- 1Laboratoire de Physique des Plasmas (LPP), CNRS, École Polytechnique, Institut Polytechnique de Paris, Observatoire de Paris, Sorbonne Université, Université Paris Saclay, Palaiseau, France
- 2CNRS, UPS, CNES, Institut de Recherche en Astrophysique et Planétologie (IRAP), Université de Toulouse, Toulouse, France
- 3INAF, Institute for space astrophysics and planetology (IAPS), Rome, Italy
- 4European Space Agency, ESTEC, Noordwijk, Netherlands
- 5Japan Aerospace Exploration Agency, Institute of Space and Astronautical Science, Sagamihara, Japan
- 6European Space Agency (ESA), European Space Astronomy Centre (ESAC), Madrid, Spain
- 7Wigner Research Centre for Physics, Budapest, Hungary
- 8Dipartimento di Fisica “E. Fermi”, Universitá di Pisa, Pisa, Italy
- 9Swedish Institute of Space Physics (IRF), Uppsala, Sweden
- 10Johns Hopkins University Applied Physics Laboratory, Laurel, MD, United States
- 11Blackett Laboratory, Imperial College, London, United Kingdom
- 12Institute for Space-Earth Environment Research, Nagoya University, Nagoya, Japan
- 13CNRS, IAS, Université Paris-Saclay, Gif-sur-Yvette, France
- 14Department of Physics, University of Helsinki, Helsinki, Finland
- 15CNRS, Laboratoire d’astrophysique de Bordeaux, University Bordeaux, Pessac, France
- 16Département d’Astrophysique/AIM, CEA/IRFU, CNRS/INSU, University Paris-Saclay, University de Paris, Gif-sur-Yvette, France
- 17Department of Climate and Space Sciences and Engineering, University of Michigan, Ann Arbor, MI, United States
- 18School of Physics and Astronomy, University of Leicester, Leicester, United Kingdom
- 19National Institute of Information and Communications Technology, Koganei, Japan
- 20Department of Physics and Astronomy, University of Turku, Turku, Finland
The investigation of multi-spacecraft coordinated observations during the cruise phase of BepiColombo (ESA/JAXA) are reported, with a particular emphasis on the recently launched missions, Solar Orbiter (ESA/NASA) and Parker Solar Probe (NASA). Despite some payload constraints, many instruments onboard BepiColombo are operating during its cruise phase simultaneously covering a wide range of heliocentric distances (0.28 AU–0.5 AU). Hence, the various spacecraft configurations and the combined in-situ and remote sensing measurements from the different spacecraft, offer unique opportunities for BepiColombo to be part of these unprecedented multipoint synergistic observations and for potential scientific studies in the inner heliosphere, even before its orbit insertion around Mercury in December 2025. The main goal of this report is to present the coordinated observation opportunities during the cruise phase of BepiColombo (excluding the planetary flybys). We summarize the identified science topics, the operational instruments, the method we have used to identify the windows of opportunity and discuss the planning of joint observations in the future.
1 Introduction
A new era of coordinated observations in the inner heliosphere has begun with the launch of NASA’s Parker Solar Probe (PSP, Fox et al., 2016) in August 2018, followed by the ESA-JAXA BepiColombo spacecraft mission in October 2018 (Benkhoff et al., 2021), and by ESA-NASA’s Solar Orbiter (Müller et al., 2020) in February 2020. Until the end of the cruise phase of BepiColombo (November 30, 2025), these three spacecraft will be traveling simultaneously in the inner heliosphere covering different heliocentric distances (Velli et al., 2020): BepiColombo’s elliptical orbit will cover distances from about 1.2 Astronomical Unit (AU) to ∼ 0.31 AU, while Solar Orbiter from ∼ 1.02 to 0.28 AU, and Parker Solar Probe from about 0.7 to 0.04 AU. Together with these missions, previous (and future) spacecraft provide continuous in situ and remote sensing measurements in our Solar System (as shown in Figure 1): at Venus ∼ 0.7 AU (Akatsuki, also known as the Venus Climate Orbiter), around Earth, including the L1 Lagrange point ∼ 1 AU (e.g., SOHO, ACE, Wind, Cluster, THEMIS, and MMS), at Mars ∼ 1.5 AU (e.g., MAVEN, Mars Express, Mars Odyssey), and at Jupiter ∼ 5 AU (e.g., JUNO). In addition many Sun-observing including, SOHO, Hinode, Solar Dynamics Observatory, PROBA-2, and STEREO-A monitor the Sun with various instrumentations. The exceptional and complementary in-situ and remote sensing payloads on-board these different satellites, together with ground based measurements, will give the opportunity to perform unprecedented multi-point measurements of the solar wind plasma and the Sun. Hence allowing the community to address different fundamental physical processes in the solar wind, such as turbulence, the generation, acceleration, and transport of solar energetic particles (SEPs), and the characterization of large scale heliospheric structures such as Interplanetary Coronal Mass Ejections (ICMEs).
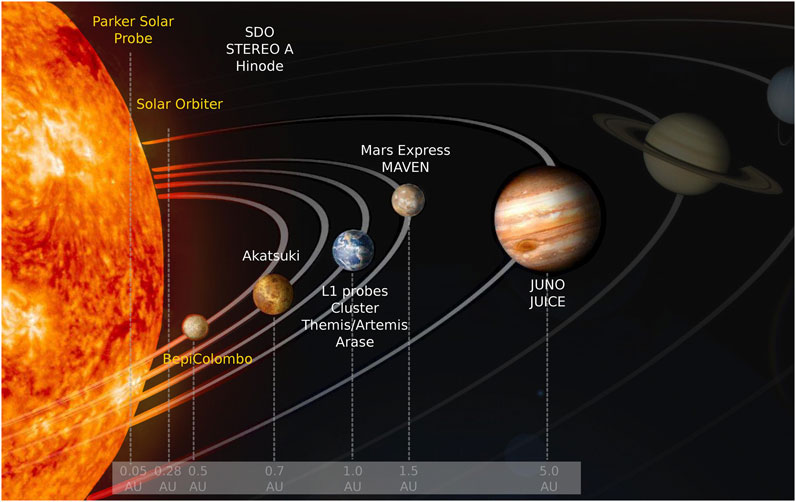
FIGURE 1. Examples of operational missions covering different heliocentric distances in our Solar System.
