- 1Osservatorio Astrofisico di Torino, Istituto Nazionale di Astrofisica, Pino Torinese, Italy
- 2Department of Computer Sciences, University of Turin, Turin, Italy
- 3Shanghai Astronomical Observatory, Chinese Academy of Sciences, Shanghai, China
The issue of preservation and improvement of the Gaia catalogue throughout the next few decades is addressed. The goal of this study is to evaluate the feasibility of astrometric parameter update, for objects already in the catalogue, by inclusion of new observations from other space missions, in particular CSST. The proposed approach consists in modeling the astrometric fit of sources in the extragalactic region of the Gaia sample with new observations, using the stars in the local field as reference for each target. The concept is verified by simulation, in the framework of the expectations on the Gaia astrometric performance and on the data from the forthcoming CSST Optical Survey. This approach can mitigate the natural degradation of the initial precision on coordinates of Gaia sources with time by improving proper motions by a factor
1 Introduction
The Gaia mission (Gaia Collaboration et al., 2016) will provide a catalogue with unprecedented astrometric accuracy and astrophysical potential. The current version of the catalogue is the Data Release 3 (DR3) (Gaia Collaboration et al., 2021; Lindegren, L. et al., 2021)1, which is already widely used by the astronomical community. For our purposes, the catalogue is materialized mainly in the astrometric parameters (position, proper motion, and parallax) of the sample of observed objects (
The ambitious goal of (near-)absolute astrometric determination of Gaia is implemented by a complex approach based on repeated measurement of relative source positions at large angular separation over a few years. The projection of a set of new measurements onto the Gaia catalogue, conversely, can be formulated as a comparably simple problem under convenient assumptions. Preservation of the precision on individual object positions is potentially achieved by means of new measurements over time. This can be conceived in the context of a full-fledged Gaia-like future mission, possibly with modifications and trade-offs to improve on precision in general or with a focus on specific science topics, but the scope of this study is mainly focused on the benefits provided, at a much lower cost, by the observations generated by forthcoming astronomical missions.
In the next few years, some scientific endeavors are going to perform ambitious surveys of large fractions of the sky (mainly at comparably high-galactic latitude) in the visible and near-infrared (NIR) range, in particular the Chinese Space Station Telescope Optical Survey (CSST-OS), Euclid (Laureijs et al., 2020)2, and the Nancy Grace Roman Space Telescope (RST) (Eifler et al., 2021)3. A joint workshop on the three missions has recently been held4, evidencing the consistency of science goals and the complementarity of measurement techniques and performance. Although the missions are designed mainly toward cosmology and exo-planets for RST and CSST, they might contribute to the goal addressed by our investigation in several ways, for example:
• Additional measurements in the visibility on Gaia catalogue sources.
• Extension of the catalogue to the near IR range.
• Extension of the catalogue to the fainter magnitude range.
Hereafter, we will focus mainly on the former aspect.
In Section 2, the issue of natural degradation of the Gaia catalogue (like any other astrometric catalogue), and its potential preservation and improvement thanks to new measurements, is discussed. In Section 3, we address the potential of, in particular, CSST-OS data toward our intended goals. Finally, in Section 4, we discuss our findings and some potential future developments; then, we draw our conclusions.
2 Catalogue degradation and preservation
For simplicity, we deal with a simplified one-dimensional problem, describing a source by the true values of a single coordinate η (either α or δ) and its component proper motion μ and parallax ϖ. In the Gaia catalogue, such parameters are provided as a set of estimated values
where the time is expressed in years and the parallax phase is set to zero for simplicity. The Gaia parameters are considered as adequate approximations to the true star kinematics, possibly to be updated thanks to additional measurements.
The uncertainty on the position at time TE is naturally larger than the initial value (at the mission epoch) because of the error propagation from proper motion uncertainty:
With increasing time elapse, the proper motion contribution becomes dominant. The error on coordinates increases also in extrapolating star positions to the past, which is relevant, for example, for reconstruction of the galaxy history. The natural degradation on positions may be reasonably expected to be of order of a factor of 3 in one decade, and about 1 order of magnitude after a 30 year time elapse. The effect is shown in Figure 1, using the Gaia objects within 20 pc. The left-hand panel is the distribution of catalogue positional errors (RA and Dec), extrapolated to the end of mission from DR3 data according to the prescriptions in Lindegren, L. et al. (2021). The right-hand panel evidences the degradation on positional uncertainty suffered after 30 years from the mission epoch, corresponding to a factor
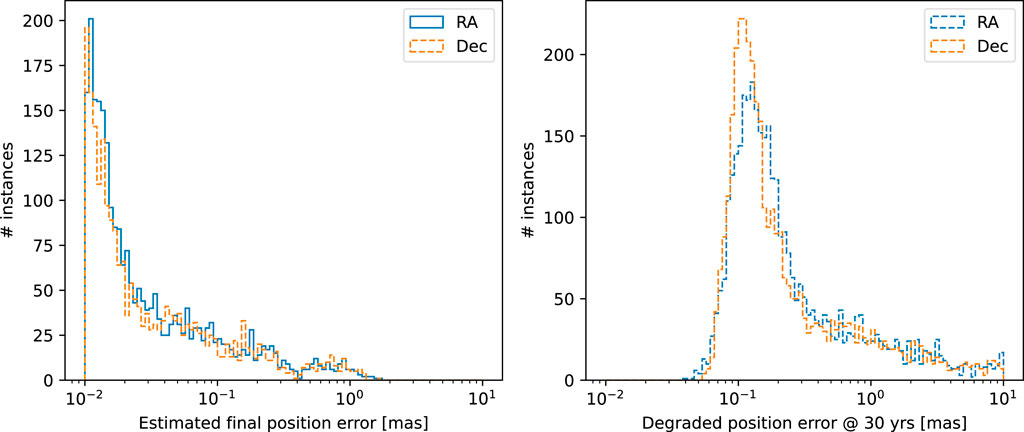
FIGURE 1. Estimated position errors at the end of mission for stars within 20 pc (left), from the Gaia EDR3 catalogue extrapolated to the end of mission, and natural degradation after 30 years due to proper motion errors.
