- 1Dipartimento di Fisica e Geologia, Università degli Studi di Perugia, Perugia, Italy
- 2INFN, Sezione di Perugia, via A. Pascoli snc, Perugia, Italy
- 3INAF, Observatorio d'Abruzzo, via Mentore Maggini snc, Teramo, Italy
- 4INFN LNS, via Santa Sofia 62, Catania, Italy
- 5Dipartimento di Fisica e Astronomia, Università degli Studi di Catania, Catania, Italy
- 6Gran Sasso Science Institute, viale Francesco Crispi, L’Aquila, Italy
Among presolar grains, oxide ones are made of oxygen, aluminum, and a small fraction of magnesium, produced by the 26Al decay. The largest part of presolar oxide grains belong to the so-called group 1 and 2, which have been suggested to form in Red Giant Branch (RGB) and Asymptotic Giant Branch (AGB) stars, respectively. However, standard stellar nucleosynthesis models cannot account for the 17O/16O, 18O/16O, and 26Al/27Al values recorded in those grains. Hence, for more than 20 years, the occurrence of mixing phenomena coupled with stellar nucleosynthesis have been suggested to account for this peculiar isotopic mix. Nowadays, models of massive AGB stars experiencing Hot Bottom Burning or low mass AGB stars where Cool Bottom Process, or another kind of extra-mixing, is at play, nicely fit the oxygen isotopic mix of group 2 oxide grains. The largest values of the 26Al/27Al ratio seem somewhat more difficult to account for.
Introduction
Dust is an alternative probe, with respect to direct astronomical observations, to explore stars and their nucleosynthesis. In particular, presolar grains are tiny refractory particles formed in the envelopes of evolved stars; they come to us as inclusions in pristine meteorites, once spread in the interstellar medium by stellar winds. Even if they were involved in the formation of the Solar System, presolar grains preserved the chemical and isotopic composition of the stellar environments where they condensed. They can therefore provide information about the nucleosynthesis of the progenitor stars with an accuracy often higher than direct stellar observations.
The evolutionary phase of the progenitor star is easily recognizable for some types of presolar grains. For example, this is the case of the MainStream SiC grains in which the presence of s-elements is the fingerprint of their origin in Asymptotic Giant Branch (AGB) stars. Instead, oxide grains do not carry any exotic trace hinting to the type of star in which they were formed. Being essentially made only of oxygen and aluminum, oxide grains are classified into four groups according to their oxygen isotopic compositions, as shown in Figure 1 (Nittler et al., 1997; Zinner, 2014).
• Grains with 17O/16O ≥ 3.82·10−4 (solar value) and 10−3 ≤18O/16O ≤ 2.01·10−3 (solar value) belong to group 1 and have oxygen isotopic ratios similar to those observed in Red Giant Branch (RGB) stars (Harris and Lambert, 1984; Abia et al., 2012), and they have therefore been suggested as progenitors of those grains.
• Grains belonging to group 2 show 17O/16O larger than the solar value and large 18O depletions (18O/17O ≤ 0.001). Such isotopic mix cannot be reproduced by standard stellar nucleosynthesis; for this reason, several authors have suggested that mixing mechanisms coupled with H-burning nucleosynthesis of low mass red giant stars can account for this grain composition. We will discuss this point in detail in this paper.
• Group 3 grains, with both 17O/16O and 18O/16O ratios smaller than solar ones, might come from low mass stars with lower-than-solar metallicity. The oxygen isotopic ratios of group 3 grains are supposed to reflect the evolution of the oxygen isotope abundances in the Galaxy. They have actually been used to obtain an estimate of the age of the Milky Way (Nittler, 2009).
• Group 4 grains show excesses of both 17O/16O and 18O/16O ratios, and they likely form in supernovae when 18O-rich material from the He/C zone can be admixed to material from oxygen-rich zones (Choi et al., 1998). However, up to now, only three oxide grains with evidence of SN origins (very rich in 16O) have been identified (Hynes and Gyngard, 2009). It has been suggested that such a paucity of SN grains is related to the fact that oxide grains from supernovae are smaller than those from red giant stars and, as such, are difficult to spot in meteorite samples (Zinner, 2014).
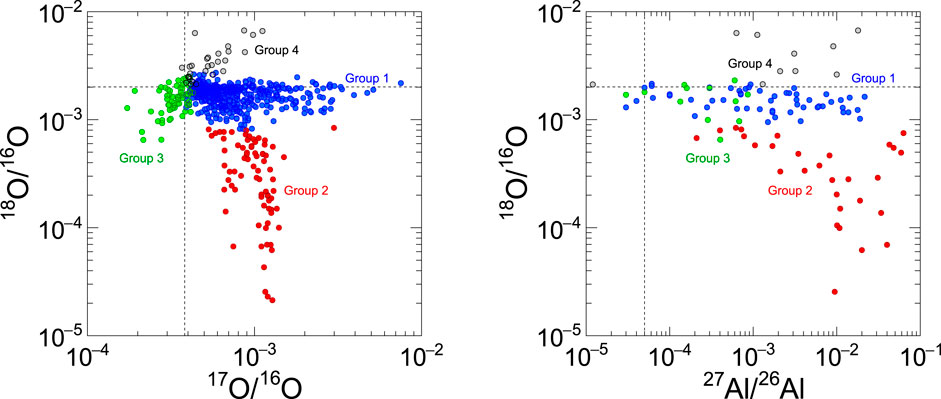
FIGURE 1. Left panel: oxygen isotopic ratios in presolar oxide grains. Different colors identify grains belonging to different groups as defined by Nittler et al. (1997) and indicated by the labels. Right panel:18O/16O and 26Al/27Al isotopic ratios in presolar oxide grains. Group 2 grains generally show significant 18O/16O depletion and higher values of 26Al/27Al even if there is no simple correlation between the two isotopic ratios. Grain data in this figure as well as in all the figures of this paper are from the Presolar Grain database of the Laboratory for Space Sciences at the Washington University in Saint Louis (Hynes and Gyngard, 2009). Dashed lines mark solar isotopic values.
Notably, some oxide grains show traces of 26Mg, hinting at the initial presence of 26Al (t1/2 = 7.16·105 years, see the right panel of Figure 1). This aspect is important to identify the grain stellar progenitor, but it is also puzzling. Indeed, excluding massive stars (the main contributors to galactic 26Al), grains with 26Al must have formed in AGB stars, as their hydrogen-burning shells are efficient 26Al producers. However, isotopic abundances of oxygen and aluminum in grains have values that can be found in the hydrogen burning shell when the temperature is higher than some 107 K (e.g., to achieve 26Al/27Al ratios ≥3·10−3, the H-shell has to burn at T ≥ 4·107 K): how could material so rich in 26Al be present in the envelopes of evolved stars with mass smaller than 2 M⊙?
