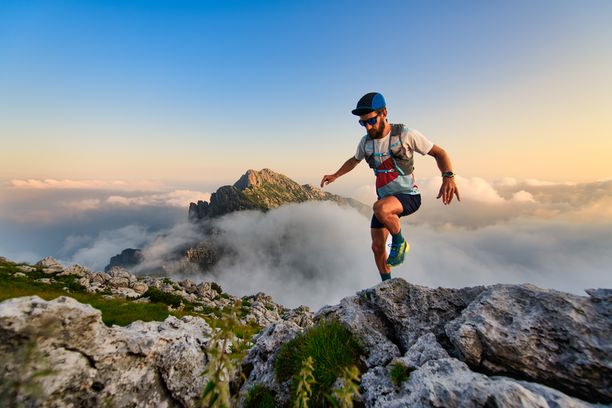
95% of researchers rate our articles as excellent or good
Learn more about the work of our research integrity team to safeguard the quality of each article we publish.
Find out more
MINI REVIEW article
Front. Astron. Space Sci., 22 September 2021
Sec. Space Physics
Volume 8 - 2021 | https://doi.org/10.3389/fspas.2021.725677
This article is part of the Research TopicCold-Ion Populations and Cold-Electron Populations in the Earth’s Magnetosphere and Their Impact on the SystemView all 18 articles
Diffuse aurora is generated by the precipitation of hot electrons from the central plasma sheet due to wave-particle interaction. Near magnetic local noon (MLN), the diffuse aurora was often observed in structured forms, such as in stripy or patchy. In the magnetosphere, when the hot electrons meet with a cold plasma structure, the threshold of resonance energy for the electrons in the cold plasma region can be lowered, leading to more electrons being involved in the wave-particle interaction and being scattered into the loss cone. As a result, stronger diffuse aurora can be produced in the correspondent region. Based on this mechanism, the structured dayside diffuse auroras have been suggested to correspond to the cold plasma structures in the dayside outer magnetosphere. This brief review focuses on showing that 1) the stripy diffuse auroras observed near MLN are specifically informative, 2) there are two types of diffuse aurora near MLN, which may correspond to cold plasmas originating from inside and outside the magnetosphere, respectively, and 3) we can study the inside-outside coupling by using the interaction between diffuse and discrete auroras observed near MLN.
The auroras observed on the ground can be classified into two broad categories, i.e., discrete and diffuse auroras, which are different in appearance and generation mechanisms. Discrete auroras are generally characterized by intense auroral emission in both green (wavelength of 557.7 nm) and red (wavelength of 630.0 nm) lines and often appear as auroral arcs, bands, curls, and rays. It is believed that the discrete auroras are produced by electrons accelerated by quasi-static electric field or Alfven waves and are associated with the field-aligned current. Diffuse auroras generally appear at the equatorward edge of the auroral oval (Lui et al., 1973) with relatively homogenous emission in the green line observations. Initially, diffuse aurora studies mainly focused on where the source particles are from, whether these particles have been accelerated, or how these particles are scattered into the loss cone. In the 1970s, thanks to the rapid development of satellites, it was revealed that the electrons for producing the diffuse aurora originated from the central plasma sheet (CPS) (Meng et al., 1979), and their energy did not significantly change from the source region to the topside ionosphere. Therefore, the research on diffuse aurora after the 1980s mainly focused on the scattering mechanism of particles. Now, it has been widely accepted that the electron diffuse aurora are generated by precipitation of hot electrons (> 1.0 Kev) from the CPS through wave-particle interaction by the whistler-mode chorus (Ni et al., 2011b; Nishimura et al., 2010; Thorne et al., 2010) or electron cyclotron harmonic (ECH) (Horne and Thorne, 2000; Liang et al., 2011; Ni et al., 2011a; Zhang et al., 2014) waves.