During the cruise phase, the BepiColombo spacecraft is in a so-called “stacked” configuration: the Mercury Magnetospheric Orbiter (MMO/Mio) is inside the Sunshield and Interface Structure (MOSIF) which is attached to the Mercury Planetary Orbiter (MPO). MPO, MOSIF, and Mio are all connected to the Mercury Transfer Module (MTM) (Mangano et al., 2021). Therefore, unlike some MPO instruments that are fully operational during cruise, all the instruments onboard Mio are shielded by MOSIF and not all of them can be scientifically operational (i.e., limited field of view, undeployed instruments). It is only after entering Mercury’s orbit in December 2025, that all the instruments will be fully deployed and operational at full capacity. Nevertheless, despite some operational and instrumental constraints, science operations could be performed during cruise in coordination with other spacecraft missions. Moreover, since long term planning is required for at least BepiColombo and Solar Orbiter (9 months and 6 months in advance respectively), a close coordination between BepiColombo, Solar Orbiter, and PSP teams was necessary (Velli et al., 2020). In this context, the BepiColombo Coordinated Observations Working Group (BC-CoObs WG) was initiated in November 2019. The BC-CoObs WG consisted of the project scientist and deputy project scientist of BepiColombo, along with instruments Principal Investigators (PIs) and members from BepiColombo, Solar Orbiter and PSP teams and researchers from the planetary and heliophysics community. The main goal of the BC-CoObs WG was to identify the science opportunities during the cruise phase of BepiColombo from September 1, 2020 till November 30, 2025, excluding the planetary flybys. In order to achieve this goal, we have defined three main tasks: 1) to specify the different science topics that are related to the multi-spacecraft observations, 2) to report the potential operational instruments on-board BepiColombo, and 3) to identify the potential windows of opportunities of BepiColombo in coordination with all the operational spacecraft, by selecting the most interesting spacecraft configurations.
In the following sections, we summarize the operational instruments on board BepiColombo, the science topics that can be addressed during cruise, and some operation constraints. Then, we describe the method and the tool that we used to identify the different windows of opportunity. Finally, we discuss the planning of the joint observations and present some specific examples.
2 Operational Instruments, Science Topics, and Constraints
The only official instrument onboard BepiColombo that was originally planned to be operating during cruise was the Mercury Orbiter Radio science Experiment (MORE, Iess et al., 2021) during superior solar conjunctions. Nevertheless, the beginning of the cruise phase has revealed that other instruments on board Mio and MPO could be operating as well and exploited for scientific studies. In fact, some of the instruments on-board MPO will be continuously operating during cruise, except for the Electric Propulsion (EP) periods, such as the BepiColombo Radiation Monitor (BERM), the Magnetometer (MPO-MAG, Glassmeier et al., 2010; Heyner et al., 2021), the Mercury Gamma-ray and Neutron Spectrometer (MGNS, Mitrofanov et al., 2010), the Italian Spring Accelerometer (ISA, Iafolla et al., 2021) and MORE. In table 1, we summarize all the operational instruments during the cruise phase, excluding the planetary flybys periods [more details regarding the planetary flybys periods and the pointing of the different instruments can be found in Mangano et al. (2021)]. A detailed description about the combined in situ and remote sensing instruments on-board Solar Orbiter and Parker Solar Probe can be found in Velli et al. (2020) and Müller et al. (2020).
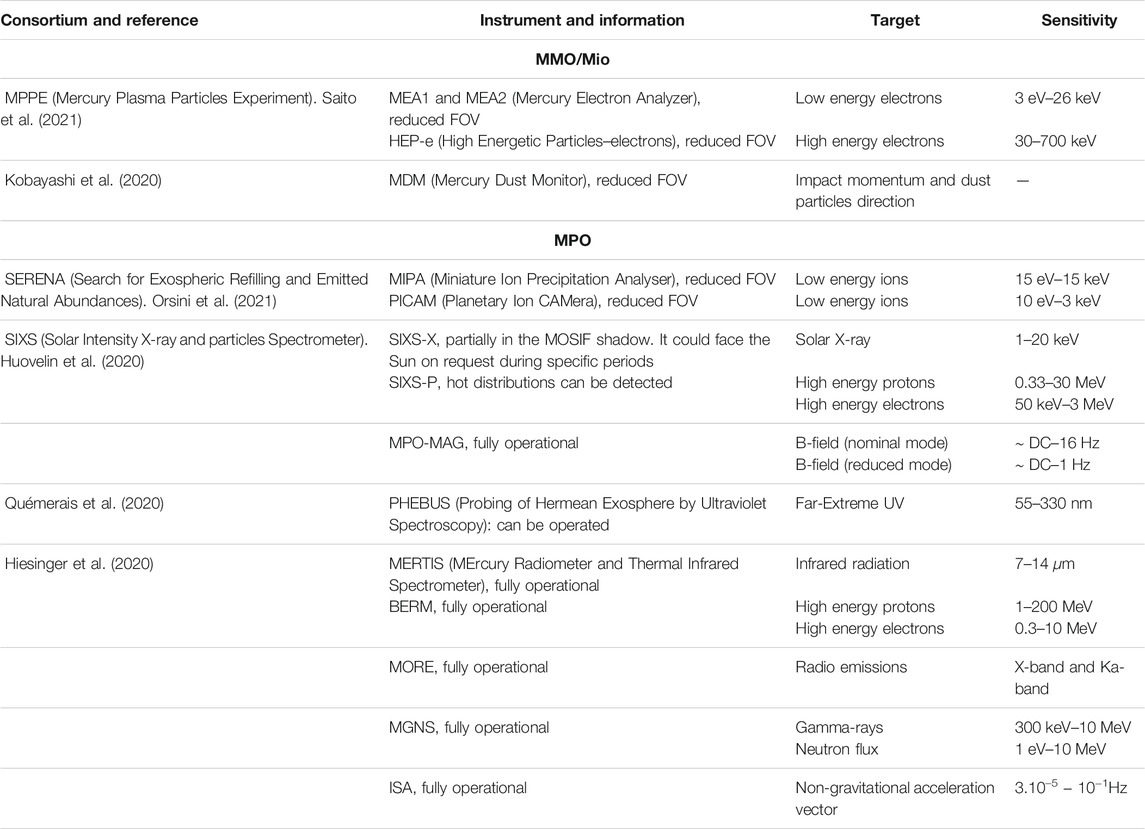
TABLE 1. Operational instruments during the cruise phase (excluding the periods of the planetary flybys).
