = 20 km s−1 and extended emission across the beam) and a background source similar to the L source in the SgrB2 N molecular complex (measured flux of ∼300 mJy within a beam of 1″ at 10 GHz). The assumed column densities for glyceraldehyde and dihydroxyacetone are 1013 cm−2 and 6.8× 1012 cm−2, respectively, while for erythrulose we assume a column density one order of magnitude lower than that of glyceraldehyde (of 1012 cm−2; see Section 3.1).
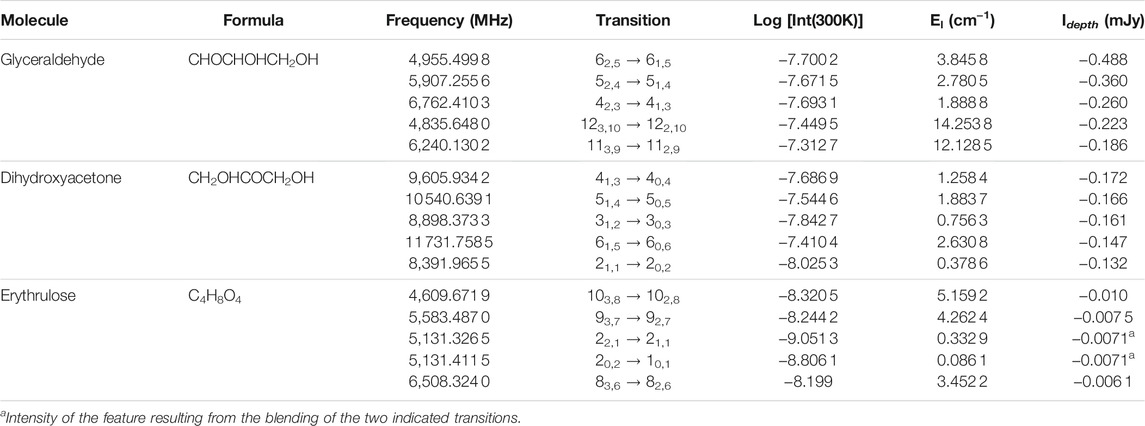
TABLE 1. List of transitions of glyceraldehyde, dihydroxyacetone and erythrulose that present the deepest features in the simulated spectra.
3.2 Time Estimates and Key Science Projects
As seen from Table1, the predicted intensities for the deepest absorption features lie in the range between −0.19 and −0.5 mJy for glyceraldehyde, −0.13 and −0.2 mJy for dihydroxyacetone, and −0.006 and −0.01 mJy for erythrulose. According to Braun et al. (2019), a line sensitivity of 90 μJy will be achieved in 1 hour of observing time with the Band 5 receivers of SKA1 MID at frequencies of 4.6–8.5 GHz, and of 85 μJy at frequencies of 8.5–15.3 GHz, for a velocity resolution of ∼ 30km s−1.
Taking these numbers into account, the deepest absorption line of glyceraldehyde at 4955 MHz could be detected with a S/N ≥ 5 in just 8.5 h assuming a velocity resolution of 3 km s−1. The weakest transitions of glyceraldehyde at 4835 MHz and at 6240 MHz, however, would require about 50 h of observing time for a similar velocity resolution and S/N. This is also the case of dihydroxyacetone, for which a total of
The time estimates to perform the detection experiments of glyceraldehyde, dihydroxyacetone and erythrulose proposed above, may seem excessive. However, we note that these long integration times are contemplated within the future Key Science Projects (KSPs) scheme that will be carried out by the SKA1. The KSPs are major surveys targeting ground-breaking transformational science in Astrophysics and Astrobiology that require considerable amount of time. Therefore, the expected integration time requests for these projects are typically of a few thousands of hours, which guarantees the detection of at least C3 sugars such as glyceraldehyde and dihydroxyacetone, if present in the ISM. As discussed in Section 4, the discovery of C4 sugars such as erythrulose may require the development of future instrumentation for SKA1 MID such as the high-frequency Band 6 receivers.