The source particle of diffuse aurora is the earthward particle injection during substorms. Because the injected electrons drift from midnight toward dawn and noon sectors, the diffuse aurora is the strongest on the post-midnight sector (Newell et al., 2009) and is gradually weakening toward the dawn and noon sectors. Dayside diffuse aurora has been early noticed (Newell and Meng, 1992; Sandholt et al., 1998). Sandholt et al. (1998) classified the dayside optical auroras into seven types, in which the type 3 aurora was the diffuse aurora. For the electron scattering mechanisms, a few studies suggested that dayside diffuse auroras are associated with the chorus waves (Ebihara et al., 2007; Ni et al., 2014; Nishimura et al., 2013). At the same time, it has been noticed that ECH waves play a role in producing the diffuse auroras on the dayside (Han et al., 2017; Lou et al., 2021). Frey et al., 2019 summarized that dayside diffuse auroras show various structures and suggested that the diffuse auroral structure may correspond to low-energy plasma density structures in the dayside outer magnetosphere.
This paper will not discuss the electron scattering mechanism for producing the diffuse aurora but will focus on the implications of structured dayside diffuse aurora on the cold plasma structuring in the dayside outer magnetosphere.
Although satellites have advantages in providing global coverage in auroral observation, they generally have limitations on spatial and temporal resolutions. In contrast, ground-based instruments can easily overcome these disadvantageous factors. On making dayside optical auroral observations on the ground, the observation site is required to be under the aurora oval and, at the same time, be dark on the dayside. Only a few places can meet these requirements on Earth. Svalbard Island in the north of Europe is one of such places in the Northern Hemisphere. Chinese Yellow River Station (YRS) is situated at Ny-Alesund in Svalbard, where is just fit for making dayside optical auroral observations from the beginning of November to the middle of February for each year. YRS has three individual all-sky imagers equipped with narrowband filters centered at 427.8, 557.5, and 630.0 nm, respectively (Hu et al., 2009). YRS has carried out auroral observations in the same mode, i.e., with 10-s time resolution, since November 2003 up to the present. The long-lasting and high-quality data accumulation provides an unprecedented opportunity for the dayside auroral study. The observational results discussed here are mainly obtained from the YRS station.
Using observations from Svalbard, Sandholt et al. (1998) suggested that the dayside diffuse auroras are caused by precipitation of CPS electrons drifted from the nightside. However, they did not do further studies on it. Using 7-years observations from YRS, Han et al. (2015) carried out a statistical survey on the dayside diffuse aurora. They found that the dayside diffuse auroras can be classified into two broad categories: structured and unstructured diffuse auroras. The structured diffuse auroras are predominantly observed near magnetic local noon (MLN), and the unstructured ones are in the morning. The main results and the implications are illustrated in Figure 1. The observational results were explained as follows by considering the source particle properties of diffuse aurora and the cold plasma structuring in the dayside magnetosphere (Han et al., 2015; Han et al., 2017).
FIGURE 1. The implications of the statistical results about dayside diffuse aurora. The convection-aligned stripy diffuse aurora observed near magnetic local noon is very informative (revised from Figure 15 in Han et al., 2015).
The source particles for diffuse auroras are hot electrons from CPS (Horne et al., 2003). These particles originate from the earthward particle injection during substorms on the nightside (Newell et al., 2009) and drift around the Earth toward dawn and local noon sectors. As drifting, a portion of the electrons can be scattered into the loss cone and produce diffuse aurora. This leads to the diffuse aurora being the most intense near midnight and gradually weakening toward dawn and noon sectors (Newell et al., 2004). At the same time, the electron density gradually decreases from midnight toward the local noon.
The essential differences for the structured and unstructured diffuse auroras lie in the size of the diffuse auroral region. If the auroral region is large enough, it will be full of the field of view and thus be observed as ‘unstructured’. Otherwise, if the diffuse auroral region is not so large and its shape can be identified in the field of view, it will be observed as “structured”. In the morning, the diffuse auroras are predominantly observed as “unstructured”. This can be understood as that the source particle for diffuse aurora still has a high density in the morning, so it can produce diffuse aurora in a large area, which is often observed as “unstructured”.