Despite the limited science operations, interesting science studies can be performed. During the cruise phase of BepiColombo, at least from September 2020 until December 2025, the Sun will be approaching Solar maximum (predicted in July 2025). The joint measurements with BepiColombo, Solar Orbiter, and Parker Solar Probe including other operational spacecraft in the interplanetary space, will allow a better understanding of the evolution of the solar wind plasma and its large scale three-dimensional distribution under different conditions of the solar activity using both remote sening and in-situ meaurements. An extensive discussion on the science investigations during the cruise phase of BepiColombo can be found in Mangano et al. (2021). Briefly, the main science topics can be grouped into seven cases: 1) Solar wind turbulence properties (e.g., Tu and Marsch, 1995; Bruno and Carbone, 2013; Sahraoui et al., 2020). They include studies of the scaling properties (e.g., Alberti et al., 2020), characterization of the wave modes propagation (e.g., Howes et al., 2012), high order statistics, and intermittency (e.g., Bruno et al., 2001), and their evolution with heliocentric distances. 2) Solar wind structures. These include the study of the properties and radial evolution of small scale structures, such as discontinuities, including reconnection exhausts (e.g., Gosling et al., 2005), magnetic holes (e.g., Turner et al., 1977; Volwerk et al., 2020; Karlsson et al., 2021), interplanetary shocks and of large scale heliospheric structures, such as transient events [e.g., ICMEs, flux ropes (Kilpua et al., 2017)], and the Co-rotating Interaction Regions (CIRs, Richardson, 2018) which are compressive regions formed in the background magnetic field where high-speed wind runs into the slower wind ahead (e.g. Pizzo, 1980; Gosling and Pizzo, 1999). Moreover, the large scale properties of the solar wind (electron number density and velocity) could be done by combining ground-based interplanetary scintillation observations (IPS) observations (e.g., Iwai et al., 2019), heliospheric imaging (e.g., Harrison et al., 2018) and global magnetohydrodynamic (MHD) simulations and models such as SUSANOO (e.g., Shiota et al., 2014), IPS-ENLIL (e.g., Odstrcil, 2003; Jackson et al., 2015), or EUHFORIA (e.g., Pomoell and Poedts, 2018). The solar wind plasma parameters measured onboard one of the spacecraft can also be propagated with the help of a simple 1D MHD model such as Heliopropa (http://heliopropa.irap.omp.eu) to another planet, comet, or spacecraft in order to provide a virtual solar wind monitor and predict the properties of the solar wind in another heliospheric location. 3) Solar Energetic Particles (SEPs). The study of their generation, acceleration and transport processes and their flux-time profiles at different locations in the solar wind (e.g., Miroshnichenko, 2018). Moreover, combining in situ SEP data with type-II radio burst data, and global MHD simulations may provide information regarding the structure of the background interplanetary magnetic fields and the propagation of ICMEs. 4) Dust and Comets. The characterization of the dust distribution in the inner Solar System (e.g., Grün et al., 1980) and the analysis of the cometary composition such as the hydrogen coma at Lyman-α in Extreme Ultraviolet Violet (EUV) (e.g., Bertaux et al., 1995). Even though this depends on the passage of the comets in the field of view of BepiColombo’s EUV spectrometer (PHEBUS), at least one observation of comet 2P/Encke would be possible by the end of November 2023 as it arrives at a distance of ∼ 0.6 AU from BepiColombo. 5) Solar Corona. Measurements of the density fluctuations of the solar corona down to a few solar radii (e.g., Miyamoto et al., 2014) will be possible using radio measurements from the Mercury Orbiter Radio-science Experiment (MORE). 6) High frequency electromagnetic radiation. These include the detection of Gamma-Ray Bursts (GRBs) and their localization, in particular the gamma-rays that originate from solar flares, and monitoring the local radiation background of the spacecraft due to bombardment by energetic particles of Galactic Cosmic Rays. Last but not least, 7) General relativity test could be performed during superior solar conjunctions (e.g., Bertotti et al., 1993; Iess et al., 2021).
Two major constraints had to be taken into account for the planning of the joint observations with BepiColombo. The first one is related to the Electric Propulsion periods during which no instruments operations can be performed, and the second one is related to the science downlink rate, as BepiColombo moves away from Earth. As one can see from Figure 2, in periods with a distance between BepiColombo and Earth larger than 1.2 AU the data downlink rate gets very low (< 0.3 kbps), not even allowing the instruments’ background operations. Therefore, we assume that all in-situ instruments can operate in background mode when the distance Bepi-Earth is less than 0.7 AU (data downlink rate > 6 kbps).
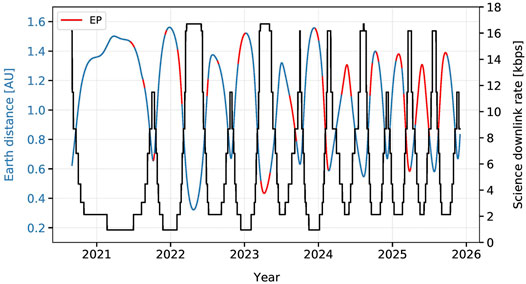
FIGURE 2. Distance of BepiColombo from Earth in AU (left axis, in blue) and the science downlink rate (right axis, in black) as a function of time during cruise. The Electric Propulsion (EP) periods are highlighted in red lines.
3 Methods
In order to identify the potential windows of opportunities, we have focused our investigation on five different spacecraft geometries and used the Centre de Données de la Physique des Plasmas (CDPP) tools, in particular the Automated Multi-Dataset Analysis (AMDA) tool. In the following subsections, we give a short overview of AMDA and describe the different spacecraft configurations.
3.1 AMDA Tool
AMDA is an online database and analysis tool (http://amda.cdpp.eu/) developed for 15 years by the CDPP (http://www.cdpp.eu/). It gathers a large variety of plasma data from space missions and ground instruments together with simulations and models. It also offers a dedicated work space to all registered users where plot layouts, data mining conditions, and event lists can be stored and reused. Hundreds of user accounts have been provided over the years from space physics students to senior researchers. Several papers describe the basics of AMDA and its various applications in heliophysics and planetary sciences (Génot et al., 2021, and references herein). Registration is done by sending an mail to amda@irap.omp.eu, and a public access (with no workspace) is also available. For the planning activities of the BC-CoObs Working Group, the choice of using AMDA arose for several reasons. First, the tool gives access to the ephemeris of all considered objects (spacecraft and planets) in several common coordinate systems and measurements at several cadences. Second, it enables to create new parameters from existing data, to perform conditional search on a large volume of data-set, and finally, to manage resulting event lists, and so, to produce catalogues which are relevant to this study.
3.2 Spacecraft Configurations
The investigation of the different windows of opportunity was done based on five different geometries, “Cone,” “Opposition,” “Parker,” “Cone-Parker,” and “Quadrature” and by considering the following spacecraft/bodies: BepiColombo, PSP, Solar Orbiter, STEREO-A, Venus, Earth, Mars, and Jupiter. Each of these configurations are defined below in the Heliocentric Earth Ecliptic (HEE) system, where the X axis points towards the Earth and the Z axis is perpendicular to the plane of the Earth’s orbit around the Sun (positive North). This system is fixed with respect to the Earth-Sun line.