The stellar structure of an AGB star is made of a CO unburning core surrounded by a He- and a H-rich shell. The latter burns radiatively below the convective envelope for most of the evolutionary phase, supplying energy to the star. The H-burning is periodically interrupted by convective episodes due to the activation of the He-burning shell, called thermal pulses. The abundances of CNO isotopes, and light nuclei in general, in the envelope of Red Giant Stars (namely the candidate progenitor of group 1 and 2 grains) are set by the first dredge-up1 (hereafter FDU). A second dredge-up (SDU) occurs in the very early AGB phase in objects with masses larger than ∼ 4 M⊙ (Boothroyd and Sackmann, 1999). Furthermore, at the exhaustion of each thermal pulse, the convective envelope penetrates down to the He-rich layers and enriches the stellar surface with the products of the He-burning and the s-process2 nucleosynthesis. This mixing episode is called third dredge-up (hereafter TDU), and, unlike the other two, it occurs numerous times during the AGB phase.
Firstly, Wasserburg et al. (1995) suggested the existence of a nonstandard mixing mechanism (namely, other than FDU, SDU, and TDU) coupled with AGB star nucleosynthesis to explain the oxygen isotopic abundances found in oxide grains (in particular of those that a few years later were identified by Nittler et al., 1997 as belonging to the group 1 and 2). A C/O ratio smaller than 1, together with 17O/16O larger than the solar value, 18O/16O much lower than the solar reference, and 26Al/27A suitable to account for abundances recorded in group 2 oxide grains can be obtained in the envelopes of AGB stars in two possible scenarios: objects with initial mass smaller than 1.5–1.7 M⊙ affected by cool bottom process (CBP) or more massive stars (M > 4 M⊙) in which hot bottom burning (HBB) is at play.
CBP is a non-convective mixing phenomenon operated by conveyor belts, which, from the cool bottom of the convective envelope, drag material toward the inner region of the star and, once they reach the area near the hydrogen burning shell, they collect the fresh nucleosynthesis products and drag those ashes upwards, pouring them into the stellar envelope. It turns out that the surface stellar abundances are modified and assume values similar (if not equal) to those of the H-burning regions. CBP and similar mixing phenomena (also called extra-mixing or non-convective mixing), as well as their possible physical causes, have been investigated by several authors (e.g., Nollett et al., 2003; Eggleton et al., 2006; Palacios et al., 2006; Denissenkov et al., 2009; Busso et al., 2010; Palmerini et al., 2011a). In particular, Nollett et al. (2003), Busso et al. (2010) and Palmerini et al. (2011a) have shown that extra-mixing is able to reproduce the oxygen isotopic mix in group 1 and group 2 oxide grains, eventually preventing stars from becoming carbon stars. However, the higher 26Al/27A ratios recorded in some group 2 grains (up to a few 10–2) can be accounted for by just a few extra-mixing models, such as that proposed by Palmerini et al. (2017). In this scenario, the advection into the envelope of magnetic bubbles, formed just above the H-burning shell, allows for effective enrichment of 26Al due to a bottom-up mixing mechanism. This mixing is different from the classic CBP, because the relatively fast up-flow of the magnetic bubbles triggers a diffusive down flow of material (Trippella et al., 2016; Vescovi et al., 2020), hence there is not a real circulation nor the transported matter has time to experience further low temperature proton captures. As it is, instead, in the semianalytic CBP calculation model presented by Nollett et al., 2003, which can account for the highest 26Al/27Al values found in “extreme oxide grains” if the mass circulation reaches the most energetic layers of the H-burning shell; in this case, however, the CBP feedback on the stellar energy balance is not negligible (Palmerini et al., 2011a).
To reproduce the oxygen and aluminum isotopic ratios recorded in group 2 grains, an extra-mixing mechanism has to efficiently pauperize in 18O the stellar envelope as well as efficiently enrich it in 26Al. The mixed material must therefore experience temperatures higher than 4−5·107 K. This fact rules out the thermohaline mixing (for details see e.g., Eggleton et al., 2008; Lagarde et al., 2012; Lattanzio et al., 2015) as being responsible for the changes in the envelope composition necessary to reproduce the composition of those grains. It is actually not deep enough because the average molecular weight inversion, which is due to the burning of the 3He(3He,2p)4He reaction and triggers this diffusive mixing, occurs in relatively superficial layers of the H-shell where the temperature never exceeds 3−3.5·107 K (Busso et al., 2010; Palmerini et al., 2011b).
An alternative explanation comes from HBB in more massive (M > 4 M⊙) AGB stars (Iben, 1975; Boothroyd and Sackmann, 1992; D’Antona and Mazzitelli, 1996; Ventura and D’Antona, 2005). In this case, the temperature at the base of the convective envelope is high enough to allow the local burning of some proton capture reactions. As a matter of fact, HBB efficiently modifies the distribution of light isotopes, enhancing the abundances of some of them (13C, 14N, 26Al) and efficiently destroying others (12C, 18O). In massive AGBs (in particular at metallicities lower than solar), two competing mechanisms are at work: TDU (which carries carbon to the surface) and HBB (which destroys it). Presolar SiC grains (formed in C-rich environments) may thus grow depending on which of the two above-mentioned mechanisms is dominating. Iliadis et al. (2008) suggested that, in the light of a new measurement of the 16O(p,γ)17F reaction rate, 16O could not be destroyed, even partially, by red giant stars (also of intermediate mass), and group 2 oxide grains therefore have to form in low-mass AGB stars. The situation took a different turn when Lugaro et al. (2017) showed that massive AGB star models are able to reproduce the oxygen isotopic composition of group 2 grains if the 17O(p,α)14N reaction rate measured by Bruno et al. (2016) is used. However, in this case, grains with 26Al/27Al > 0.06 cannot be reproduced by those models. In this paper, we review the possibility of reproducing the isotopic abundances of oxygen and aluminum recorded in group 2 grains with low and intermediate mass AGB models by adopting the 17O+p reaction rates proposed by different authors.
To provide the reader with a better comparison of the discussed mixing models, we report in Figure 2 a scheme of the three mentioned mechanisms: the conveyor belt CBP, the magnetic induce extra-mixing, and the HBB. Before analyzing in detail their effects on the oxygen and aluminum isotopic mix in the envelopes of AGB stars, in the next section, we discuss how reaction rates affect the oxygen isotope nucleosynthesis during H-burning.