We note that, when performing deep integrations as proposed here, it is expected that the level of line confusion increases even at the low frequencies covered by the SKA1 as a consequence of weak features of low-abundance molecules becoming bright in the spectra. However, this potential problem is likely not an issue for the discovery of intermediate-sized species such as C3 and C4 sugars. As recently found by Jiménez-Serra et al. (2022) and by Rodríguez-Almeida et al. (2021), the addition of a − CH2 group to the structure of alcohols, thiols, isocyanates, and carboxylic acids implies a decrease in their abundance by at least one order of magnitude (see also Section 3.1). This decrease in the abundance would imply an increase in the required SKA1 observing time by a factor of 100 and thus, for a reasonable integration time within the context of SKA1 Key Science Projects, the only sugars for which absorption features could be detected with SKA1 are the C3 and the C4 sugars together with their lowest-energy conformers. Indeed, for the case of n-propanol, only the Ga and Aa conformers with relative energies of E = 0 K and E = 40 K, have been found toward G+0.693 (the rest of conformers with energies
4 Future Expansion of the SKA: The Band 6 Receivers
From Section 3.2, it is clear that, while relatively small molecules such as glyceraldehyde or dihydroxyacetone could be detected with a moderate investment of SKA1 MID observing time, larger prebiotic COMs such as erythrulose would still be well below the limit of what the SKA1 will be able to detect in its Phase 1. For this reason, one possible future expansion of SKA1 MID contemplates the development of higher-frequency receiver(s), the Band 6 receivers, which will increase the frequency coverage of SKA1 MID to higher frequencies from 15.3 up to 50 GHz. As reported in Memo 20-01 of SKA1 titled “SKA1 Beyond 15 GHz: The Science case for Band 6” (see Conway et al., 2021), high-dipole moment molecules such as COMs present rotational transitions at frequencies
5 Conclusion
In this contribution, we evaluate the feasibility of detecting small sugars in the ISM using the Band 5 receivers of SKA1 in its Phase 1. Our simulations show that Galactic Center Giant Molecular Clouds such as G+0.693 represent prime targets for future searches of these key prebiotic species in space. As shown in Section 3.1, the detection of small sugars could be achieved by carrying out broad spectroscopic surveys between 5 and 14 GHz in absorption against a bright continuum background source. By taking as template the L HII region source located in the SgrB2 N molecular complex, we estimate that the detection of C3 sugars could be achieved in a few hundreds of hours. Larger C4 sugars such as erythrulose would require thousands of hours of observing time. Future developments of the SKA such as the Band 6 receivers (which will increase the frequency coverage of SKA1 MID up to, at least, 24 GHz), will enable the search of these large sugars and other prebiotic COMs in just a few hundreds of hours.
Data Availability Statement
The original contributions presented in the study are included in the article/supplementary material, further inquiries can be directed to the corresponding author.
Author Contributions
IJ-S has written the first version of the manuscript. IJ-S and JM-P have produced the MADCUBA-SLIM simulations of the spectra of glyceraldehyde, dihydroxyacetone and erythrulose reported in Section 3.1. All authors have contributed to the discussion of the results and have provided comments on the text.
Funding
IJ-S and JM-P acknowledge partial support from the Spanish State Research Agency (AEI) through project numbers PID 2019-105552RB-C41 and MDM-2017-0737 Unidad de Excelencia María de Maeztu-Centro de Astrobiología (CSIC-INTA). EC thanks the support received from the MINECO (PID 2020-117892RB-I00), the Basque Government (IT1162-19 and PIBA 2018-11), the UPV/EHU (PPG17/10, GIU18/207), and CSIC (PIC 2018, LINKA20249). Computational resources and laser facilities of the UPV/EHU (SGIker) and CESGA were used in this work.
Conflict of Interest
The authors declare that the research was conducted in the absence of any commercial or financial relationships that could be construed as a potential conflict of interest.
Publisher’s Note
All claims expressed in this article are solely those of the authors and do not necessarily represent those of their affiliated organizations, or those of the publisher, the editors, and the reviewers. Any product that may be evaluated in this article, or claim that may be made by its manufacturer, is not guaranteed or endorsed by the publisher.
Acknowledgments
We would like to thank an anonymous referee for his/her positive and constructive comments on an earlier version of the manuscript.
Footnotes
1COMs are defined as carbon-based molecules with ≥6 atoms in their structure (as e.g., methanol or CH3OH; see Herbst and van Dishoeck, 2009).