• Cone: radial alignments between two or more spacecraft/bodies, i.e., when they are within a small longitude cone from the Sun. For the radial alignment we require here the maximum cone width of 10 ± 3 in longitude without putting any constraints on the latitude assuming that it’s sufficient to identify all the events that are radially aligned within a cone (Figure 3B). The “Cone” geometry allows mainly to study the in situ evolution of the same plasma parcel and the underlying processes as a function of the distance from the Sun, such as the properties of the solar wind plasma, and turbulence as it has been already done using Helios 1 and 2 probes (Schwartz and Marsch, 1983), Ulysses and ACE (D’Amicis et al., 2010) and more recently using PSP and Solar Orbiter (Telloni et al., 2021), as well as the evolution of large-scale heliospheric structures (e.g., Gosling and Pizzo, 1999).
• Opposition: two or more spacecraft/bodies are oppositely aligned forming a cone with respect to the Sun. The difference in longitude (in absolute values) between the spacecraft is defined as 180 ± 10. This geometry is particularly interesting for periods of superior solar conjunctions, during which simultaneous radio occultation observations can be performed to observe remotely the solar corona using both remote sensing measurements and ground based observations.
• Parker: magnetic alignment between two or more bodies, i.e., when the footpoints of Parker field lines passing through the bodies are in a small latitude/longitude region at the so-called “source surface” (Rs), which is an imaginary spherical surface above the corona. The alignment along the same spiral field line would allow to study the evolution of the solar wind plasma that originate from the same source at different locations on the field line (with a time difference that corresponds to the travel time of the plasma that would change over this time). Moreover, it would allow to analyze unpredictable bursty events (e.g., SEPs) that originate from the same “active” region on the solar surface.
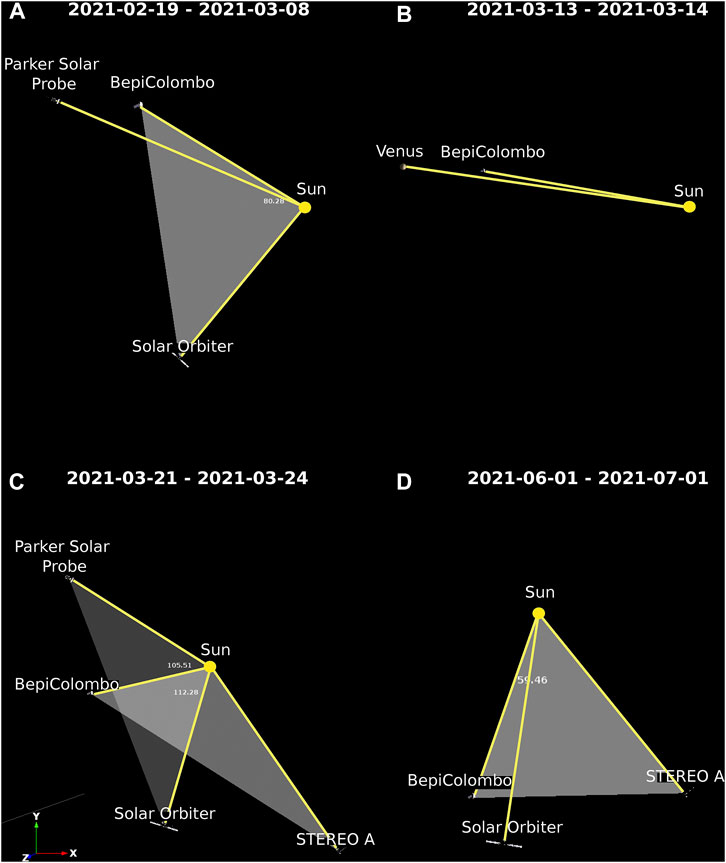
FIGURE 3. 3DView illustration of the downselected configurations for the first half of 2021, in the HEE coordinate system: (A) 2021-02-19–2021-03-08: Parker Solar Probe and BepiColombo are in a cone geometry and in Quadrature geometry with Solar Orbiter. (B) 2021-03-13–2021-03-14: BepiColombo and Venus are in a cone geometry. (C) 2021-03-21–2021-03-24: Parker Solar Probe is in quadrature geometry with Solar Orbiter and BepiColombo is in quadrature geometry with STEREO A. (D) 2021-06-01–2021-07-01: BepiColombo and Solar Orbiter are in cone geometry and in quadrature geometry with STEREO A.
For this configuration, the longitude of the Parker field line at the source surface (ϕs) has first to be computed for a given Solar Wind velocity uSW; the formula is given below by:
where ϕ(r) is the spacecraft heliocentric longitude at distance r, Ω = 2π/25.38 days, and Rs = 2.5RSun. Several values of uSW were used in the range 200–800 km/s, to take into account the slow (200–400 km/s), intermediate (400–500 km/s) and fast solar wind (600–800 km/s). At the source surface a small region of 3 × 3 in longitude/latitude is considered for the footpoints to be said co-located. Varying the size of this region impacts the duration of the magnetic conjunction. The footprint of a given spacecraft at a given time is defined by its longitude (ϕs) and by its latitude (equal to the one of the spacecraft as a Parker field line is inscribed on a cone of constant latitude). Below the source surface, the Parker approximation to model the magnetic field generally fails as the coronal magnetic field becomes more complex; refined models that take into account observed/modeled magnetograms (e.g., Potential-Field Source-Surface model Wang et al., 1992) can then be used. This is what is done, for instance, in the “Magnetic Connectivity” tool developed at IRAP1 to estimate the solar source location of the solar wind and energetic particles measured by different spacecraft. Nevertheless the first order approach explained above and used in AMDA by the working group is a quick way to derive times of interest for the coordinated analysis.
• Cone-Parker: the combination of the Cone and Parker geometries. This configuration is mainly interesting to study and analyze ICMEs, SEPs, or any other bursty event that propagates and expands at different longitude and radial distances.
• Quadrature: Spacecraft with remote sensing instruments (e.g., imagers, coronographs) forming an angle of 90 ± 10 with the Sun and other spacecraft/planet. For this particular geometry we only considered BepiColombo, Earth (L1 probes, e.g., Wind, SOHO) and the solar missions: PSP, Solar Orbiter, and STEREO-A. This configuration is optimal for observing remotely the structure of the Solar corona (Lamy et al., 2020) or any transient events that emanate from the Sun, and linking them to the heliosphere observed in-situ 90◦ from the Sun-spacecraft line. An example would be when Parker Solar Probe is in quadrature with Solar Orbiter. Close to the Sun, the remote sensing instruments onboard Solar Orbiter (such as the Solar Orbiter Heliospheric Imager using Thomson scattering) would be providing imagery of the plasma lifting up from the limb and so the solar wind plasma parcel that is, expected to be encountered by Parker Solar Probe further away from the Sun at 90◦ from the Sun-Solar Orbiter line (Howard et al., 2020). The quadrature geometry and the combination of the in situ and remote sensing measurement would allow to observe the global structure of the eruptive events as they evolve in the interplanetary space and contribute to the understanding of their initiation at the Sun.