Let us assume that a novel set of adequate precision measurements is provided by a forthcoming imaging instrument, for example, CSST-OS. They must be calibrated from a geometric standpoint, in particular, “attaching” them to the reference frame, for example, matching many detected objects to the available catalogue counterparts. The geometric calibration may take the form of plate correction factors. For each object, the coordinate estimate at the current epoch must be
- linked to the Gaia catalogue and reference frame and
- integrated with the previous knowledge to estimate new astrometric parameters.
The two steps will be discussed separately in the next two subsections. In principle, the new observations may help to.
- improve the precision of catalogue positions at different epochs and
- improve on the precision of proper motions.
Even moderate precision measurements, thanks to improvement on proper motion, will substantially mitigate position uncertainty degradation at a later epoch.
2.1 Materialization of the reference frame
The quality of the applied geometric calibration may be evaluated in terms of consistency of the calibrated observations with the reference frame. In the Gaia concept, the reference frame is materialized locally by the astrometric parameters of stars in that region, each source contributing its own uncertainty σηE from Eq. 2 at time TE of new observations. We assume that observed fields include a certain number K of Gaia sources, observed with individual uncertainty σηok depending on the current measurement parameters (instrument and operation setup). It is then possible to use K − 1 of them as references for the position of the remaining Kth object, and the process can be iterated on all sources. Actually, a few more constraints are imposed by calibration and geometric adjustment of the new observations (plate constants), but the number of degrees of freedom does not change significantly when many stars are available. The precision associated to the local framework (Abbas et al., 2017) can be considered as the collective uncertainty σηC associated to the coordinate ηC of the overall photo-center, computed as a weighted mean to account for individual uncertainties:
The frame materialization error adds to the measurement error, either as a systematic term for an individual observation, or randomly for a set of independent observations in different fields. The target position (dropping index K) projected in the Gaia reference frame materialized by the other K − 1 stars has therefore an accumulated uncertainty
The precision improves with the number of available reference stars (Gai et al., 2022c) and hence the field size. In favorable cases, the field referencing error is negligible with respect to the individual source uncertainty due to photon noise.
2.2 Update of astrometric parameters
For a given set of targets, the set of Gaia estimated parameters is assumed to be complemented by a single additional observation ηo at a subsequent epoch TE, affected by the noise level σηa and catalogue uncertainty σηE from Eq. 2, that is,
The solution of Eq. 1 for all three astrometric parameters, based on a single new measurement, must also be consistent with our previous knowledge on parameters from the Gaia catalogue. We define, thus, an error function
adopting the notation
With some computations, we get the system of linear equations:
which can be solved with ordinary techniques to provide the new estimate of object coordinate, proper motion, and parallax consistent with both Gaia and the additional measurement. Additionally, further computations (Gai et al., 2017), omitted here for the sake of brevity, lead to the estimate of the expected uncertainty on astrometric parameters.
3 CSST-OS case study
Xuntian, the Chinese Space Station Telescope (CSST) (Zhan, 2011; Gong et al., 2019; Cao et al., 2022), is a 2 m class instrument, planned for launch at the end of 2023, and located in proximity to the China Manned Space Station, thus allowing for periodic docking aimed at maintenance and upgrade. Nominal lifetime is set to 10 years. The CSST Optical Survey (Cao et al., 2018; Zhan, 2018) includes a Wide Survey, which will cover 17,500 square degrees of the extra-galactic sky in several bands from near-ultraviolet (NUV) to near-infrared (NIR), with a typical exposure time of 2 × 150 s, and a Deep Survey observing
An approximate estimation of typical CSST-OS astrometric performance based on the aforementioned literature, in terms of position uncertainty vs. magnitude for unresolved, near-solar spectral type sources, observed over several of the available bands according to the current operation definition, is shown in Figure 2 (left-hand side panel), evidencing the position uncertainties of the Wide Survey (solid blue line), Deep Survey (dashed red line), and Gaia position uncertainties expected at the end-of-mission (dotted black line) and at CSST epoch (dashed-dotted line).