2In massive hot cores and hot corinos, Tex ∼Tkin because of the high H2 gas densities (≥106 cm−3) found in these star-forming regions
3MADCUBA, or MAdrid Data CUBe Analysis, is a software developed at the Center of Astrobiology in Madrid: https://cab.inta-csic.es/madcuba/index.html
References
Altwegg, K., Balsiger, H., Bar-Nun, A., Berthelier, J.-J., Bieler, A., Bochsler, P., et al. (2016). Prebiotic Chemicals-Amino Acid and Phosphorus-In the Coma of Comet 67P/Churyumov-Gerasimenko. Sci. Adv. 2, e16002855. doi:10.1126/sciadv.1600285
Anders, E. (1989). Pre-biotic Organic Matter from Comets and Asteroids. Nature 342, 255–257. doi:10.1038/342255a0
Apponi, A. J., Halfen, D. T., Ziurys, L. M., Hollis, J. M., Remijan, A. J., and Lovas, F. J. (2006). Investigating the Limits of Chemical Complexity in Sagittarius B2(N): A Rigorous Attempt to Confirm 1,3-Dihydroxyacetone. ApJ 643, L29–L32. doi:10.1086/504979
Becker, S., Feldmann, J., Wiedemann, S., Okamura, H., Schneider, C., Iwan, K., et al. (2019). Unified Prebiotically Plausible Synthesis of Pyrimidine and Purine Rna Ribonucleotides. Science 366, 76–82. doi:10.1126/science.aax2747
Belloche, A., Garrod, R. T., Müller, H. S. P., and Menten, K. M. (2014). Detection of a Branched Alkyl Molecule in the Interstellar Medium: Iso -propyl Cyanide. Science 345, 1584–1587. doi:10.1126/science.1256678
Belloche, A., Garrod, R. T., Müller, H. S. P., Menten, K. M., Medvedev, I., Thomas, J., et al. (2019). Re-exploring Molecular Complexity with ALMA (ReMoCA): Interstellar Detection of Urea. A&A 628, A10. doi:10.1051/0004-6361/201935428
Belloche, A., Menten, K. M., Comito, C., Müller, H. S. P., Schilke, P., Ott, J., et al. (2008). Detection of Amino Acetonitrile in Sgr B2(N). A&A 482, 179–196. doi:10.1051/0004-6361:20079203
Beltrán, M. T., Codella, C., Viti, S., Neri, R., and Cesaroni, R. (2009). First Detection of Glycolaldehyde outside the Galactic Center. ApJ 690, L93–L96. doi:10.1088/0004-637X/690/2/L93
Braun, R., Bonaldi, A., Bourke, T., Keane, E., and Wagg, J. (2019). Anticipated Performance of the Square Kilometre Array - Phase 1 (SKA1). arXiv e-printsarXiv:1912.12699.
Calabrese, C., Uriarte, I., Insausti, A., Vallejo-López, M., Basterretxea, F. J., Cochrane, S. A., et al. (2020). Observation of the Unbiased Conformers of Putative Dna-Scaffold Ribosugars. ACS Cent. Sci. 6, 293–303. doi:10.1021/acscentsci.9b01277PMID: 32123748
Callahan, M. P., Smith, K. E., Cleaves, H. J., Ruzicka, J., Stern, J. C., Glavin, D. P., et al. (2011). Carbonaceous Meteorites Contain a Wide Range of Extraterrestrial Nucleobases. Proc. Natl. Acad. Sci. 108, 13995–13998. doi:10.1073/pnas.1106493108
Cernicharo, J., Agúndez, M., Cabezas, C., Tercero, B., Marcelino, N., Pardo, J. R., et al. (2021). Pure Hydrocarbon Cycles in TMC-1: Discovery of Ethynyl Cyclopropenylidene, Cyclopentadiene, and Indene. A&A 649, L15. doi:10.1051/0004-6361/202141156
Chyba, C., and Sagan, C. (1992). Endogenous Production, Exogenous Delivery and Impact-Shock Synthesis of Organic Molecules: an Inventory for the Origins of Life. Nature 355, 125–132. doi:10.1038/355125a0
Cocinero, E. J., Lesarri, A., Écija, P., Basterretxea, F. J., Grabow, J.-U., Fernández, J. A., et al. (2012). Ribose Found in the Gas Phase. Angew. Chem. Int. Ed. 51, 3119–3124. doi:10.1002/anie.201107973
Conway, J., Beswick, R., Bourke, T., Coriat, M., Ferrari, C., Jimenez-Serra, I., et al. (2021). Memo 20-01, SKA1 beyond 15GHz: The Science Case for Band 6 (Square Kilometre Array).