In a recent study, Davies et al. (2021) could investigate in situ the propagation of an ICME through the interplanetary medium as Solar Orbiter, BepiColombo, and Wind spacecraft were radially aligned, and could observe its global shape and expansion using the remote observations from STEREO-A as it was in a quadrature geometry with respect to the Sun-Earth line.
A detailed description of AMDA’s data mining conditions can be found in the Bepi-CoObs WG AMDA manual (see data availability statement). It is worth noting that the travel time of the plasma from one spacecraft to another was not taken into account when selecting the different events and this should be taken into account when considering the radial alignment case studies.
4 Windows of Opportunity and Future Plannings
Using the AMDA tool and applying the method described in section 3, the investigation of the joint observations with BepiColombo by the CoObs WG, has led to 921 windows of opportunity between September 1st, 2020 and November 30th, 2025. We note that this large number of events is mainly due to the “Parker” geometry condition, that was applied assuming different ranges of the solar wind speed. The full list of events is publicly available on AMDA, and will be shared on BepiColombo’s Cruise Science study group ESA’s website (see data availability statement). The timetable includes an identifier (ID) for each case study, the starting and ending time of the observation window, the geometry, the name of the spacecraft or objects, whether the event coincides with any particular flyby of BepiColombo, Solar Orbiter or PSP, or to an EP period. Furthermore, for each of the considered objects (BepiColombo, PSP, Solar Orbiter, STEREO-A, Venus, Earth, Mars, and Jupiter) we added the minimum, maximum and average values of the latitude, longitude, and radial distance from the Sun. We note that movies of Solar Orbiter, BepiColombo, PSP and STEREO-A trajectories, and the planning of Solar Orbiter’s joint observations for July-December 2021 (and future plans) are available at Solar Orbiter’s Science Operation Center website (see data availability statement).
After identifying all the potential opportunities with the different conjunctions, a down-selection of the most feasible and realistic cases was necessary in order to plan and coordinate well in advance the joint observations, in particular between the two ESA missions, BepiColombo and Solar Orbiter teams. The down-selection is based on the following selection approach: 1) we assume that the BERM instrument is always operational in the background and not further analyzed, as its downlink rate is relatively low (38 bps); 2) the EP and instruments checkout periods are immediately excluded from any analysis; 3) all the in situ instruments are assumed to be operating in background mode when the distance BepiColombo-Earth is less than 0.7 AU. The only question to the teams during these periods would be related to the spacecraft pointing requirements; and 4) no events near the flyby’s periods (closest approach ± 1 week) are down-selected, as the flyby operations are discussed withing the flyby working group.
The down-selection step is implemented by a small group including the Project Scientists of the missions, who discusses 9 months in advance, a 6 months planning period for the coordinated observations between BepiColombo and Solar Orbiter - and any other non-ESA missions, taking into account the constraints as discussed above in the selection approach (Figure 2). The down-selected opportunities are then sent by the project scientists to the different instruments PIs of both missions, who analyze the different periods and specify for each of them a set of information (a priority level, a description of the observation, operational mode, pointing requests, and the expected science downlink rate and volume) that are then studied and validated by the ESA Mission Operation Center (MOC). Due to the spacecraft and payload constraints, only few windows of opportunities could be down-selected. In fact, for the planning of the year 2021 and the first half of 2022 (January 1st, 2022–June 30, 2022), only 17 events out of 349 could be requested to MOC. Examples of these joint observations, plotted using 3DView tool (Génot et al., 2018), are shown in Figure 3 and listed below:
• 2021-02-19–2021-03-08: BepiColombo is in a cone geometry with PSP and in quadrature geometry with Solar Orbiter.
• 2021-03-13–2021-03-14: BepiColombo and Akatsuki in solar conjunction.
• 2021-03-21–2021-03-24: PSP, BepiColombo, Solar Orbiter, and STEREO-A are longitudinally distributed around the Sun. PSP-Solar Orbiter and BepiColombo-STEREO-A are more or less in quadrature geometry.
• 2021-06-01–2021-06-12: BepiColombo is in a cone geometry with Solar Orbiter and in quadrature geometry with STEREO-A.
• 2021-07-01–2021-08-02: BepiColombo, Solar Orbiter and Mars are in Cone-Parker geometry. On 2021-07-15 BepiColombo is radially aligned with Solar Orbiter, and STEREO-A is in quadrature geometry.
• 2021-08-14–2021-08-26: BepiColombo and Solar Orbiter are magnetically aligned, and STEREO-A in quadrature geometry.
• 2021-09-05–2021-09-25: PSP, Solar Orbiter, and STEREO-A are in a Cone geometry and BepiColombo is nearby.
• 2021-10-07–2021-10-08: BepiColombo, Solar Orbiter, and STEREO-A are magnetically connected on the same parker spiral.
• 2021-10-14–2021-10-15: BepiColombo, Solar Orbiter, and STEREO-A are magnetically connected on the same parker spiral.
• 2021-10-23–2021-10-28: BepiColombo is radially aligned with Earth and Solar Orbiter is in a quadrature geometry.
• 2022-03-11–2022-03-31: BepiColombo is radially aligned with STEREO-A and Solar Orbiter is in a quadrature geometry.
• 2022-04-06–2022-04-18: BepiColombo is radially aligned with Earth.
• 2022-06-09–2022-06-10: BepiColombo is close to Mercury and radially aligned with PSP, and STEREO-A is in a quadrature geometry.
Conclusion
In this short report we attempt to summarize the extensive investigation that we have done within the BepiColombo CoObs WG to identify the potential scientific coordinated observations related to BepiColombo, Solar Orbiter and any other operational spacecraft mission. Despite some spacecraft and payload constraints, this work has revealed many interesting science opportunities for in-situ and remote sensing synergistic observations in the inner heliosphere even before the orbit insertion of BepiColombo around Mercury in December 2025. The joint in situ and remote sensing observations of BepiColombo, Solar Orbiter, Parker Solar Probe including other space, and ground based observatories, would allow detailed mapping of the inner heliosphere and to better understand the solar wind properties, structures and magnetic field expansion at different heliocentric distances. Recent studies of coordinated observations between BepiColombo, Solar Orbiter and Wind (Davies et al., 2021), and Parker Solar Probe and Solar Orbiter (Telloni et al., 2021) and additional on-going studies, highlight the need for careful advance planning to optimize the scientific return from this unprecedented opportunity of synergistic observations. At the time of writing this report, regular meetings for the planing of the future years are being held and the approved selected events will be made public to the community and will available on BepiColombo’s Cruise Science Study Group ESA cosmos website (see Data Availability Statement).