Han et al. (2015) suggested that the reasons for the diffuse auroras observed near MLN become much structured should be related to cold-plasma structuring in the dayside outer magnetosphere. In previous, both theoretical (Demekhov and Yu., 1994; Ni et al., 2014) and observational (Nishimura et al., 2013) works have shown that a structured diffuse aurora corresponds to a cold plasma structure in the magnetosphere. The cold plasma structure plays a crucial role in the generation of the structured diffuse aurora by decreasing the energy threshold and increasing the growth rate of whistler cyclotron instability (Brice and Lucas, 2012; Li et al., 2011). This means that the existence of the cold plasma structure will enable more particles to be scattered into the loss cone and thus lead to a stronger aurora. Based on this theory, because the density of the source particle decreases toward local noon, even though some of them still can be scattered into loss cone and produce diffuse aurora, the auroral intensity may be too weak to be detected by a camera in general. Under such a condition, if there is a cold plasma structure in the magnetosphere, more electrons passing through this structure will be scattered into the loss cone. Thus, the auroral intensity conjugate to the cold plasma structure will be increased and thus be observed as structured diffuse aurora. This well explained why the structured diffuse auroras are predominantly observed near local noon.
Satellite observations frequently detected cold plasma structures in the dayside outer magnetosphere (Chen and Moore, 2006; Lee et al., 2015). However, it is not easy to determine the three-dimensional (3-D) shape of the cold plasma even with simultaneous observations from multiple satellites. Han et al. (2015) noticed that the structured diffuse auroras observed near MLN mainly show stripy and patchy forms. Importantly, they found that the stripy diffuse auroras are convection-aligned and show tapered at the end. Based on these properties, Han et al., 2015 suggested that these stripy diffuse auroras should correspond to wedge-like cold plasma structures radially aligned from low to high L shells in the dayside outer magnetosphere. Figure 1 shows a stripy diffuse aurora example and schematically illustrates the projection of the wedge-like structure on the equatorial plane.
The possible origin for the cold plasmas observed in the dayside outer magnetosphere include ionospheric outflow, plasma aspherical drainage plume, and solar wind penetration (Delzanno et al., 2021). The 3-D shape of the cold plasma structure is useful in inferring its generation mechanism. Based on the convection-aligned orientation of the stripy diffuse aurora, Han et al. (2015) suggested that the wedge-like cold plasma structure in the magnetosphere should also be convection-aligned, as indicated in Figure 1. This information led Han et al. (2017) to suggest that the cold plasmas forming the wedge-like structure are most likely from the ionospheric outflow. It is well known that solar EUV ionization can produce dense plasma in the midlatitude ionosphere. When these plasmas were conveyed toward high latitudes by ionospheric convection, stripy high-density plasma structures may be formed in the ionosphere. These structures have been observed as the tongue of ionization (Foster et al., 2005) or poleward moving plasma concentration enhancement (PMPCE) (Zhang et al., 2013). Further, Zhang et al. (2016) indicated that the PMPCE might be associated with ionospheric outflows. Thus, from the convection-aligned property of the stripy diffuse aurora, we infer that 1) the correspondent cold plasma structure in the dayside outer magnetosphere should be in a wedge-like form and be convection-aligned, 2) these cold plasmas are originated from the ionospheric outflow, and 3) it is the ionosphere that determines the convection-aligned features for the wedge-like cold plasma structure. We expect future observations to verify these inferences.
By examining the observations at Yellow River Station, Han et al. (2017) revealed that there exist two types of structured diffuse auroras near MLN, which are with obviously different dynamical properties and are called Type 1 and Type 2 diffuse auroras. The auroral movies provided in Supporting Information (SI) in Han et al. (2017) showed the differences in the dynamic properties for the two types of diffuse auroras in detail. Han et al. (2017) showed that Type 1 diffuse auroras are generally in stripy or patchy forms and show fast drifting or pulsating. Actually, Type 1 diffuse auroras are the same as the structured patchy diffuse auroras defined in Han et al. (2015). Thus, they may reflect the cold plasma structuring in the dayside outer magnetosphere, as discussed above.