Cooper, G., Kimmich, N., Belisle, W., Sarinana, J., Brabham, K., and Garrel, L. (2001). Carbonaceous Meteorites as a Source of Sugar-Related Organic Compounds for the Early Earth. Nature 414, 879–883. doi:10.1038/414879a
de Marcellus, P., Meinert, C., Myrgorodska, I., Nahon, L., Buhse, T., d’Hendecourt, L. L. S., et al. (2015). Aldehydes and Sugars from Evolved Precometary Ice Analogs: Importance of Ices in Astrochemical and Prebiotic Evolution. Proc. Natl. Acad. Sci. USA 112, 965–970. doi:10.1073/pnas.1418602112
de Pree, C. G., Gaume, R. A., Goss, W. M., and Claussen, M. J. (1995). The Sagittarius B2 Star-forming Region. II. High-Resolution H66 Alpha Observations of Sagittarius B2 North. ApJ 451, 284. doi:10.1086/176218
Endres, C. P., Schlemmer, S., Schilke, P., Stutzki, J., and Müller, H. S. P. (2016). The Cologne Database for Molecular Spectroscopy, CDMS, in the Virtual Atomic and Molecular Data Centre, VAMDC. J. Mol. Spectrosc. 327, 95–104. doi:10.1016/j.jms.2016.03.005
Furukawa, Y., Chikaraishi, Y., Ohkouchi, N., Ogawa, N. O., Glavin, D. P., Dworkin, J. P., et al. (2019). Extraterrestrial Ribose and Other Sugars in Primitive Meteorites. Proc. Natl. Acad. Sci. USA 116, 24440–24445. doi:10.1073/pnas.1907169116
Glavin, D. P., McLain, H. L., Dworkin, J. P., Parker, E. T., Elsila, J. E., Aponte, J. C., et al. (2020). Abundant Extraterrestrial Amino Acids in the Primitive Cm Carbonaceous Chondrite Asuka 12236. Meteorit Planet. Sci. 55, 1979–2006. doi:10.1111/maps.13560
Herbst, E., and van Dishoeck, E. F. (2009). Complex Organic Interstellar Molecules. Annu. Rev. Astron. Astrophys. 47, 427–480. doi:10.1146/annurev-astro-082708-101654doi:10.1146/annurev-astro-082708-101654
Hollis, J. M., Jewell, P. R., Lovas, F. J., and Remijan, A. (2004). Green Bank Telescope Observations of Interstellar Glycolaldehyde: Low-Temperature Sugar. ApJ 613, L45–L48. doi:10.1086/424927
Hollis, J. M., Lovas, F. J., and Jewell, P. R. (2000). Interstellar Glycolaldehyde: The First Sugar. Astrophysical J. 540, L107–L110. doi:10.1086/312881
Insausti, A., Alonso, E. R., Tercero, B., Santos, J. I., Calabrese, C., Vogt, N., et al. (2021). Laboratory Observation of, Astrochemical Search for, and Structure of Elusive Erythrulose in the Interstellar Medium. J. Phys. Chem. Lett. 12, 1352–1359. doi:10.1021/acs.jpclett.0c03050
Jiménez-Serra, I., Martín-Pintado, J., Rivilla, V. M., Rodríguez-Almeida, L., Alonso Alonso, E. R., Zeng, S., et al. (2020). Toward the RNA-World in the Interstellar Medium-Detection of Urea and Search of 2-Amino-Oxazole and Simple Sugars. Astrobiology 20, 1048–1066. doi:10.1089/ast.2019.2125
Jiménez-Serra, I., Rodríguez-Almeida, L. F., Martín-Pintado, J., Rivilla, V. M., Melosso, M., Zeng, S., et al. (2022). Precursors of Fatty Alcohols in the ISM: Discovery of N-Propanol.