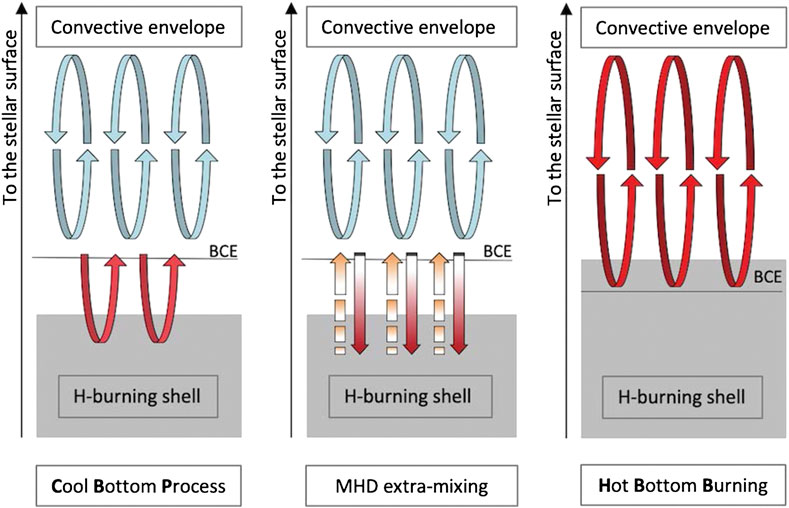
FIGURE 2. Scheme of the mixing phenomena that coupled with the stellar nucleosynthesis might account for the oxygen and aluminum isotopic ratios recorded in group 2 oxide grains. Left panel: the classic CBP operated by a conveyor-belt-like mechanism that starts from the bottom of the convective envelope (BCE, as thought and presented by Wasserburg et al., 1995; Nollett et al., 2003). Central panel: the magnetic induced extra-mixing, the stellar magnetic fields induce a bottom-up mixing triggered by the up-flow of “bubbles” according to Nucci and Busso (2014); Trippella et al. (2016) and Palmerini et al. (2017). Right panel: the HBB that takes place when the temperature above the BCE is high enough to allow the further occurrence of some proton capture reactions. The gray areas in the three panels show the stellar region where temperatures are sufficiently high to burn H. Figures are not to scale.
Sensitivity of 17O/16O Ratio to the H-Burning Temperature and the Nuclear Reaction Rates
The nucleosynthesis of 17O and 26Al is so sensitive to the temperature that their abundances can be used as a thermometer for the H-burning of the stellar layers where they form. In the case of 26Al, the higher the temperature the larger is the abundance. This is valid also for the 26Al/27Al isotopic ratio, being the proton capture on 27Al less efficient than that on 26Al. On the other side, the situation is a bit more complicate for 17O. Indeed, the 16O is efficiently consumed by the 16O(p,γ)17F reaction only in the inner and hotter layers of the H-burning shell, while the 17O+p reaction rate has not a linear or monotonic dependence with temperature. It turns out that in the temperature range typical of H-burning in red giants, the equilibrium value of the 17O/16O as a function of the temperature has the trend shown in Figure 3. Such a value is uniquely determined by the proton capture reactions. From this competition, the temporal evolution of the 17O abundance is described by the equation
and then in equilibrium condition
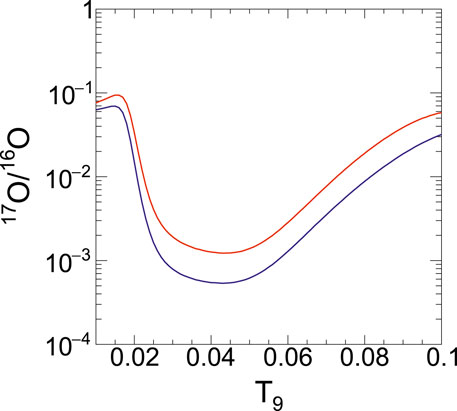
FIGURE 3. 17O/16O equilibrium values as a function of temperature computed by using reaction rates of set A (red line) and set B (blue line). See Table 1 and the text for more details. Only results obtained for recommended values of the reaction rates are shown because nucleosynthesis calculations have been run employing only recommended values.
From Eq. 2, it is clear that if the reaction rates are well known, the isotopic ratio 17O/16O can reveal the temperature of the stellar envelope where the nucleosynthesis took place as well as the maximum temperature experienced by the materials reversed into the stellar envelope by convective or non-convective mixing phenomena. In this way, the 17O abundance can be a valuable probe to investigate stars and their interiors. For example, Abia et al. (2012) have suggested an estimation of the stellar mass of α−Boo and α−Tau, with a precision of 1/10 of the solar mass, on the basis of the 17O/16O surface abundances observed for the two red giants. However, this scenario is affected by a very strong criticality: each new measurement of a nuclear reaction rate might lead to significant changes in the nucleosynthesis predictions and their agreement, or disagreement, with the observational constraints. This is exactly the case of the puzzling isotopic composition of the presolar oxide grains, which has been gradually understood over the years as new measures have allowed us to increase the accuracy of nuclear physics inputs in stellar nucleosynthesis models. An extended discussion on the state of the art of measurements and uncertainty estimations on the 17O + p reaction rate is presented in review paper by Sergi et al. (2020).
As mentioned in the previous section, the envelope abundances of oxygen isotopes of red giant stars are set by the FDU (or in a few cases the SDU) and they will remain unchanged until CBP or HBB takes place. The 17O/16O ratio with which a star approaches the AGB phase is thus determined by the H-burning temperature in its interior and the stellar mass. Firstly, Boothroyd et al. (1994) and Wasserburg et al. (1995) pointed out that just the narrow range of 10–3 ≤ 17O/16O ≤ 2.2 10–3 was accessible to stars with mass smaller than 2 M⊙, while more massive objects (up to 7 M⊙) were suggested to be the progenitor of grains showing larger values of 17O/16O. In any case, CBP (in low mass stars) and HBB (in more massive ones) were needed to reduce the 18O/16O isotopic ratio from values around 0.0015 (the average value set by FDU and SDU) down to a few 10–4, as recorded in the first presolar grains analyzed. Moreover, in the 18O/16O vs. 17O/16O plane, a forbidden region was found at 17O/16O < 0.0005 and 18O/16O < 0.0015. This portion of the plane, even if occupied by several oxide grains, was not accessible by HBB nor CBP models.
A few years later, Nollett et al. (2003), in examining the effects of CBP on the C, N, O, and Al isotopic ratios in AGB star nucleosynthesis, demonstrated that oxide grain isotopic ratios could be accounted for by the conveyor belt mixing model. This is so because 17O can produced or destroyed according to the mixing depth, the 18O depleted, and the 26Al efficiently synthesized. However, the region accessible to low-mass star nucleosynthesis remained limited to 5. 10–4 ≤ 17O/16O ≤ 2.2. 10–3.