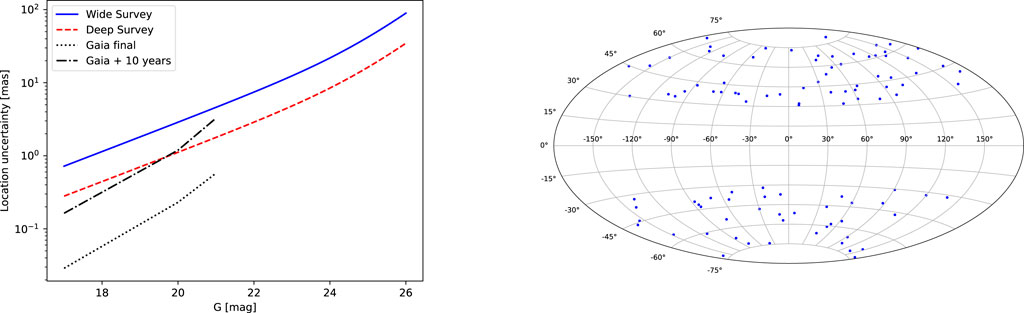
FIGURE 2. Left: approximate plot of position uncertainty vs. magnitude for unresolved sources with near solar color in CSST-OS observations, in the Wide Survey (solid blue line), Deep Survey (dashed red line), and Gaia position uncertainties, final (dotted black line) and extrapolated to CSST epoch (dashed-dotted black line). Right: field positions (in Galactic coordinates) selected for simulation of Gaia catalogue update by CSST-OS observations.
It may be noted that the bright limiting magnitude is significantly affected not only by the different exposure time of Wide and Deep Surveys but also by the actual source spectrum; precision is affected also by the number of observations in different filters. Also, saturation may not impose a severe limitation to astrometric performance, depending on detector response and operation, for example, CCD images affected by moderate blooming (Gai et al., 2022b) still provide high-astrometric precision in the horizontal (row) direction. Further investigations are in progress and will be presented in a forthcoming study.
Due to the large FOV, the CSST-OS images will include a large number of Gaia sources, even observing at comparably high-galactic latitude
Many faint components of the galactic populations will also be observed by the CSST-OS, as well as by other future missions, for example, Euclid and RST, sometimes more than once, at different epochs. Whenever enough observations are available, this will allow for determination of their astrometric parameters directly in the Gaia framework, which will greatly benefit, for example, galactic science studies. However, the issue depends on a number of as yet undefined aspects, in particular, actual operation, performance, and data policy of each mission, and shall not be further addressed herein.
We focus on the set of sources common to both Gaia and the CSST-OS, appearing, respectively, in the faint and bright region of the magnitude range of their observations. Therefore, the CSST-OS images will provide a high signal-to-noise ratio (SNR) measurement, with comparable bidimensional resolution, corresponding to an astrometric precision level comparable to the Gaia performance. In particular, we focus on the CSST-OS Wide Survey data, since they provide larger sky coverage than the Deep Survey. This context appears to have the potential for significant contributions to the maintenance of position estimates of the sources involved, according to the evaluation in Section 2.2, and by extension to the preservation of the overall catalogue.
3.1 Gaia DR3 population on high-galactic latitude sky
We address the potential benefits of the technique by selecting N = 100 random positions on the sky at comparably high-galactic latitude
The histograms illustrating the Gaia DR3 source distribution in the selected fields are shown in Figure 3. On the left-hand side panel, the effects of bright limiting magnitude is evidenced by the reduction in source density when the total population (red solid line) is cut, respectively, at G = 16 mag (black dashed-dotted line) and at G = 18 mag (blue dashed line); the number of sources affects the performance of field referencing, that is, the quality (astrometric precision) of the reference frame materialization. The two magnitude cutoffs are selected as an indication of realistic saturation levels over the range of CSST spectral bands.
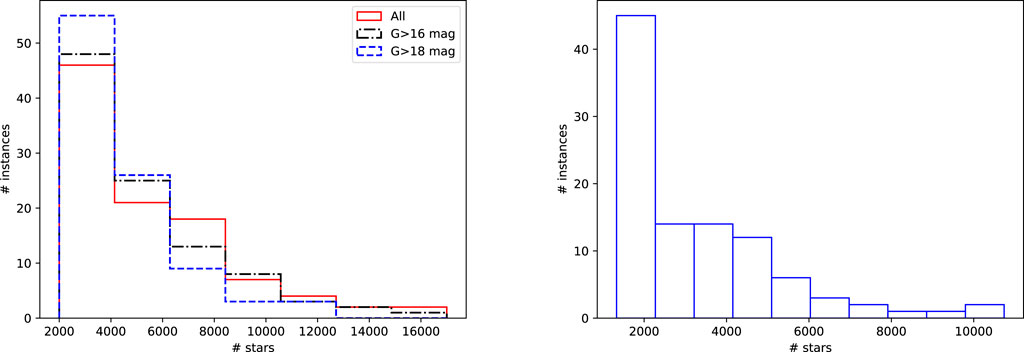
FIGURE 3. Histograms of number of fields populated with a given Gaia source density. Left: total DR3 population (red solid line), reduced by cutting to bright CSST-OS limiting magnitude G = 16 mag (black dashed-dotted line) and G = 18 mag (blue dashed line). Right: density of five- or six-parameter DR3 sources in the selected fields.