On the other hand, Type 2 diffuse auroras are always adjacent to the discrete aurora oval and drift together with the nearby discrete aurora much slower than the drifting speed of Type 1. This result naturally reminds us to consider the possible linkage between the Type 2 diffuse aurora and the nearby discrete aurora. Han et al. (2017) confirmed this linkage by coordinated observations from Magnetospheric Multiscale (MMS) satellites near the magnetopause and ground-based all-sky imager at Yellow River Station. Han et al. (2017) found that Type1 and Type 2 diffuse auroras correlate with the number density increase of O+ and He2+ ions, respectively. It is generally accepted that O+ ions are originated from the ionosphere. In contrast, highly charged ion species, such as He2+ ions, are originated from the solar wind. Therefore, Han et al. (2017) suggested that Type 2 diffuse auroras are related to cold plasmas originating from the solar wind.
The possible mechanisms for the solar wind particles entering the magnetosphere include the dayside reconnection (Fuselier and Lewis, 2011), high-latitude reconnection (Song et al., 1994), and impulsive plasma penetration (Lemaire, 1977). The solar wind consists primarily of electrons and protons with the temperature of the order of 10 eV flowing at around 400 km/s. For ions, the flow kinetic energy is ∼ 1.0 keV and dominates over the thermal energy. The total energy of the magnetosheath ions is ∼ 1.0 keV, while that of the electrons is even lower. Although solar wind particles are traditionally not regarded as cold plasmas, their temperature is about one order lower than that of the electrons in the CPS. Therefore, once entering the magnetosphere, they can also act as a cold plasma structure in producing the diffuse aurora by decreasing the threshold energy of the electrons participating in the wave-particle resonances.
In summary, Han et al., 2017 showed two types of diffuse aurora near MLN, which may correspond to cold plasmas originating from inside and outside the magnetosphere, respectively. It provides a feasible method for inferring the two-dimensional information of magnetosheath particles penetrating into the magnetosphere near the dayside magnetopause.
The diffuse auroras are all on the closed field lines (inside the magnetosphere). Near MLN, the discrete auroras are believed to be on the open field lines (outside the magnetosphere) (Lockwood, 1997). The diffuse and discrete auroras observed near MLN often show close interaction (Han et al., 2015). Therefore, we suggest that we can study the inside-outside coupling by using the interaction between the diffuse and discrete auroras observed near MLN. Han (2019) analyzed such an event observed on November 27, 2008, as shown in Figure 2.
FIGURE 2. A typical case observed on Nov. 27, 2008 for showing how the diffuse auroras can be used to analyze the dayside magnetospheric processes. Yellow and red arrows indicate Type 1 and Type 2 diffuse auroras, respectively. Type 2 diffuse aurora is believed to be related to the penetrated magnetosheath particles. A throat aurora is developed from the Type2 diffuse aurora from 08:22:30UT. At the same time, a black region is appeared. From 08:23:30, the Type 1 diffuse aurora drift poleward but cannot come into the black region, which means the field lines threading the black region is opened.