The situation was improved by a new measurement of the bottleneck reaction of the CNO cycle: the 14N(p,γ)15O. Indeed, the reaction rate was found to be the 50% smaller and this requires the CNO burning to take place at higher densities and temperatures (25% and the 10%, respectively) in the H-shell during the RGB phase as well as inside the stellar core during the main sequence (see Palmerini et al., 2011a, for an extended discussion). This induces small but effective changes not only in the nucleosynthesis but also in the stellar structure and, as a consequence, the surface 17O/16O abundance of a 2 M⊙ star after the FDU moves from 2·10−3 to 5.14·10−3 and the whole range of the 17O/16O values recorded in group 1 oxide grains is covered by RGB stars with mass ≤2 M⊙. Palmerini et al., 2011a have shown that in stars with mass smaller than 2.2 M⊙ CBP can be at play also during the RGB phase, and, if so, it might account for subsolar values of the 18O/16O isotopic ratio recorded in group 1 grains. The mixing model in the quoted paper is a parametric one and the authors assume CBP during the RGB phase to have both the mixing rate and the mixing depth smaller than during the AGB phase. However, even assuming the same CBP parameters assumed for the AGB phase, the temperature of the H-burning shell of a low mass RGB star is not high enough to allow a18O/16O depletion below 7·104, which is the upper limit of the isotopic ratio recorded in group 2 grains. For the same reason (low temperature) also the 26Al enrichment due to CBP in the RGB phase is limited to 26Al/27Al ≤ 10–4. The temperatures of the H-burning during the AGB phase are higher than in the previous RGB phase, and, as a consequence, CBP or a similar extra-mixing phenomenon at play during the AGB phase in a star with mass ≤1.5 M⊙ leads the surface abundance of 17O and 18O to the values typical of group 2 grains. Finally, updates of the proton capture cross sections on 17O and 18O (La Cognata et al., 2010; Palmerini et al., 2013) allowed the nucleosynthesis models to cross the forbidden region.
In 2013, after the cited updates in the nuclear physics data, the scenario was roughly the same as shown by the blue curves of Figure 4 (panel A). In the light of the agreement between models and oxygen isotopic mix of grains, it would be possible to state that group 1 dust might form in RGB stars with mass smaller than 2 M⊙ and that group 2 grains form in AGB of 1.2–1.5 M⊙ being an extra-mixing mechanism at play. All the stars being of solar metallicity. Despite this nice agreement between observational constraints and theory for oxygen isotopes, there was a problem: the 26Al/27Al values larger than a few 10–3 could not be reached by extra-mixing models. This was a severe limit for the extra-mixing models because the composition of group 2 grains, and especially those rich in 26Al/27Al, necessarily requires relatively high temperatures to be reproduced. However, mixing mechanisms cannot push the mixed materials beyond a certain depth of H-burning shell to avoid a luminosity feedback due to extra energy provided by the burning of the mixed material.
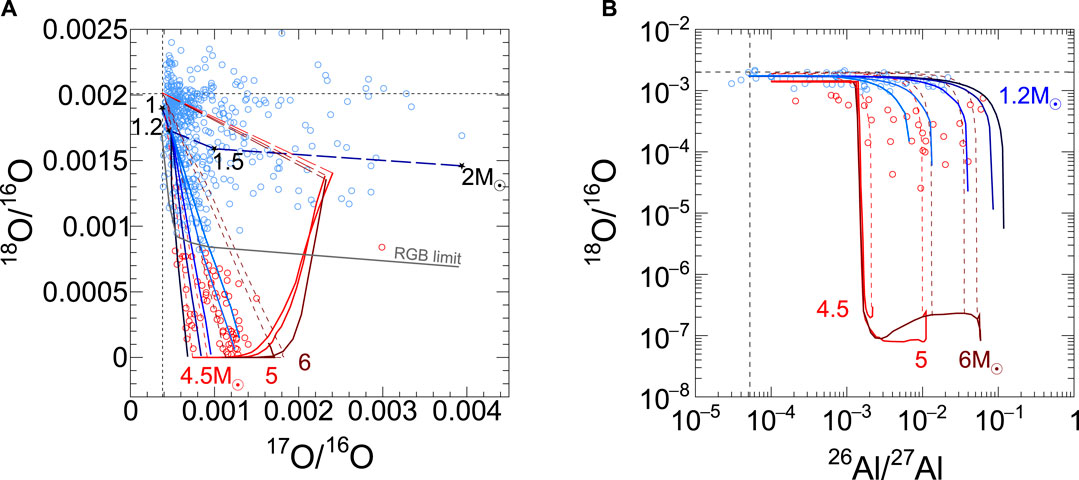
FIGURE 4. Panel(A). Evolution of the 17O/16O and 18O/16O isotopic ratios in the envelope of low mass and intermediate mass AGB stars of solar metallicity due to magnetic mixing (blu curves) and HBB (red curves). Star markers along the blue dashed line indicate the post-FDU composition of stars with mass from 1 to 2 M⊙ as indicated by the labels. Open blue circles are group 1 oxide grains while red ones are those of group 2. The descending blue lines refer to magnetic mixing model results for a 1.2 M⊙ AGB star for different values of k, computed employing reaction rates from set A (see the text for details). The maximum modification of the envelope composition that can be produced by the mixing during the RGB phase is indicated by the gray curve. Red curves deal with the evolution of 17O/16O and 18O/16O isotopic ratio in the envelope of a 4.5, a 5, and a 6 M⊙ star affected by HBB, as reported by Lugaro et al. (2017), which runs calculations with reaction rates of set B. The long dashed red lines indicate the evolution of the oxygen isotopic ratio in the stellar evolution before the AGB phase, while the short dashed red curves show effects of diluting the stellar wind with early solar system materials (see the text for details). The two gray dashed lines mark the 17O/16O and 18O/16O solar values. Panel(B). Same models and grain data of plot in panel(A), but dealing with the 18O/16O isotopic ratio vs. the 26Al/27Al one. The horizontal dashed line shows the 18O/16O solar value while the vertical one the estimate value of the 26Al/27A in the early solar system as reported by Vescovi et al. (2018).
As mentioned in the introduction and shown by Palmerini et al. (2017), the sole low mass AGB nucleosynthesis model able to simultaneously reproduce the 17O/16O, the 18O/16O and the 26Al/27Al ratios measured in group 2 oxide grains is the one based on the theory by Nucci and Busso (2014). In this scenario the natural expansion of magnetic structures from above the H-burning shell induces a bottom-up mixing that reverses in the stellar envelope materials relatively hot and rich enough in 26Al to reproduce also the highest value of the Al isotopic ratio recorded in group 2 oxide grains. In the same year, Lugaro et al. (2017) suggested that group 2 oxide grains condensate in the envelope of more massive AGB stars (4.5–6 M⊙) of solar metallicity. Their HBB models well reproduce the oxygen isotopic mix shown by those grains and also the 26Al/27Al isotopic ratio when smaller than 0.06. This paper has brought attention back to the hypothesis that oxide grains might have formed in intermediate mass stars due to the presence of HBB: a scenario that had been cast aside in the last years.
Beside the stellar models, the papers by Palmerini et al. (2017) and Lugaro et al. (2017) differ for the nuclear physics inputs employed: the first study mainly uses reaction rates (for proton captures on oxygen isotopes) measured with the Trojan Horse Method by the AsFiN collaboration (Tribble et al., 2014, set A in Table 1), while the second uses reaction rates directly measured by the LUNA collaboration (Broggini et al., 2018, set B in Table 1).