On the right-hand side panel, the distribution of sources actually used in our evaluation over the selected fields is shown. The sample is selected in the G ≥ 18 mag range (
The nominal Gaia errors used in our evaluation are derived from those reported in DR3, by simple scaling to final catalogue expectations according to the improvements related to the larger number of measurements, increasing from three to about 10 years of operations, that is, roughly by a factor
3.2 CSST-OS materialization of the reference frame
The assessment described in Section 2.1 is applied to the selected fields in order to provide a feeling of the realistic expectations on noise achieved in using the limited set of Gaia sources as materialization of the reference frame. According to the aforementioned considerations on bright limiting magnitude of CSST-OS observations, the computation is performed either on all DR3 sources or on those over the range G ≥ 16 mag or over the range G ≥ 18 mag.
The results are shown in Figure 4 as the solid red, black dashed-dotted, and blue dashed lines. In the most conservative case (G ≥ 18 mag), the reference frame materialization is affected by a typical error in the 3–5 μ as range, which is reduced to 1.5–2 μ as in the intermediate case (G ≥ 16 mag), and drops below 1 μas for most fields in the most optimistic case in which all Gaia sources are included. This may be achieved, for example, by extending CSST-OS observations with shorter exposures or by exploitation of moderately saturated images (Gai et al., 2022b). The feasibility and benefits of extended dynamic range observations shall be addressed in future with more detailed investigations. Independent observations of the same regions by other instruments (e.g., Euclid and RST) may further strengthen the reference frame materialization.
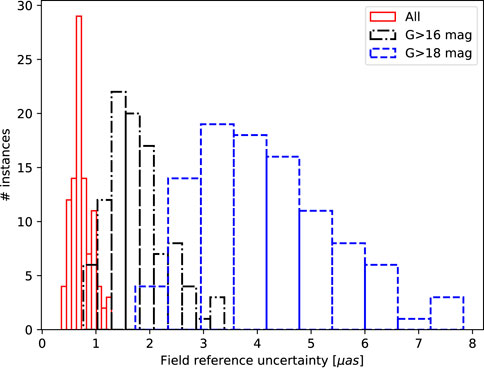
FIGURE 4. Histogram of uncertainty on reference frame materialization over the selected fields, using all stars (solid red line) or cutting to bright CSST-OS limiting magnitude G = 16 mag (black dashed-dotted line) and G = 18 mag (blue dashed line).
3.3 Astrometric parameter update on Gaia stars
Following the approach described in Section 2.2, the selected Gaia sources from Section 3.1 in each field are fed to a Monte Carlo process to evaluate numerically the noise level associated to a new estimate of astrometric parameters taking advantage of CSST-OS observations. For each source, a set of “true” values of position η, proper motion μ, and parallax ϖ is generated, compatible with the Gaia DR3 catalogue. Then, the uncertainty on parameters is derived by extrapolating the expected final catalogue errors to the measurement epoch TE. A set of NI = 10, 000 noisy observations is built according to the presumed CSST-OS position precision (Figure 2, left), each fed to the astrometric parameter estimation of Eqs. 7. Finally, the new estimates are compared with the initial “true” values, in order to assess the performance of the estimation process.
The histogram of discrepancy between estimated and input values of position δη and proper motion δμ is shown in Figure 5 on the left-hand side and right-hand side panels, respectively. The sample mean is consistent with the desired zero value, and the bell-shaped curve is not obviously different from a normal distribution. It is indeed possible that residual sample selection effects are present, at a level which does not appear to be critical with respect to the current preliminary investigation. The relevant statistical parameters (mean, median, and standard deviation) are reported in Table 1.
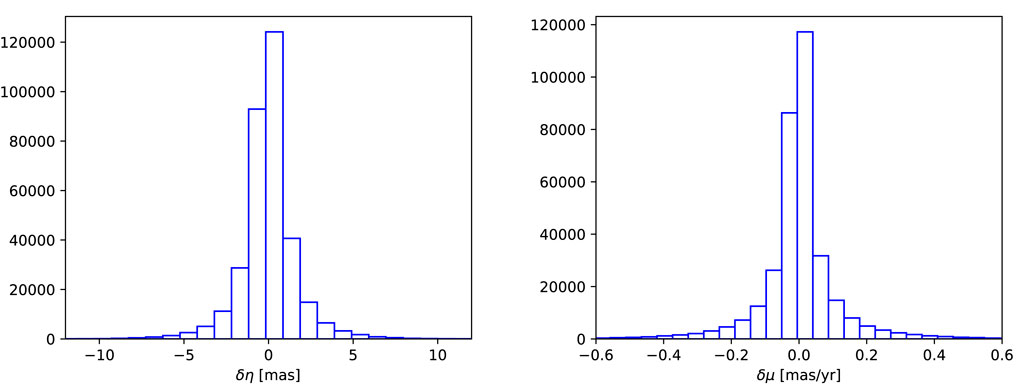
FIGURE 5. New estimate of the astrometric parameters with inclusion of CSST-OS measurements: distribution of the variation of position η (left) and proper motion μ (right).