In Figure 2, a yellow arrow on each image approximately indicates the poleward edge of Type 1 diffuse aurora, and a red arrow indicates the Type 2 diffuse aurora. From 08:22:30UT, the Type 2 diffuse aurora split, and a black region appeared, as indicated by the blue arrow. At the same time, a discrete aurora started to appear in the center of the diffuse aurora, as indicated by the white arrow. This discrete aurora is called throat aurora (Han et al., 2015) and has been suggested to be associated with magnetopause reconnection (Han et al., 2019; Feng et al., 2020). With the throat aurora gradually brightened, a large area of Type 1 diffuse aurora (indicated by yellow arrow) just drifted poleward and surrounded the throat aurora from 08:23:30UT to 08:24:30UT. Most interestingly, the black region surrounding the throat aurora kept dark all the time, i.e., the large area of Type 1 diffuse drifting poleward but cannot enter into the black region. Because diffuse auroras are caused by particles on the closed field lines, this observational fact means that the electrons on the closed field lines cannot reach the black region anyway. This can be explained by that the black region was on opened field lines at that time. Because the black region was initially covered by diffuse aurora, i.e., on the closed field lines, Han [2019] suggested that the black region’s field lines are newly opened by magnetopause reconnection. Further, because the appearance of the black region is closely related to the throat aurora, this indicates that the generation of throat aurora is associated with the magnetopause reconnection. This example well demonstrates the inside-outside magnetospheric coupling by taking advantage of the two-dimensional continuous observations of aurora. The auroral observations may also be used to study how the cold plasmas affect the magnetopause reconnection (Borovsky and Denton, 2008; Borovsky et al., 2008).
Based on theoretical works of inferring cold plasma structuring from the structured diffuse auroras, observational results about the dayside diffuse aurora are well explained. Among the dayside diffuse aurora structures, the north-south aligned stripy diffuse auroras are the most informative. The convection-aligned feature reminds us to consider that the related cold plasma structure should be wedge-like in the magnetosphere and these cold plasmas are most likely from the ionospheric outflow. These outflowed cold plasmas may involve the dayside magnetopause reconnection, although the details need further study. Near magnetic local noon, two types of structured diffuse auroras were resolved. Type 1 is suggested to be related to ionospheric outflow, while Type 2 is believed to be involved in particles penetrated from the magnetosheath. Based on these understandings and combined with the advantages of two-dimensional continuous auroral observation, we can infer some physical processes near the dayside magnetopause.
The author confirms being the sole contributor of this work and has approved it for publication.
This work is supported by the National Natural Science Foundation of China (No. 42030101) and Shanghai Science and Technology Innovation Action Plan (No.21DZ1206102). D-SH is the PI of these fundings.
The author declares that the research was conducted in the absence of any commercial or financial relationships that could be construed as a potential conflict of interest.