Magnetic Induced Mixing in Low Mass AGB Stars
The occurrence of mixing mechanisms during the AGB phase is one of the most critical issue in the stellar modeling; they have been invoked to explain both the superficial anomalies of light element/isotope abundances and the formation of a reservoir of 13C in the He-rich layers below the H-burning shell, which provides the necessary neutrons for the s-process in low mass AGBs (M ≤ 3 M⊙). Single and coupled effects of stellar rotation, magnetic fields, inversion of the average molecular weight, gravity waves, overshooting, and many other phenomena have been extensively studied over the decades to explain the peculiarities observed in the nucleosynthesis of red giant stars (for an extended discussion see the reviews by Lattanzio, 2019; El Eid, 2016; Nomoto et al., 2013; Herwig, 2005, and references therein). We concentrate on the mixing induce by stellar magnetic fields that, as demonstrated in Nucci and Busso (2014), might trigger the formation and the expansion of bubbles of magnetized materials in the radiative regions of low mass red giant stars. Trippella et al. (2016) have shown that such a mechanism can induce the formation of a 13C-pocket suitable to address the observational constrains to s-process nucleosynthesis in low mass AGB stars, but our interest in the magnetic induced mixing is due to the possibility of reproducing the isotopic composition recorded in both SiC and oxide presolar grains of AGB origin (Palmerini et al., 2017, Palmerini et al., 2018).
According to Nucci and Busso (2014) the velocity v(r) by which the bubbles of magnetized material expand from above the H-burning shell of AGB stars to the base of the convective envelope is describe by the following equation:
where r is the position along the stellar radius, k is the index of the power law
being ρe the density of the radiative layers just below the border of the convective envelope. The factors ƒ1 ∼ (0.01÷0.02) and ƒ1 ∼ 0.01 represent the fraction of the stellar surface covered by the magnetized bubbles and their filling factor, respectively. These values are assumed, following the hypothesis that (i) the fraction of stellar surface covered by the bubbles (which are portions of magnetic flux tubes) at the base of convective should be less than 1/100 Nucci and Busso (2014), and ii) the mass in flux tubes is concentrated in thin current sheets of about 1/100 of the tube section (Hirayama, 1992). As mentioned at the beginning of this section, this magnetic mixing scenario has been demonstrated to account for both the anomalies in light element abundances and the formation of the 13C-pocket responsible for the typical s-process nucleosynthesis in low mass AGB stars by adopting the same choices for the free parameters of the mixing model (as the reader can infer from Trippella et al., 2016; Palmerini et al., 2017, Palmerini et al., 2018). To be efficient enough, the mixing has to be triggered by a magnetic field of a few 105 G. Such a value is in perfect agreement with the intensity of internal magnetic fields estimated with asteroseismology studies for many low mass red giants by the Kepler Observatory (Fuller et al., 2015).
The magnetic extra-mixing model, once applied to a 1.2 M⊙ AGB star with solar metallicity, modifies the oxygen isotopic ratios in the stellar envelopes as reported in Figure 4. Panel A shows nucleosynthesis calculations run by Palmerini et al. (2017) with nuclear reaction rates from set A. The descending blue curves show the evolution of the oxygen isotopic mix due to magnetic buoyancy during the RGB and AGB phase, starting from the surface composition left by the FDU and adopting different values of k (−3.5, −3.4, −3.3, −3.2, and −3.1). The smaller the value of k, the darker the curve. In the same way, panel B of Figure 4 shows the evolution of the surface abundance of 18O/16O as a function of the 26Al/27Al ratio for the same models. Oxide grains of group 1 (open blue circles) and group 2 (open red circles) are reported Figure 4 for comparison with model predictions. The various choices of k, for the reported models, correspond to various depth of the mixing starting layers and define a zone thick enough to host different abundances and temperatures. For this reason models with a smaller k are more efficient in destroying 18O and enriching the stellar envelope in 26Al. The dependence of the final values of the 17O/16O isotopic ratio to the adopted k is instead a bit more complicated because the trend of the equilibrium value of this isotopic ratio as a function of the temperature is not linear (see Figure 3). From panel A of Figure 4, one can observe that magnetic mixing calculations for the 1.2 M⊙ AGB well accounts for the majority of the grains, with small changes in the value of the parameter k. In same way, panel B of the Figure shows that the 1.2 M⊙ AGB model is also a valuable candidates to be progenitors of group 2 grains because the whole range of the 26Al/27Al isotopic ratio recorded in the grains is covered by the lines dealing with the surface abundance evolution due to the magnetic mixing. In the figures we only report the models that better match the oxygen isotopic mix of the grains, but even if the agreement between predictions and observations is slightly worse for the oxygen isotopes, the 1.5 M⊙ model in Palmerini et al. (2017) also results in being a possible candidate as a progenitor of at least group 2 oxide grains with 17O/16O > 0.001.
It has to be pointed out that in AGB stars 26Al is produced and destroyed through its decay and through neutron and/or proton captures. However, the products of neutron capture reactions are visible on the surface of an AGB star only after some TDU episodes. The low mass star considered here is characterized by a low number of TDUs. We can therefore safely conclude that, in the study of presolar oxide grains, the contribution of (n,γ) reactions on 26Al nucleosynthesis is almost negligible.
Hot Bottom Burning and Alumina Dust Production in Intermediate Mass Stars
The detection of an Al2O3 dust shell in several oxygen-rich AGB stars (through mid-infrared interferometric observations at VLTI/MIDI, Karovicova et al., 2013), as long with the detection of a rotational line emission of AlO in the wind of a few stars of the same kind (De Beck et al., 2017), proves that O-rich AGB stars are the main source of alumina dust in the Galaxy, as suggested by several authors starting from Onaka et al. (1986). It is without doubt that more massive objects reverse a major amount of dust in the interstellar medium and that the mass of the Al2O3 grains produced increases with metallicity; intermediate-mass AGB stars, namely, those with a mass up to 7–8 M⊙ and those with solar metallicity, or higher, affected by HBB, are reliable progenitors of presolar oxide grains (Ventura et al., 2020, and references therein). Moreover, the thesis of the production of alumina dust in AGB stars experiencing HBB is so strong and shared that the aluminum abundance in grains has been suggested as an indicator of the mass and of the evolutionary stage of the stellar progenitor (Dell’Agli et al., 2014). Even if several authors discuss the elemental abundances of Al and O in AGB dust, only a few papers in the literature show nucleosynthesis calculations with predictions for oxygen and aluminum isotopic abundances to be compared with the ratios recorded in presolar oxide grains and those of group 2 in particular. Such a lack of data might be due to our still partial knowledge of the physical processes at play and the related computational limitations strongly limits the predictive power of intermediate mass AGB models. The modeling of those stars is actually far from being completely understood and is dependent on many theoretical aspects:
• first, the mixing scheme adopted is extremely important. The use of instantaneous convective mixing (Iben 1973) leads to different (wrong) abundances with respect to the adoption of a time-dependent convective algorithm (Sackmann and Boothroyd, 1992).