TABLE 1. Estimated reference frame tie errors (CSST-OS data to Gaia frame) per field for position and proper motion: mean, median, and standard deviation of the simulated sample.
The position precision is shown in Figure 6, left-hand panel, illustrating the uncertainty at the Gaia catalogue central epoch (dotted black line), its degradation at epoch TE (dashed-dotted red line), and the histogram of RMS errors from the Monte Carlo (solid blue line), considered as representative of the performance of catalogue update with inclusion of the CSST-OS observations. The average position error on our sample is 0.3386 mas on the final Gaia catalogue, degraded after 10 years to 3.59 mas (due to the larger proper motion errors at fainter magnitudes), that is, by a factor of 10.60; the correction by CSST-OS data mitigates the average position error to 1.74 mas, that is, by a factor of 2.
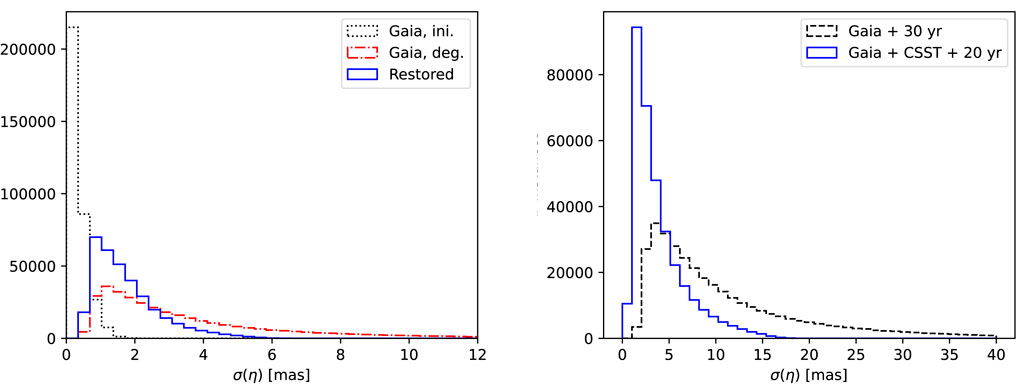
FIGURE 6. Left: position precision of Gaia, initial (dotted black line), degraded after 10 years (dashed-dotted red line), and partially restored with inclusion of CSST-OS measurements (solid blue line). Right: position precision of Gaia after 30 years, degraded without CSST-OS update (dashed black line) and mitigated with inclusion of CSST-OS measurements (solid blue line).
The right-hand panel shows the natural degradation of the Gaia catalogue position uncertainty at 30 years, without any update (dashed black line), and mitigated by inclusion of the CSST-OS observations taken 10 years after the mission. The average position error on our sample at 30 years is 11.06 mas without mitigation and 3.89 mas with it, corresponding to an improvement factor of 2.7.
The new measurements are actually effective in mitigating significantly the natural degradation with the time of catalogue positions.
The precision on proper motion is shown in Figure 7, evidencing the Gaia catalogue uncertainty (dashed black line) and the histogram of RMS errors from the Monte Carlo (solid blue line). CSST-OS observations, thanks to the longer time baseline of the compound dataset, actually improve on Gaia proper motions by an average factor of 3.6. The improvement on parallax is marginal, but this is not surprising since a single epoch observation is considered in our simple approach; basically, parallax just has to be taken into account to perform a correct estimate of position and proper motion, which benefit substantially from the additional measurement.
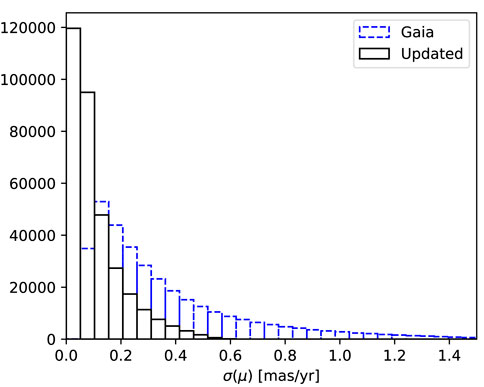
FIGURE 7. Proper motion precision of Gaia (dashed blue line) and improvement with inclusion of CSST-OS measurements (solid black line).
3.4 Gaia catalogue densification
The Gaia catalogue includes a number of extra-galactic sources or stars (mainly at the faint end) not endowed with full astrometric information, for which only position information is provided (i.e., two-parameter astrometry in the Gaia jargon). About 360 million such objects are included in DR2, with more than 500,000 bona fide QSOs. CSST-OS observations include most of them, in the common areas, as well as a huge number of additional sources, either extragalactic objects or faint components of the Milky Way (e.g., far away or dwarf stars), providing a quite interesting complement to the Gaia survey. Since CSST-OS-only sources have in general a single observation, no proper motion or parallax can be deduced but only positions (two-parameter astrometry). The individual precision degrades naturally with increasing magnitude, consistently with the decreasing photon level (an actual image size for resolved objects).