Jiménez-Serra, I., Testi, L., Caselli, P., and Viti, S. (2014). Detectability of Glycine in Solar-type System Precursors. ApJ 787, L33. doi:10.1088/2041-8205/787/2/L33
Jørgensen, J. K., Favre, C., Bisschop, S. E., Bourke, T. L., van Dishoeck, E. F., and Schmalzl, M. (2012). Detection of the Simplest Sugar, Glycolaldehyde, in a Solar-type Protostar with ALMA. ApJ 757, L4. doi:10.1088/2041-8205/757/1/L4
Jørgensen, J. K., van der Wiel, M. H. D., Coutens, A., Lykke, J. M., Müller, H. S. P., van Dishoeck, E. F., et al. (2016). The ALMA Protostellar Interferometric Line Survey (PILS). A&A 595, A117. doi:10.1051/0004-6361/201628648
Li, J., Shen, Z., Wang, J., Chen, X., Li, D., Wu, Y., et al. (2017). Widespread Presence of Glycolaldehyde and Ethylene Glycol Around Sagittarius B2. ApJ 849, 115. doi:10.3847/1538-4357/aa9069
Li, J., Wang, J., Qiao, H., Quan, D., Fang, M., Du, F., et al. (2020). Mapping Observations of Complex Organic Molecules Around Sagittarius B2 with the ARO 12 M Telescope. Monthly Notices R. Astronomical Soc. 492, 556–565. doi:10.1093/mnras/stz3337
Martín, S., Martín-Pintado, J., Blanco-Sánchez, C., Rivilla, V. M., Rodríguez-Franco, A., and Rico-Villas, F. (2019). Spectral Line Identification and Modelling (SLIM) in the MAdrid Data CUBe Analysis (MADCUBA) Package. A&A 631, A159. doi:10.1051/0004-6361/201936144
Martín-Pintado, J., Rizzo, J. R., de Vicente, P., Rodríguez-Fernández, N. J., and Fuente, A. (2001). Large-Scale Grain Mantle Disruption in the Galactic Center. Astrophysical J. 548, L65–L68. doi:10.1086/318937
McGuire, B. A. (2021). 2021 Census of Interstellar, Circumstellar, Extragalactic, Protoplanetary Disk, and Exoplanetary Molecules. arXiv e-printsarXiv:2109.13848.
McGuire, B. A., Loomis, R. A., Burkhardt, A. M., Lee, K. L. K., Shingledecker, C. N., Charnley, S. B., et al. (2021). Detection of Two Interstellar Polycyclic Aromatic Hydrocarbons via Spectral Matched Filtering. Science 371, 1265–1269. doi:10.1126/science.abb7535
Menor Salván, C., Bouza, M., Fialho, D. M., Burcar, B. T., Fernández, F. M., and Hud, N. V. (2020). Prebiotic Origin of Pre‐RNA Building Blocks in a Urea "Warm Little Pond" Scenario. ChemBioChem 21, 3504–3510. doi:10.1002/cbic.202000510
Patel, B. H., Percivalle, C., Ritson, D. J., Duffy, C. D., and Sutherland, J. D. (2015). Common Origins of RNA, Protein and Lipid Precursors in a Cyanosulfidic Protometabolism. Nat. Chem 7, 301–307. doi:10.1038/nchem.2202
Pickett, H. M., Poynter, R. L., Cohen, E. A., Delitsky, M. L., Pearson, J. C., and Müller, H. S. P. (1998). Submillimeter, Millimeter, and Microwave Spectral Line Catalog. J. Quantitative Spectrosc. Radiative Transfer 60, 883–890. doi:10.1016/S0022-4073(98)00091-0
Pizzarello, S., Cooper, G. W., and Flynn, G. J. (2006). The Nature and Distribution of the Organic Material in Carbonaceous Chondrites and Interplanetary Dust Particles, 625–652. doi:10.2307/j.ctv1v7zdmm.36
Requena‐Torres, M. A., Martin‐Pintado, J., Martín, S., and Morris, M. R. (2008). The Galactic Center: The Largest Oxygen‐bearing Organic Molecule Repository. ApJ 672, 352–360. doi:10.1086/523627
Requena-Torres, M. A., Martín-Pintado, J., Rodríguez-Franco, A., Martín, S., Rodríguez-Fernández, N. J., and de Vicente, P. (2006). Organic Molecules in the Galactic center. A&A 455, 971–985. doi:10.1051/0004-6361:20065190