• second, the presence of a strong mass-loss hampers the core mass growth, strongly limiting the increase of the HBB efficiency (Mazzitelli et al., 1999).
• last but not least, the resulting nucleosynthesis distribution of light isotopes depends on the degree of coupling between the different physical processes at work at the base of the envelope of massive AGB, particularly the one between mixing and burning (Ventura and D’Antona, 2005).
As already highlighted in the text, the delicate balance between TDU and HBB establishes the production of presolar oxide and SiC grains in massive AGBs, and Lugaro et al. (2017) show that AGB stellar models with mass between 4.5 and 6 M⊙ (and solar metallicity) and HBB at play might account for the oxygen isotopic ratios observed in group 2 oxide grains. Such agreement is possible thanks to the increased rate of 17O(p,α)14N reaction rate measured by Bruno et al. (2016), while data grains are not reproduced by the same nucleosynthesis models run with a different reaction rate, such as the one reported by Iliadis et al. (2010). The comparison of the model predictions with grain isotopic mix is shown by the red curves in Figure 4, where the darker the color the more massive the star is. The red solid lines deal with the evolution in the stellar envelopes of the 18O/16O isotopic ratio as a function of the 17O/16O and the 26Al/27Al (in panel A and B, respectively). The stellar models are supposed to approach the AGB phase with 17O/16O ≥ 0.0023, 18O/16O ≤ 0.0014 (see the corner points of the curves in panel A) and 26Al/27Al ≥ 0.005; beyond these values, the HBB is at play. A careful inspection of Figure 4 might lead to the conclusion that intermediate mass models are not compatible to the oxygen ratios measured in oxide grains because the solid curves are too rich in 17O/16O to match the grains area for most of the grains in panel A. Moreover, the models reach a range of 26Al/27Al values of the grains when the 18O/16O is two or three orders of magnitude smaller than the observed ones (panel B). However, Lugaro et al. (2017) highlighted the possibility that material coming from ancient AGB stars may have been diluted with solar-system material, as illustrated by the dashed lines in Figure 4 that nicely cross the plot areas occupied by grains. If real, such a dilution should be applied to isotopes that are mainly destroyed by HBB, such as 18O, but it should have a comparatively minor effect on those isotopes which are mainly produced in AGBs, as 17O and 26Al.
Conclusion
At the state of the art, low mass AGB stars with a bottom-up extra-mixing (as the magnetic induced one) are likely candidates to be progenitors of group 2 presolar oxide grains. This conclusion is based on the comparison between nucleosynthesis prediction and the isotopic mix recorded in the grains. On the other hand, intermediate mass AGB stars, where HBB is at play, are recognized to be the main sources of alumina dust in the galaxy, but the nucleosynthesis models of those stars are able to reproduce the O and Al isotopic abundances of the grain sample only, 1) assuming a dilution of the stellar wind of the progenitor star with solar-system material and 2) employing in calculations the nuclear data set B (and the 17O(p,α)14N reaction rate by Bruno et al., 2016 in particular). These are two factors that weaken the likelihood of this second hypothesis.
To definitively answer the question whether the progenitors of group 2 oxide grains are low mass AGB with a bottom-up mixing or intermediate mass AGB affected by HBB, a study of the sensitivity of those two scenarios to the nuclear physics inputs should be carried out. A possible way is recomputing the nucleosynthesis of both low mass and intermediate mass AGB stars with the two sets of nuclear reaction rates reported in Table 1. The results of this former analysis will be presented in a future paper (Palmerini et al., in preparation).
Author Contributions
SP, SC, MB and DV run the stellar models for nucleosynthesis calculations. MC and LS took care of the nuclear physics data employed in calculations. All the authors collaborated in writing the paper.
Conflict of Interest
The authors declare that the research was conducted in the absence of any commercial or financial relationships that could be construed as a potential conflict of interest.The reviewer SC declared a shared affiliation with the authors, DV and SC, to the handling editor at time of review.
1The convective mixing episode that affects stars at the beginning of the RGB and enriches the stellar surface with the product of core H-burning nucleosynthesis.
2The slow neutron capture nucleosynthesis process typical of AGB stars and responsible for the production of many elements heavier than Fe in the Galaxy.
References
Abia, C., Palmerini, S., Busso, M., and Cristallo, S. (2012). Carbon and oxygen isotopic ratios in Arcturus and Aldebaran. Astron. Astrophys. 548, A55. doi:10.1051/0004-6361/201220148
Boothroyd, A. I., and Sackmann, I.-J. (1992). Breakdown of the core mass-luminosity relation at high luminosities on the asymptotic giant branch. Astrophys. J. 393, L21. doi:10.1086/186441
Boothroyd, A. I., Sackmann, I.-J., and Wasserburg, G. J. (1994). Predictions of oxygen isotope ratios in stars and of oxygen-rich interstellar grains in meteorites. Astrophys. J. 430, L77. doi:10.1086/187442
Boothroyd, A. I., and Sackmann, I. J. (1999). The CNO isotopes: deep circulation in red giants and first and second dredge‐up. Astrophys. J. 510, 232–250. doi:10.1086/306546
Broggini, C., Bemmerer, D., Caciolli, A., and Trezzi, D. (2018). LUNA: status and prospects. Prog. Part. Nucl. Phys. 98, 55–84. doi:10.1016/j.ppnp.2017.09.002