Sources are observed against a framework of Gaia sources and therefore may be located in the Gaia reference frame, with reference frame tie accuracy corresponding to the field averaging error in available reference Gaia sources, that is, at the level of a few μ as (Figure 4) or better. The net result is that a large number of faint sources, beyond the Gaia limiting magnitude, can be included in an “extended catalogue” with much higher density, useful for narrow field observations, for example, by large size, narrow field telescopes. Such fainter-than-Gaia sources can be used in all legitimacy as auxiliary materializations of the Gaia catalogue proper. Also, since most sources are imaged in both visible and near-infrared (NIR) bands, the catalogue is implicitly extended to the longer wavelength range; this aspect is promising, but it will require dedicated future investigations.
4 Discussion
The astrometric benefit achieved from complementing the Gaia catalogue with the CSST-OS Wide Survey observations is remarkable, since the Gaia catalogue may be preserved to a large extent, on positions, and significantly improved, on proper motions, over a significant fraction of the sky
Besides, it may be noted that the technique outlined in this study is based on local field and individual source processing, thus implying a very low-computational cost of astrometric processing per se. In computer science terms, the construction of the enhanced Gaia catalogue from the combination of the final release of Gaia and CSST-OS data is an “embarrassingly parallel” problem. Potential troubles are mostly hidden in the calibration aspects within either project and should be investigated in detail in the implementation process. The cost to benefit budget of the proposed CSST-OS enhanced Gaia catalogue seems to be appealing, thus suggesting the worth of further investigation, also focused on the NIR extension.
The proposed technique for catalogue maintenance and extension is applicable, in principle, to the data provided by any other space mission, for example, Euclid and the Nancy Grace Roman Space Telescope (RST), providing comparably deep exposures on rather wide fields in the visible and/or NIR range. A preliminary assessment of the performance for either mission may be deployed according to the present study case and eventually implemented in more detailed frameworks.
For each set of measurements and for many individual five-parameter sources, the Gaia catalogue precision may be largely restored on positions and improved on proper motion. Furthermore, the combination of several observations at different epochs can be expected to provide even better results, in this case also on parallaxes.
Many sources already in Gaia may thus be “boosted” from two-parameter to five-parameter astrometry, as the additional information will allow to retrieve their parallax and proper motion. Besides, sources not included in the Gaia catalogue, for example, faint stars and extragalactic objects beyond Gaia’s limiting magnitude, may be “attached” to it by position, even with a single observation by the CSST-OS, Euclid, or RST, as two-parameter objects, with sufficient observations, parallax, and proper motion may be derived as well.
This densified catalogue will be useful for operation of several existing or future large telescopes, either in space or ground based, which in turn may provide high-precision relative astrometry (mainly in space), based on the improved reference frame. An important application example is the recently launched JWST (McElwain et al., 2020; Stiavelli, 2022)5 also because of its potentially much higher astrometric precision, bearing the potential of impressive improvements on few selected targets. After a default period of one year, in which exclusive access is reserved to proposers, JWST observations will be made available to other scientists for additional research, for example, for astrometric purposes. The main shortcoming of JWST, in our opinion, is the limited field of view (few arc minutes) of its imaging instrumentation, which result in low probability of having a sufficient number of reference Gaia objects. JWST, in particular, is endowed with the near-infrared camera6 (NIRCam), observing from 0.6 to 5 μm on a field of view of 2 × 2′.2 × 2′.2, with a pixel scale of 0.031 arc sec/pixel, through a set of narrow to wide band filters.
However, the densified Gaia catalogue, derived by inclusion of CSST-OS observations, provides just the required extension of the reference frame to fainter magnitudes, significantly raising the probability of having enough reference sources in the NIRCam field. Therefore, the densified Gaia catalogue appears to be an extremely convenient tool for future JWST observation and similarly for other telescopes.
An obvious solution for preservation and improvement of the Gaia catalogue may appear to be a replication of the Gaia mission itself after some decades, but this is not necessarily the case. First of all, space technology develops rapidly, and this may suggest deep modifications in the implementation concept; also, our understanding of the Universe evolves as well, and the science goals of the next decades may set performance and/or operation requirements significantly different from the current Gaia design. Bidimensional measurement is likely to be a must (Vecchiato et al., 2022). Besides, future missions will build on an already consolidated ground taking advantage of the high-precision Gaia catalogue, which, as outlined herein, may be preserved and somewhat improved without the need for a full-fledged global astrometric instrument. High precision, relative astrometry missions are being actively investigated for exoplanet characterization and cosmology (Malbet et al., 2021; Gai et al., 2022c).
Significant improvements in terms of “absolute” measurement quality, for example, pushing the full sky reference frame at (or well below) the micro-arc sec level would probably require a scale of mission significantly larger than Gaia. Indeed, a scanning satellite appears to be a most convenient way to provide such coverage, although propositions for a pointed mission concept have been advanced (Zacharias and Dorland, 2006), not without merit. However, a significantly larger instrument is probably required in order to improve on individual astrometric precision and sensitivity and to achieve higher source density and include a larger set of extragalactic sources. Multiple epoch measurements are necessary for proper motion and parallax, and in any case of complex objects requiring dynamical solutions, for example, binary systems, solar system objects, and extra-solar planetary systems (Gaia Collaboration et al., 2021). Options toward the goal of a large size, multiple line-of-sight instrument implementing true simultaneous large-angle astrometric measurements on the sky are being investigated by the authors’ team (Gai et al., 2022a; Riva et al., 2022).