All claims expressed in this article are solely those of the authors and do not necessarily represent those of their affiliated organizations, or those of the publisher, the editors, and the reviewers. Any product that may be evaluated in this article, or claim that may be made by its manufacturer, is not guaranteed or endorsed by the publisher.
Borovsky, J. E., and Denton, M. H. (2008). A Statistical Look at Plasmaspheric Drainage Plumes. J. Geophys. Res. 113 (A9). doi:10.1029/2007ja012994
Borovsky, J. E., Hesse, M., Birn, J., and Kuznetsova, M. M. (2008). What Determines the Reconnection Rate at the Dayside Magnetosphere? J. Geophys. Res. 113 (A7). doi:10.1029/2007ja012645
Brice, N., and Lucas, C. (2012). Influence of Magnetospheric Convection and Polar Wind on Loss of Electrons from the Outer Radiation belt. J. Geophys. Res. 76 (4), 900–908. doi:10.1029/ja076i004p00900
Chen, S. H., and Moore, T. E. (2006). Magnetospheric Convection and thermal Ions in the Dayside Outer Magnetosphere. J. Geophys. Res. 111 (A3). doi:10.1029/2005ja011084
Delzanno, G. L., Borovsky, J. E., Henderson, M. G., Resendiz Lira, P. A., Roytershteyn, V., and Welling, D. T. (2021). The Impact of Cold Electrons and Cold Ions in Magnetospheric Physics. J. Atmos. Solar-Terrestrial Phys. 220, 105599. doi:10.1016/j.jastp.2021.105599
Demekhov, A. G., and Yu, T. V. (1994). A Mechanism of Formation of Pulsating Aurorae. J. Geophys. Res. 99 (99), 5831–5841. doi:10.1029/93ja01804
Ebihara, Y., Tanaka, Y. M., Takasaki, S., Weatherwax, A. T., and Taguchi, M. (2007). Quasi-stationary Auroral Patches Observed at the South Pole Station. J. Geophys. Res. 112 (A1), A01201. doi:10.1029/2006ja012087
Feng, H.‐T., Han, D.‐S., Chen, X.‐C., Liu, J.‐J., and Xu, Z.‐H. (2020). Interhemispheric Conjugacy of Concurrent Onset and Poleward Traveling Geomagnetic Responses for Throat aurora Observed under Quiet Solar Wind Conditions. J. Geophys. Res. Space Phys. 125, e2020JA027995. doi:10.1029/2020JA027995
Foster, J. C., Coster, A. J., Erickson, P. J., Holt, J. M., Lind, F. D., Rideout, W., et al. (2005). Multiradar Observations of the Polar Tongue of Ionization. J. Geophys. Res. Space Phys. 110 (A9), A09S31-01–A09S31-12. doi:10.1029/2004ja010928
Frey, H. U., Han, D., Kataoka, R., Lessard, M. R., Milan, S. E., Nishimura, Y., et al. (2019). Dayside Aurora. Space Sci. Rev. 215 (8), 51. doi:10.1007/s11214-019-0617-7
Fuselier, S. A., and Lewis, W. S. (2011). Properties of Near-Earth Magnetic Reconnection from In-Situ Observations. Space Sci. Rev. 160 (1-4), 95–121. doi:10.1007/978-1-4614-3046-9_6
Han, D.-S., Xu, T., Jin, Y., Oksavik, K., Cai Chen, X., Liu, J-J., et al. (2019). Observational Evidence for Throat aurora Being Associated with Magnetopause Reconnection. Geophys. Res. Lett. 46, 7. doi:10.1029/2019GL083593
Han, D., Chen, X.-C., Liu, J.-J., Qiu, Q., Keika, K., Hu, Z.-J., et al. (2015). An Extensive Survey of Dayside Diffuse aurora Based on Optical Observations at Yellow River Station. J. Geophys. Res. Space Phys. 120 (9), 7447–7465. doi:10.1002/2015ja021699
Han, D. (2019). Ionospheric Polarization Electric Field Guiding Magnetopause Reconnection: A Conceptual Model of Throat aurora. Sci. China Earth Sci. 62 (12), 2099–2105. doi:10.1007/s11430-019-9358-8
Han, D. S., Li, J.-X., Nishimura, Y., Lyons, L. R., Bortnik, J., Zhou, M., et al. (2017). Coordinated Observations of Two Types of Diffuse Auroras Near Magnetic Local Noon by Magnetospheric Multiscale mission and Ground All-Sky Camera. Geophys. Res. Lett. 44 (16), 8130–8139. doi:10.1002/2017gl074447