Bruno, C. G., Scott, D. A., Aliotta, M., Formicola, A., Best, A., Boeltzig, A., et al. (2016). Improved direct measurement of the 64.5 keV resonance strength in the ^{17}O(p,α)^{14}N reaction at LUNA. Phys. Rev. Lett. 117, 142502. doi:10.1103/PhysRevLett.117.142502
Busso, M., Palmerini, S., Maiorca, E., Cristallo, S., Straniero, O., Abia, C., et al. (2010). On the need for deep-mixing in asymptotic giant branch stars of low mass. Astrophys. J. 717, L47. doi:10.1088/2041-8205/717/1/L47
Choi, B. G., Huss, G. R., Wasserburg, G. J., and Gallino, R. (1998). Presolar corundum and spinel in ordinary chondrites: origins from AGB stars and a supernova. Science 282, 1284. doi:10.1126/science.282.5392.1284
Cognata, M. L., Spitaleri, C., and Mukhamedzhanov, A. M. (2010). Effect of high-energy resonances on the 18O(p,α)15N reaction rate at AGB and post-AGB relevant temperatures. Astrophys. J. 723, 1512–1522. doi:10.1088/0004-637X/723/2/1512
De Beck, E., Decin, L., Ramstedt, S., Olofsson, H., Menten, K. M., Patel, N. A., et al. (2017). Search for aluminium monoxide in the winds of oxygen-rich AGB stars. Astron. Astrophys. 598, A53. doi:10.1051/0004-6361/201628928
Dell’Agli, F., García-Hernández, D. A., Rossi, C., Ventura, P., Di Criscienzo, M., and Schneider, R. (2014). On the alumina dust production in the winds of O-rich asymptotic giant branch stars. Mon. Not. Roy. Astron. Soc. 441, 1115–1125. doi:10.1093/mnras/stu647
Denissenkov, P. A., Pinsonneault, M., and MacGregor, K. B. (2009). Magneto-thermohaline mixing in red giants. Astrophys. J. 696, 1823–1833. doi:10.1088/0004-637X/696/2/1823
Di Leva, A., Scott, D. A., Caciolli, A., Formicola, A., Strieder, F., Aliotta, M., et al. (2014). Underground study of the O17(p,γ)F18 reaction relevant for explosive hydrogen burning. Phys. Rev. C 89, 015803. doi:10.1103/PhysRevC.89.015803
D’Antona, F., and Mazzitelli, I. (1996). Hot bottom burning in asymptotic giant branch stars and the turbulent convection model. Astrophys. J. 470, 1093. doi:10.1086/177933
Eggleton, P. P., Dearborn, D. S., and Lattanzio, J. C. (2006). Deep mixing of 3He: reconciling Big Bang and stellar nucleosynthesis. Science 314, 1580. doi:10.1126/science.1133065
Eggleton, P. P., Dearborn, D. S. P., and Lattanzio, J. C. (2008). Compulsory deep mixing of 3He and CNO isotopes in the envelopes of low‐mass red giants. Astrophys. J. 677, 581–592. doi:10.1086/529024
El Eid, M. F. (2016). Introduction to asymptotic giant branch stars. J. Phys. Conf. Ser. 703, 012005. doi:10.1088/1742-6596/703/1/012005
Fuller, J., Cantiello, M., Stello, D., Garcia, R. A., and Bildsten, L. (2015). Asteroseismology can reveal strong internal magnetic fields in red giant stars. Science 350, 423–426. doi:10.1126/science.aac6933
Harris, M. J., and Lambert, D. L. (1984). Oxygen isotopic abundances in the atmospheres of seven red giant stars. Astrophys. J. 285, 674–682. doi:10.1086/162544
Herwig, F. (2005). Evolution of asymptotic giant branch stars. Annu. Rev. Astron. Astrophys. 43, 435–479. doi:10.1146/annurev.astro.43.072103.150600
Hirayama, T. (1992). The current sheet and joule heating of a slender magnetic tube in the upper photosphere. Sol. Phys. 137, 33–50. doi:10.1007/BF00146574
Hynes, K. M., and Gyngard, F. (2009). The Presolar Grain Database: http://presolar.wustl.edu. In Lunar and Planetary Science Conference, Woodlands, TX, March 23–27, 2009, 1198.
Iben, I. J. (1973). On the abundance of lithium in red giants of intermediate mass. Astrophys. J. 185, 209–212. doi:10.1086/152409
Iben, I. J. (1975). Thermal pulses; p-capture, alpha-capture, s-process nucleosynthesis; and convective mixing in a star of intermediate mass. Astrophys. J. 196, 525–547. doi:10.1086/153433
Iliadis, C., Angulo, C., Descouvemont, P., Lugaro, M., and Mohr, P. (2008). New reaction rate for 16O(p,γ)17F and its influence on the oxygen isotopic ratios in massive AGB stars. Phys. Rev. C 77, 045802. doi:10.1103/PhysRevC.77.045802
Iliadis, C., Longland, R., Champagne, A. E., Coc, A., and Fitzgerald, R. (2010). Charged-particle thermonuclear reaction rates: II. Tables and graphs of reaction rates and probability density functions. Nucl. Phys. 841, 31–250. doi:10.1016/j.nuclphysa.2010.04.009
Karovicova, I., Wittkowski, M., Ohnaka, K., Boboltz, D. A., Fossat, E., and Scholz, M. (2013). New insights into the dust formation of oxygen-rich AGB stars. Astron. Astrophys. 560, A75. doi:10.1051/0004-6361/201322376
La Cognata, M., Spitaleri, C., and Mukhamedzhanov, A. M. (2010). Effect of High-energy Resonances on the 18O(p, α)15N Reaction Rate at AGB and Post-AGB Relevant Temperatures. Astrophys. J. 723, 1512–1522. doi:10.1088/0004-637X/723/2/1512
Lagarde, N., Decressin, T., Charbonnel, C., Eggenberger, P., Ekström, S., and Palacios, A. (2012). Thermohaline instability and rotation-induced mixing. Astron. Astrophys. 543, A108. doi:10.1051/0004-6361/201118331
Lattanzio, J. (2019). AGB stars: remaining problems. Proc. IAU 14, 3–8. doi:10.1017/S1743921318007019
Lattanzio, J. C., Siess, L., Church, R. P., Angelou, G., Stancliffe, R. J., Doherty, C. L., et al. (2015). On the numerical treatment and dependence of thermohaline mixing in red giants. Mon. Not. Roy. Astron. Soc. 446, 2673–2688. doi:10.1093/mnras/stu2238
Lugaro, M., Karakas, A. I., Bruno, C. G., Aliotta, M., Nittler, L. R., Bemmerer, D., et al. (2017). Origin of meteoritic stardust unveiled by a revised proton-capture rate of 17O. Nat Astron 1, 0027. doi:10.1038/s41550-016-0027
Mazzitelli, I., D’Antona, F., and Ventura, P. (1999). Full spectrum of turbulence convective mixing. II. Lithium production in AGB stars. Astron. Astrophys. 348, 846–860.