Rivilla, V. M., Jiménez-Serra, I., Martín-Pintado, J., Briones, C., Rodríguez-Almeida, L. F., Rico-Villas, F., et al. (2021). Discovery in Space of Ethanolamine, the Simplest Phospholipid Head Group. Proc. Natl. Acad. Sci. USA 118, e2101314118. doi:10.1073/pnas.2101314118
Rivilla, V. M., Martín-Pintado, J., Jiménez-Serra, I., Martín, S., Rodríguez-Almeida, L. F., Requena-Torres, M. A., et al. (2020). Prebiotic Precursors of the Primordial RNA World in Space: Detection of NH2OH. ApJ 899, L28. doi:10.3847/2041-8213/abac55
Rodríguez-Almeida, L. F., Jiménez-Serra, I., Rivilla, V. M., Martín-Pintado, J., Zeng, S., Tercero, B., et al. (2021). Thiols in the Interstellar Medium: First Detection of HC(O)SH and Confirmation of C2H5SH. ApJL 912, L11. doi:10.3847/2041-8213/abf7cb
Rodríguez-Fernández, N. J., Martín-Pintado, J., de Vicente, P., Fuente, A., Hüttemeister, S., Wilson, T. L., et al. (2000). Non-equilibrium H_2 Ortho-To-Para Ratio in Two Molecular Clouds of the Galactic Center. Giant Mol. Clouds Galaxy 356, 695–704.
Widicus Weaver, S. L., and Blake, G. A. (2005). 1,3-Dihydroxyacetone in Sagittarius B2(N-LMH): The First Interstellar Ketose. ApJThe First Interstellar Ketose 624, L33–L36. doi:10.1086/430407
Zeng, S., Jiménez-Serra, I., Rivilla, V. M., Martín, S., Martín-Pintado, J., Requena-Torres, M. A., et al. (2018). Complex Organic Molecules in the Galactic Centre: the N-Bearing Family. Monthly Notices R. Astronomical Soc. 478, 2962–2975. doi:10.1093/mnras/sty1174
Zeng, S., Jiménez-Serra, I., Rivilla, V. M., Martín-Pintado, J., Rodríguez-Almeida, L. F., Tercero, B., et al. (2021). Probing the Chemical Complexity of Amines in the ISM: Detection of Vinylamine (C2H3NH2) and Tentative Detection of Ethylamine (C2H5NH2). ApJL 920, L27. doi:10.3847/2041-8213/ac2c7e
Zeng, S., Quénard, D., Jiménez-Serra, I., Martín-Pintado, J., Rivilla, V. M., Testi, L., et al. (2019). First Detection of the Pre-biotic Molecule Glycolonitrile (HOCH2CN) in the Interstellar Medium. Monthly Notices R. Astronomical Soc. Lett. 484, L43–L48. doi:10.1093/mnrasl/slz002
Keywords: square kilometre array, complex organic molecules, prebiotic chemistry, interstellar medium, astrochemistry
Citation: Jiménez-Serra I, Martín-Pintado J, Insausti A, Alonso ER, Cocinero EJ and Bourke TL (2022) The SKA as a Prebiotic Molecule Detector. Front. Astron. Space Sci. 9:843766. doi: 10.3389/fspas.2022.843766
Received: 26 December 2021; Accepted: 08 February 2022;
Published: 15 March 2022.
Edited by:
Ashraf - Ali, University of Maryland, College Park, United StatesReviewed by:
Eric Herbst, University of Virginia, United StatesCopyright © 2022 Jiménez-Serra, Martín-Pintado, Insausti, Alonso, Cocinero and Bourke. This is an open-access article distributed under the terms of the Creative Commons Attribution License (CC BY). The use, distribution or reproduction in other forums is permitted, provided the original author(s) and the copyright owner(s) are credited and that the original publication in this journal is cited, in accordance with accepted academic practice. No use, distribution or reproduction is permitted which does not comply with these terms.
*Correspondence: Izaskun Jiménez-Serra, aWppbWVuZXpAY2FiLmludGEtY3NpYy5lcw==