Horne, R. B., and Thorne, R. M. (2000). Electron Pitch Angle Diffusion by Electrostatic Electron Cyclotron Harmonic Waves: The Origin of Pancake Distributions. J. Geophys. Res. Atmospheres 105 (A3), 5391–5402. doi:10.1029/1999ja900447
Horne, R. B., Thorne, R. M., Meredith, N. P., and Anderson, R. R. (2003). Diffuse Auroral Electron Scattering by Electron Cyclotron Harmonic and Whistler Mode Waves during an Isolated Substorm. J. Geophys. Res. Space Phys. 108 (A7). doi:10.1029/2002ja009736
Hu, Z. J., Yang, H., Huang, D., Araki, T., Sato, N., Taguchi, M., et al. (2009). Synoptic Distribution of Dayside aurora: Multiple-Wavelength All-Sky Observation at Yellow River Station in Ny-Ålesund, Svalbard. J. Atmos. Solar-Terrestrial Phys. 71 (8), 794–804. doi:10.1016/j.jastp.2009.02.010
Lee, S. H., Zhang, H., Zong, Q. G., Wang, Y., Otto, A., Rème, H., et al. (2015). Asymmetric Ionospheric Outflow Observed at the Dayside Magnetopause. J. Geophys. Res. Space Phys. 120 (5), 3564–3573. doi:10.1002/2014ja020943
Lemaire, J. (1977). Impulsive Penetration of Filamentary Plasma Elements into the Magnetospheres of the Earth and Jupiter. Planet. Space Sci. 25 (9), 887–890. doi:10.1016/0032-0633(77)90042-3
Li, W., Bortnik, J., Thorne, R. M., Nishimura, Y., Angelopoulos, V., and Chen, L. (2011). Modulation of Whistler Mode Chorus Waves: 2. Role of Density Variations. J. Geophys. Res. Atmospheres 116 (A6), 2235–2239. doi:10.1029/2010ja016313
Liang, J., Ni, B., Spanswick, E., Kubyshkina, M., Donovan, E. F., Uritsky, V. M., et al. (2011). Fast Earthward Flows, Electron Cyclotron Harmonic Waves, and Diffuse Auroras: Conjunctive Observations and a Synthesized Scenario. J. Geophys. Res. 116 (A12), A12220. doi:10.1029/2011ja017094
Lockwood, M. (1997). Relationship of Dayside Auroral Precipitations to the Open-Closed Separatrix and the Pattern of Convective Flow. J. Geophys. Res. 102 (A8), 17475–17487.
Lou, Y., Cao, X., Ni, B., Tu, W., Gu, X., Fu, S., et al. (2021). Diffuse Auroral Electron Scattering by Electrostatic Electron Cyclotron Harmonic Waves in the Dayside Magnetosphere. Geophys. Res. Lett. 48 (5), e2020GL092208. doi:10.1029/2020GL092208
Lui, A. T. Y., Perreault, P., Akasofu, S. I., and Anger, C. D. (1973). The Diffuse aurora. Planet. Space Sci. 21 (5), 857. doi:10.1016/0032-0633(73)90102-5
Meng, C. I., Mauk, B., and Mcilwain, C. E. (1979). Electron Precipitation of Evening Diffuse aurora and its Conjugate Electron Fluxes Near the Magnetospheric Equator. J. Geophys. Res. Space Phys. 84 (A6), 2545–2558. doi:10.1029/ja084ia06p02545
Newell, P. T., and Meng, C. I. (1992). Mapping the Dayside Ionosphere to the Magnetosphere According to Particle Precipitation Characteristics. Geophys. Res. Lett. 19 (6), 609–612. doi:10.1029/92gl00404
Newell, P. T., Ruohoniemi, J. M., and Meng, C.-I. (2004). Maps of Precipitation by Source Region, Binned by IMF, with Inertial Convection Streamlines. J. Geophys. Res. 109 (A10). doi:10.1029/2004ja010499
Newell, P. T., Sotirelis, T., and Wing, S. (2009). Diffuse, Monoenergetic, and Broadband aurora: The Global Precipitation Budget. J. Geophys. Res. 114 (A9). doi:10.1029/2009ja014326
Ni, B., Bortnik, J., Nishimura, Y., Thorne, R. M., Li, W., Angelopoulos, V., et al. (2014). Chorus Wave Scattering Responsible for the Earth's Dayside Diffuse Auroral Precipitation: A Detailed Case Study. J. Geophys. Res. Space Phys. 119 (2), 897–908. doi:10.1002/2013ja019507