A family of high-precision global astrometry missions might be envisaged, on a time scale of a few 10 years after each other, to ensure maintenance and improvement of an excellent reference frame in the visible and other spectral bands over the long period. Of course, the scientific requirements and available technologies cannot easily be extrapolated to the remote future. Therefore, the discussion of even the first Gaia successor is beyond the scope of this study. In the meantime, the CSST-OS may prove the feasibility of such perspective and achieve quite relevant early results.
5 Conclusion
The preliminary investigation of a simple approach for maintenance and improvement of the Gaia catalogue, preventing natural degradation of the knowledge on individual positions, provides encouraging results. Usage of available sources as proxies of the Gaia catalogue, in a comparably small field around each object of interest, is straightforward and quite effective, providing a good local reference.
The case study of the CSST-OS Wide Survey is considered: the coordinates of the faint Gaia sample in extragalactic regions (about 40% of the sky) can be restored nearly to the initial Gaia precision, improving at the same time the proper motions by a factor
Besides, thanks to the sensitivity of CSST-OS, this process may provide a densification of the Gaia catalogue to fainter magnitudes, including many extragalactic objects and the faint galactic population. This enhanced Gaia catalogue will be precious in support of future space and ground based telescopes.
Finally, dedicated missions for maintenance and improvement of the Gaia catalogue, or for relative astrometry, may be planned with considerable flexibility, building on the Gaia catalogue and its enhanced version achieved also thanks to the inclusion of CSST-OS observations.
Data availability statement
The raw data supporting the conclusion of this article will be made available by the authors, without undue reservation.
Author contributions
GM had the idea for the study and wrote the main part of code and manuscript. VA, BD, and RA helped develop and test the code and write the manuscript. RC made critical contributions to the inversion algorithm. LM made a critical review of the idea and supported the work throughout the process. QZ helped with CSST information. All authors read and commented on the manuscript.
Funding
The activity has been partially funded by a grant (ASTRA: Astrometric Science and Technology Roadmap for Astrophysics) from the Italian Ministry of Foreign Affairs and International Cooperation (MAECI) and by the Italian Space Agency (ASI) under contract 2018-24-HH.0. Funding for the DPAC has been provided by national institutions, in particular, the institutions participating in the Gaia Multilateral Agreement.
Acknowledgments
The authors acknowledge the support from the Italian Ministry of Foreign Affairs and International Cooperation (MAECI), grant ASTRA (Astrometric Science and Technology Roadmap for Astrophysics), and from the Italian Space Agency (ASI). This work has made use of data from the European Space Agency (ESA) mission Gaia (https://www.cosmos.esa.int/gaia), processed by the Gaia Data Processing and Analysis Consortium (DPAC, https://www.cosmos.esa.int/web/gaia/dpac/consortium). The Reviewers comments significantly helped the authors in improving on paper clarity and readability. This work has been partially funded by a grant from the executive programme of scientific and technological cooperation between Italy and China, grant number 2018YFE0118500.
Conflict of interest
The authors declare that the research was conducted in the absence of any commercial or financial relationships that could be construed as a potential conflict of interest.
Publisher’s note
All claims expressed in this article are solely those of the authors and do not necessarily represent those of their affiliated organizations, or those of the publisher, the editors, and the reviewers. Any product that may be evaluated in this article, or claim that may be made by its manufacturer, is not guaranteed or endorsed by the publisher.
Footnotes
1Description at https://cosmos.esa.int/web/gaia/dr3; data available on https://gea.esac.esa.int/archive/
2https://sci.esa.int/web/euclid.
4https://local.strw.leidenuniv.nl/cms/web/2021/20210621/info.php?wsid=72
6https://jwst.stsci.edu/instrumentation/nircam
References
Abbas, U., Bucciarelli, B., Lattanzi, M., Crosta, M., Gai, M., Smart, R., et al. (2017). The short-term stability of a simulated differential astrometric reference frame in the gaia era. Publ. Astron. Soc. Pac. 129, 054503. doi:10.1088/1538-3873/aa60ba
Cao, Y., Gong, Y., Liu, D., Cooray, A., Feng, C., and Chen, X. (2022). Anisotropies of cosmic optical and near-IR background from the China space station telescope (CSST). Mon. Not. R. Astron. Soc. 511, 1830–1840. doi:10.1093/mnras/stac151
Cao, Y., Gong, Y., Meng, X., Xu, C. K., Chen, X., Guo, Q., et al. (2018). Testing photometric redshift measurements with filter definition of the Chinese Space Station Optical Survey (CSS-OS). Mon. Not. R. Astron. Soc. 480, 2178–2190. doi:10.1093/mnras/sty1980
Eifler, T., Miyatake, H., Krause, E., Heinrich, C., Miranda, V., Hirata, C., et al. (2021). Cosmology with the roman space telescope - multiprobe strategies. Mon. Not. R. Astron. Soc. 507, 1746–1761. doi:10.1093/mnras/stab1762
Gai, M., Busonero, D., and Cancelliere, R. (2017). Performance of an algorithm for estimation of flux, background, and location on one-dimensional signals. Publ. Astron. Soc. Pac. 129, 054502. doi:10.1088/1538-3873/aa5c9c
Gai, M., Riva, A., Vecchiato, A., Busonero, D., Bucciarelli, B., Crosta, M., et al. (2022a). Triple line of sight telescopes for space astrometry. SPIE 12180, 121801G-1–121801G-8.