Data Availability Statement
The SPICE kernels are used for the different missions with the official data sources as given below. The kernels are regularly updated at the official sources and in orbitography services (including AMDA), for instance when reconstructed kernels are replacing predicted ones. As a consequence the catalogue of events as it is presented at the time of publication of the article may change. However, it has been checked that these possible changes (shift in start/stop times of some events) are limited, and, for example, on the same scale as the shift induced by varying the cone aperture of a few degrees (for “Cone” configuration), or the solar wind velocity by a few 10 km/s (for “Parker” configuration).
List of the SPICE kernels:
• BepiColombo: ESA/ESAC https://doi.org/10.5270/esa-dwuc9bs
• Solar Orbiter: ESA/ESAC https://doi.org/10.5270/esa-kt1577e
• Parker Solar Probe: https://sppgway.jhuapl.edu/lpredict_ephem and https://sppgway.jhuapl.edu/recon_ephem
• STEREO-A: https://sohowww.nascom.nasa.gov/solarsoft/stereo/gen/data/spice/depm/ahead/
• Planets: NASA/NAIF https://naif.jpl.nasa.gov/naif/
The websites of the different tools and movies are:
• CDPP: http://www.cdpp.eu/
• AMDA: http://amda.cdpp.eu/
• 3D View: http://3dview.irap.omp.eu/
• Magnetic Connectivity: http://connect-tool.irap.omp.eu/
• Heliopropa: http://heliopropa.irap.omp.eu
• Bepi-CoObs WG Amda manual
• Solar Orbiter’s Science Operation Center: https://issues.cosmos.esa.int/solarorbiterwiki/display/SOSP/Solar+Orbiter+SOC+Public
BepiColombo planning - the generated catalogue and the down-selected list of events are available in:
• AMDA: “Shared catalogue” directory http://amda.cdpp.eu/
• BepiColombo’s Cruise Science: https://www.cosmos.esa.int/web/bepicolombo-yssg-cs
Solar Orbiter planning - the future planning during the cruise phase of Solar Orbiter is available in:
• https://issues.cosmos.esa.int/solarorbiterwiki/display/SOSP/Cruise+Phase
Author Contributions
All the co-authors have contributed in discussing the science topics, operational instruments and geometries for identifying the potential coordinated observations. In addition, LH, VG, and SA have investigated the potential windows of opportunities using the AMDA tool.
Funding
The CDPP is supported by CNRS, CNES, Observatoire de Paris and Université Paul Sabatier Toulouse, and parts of the aforementioned tools are currently being developed through the Sun Planet Interactions Digital Environment on Request (SPIDER) Virtual Activity of the Europlanet H2024 Research Infrastucture funded by the European Union’s Horizon 2020 research and innovation programme under grant agreement No 871149. B.S.-C. acknowledges support through UK-STFC grants ST/S000429/1 and ST/V000209/1.
Conflict of Interest
The authors declare that the research was conducted in the absence of any commercial or financial relationships that could be construed as a potential conflict of interest.
The reviewer DH declared a past co-authorship with several of the authors SA, AM, JZ, JB, LG, TH, EK, and BS to the handling editor.
Publisher’s Note
All claims expressed in this article are solely those of the authors and do not necessarily represent those of their affiliated organizations, or those of the publisher, the editors and the reviewers. Any product that may be evaluated in this article, or claim that may be made by its manufacturer, is not guaranteed or endorsed by the publisher.
Acknowledgments
AMDA team thanks all active users who provided very useful feedback over the years and therefore contributed to enhance the quality of the system. French co-authors ackowledge the support of CNES for the BepiColombo, Solar Orbiter, and Parker Solar Probe missions. This analysis was performed in the frame of the ESA/JAXA working group: BepiColombo’s cruise phase coordinated observations.
Footnotes
1http://connect-tool.irap.omp.eu/References
Alberti, T., Laurenza, M., Consolini, G., Milillo, A., Marcucci, M. F., Carbone, V., et al. (2020). On the Scaling Properties of Magnetic-Field Fluctuations through the Inner Heliosphere. ApJ 902, 84. doi:10.3847/1538-4357/abb3d2
Benkhoff, J., Benkhoff, J., and Benkhoff, J. (2021). BepiColombo - Mission Overview and Science Goals in Space. Space Sci. Rev. 217.
Bertaux, J. L., Kyrölä, E., Quémerais, E., Pellinen, R., Lallement, R., Schmidt, W., et al. (1995). SWAN: A Study of Solar Wind Anisotropies on SOHO with Lyman Alpha Sky Mapping. The SOHO Mission, 403–439. Germany: Springer. doi:10.1007/978-94-009-0191-910.1007/978-94-009-0191-9_11
Bertotti, B., Comoretto, G., and Iess, L. (1993). Doppler Tracking of Spacecraft with Multi-Frequency Links. Astron. Astrophysics 269, 608–616.
Bruno, R., and Carbone, V. (2013). The Solar Wind as a Turbulence Laboratory. Living Rev. Solar Phys. 10. doi:10.12942/lrsp-2013-2
Bruno, R., Carbone, V., Veltri, P., Pietropaolo, E., and Bavassano, B. (2001). Identifying Intermittency Events in the Solar Wind. Planet. Space Sci. 49, 1201–1210. Nonlinear Dynamics and Fraactals in Space. doi:10.1016/S0032-0633(01)00061-7
D’Amicis, R., Bruno, R., Pallocchia, G., Bavassano, B., Telloni, D., Carbone, V., et al. (2010). Radial Evolution of Solar Wind Turbulence during Earth and Ulysses Alignement of 2007 August. Astrophysical J. 717, 474–480. doi:10.1088/0004-637x/717/1/474
Davies, E. E., Möstl, C., Owens, M. J., Weiss, A. J., Amerstorfer, T., Hinterreiter, J., et al. (2021). In Situ multi-spacecraft and Remote Imaging Observations of the First Cme Detected by Solar Orbiter and Bepicolombo. A&A. doi:10.1051/0004-6361/202040113