Ni, B., Thorne, R. M., Horne, R. B., Meredith, N. P., Shprits, Y. Y., Chen, L., et al. (2011a). Resonant Scattering of Plasma Sheet Electrons Leading to Diffuse Auroral Precipitation: 1. Evaluation for Electrostatic Electron Cyclotron Harmonic Waves. J. Geophys. Res. 116 (A4). doi:10.1029/2010ja016232
Ni, B., Thorne, R. M., Meredith, N. P., Horne, R. B., and Shprits, Y. Y. (2011b). Resonant Scattering of Plasma Sheet Electrons Leading to Diffuse Auroral Precipitation: 2. Evaluation for Whistler Mode Chorus Waves. J. Geophys. Res. 116 (A4), A04219. doi:10.1029/2010ja016233
Nishimura, Y., Bortnik, J., Li, W., Thorne, R. M., Lyons, L. R., Angelopoulos, V., et al. (2010). Identifying the Driver of Pulsating aurora. Science 330 (6000), 81–84. doi:10.1126/science.1193186
Nishimura, Y., Bortnik, J., Li, W., Thorne, R. M., Ni, B., Lyons, L. R., et al. (2013). Structures of Dayside Whistler-Mode Waves Deduced from Conjugate Diffuse aurora. J. Geophys. Res. Space Phys. 118 (2), 664–673. doi:10.1029/2012ja018242
Sandholt, P. E., Farrugia, C. J., and Cowley, S. W. H. (1998). Pulsating Cusp aurora for Northward Interplanetary Magnetic Field. J. Geophys. Res. 103 (A11), 26507. doi:10.1029/98ja02433
Song, P., Holzer, T. E., Russell, C. T., and Wang, Z. (1994). Modelling the Low-Latitude Boundary Layer with Reconnection Entry. Geophys. Res. Lett. 21 (7), 625–628. doi:10.1029/94GL00374
Thorne, R. M., Ni, B., Tao, X., Horne, R. B., and Meredith, N. P. (2010). Scattering by Chorus Waves as the Dominant Cause of Diffuse Auroral Precipitation. Nature 467 (7318), 943–946. doi:10.1038/nature09467
Zhang, Q.-H., Zong, Q-G., Lockwood, M., Heelis, R. A., Hairston, M., Liang, J., et al. (2016). Earth’s Ion Upflow Associated with Polar Cap Patches: Global and In Situ Observations. Geophys. Res. Lett. 43 (5), 1845–1853. doi:10.1002/2016gl067897
Zhang, Q. H., Zhang, B. C., Moen, J., Lockwood, M., McCrea, I. W., Yang, H. G., et al. (2013). Polar Cap Patch Segmentation of the Tongue of Ionization in the Morning Convection Cell. Geophys. Res. Lett. 40 (12), 2918–2922. doi:10.1002/grl.50616
Keywords: diffuse aurora, dayside aurora, cusp aurora, cold plasma, throat aurora
Citation: Han D-S (2021) Dayside Diffuse Aurora and the Cold-Plasma Structuring: A Brief Review. Front. Astron. Space Sci. 8:725677. doi: 10.3389/fspas.2021.725677
Received: 15 June 2021; Accepted: 10 September 2021;
Published: 22 September 2021.
Edited by:
Gian Luca Delzanno, Los Alamos National Laboratory (DOE), United StatesReviewed by:
Toshi Nishimura, Boston University, United StatesCopyright © 2021 Han. This is an open-access article distributed under the terms of the Creative Commons Attribution License (CC BY). The use, distribution or reproduction in other forums is permitted, provided the original author(s) and the copyright owner(s) are credited and that the original publication in this journal is cited, in accordance with accepted academic practice. No use, distribution or reproduction is permitted which does not comply with these terms.
*Correspondence: De-Sheng Han, aGFuZGVzaGVuZ0B0b25namkuZWR1LmNu
Disclaimer: All claims expressed in this article are solely those of the authors and do not necessarily represent those of their affiliated organizations, or those of the publisher, the editors and the reviewers. Any product that may be evaluated in this article or claim that may be made by its manufacturer is not guaranteed or endorsed by the publisher.
Research integrity at Frontiers
Learn more about the work of our research integrity team to safeguard the quality of each article we publish.