Nittler, L. R., Alexander, C. M. O. D., Gao, X., Walker, R. M., and Zinner, E. (1997). Stellar sapphires: the properties and origins of presolar Al2O3in meteorites. Astrophys. J. 483, 475–495. doi:10.1086/304234
Nittler, L. R. (2009). On the mass and metallicity distributions of the parent AGB stars of O-rich presolar stardust grains, Publ. Astron. Soc. Aust., 26, 271–277. doi:10.1071/AS08071
Nollett, K. M., Busso, M., and Wasserburg, G. J. (2003). Cool bottom processes on the thermally pulsing asymptotic giant branch and the isotopic composition of circumstellar dust grains. Astrophys. J. 582, 1036–1058. doi:10.1086/344817
Nomoto, K. i., Kobayashi, C., and Tominaga, N. (2013). Nucleosynthesis in stars and the chemical enrichment of galaxies. Annu. Rev. Astron. Astrophys. 51, 457–509. doi:10.1146/annurev-astro-082812-140956
Nucci, M. C., and Busso, M. (2014). Magnetohydrodynamics and deep mixing in evolved stars. I. Two- and three-dimensional analytical models for the asymptotic giant branch. Astrophys. J. 787, 141. doi:10.1088/0004-637X/787/2/141
Onaka, T., Nakada, Y., Tanabe, T., Sakata, A., and Wada, S. (1986). A Quenched Carbonaceous Composite/QCC/Grain model for the interstellar 220-NM extinction hump. Astrophys. Space Sci., 118, 411. doi:10.1007/BF00651159
Palacios, A., Charbonnel, C., Talon, S., and Siess, L. (2006). Rotational mixing in low-mass stars. Astron. Astrophys. 453, 261–278. doi:10.1051/0004-6361:20053065
Palmerini, S., La Cognata, M., Cristallo, S., and Busso, M. (2011a). Deep mixing in evolved stars. I. The effect of reaction rate revisions from C to Al. Astrophys. J. 729, 3. doi:10.1088/0004-637X/729/1/3
Palmerini, S., Cristallo, S., Busso, M., Abia, C., Uttenthaler, S., Gialanella, L., et al. (2011b). Deep mixing in evolved stars. II. interpreting Li abundances in red giant branch and asymptotic giant branch stars. Astrophys. J., 741, 26. doi:10.1088/0004-637X/741/1/26
Palmerini, S., Sergi, M. L., La Cognata, M., Lamia, L., Pizzone, R. G., and Spitaleri, C. (2013). The RGB and AGB star nucleosynthesis in light of the recent 17O(p, α)14N and 18O(p, α)15N reaction-rate determinations. Astrophys. J. 764, 128. doi:10.1088/0004-637X/764/2/128
Palmerini, S., Trippella, O., and Busso, M. (2017). A deep mixing solution to the aluminum and oxygen isotope puzzles in presolar grains. Mon. Not. Roy. Astron. Soc. 467, stx137–1201. doi:10.1093/mnras/stx137
Palmerini, S., Trippella, O., Busso, M., Vescovi, D., Petrelli, M., Zucchini, A., et al. (2018). s-Processing from MHD-induced mixing and isotopic abundances in presolar SiC grains. Geochem. Cosmochim. Acta 221, 21–36. doi:10.1016/j.gca.2017.05.030
Sackmann, I.-J., and Boothroyd, A. I. (1992). The creation of superrich lithium giants. Astrophys. J. 392, L71. doi:10.1086/186428
Sergi, M. L., La Cognata, M., Guardo, G. L., Gulino, M., Mrazek, J., Palmerini, S., et al. (2020). Indirect measurements of oxygen burning reactions of astrophysical interest. Front. Astron. Space Sci., 60, 7. doi:10.3389/fspas.2020.00060
Sergi, M. L., Spitaleri, C., La Cognata, M., Lamia, L., Pizzone, R. G., Rapisarda, G. G., et al. (2015). Improvement of the high-accuracy 17O(p,α)14N reaction-rate measurement via the Trojan Horse method for application to O nucleosynthesis. Phys. Rev. C 91, 065803. doi:10.1103/PhysRevC.91.065803
Straniero, O., Imbriani, G., Strieder, F., Bemmerer, D., Broggini, C., Caciolli, A., et al. (2013). Impact of a revised 25Mg(p, γ)26Al reaction rate on the operation of the Mg-Al cycle. Astrophys. J. 763, 100. doi:10.1088/0004-637X/763/2/100
Tribble, R. E., Bertulani, C. A., Cognata, M., Mukhamedzhanov, A. M., and Spitaleri, C. (2014). Indirect techniques in nuclear astrophysics: a review. Rep. Prog. Phys. 77, 106901. doi:10.1088/0034-4885/77/10/106901
Trippella, O., Busso, M., Palmerini, S., Maiorca, E., and Nucci, M. C. (2016). s-Processing in AGB stars revisited. ii. enhanced13c production through MHD-induced mixing. Astrophys. J. 818, 125. doi:10.3847/0004-637X/818/2/125
Ventura, P., and D’Antona, F. (2005). Full computation of massive AGB evolution. Astron. Astrophys. 431, 279–288. doi:10.1051/0004-6361:20041917
Ventura, P., Dell’Agli, F., Lugaro, M., Romano, D., Tailo, M., and Yagüe, A. (2020). Gas and dust from metal-rich AGB stars. Astron. Astrophys. 641, A103. doi:10.1051/0004-6361/202038289
Vescovi, D., Busso, M., Palmerini, S., Trippella, O., Cristallo, S., Piersanti, L., et al. (2018). On the origin of early solar system radioactivities: problems with the asymptotic giant branch and massive star scenarios. Astrophys. J. 863, 115. doi:10.3847/1538-4357/aad191
Vescovi, D., Cristallo, S., Busso, M., and Liu, N. (2020). Magnetic-buoyancy-induced mixing in AGB stars: presolar SiC grains. Astrophys. J. 897, L25. doi:10.3847/2041-8213/ab9fa1
Wasserburg, G. J., Boothroyd, A. I., and Sackmann, I.-J. (1995). Deep circulation in red giant stars: a solution to the carbon and oxygen isotope puzzles? Astrophys. J. Lett. 447, L37. doi:10.1086/309555
Zinner, E. (2014). “Presolar Grains,” in: Meteorites and cosmochemical processes. Editors Davis and M. Andrew, Vol. 1, 181–213. Available at: https://ui.adsabs.harvard.edu/abs/2014mcp..book..181Z. Provided by the SAO/NASA Astrophysics Data System.
Keywords: AGB stars, presolar grains, nucleosynthesis–stars: abundances, reaction rates, isotopic abundances
Citation: Palmerini S, Cristallo S, Busso M, La Cognata M, Sergi ML and Vescovi D (2021) Low Mass Stars or Intermediate Mass Stars? The Stellar Origin of Presolar Oxide Grains Revealed by Their Isotopic Composition. Front. Astron. Space Sci. 7:607245. doi: 10.3389/fspas.2020.607245
Received: 16 September 2020; Accepted: 18 November 2020;
Published: 15 February 2021.
Edited by:
Scilla Degl’Innocenti, University of Pisa, ItalyReviewed by:
Flavia Dell’Agli, Astronomical Observatory of Rome (INAF), ItalySanti Cassisi, Astronomical Observatory of Abruzzo (INAF), Italy
Copyright © 2021 Palmerini, Cristallo, Busso, La Cognata, Sergi and Vescovi. This is an open-access article distributed under the terms of the Creative Commons Attribution License (CC BY). The use, distribution or reproduction in other forums is permitted, provided the original author(s) and the copyright owner(s) are credited and that the original publication in this journal is cited, in accordance with accepted academic practice. No use, distribution or reproduction is permitted which does not comply with these terms.
*Correspondence: S. Palmerini, c2FyYS5wYWxtZXJpbmlAcGcuaW5mbi5pdA==