Fox, N. J., Velli, M. C., Bale, S. D., Decker, R., Driesman, A., Howard, R. A., et al. (2016). The Solar Probe Plus Mission: Humanity's First Visit to Our Star. Space Sci. Rev. 204, 7–48. doi:10.1007/s11214-015-0211-6
Génot, V., Beigbeder, L., Popescu, D., Dufourg, N., Gangloff, M., Bouchemit, M., et al. (2018). Science Data Visualization in Planetary and Heliospheric Contexts with 3DView. Planet. Space Sci. 150, 111–130. doi:10.1016/j.pss.2017.07.007
Génot, V., Budnik, E., Jacquey, C., Bouchemit, M., Renard, B., Dufourg, N., et al. (2021). Automated Multi-Dataset Analysis (Amda): an On-Line Database and Analysis Tool for Heliospheric and Planetary Plasma Data. Planet. Space Sci. 201, 105214. doi:10.1016/j.pss.2021.105214
Glassmeier, K.-H., Auster, H.-U., Heyner, D., Okrafka, K., Carr, C., Berghofer, G., et al. (2010). The Fluxgate Magnetometer of the Bepicolombo Mercury Planetary Orbiter. Planet. Space Sci. 58, 287–299. Comprehensive Science Investigations of Mercury: The scientific goals of the joint ESA/JAXA mission BepiColombo. doi:10.1016/j.pss.2008.06.018
Gosling, J. T., and Pizzo, V. J. (1999). Formation and Evolution of Corotating Interaction Regions and Their Three Dimensional Structure. Space Sci. Rev. 89, 21–52. doi:10.1007/978-94-017-1179-1_3
Gosling, J. T., Skoug, R. M., McComas, D. J., and Smith, C. W. (2005). Magnetic Disconnection from the Sun: Observations of a Reconnection Exhaust in the Solar Wind at the Heliospheric Current Sheet. Geophys. Res. Lett. 32. doi:10.1029/2005gl022406
Grün, E., Pailer, N., Fechtig, H., and Kissel, J. (1980). Orbital and Physical Characteristics of Micrometeoroids in the Inner Solar System as Observed by Helios 1. Planet. Space Sci. 28, 333–349. doi:10.1016/0032-0633(80)90022-7
Harrison, R. A., Davies, J. A., Barnes, D., Byrne, J. P., Perry, C. H., Bothmer, V., et al. (2018). Cmes in the Heliosphere: I. A Statistical Analysis of the Observational Properties of Cmes Detected in the Heliosphere from 2007 to 2017 by Stereo/hi-1. Sol. Phys. 293, 77. doi:10.1007/s11207-018-1297-2
Heyner, D., Auster, H.-U., Fornaçon, K.-H., Carr, C., Richter, I., Mieth, J. Z. D., et al. (2021). The Bepicolombo Planetary Magnetometer Mpo-Mag: What Can We Learn from the Hermean Magnetic Field?. Space Sci. Rev. 217, 52. doi:10.1007/s11214-021-00822-x
Hiesinger, H., Helbert, J., Helbert, J., Alemanno, G., Bauch, K. E., D’Amore, M., et al. (2020). Studying the Composition and Mineralogy of the Hermean Surface with the Mercury Radiometer and thermal Infrared Spectrometer (Mertis) for the Bepicolombo mission: An Update. Space Sci. Rev. 216, 110. doi:10.1007/s11214-020-00732-4
Howard, R. A., Vourlidas, A., Colaninno, R. C., Korendyke, C. M., Plunkett, S. P., Carter, M. T., et al. (2020). The Solar Orbiter Heliospheric Imager (Solohi). A&A 642, A13. doi:10.1051/0004-6361/201935202
Howes, G. G., Bale, S. D., Klein, K. G., Chen, C. H. K., Salem, C. S., and TenBarge, J. M. (2012). The Slow-Mode Nature of Compressible Wave Power in Solar Wind Turbulence. ApJ 753, L19. doi:10.1088/2041-8205/753/1/l19
Huovelin, J., Vainio, R., Kilpua, E., Lehtolainen, A., Korpela, S., Esko, E., et al. (2020). Solar Intensity X-ray and Particle Spectrometer Sixs: Instrument Design and First Results. Space Sci. Rev. 216, 94. doi:10.1007/s11214-020-00717-3
Iess, L., Asmar, S. W., Cappuccio, P., Cascioli, G., De Marchi, F., di Stefano, I., et al. (2021). Gravity, Geodesy and Fundamental Physics with BepiColombo's MORE Investigation. Space Sci. Rev. 217, 21. doi:10.1007/s11214-021-00800-3
Iwai, K., Shiota, D., Tokumaru, M., Fujiki, K. i., Den, M., and Kubo, Y. (2019). Development of a Coronal Mass Ejection Arrival Time Forecasting System Using Interplanetary Scintillation Observations. Earth Planets Space 71, 39. doi:10.1186/s40623-019-1019-510.1186/s40623-019-1019-5
Jackson, B. V., Odstrcil, D., Yu, H.-S., Hick, P. P., Buffington, A., Mejia-Ambriz, J. C., et al. (2015). The Ucsd Kinematic Ips Solar Wind Boundary and its Use in the Enlil 3-d Mhd Prediction Model. Space Weather 13, 104–115. doi:10.1002/2014SW001130
Karlsson, T., Heyner, D., Volwerk, M., Morooka, M., Plaschke, F., Goetz, C., et al. (2021). Magnetic Holes in the Solar Wind and Magnetosheath Near Mercury. J. Geophys. Res. Space Phys. 126. doi:10.1029/2020JA028961
Kilpua, E., Koskinen, H. E. J., and Pulkkinen, T. I. (2017). Coronal Mass Ejections and Their Sheath Regions in Interplanetary Space. Living Rev. Sol. Phys. 14, 5. doi:10.1007/s41116-017-0009-6
Kobayashi, M., Shibata, H., Nogami, K. i., Fujii, M., Hasegawa, S., Hirabayashi, M., et al. (2020). Mercury Dust Monitor (Mdm) Onboard the mio Orbiter of the Bepicolombo mission. Space Sci. Rev. 216, 144. doi:10.1007/s11214-020-00775-7
Lamy, P., Llebaria, A., Boclet, B., Gilardy, H., Burtin, M., and Floyd, O. (2020). Coronal Photopolarimetry with the LASCO-C2 Coronagraph over 24 Years [1996 - 2019]. Sol. Phys. 295, 89. doi:10.1007/s11207-020-01650-y
Mangano, V., Dósa, M., Fränz, M., Milillo, A., Oliveira, J. S., Lee, Y. J., et al. (2021). Bepicolombo Science Investigations during Cruise and Flybys at the Earth, Venus and Mercury. Space Sci. Rev. 217, 23. doi:10.1007/s11214-021-00797-9
Miroshnichenko, L. I. (2018). Retrospective Analysis of Gles and Estimates of Radiation Risks. J. Space Weather Space Clim. 8, A52. doi:10.1051/swsc/2018042
Mitrofanov, I. G., Kozyrev, A. S., Konovalov, A., Litvak, M. L., Malakhov, A. A., Mokrousov, M. I., et al. (2010). The Mercury Gamma and Neutron Spectrometer (Mgns) on Board the Planetary Orbiter of the Bepicolombo mission. Planet. Space Sci. 58, 116–124. Comprehensive Science Investigations of Mercury: The scientific goals of the joint ESA/JAXA mission BepiColombo. doi:10.1016/j.pss.2009.01.005