Gai, M., Vecchiato, A., Riva, A., Busonero, D., Lattanzi, M., Bucciarelli, B., et al. (2022b). Astrometric precision tests on TESS data. Publ. Astron. Soc. Pac. 134, 035004. doi:10.1088/1538-3873/ac584a
Gai, M., Vecchiato, A., Riva, A., Butkevich, A. G., Busonero, D., Qi, Z., et al. (2022c). Relative astrometry in an annular field. Publ. Astron. Soc. Pac. 134, 035001. doi:10.1088/1538-3873/ac50a1
Gaia Collaboration, Brown, A. G. A., Vallenari, A., Prusti, T., Babusiaux, C., Biermann, M., et al. (2021). Gaia early data release 3. Summary of the contents and survey properties. Astron. Astrophys. 649, A1. doi:10.1051/0004-6361/202039657
Gaia Collaboration, Prusti, T., de Bruijne, J. H. J., Brown, A. G. A., Babusiaux, C., Bailer-Jones, C. A. L., et al. (2016). The Gaia mission. Astron. Astrophys. 595, A1. doi:10.1051/0004-6361/201629272
Gong, Y., Liu, X., Cao, Y., Chen, X., Fan, Z., Li, R., et al. (2019). Cosmology from the Chinese space station optical survey (CSS-OS). Astrophys. J. 883, 203. doi:10.3847/1538-4357/ab391e
Laureijs, R., Racca, G. D., Mellier, Y., Musi, P., Brouard, L., Boenke, T., et al. (2020). Euclid mission status after mission critical design. Soc. Photo-Optical Instrum. Eng. (SPIE) 11443, 114430F. doi:10.1117/12.2563145
Lindegren, L., Klioner, S. A., Hernández, J., Bombrun, A., Ramos-Lerate, M., Steidelmuller, H., et al. (2021). Gaia early data release 3 - the astrometric solution. Astron. Astrophys. 649, A2. doi:10.1051/0004-6361/202039709
Malbet, F., Boehm, C., Krone-Martins, A., Amorim, A., Anglada-Escude, G., Brandeker, A., et al. (2021). Faint objects in motion: The new frontier of high precision astrometry. Exp. Astron. (Dordr). 51, 845–886. doi:10.1007/s10686-021-09781-1
McElwain, M. W., Feinberg, L. D., Kimble, R. A., Bowers, C. W., Knight, J. S., Niedner, M. B., et al. (2020).Status of the james webb space telescope mission, Soc. Photo-Optical Instrum. Eng. (SPIE) Conf. Ser., 11443, 114430T. doi:10.1117/12.2562425
Riva, A., Gai, M., Vecchiato, A., et al. (2022). Robotic unit multiple lines of vieW. SPIE 12182, 121821X-1–121821X-8. doi:10.1117/12.2630111
Stiavelli, M. (2022). “JWST first light,”. APS april meeting abstracts, 2022.of APS Meeting Abstracts.
Vecchiato, A., Gai, M., Lattanzi, M. G., et al. (2022). Experimenting techniques of global astrometry from space. SPIE 12180, 121801H-1–121801H-9.
Zacharias, N., and Dorland, B. (2006). The concept of a stare-mode astrometric space mission. Publ. Astron. Soc. Pac. 118, 1419–1427. doi:10.1086/508299
Zhan, H. (2018). 42nd COSPAR Scientific Assembly, 42, 16.An overview of the Chinese space station optical survey.
Keywords: astrometry, catalogs, instrumentation: high angular resolution, methods: numerical, techniques: image processing
Citation: Gai M, Vecchiato A, Busonero D, Riva A, Cancelliere R, Lattanzi M and Qi Z (2022) Consolidation of the Gaia catalogue with Chinese Space Station Telescope astrometry. Front. Astron. Space Sci. 9:1002876. doi: 10.3389/fspas.2022.1002876
Received: 25 July 2022; Accepted: 14 November 2022;
Published: 02 December 2022.
Edited by:
László Szabados, Konkoly Observatory (MTA), HungaryReviewed by:
Norbert Zacharias, United States Naval Observatory (USNO), United StatesLuis Henry Quiroga-Nuñez, Florida Institute of Technology, United States
Copyright © 2022 Gai, Vecchiato, Busonero, Riva, Cancelliere, Lattanzi and Qi. This is an open-access article distributed under the terms of the Creative Commons Attribution License (CC BY). The use, distribution or reproduction in other forums is permitted, provided the original author(s) and the copyright owner(s) are credited and that the original publication in this journal is cited, in accordance with accepted academic practice. No use, distribution or reproduction is permitted which does not comply with these terms.
*Correspondence: M. Gai, bWFyaW8uZ2FpQGluYWYuaXQ=