Miyamoto, M., Imamura, T., Tokumaru, M., Ando, H., Isobe, H., Asai, A., et al. (2014). Radial Distribution of Compressive Waves in the Solar Corona Revealed Byakatsukiradio Occultation Observations. ApJ 797, 51. doi:10.1088/0004-637x/797/1/51
Müller, D., St. Cyr, O. C. O. C., Zouganelis, I., Gilbert, H. R., Marsden, R., Nieves-Chinchilla, T., et al. (2020). The Solar Orbiter mission. A&A 642, A1. doi:10.1051/0004-6361/202038467
Odstrcil, D. (2003). Modeling 3-d Solar Wind Structure. Adv. Space Res. 32, 497–506. Heliosphere at Solar Maximum. doi:10.1016/S0273-1177(03)00332-6
Orsini, S., Livi, S. A., Lichtenegger, H., Barabash, S., Milillo, A., De Angelis, E., et al. (2021). Serena: Particle Instrument Suite for Determining the Sun-Mercury Interaction from Bepicolombo. Space Sci. Rev. 217, 11. doi:10.1007/s11214-020-00787-3
Pizzo, V. J. (1980). A Three-Dimensional Model of Corotating Streams in the Solar Wind 2. Hydrodynamic Streams. J. Geophys. Res. 85, 727–743. doi:10.1029/JA085iA02p00727
Pomoell, J., and Poedts, S. (2018). Euhforia: European Heliospheric Forecasting Information Asset. J. Space Weather Space Clim. 8, A35. doi:10.1051/swsc/2018020
Quémerais, E., Chaufray, J.-Y., Koutroumpa, D., Leblanc, F., Reberac, A., Lustrement, B., et al. (2020). Phebus on Bepi-Colombo: Post-launch Update and Instrument Performance. Space Sci. Rev. 216, 67. doi:10.1007/s11214-020-00695-6
Richardson, I. G. (2018). Solar Wind Stream Interaction Regions throughout the Heliosphere. Living Rev. Sol. Phys. 15, 1. doi:10.1007/s41116-017-0011-z
Sahraoui, F., Hadid, L., and Huang, S. (2020). Magnetohydrodynamic and Kinetic Scale Turbulence in the Near-Earth Space Plasmas: a (Short) Biased Review. Rev. Mod. Plasma Phys. 4, 4. doi:10.1007/s41614-020-0040-2
Saito, Y., Delcourt, D., Hirahara, M., Barabash, S., André, N., Takashima, T., et al. (2021). Pre-flight Calibration and Near-Earth Commissioning Results of the Mercury Plasma Particle Experiment (MPPE) Onboard MMO (Mio). Space Sci. Rev. 217 (5), 70. doi:10.1007/s11214-021-00839-2
Schwartz, S. J., and Marsch, E. (1983). The Radial Evolution of a Single Solar Wind Plasma Parcel. J. Geophys. Res. 88, 9919–9932. doi:10.1029/JA088iA12p09919
Shiota, D., Kataoka, R., Miyoshi, Y., Hara, T., Tao, C., Masunaga, K., et al. (2014). Inner Heliosphere Mhd Modeling System Applicable to Space Weather Forecasting for the Other Planets. Space Weather 12, 187–204. doi:10.1002/2013sw000989
Telloni, D., Sorriso-Valvo, L., Woodham, L. D., Panasenco, O., Velli, M., Carbone, F., et al. (2021). Evolution of Solar Wind Turbulence from 0.1 to 1 au during the First Parker Solar Probe-Solar Orbiter Radial Alignment. ApJL 912, L21. doi:10.3847/2041-8213/abf7d1
Tu, C.-Y., and Marsch, E. (1995). MHD Structures, Waves and Turbulence in the Solar Wind: Observations and Theories. Space Sci. Rev. 73. 1-210. doi:10.1007/bf00748891
Turner, J. M., Burlaga, L. F., Ness, N. F., and Lemaire, J. F. (1977). Magnetic Holes in the Solar Wind. J. Geophys. Res. 82, 1921–1924. doi:10.1029/JA082i013p01921 19771896.
Velli, M., Harra, L. K., Vourlidas, A., Schwadron, N., Panasenco, O., Liewer, P. C., et al. (2020). Understanding the Origins of the Heliosphere: Integrating Observations and Measurements from parker Solar Probe, Solar Orbiter, and Other Space- and Ground-Based Observatories. A&A 642, A4. doi:10.1051/0004-6361/202038245
Volwerk, M., Goetz, C., Plaschke, F., Karlsson, T., Heyner, D., and Anderson, B. (2020). On the Magnetic Characteristics of Magnetic Holes in the Solar Wind between Mercury and Venus. Ann. Geophys. 38, 51–60. doi:10.5194/angeo-38-51-2020
Wang, Y.-M., and Sheeley, N. R. (1992). On Potential Field Models of the Solar Corona. ApJ 392, 310. doi:10.1086/171430
Keywords: solar wind, multi-spacecraft measurements, inner heliosphere, spacecraft mission, coordinated measurements
Citation: Hadid LZ, Génot V, Aizawa S, Milillo A, Zender J, Murakami G, Benkhoff J, Zouganelis I, Alberti T, André N, Bebesi Z, Califano F, Dimmock AP, Dosa M, Escoubet CP, Griton L, Ho GC, Horbury TS, Iwai K, Janvier M, Kilpua E, Lavraud B, Madar A, Miyoshi Y, Müller D, Pinto RF, Rouillard AP, Raines JM, Raouafi N, Sahraoui F, Sánchez-Cano B, Shiota D, Vainio R and Walsh A (2021) BepiColombo’s Cruise Phase: Unique Opportunity for Synergistic Observations. Front. Astron. Space Sci. 8:718024. doi: 10.3389/fspas.2021.718024
Received: 31 May 2021; Accepted: 30 August 2021;
Published: 14 September 2021.
Edited by:
Rudolf A. Treumann, Ludwig Maximilian University of Munich, GermanyReviewed by:
Daniel Heyner, Technische Universitat Braunschweig, GermanyAlexander Kozyrev, Space Research Institute (RAS), Russia
Copyright © 2021 Hadid, Génot, Aizawa, Milillo, Zender, Murakami, Benkhoff, Zouganelis, Alberti, André, Bebesi, Califano, Dimmock, Dosa, Escoubet, Griton, Ho, Horbury, Iwai, Janvier, Kilpua, Lavraud, Madar, Miyoshi, Müller, Pinto, Rouillard, Raines, Raouafi, Sahraoui, Sánchez-Cano, Shiota, Vainio and Walsh. This is an open-access article distributed under the terms of the Creative Commons Attribution License (CC BY). The use, distribution or reproduction in other forums is permitted, provided the original author(s) and the copyright owner(s) are credited and that the original publication in this journal is cited, in accordance with accepted academic practice. No use, distribution or reproduction is permitted which does not comply with these terms.
*Correspondence: L. Z. Hadid, lina.hadid@lpp.polytechnique.fr