- 1Max-Planck Institut für Extraterrestrische Physik, Garching, Germany
- 2Excellence Cluster Universe, Technische Universität München, Garching, Germany
- 3Department of Physics and Astronomy, Purdue University, West Lafayette, IN, United States
- 4Sternberg Astronomical Institute, Lomonossov University, Moscow, Russia
The detection of a faint, short gamma-ray burst (GRB 170817A) in coincidence with the gravitational wave (GW 170817) detection by LIGO/Virgo is at odds with the expected known luminosity and redshift distribution of short GRBs (sGRB). Examining the observer-frame parameter space of all Fermi-GBM sGRBs shows that the sGRB associated with GW 170817 is extreme in its combination of flux, spectral softness and temporal structure. We identify a group of similar GRBs, one of which has been associated to a bright galaxy at 75 Mpc. We speculate that a good fraction of the previously detected faint sGRBs is not at large redshifts, but local, at redshift smaller than 0.1, seen off-axis. We incorporate off-axis emission in the estimate of the rates of sGRBs, and predict that a large fraction of future GW-detections of NS-NS mergers will be accompanied by faint γ-ray emission, contrary to previous thinking. The much wider gamma-ray emission cone from NS-NS mergers also implies a higher deadly rate of γ-rays for extraterrestrial life in the Universe.
1. Introduction
The coincident detection of gravitational waves (GW 170817) from a binary neutron star merger with LIGO/Virgo (Abbott et al., 2017a; The LIGO Scientific Collaboration and Virgo Collaboration, 2017a,b) and short-lived gamma-ray emission with Fermi-GBM (Abbott et al., 2017b; Connaughton et al., 2017; Goldstein et al., 2017; von Kienlin et al., 2017) (called GRB 170817A) is a milestone for the establishment of multi-messenger astronomy. Merging neutron stars (NS) represent the standard scenario for short-duration (<2 s) gamma-ray bursts (GRBs) (Eichler et al., 1989) which are produced in a collimated, relativistically expanding jet with an opening angle of a few degrees and a bulk Lorentz factor of 300–1,000. While the LIGO/Virgo detection is consistent with predictions, the measured faint γ-ray emission from GRB 170817A, if associated to the merger event at a distance of 40 Mpc, is about 1,000 × less luminous than known short-duration GRBs (Guetta and Piran, 2005). Hence, the presence of this sGRB in the local Universe is either a very rare event, i.e., not expected from past observations, or points to a dramatic lack of knowledge of the emission properties of sGRBs outside their narrow jets. It has been thought that such events would be bright as most models predict that the emission from sGRBs must be on-axis. Many relations, such as the Amati relation (Amati et al., 2002) predict that apparently brighter objects are closer and dimmer objects are further away. Thus, as this object deviates from the expected relation, it is surely unexpected.
Here, we investigate the possibility of being rare by searching the sGRB sample of Fermi-GBM for bursts with similar spectral and temporal properties. Motivated by finding similar events, we then compute the impact of faint off-axis emission on the observed rates of sGRBs.
2. Observations and Data Analysis
2.1. Gamma-Ray Burst 170817A
The Gamma-ray Burst Monitor (GBM) (Meegan et al., 2009) onboard Fermi triggered on a burst-like transient on August 17, 2017 at 12:41:06.5 UT (trigger 524666471/170817529) (Connaughton et al., 2017; von Kienlin et al., 2017). With a GBM localization (Connaughton et al., 2017; von Kienlin et al., 2017) consistent with that of the LIGO/Virgo trigger (The LIGO Scientific Collaboration and Virgo Collaboration, 2017a) about 2 s earlier, there is little doubt that these two triggers are from the same event, a neutron star binary coalescence (Abbott et al., 2017a; The LIGO Scientific Collaboration and Virgo Collaboration, 2017b). It is possible that the LIGO and Fermi-GBM events are not related, however, the combination of observations across many wavelengths (e.g., Goldstein et al., 2017; Troja et al., 2017, 2018; Gottlieb et al., 2019), and the previous predictions falling perfectly inline with these observations push even the most pessimistic observers to accept the relation between the two events.
To obtain the spectral properties of the GRB associated to GW 170817, the temporal off-source photon event light curve was fitted with a polynomial via an unbinned Poisson likelihood. This polynomial was used to estimate the background counts during the source temporal interval. The on-source interval was derived by choosing bins via the Bayesian blocks method (Scargle et al., 2013) and selecting bins with a 3σ excess over the estimated background. This resulted in a single bin lasting 0.65 s (Figure 1). After the initial spike, there is no statistically significant emission from which to perform spectral analysis. The count spectra covering 10 keV to 40 MeV of this bin were fit via a Poisson-Gaussian likelihood to an exponentially cutoff power law. The spectral parameters were estimated by computing the posterior of the priors and likelihood with the Multinest (Feroz et al., 2009) nested sampling algorithm. The fits resulted in a photon index of α = −1.00±0.23 and a cutoff energy of Ec = 230 ± 100 keV. The event fluence during the ~0.65 s prime emission time interval in the 10–1,000 keV energy range is (2.45 ± 0.4) × 10−7 erg cm−2. Combined with the LIGO distance estimate of 40 Mpc (Abbott et al., 2017a; The LIGO Scientific Collaboration and Virgo Collaboration, 2017c) this implies an isotropic equivalent energy of 5.2 × 1046 erg in the 1 keV–10 MeV band (this energy band is given for comparability; no photons above 400 keV are measured for this event). Comparing the spectral properties of GRB 170817A to the sample of previously detected GRBs (Bhat et al., 2016) by Fermi-GBM shows that it is not a typical short GRB. The event resides on the boundary of the α-flux, α-Ec plane and temporal smoothness (see below), but is accompanied by a few sGRBs with similar properties (Figure 2). Spectral and temporal analyses were performed with the Multi-Mission Maximum Likelihood framework (3ML) (Vianello et al., 2015).
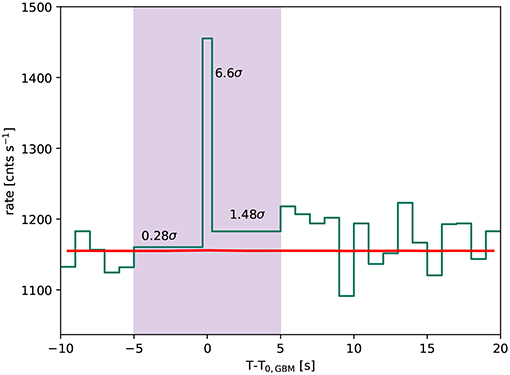
Figure 1. The Bayesian-block binned count rate light curve of the GBM detected sGRB associated with GW 170817. The red line indicates the fitted background level and the shaded region indicates the times over which Bayesian blocks were applied. Additionally, the significance of the each temporal bin is displayed.
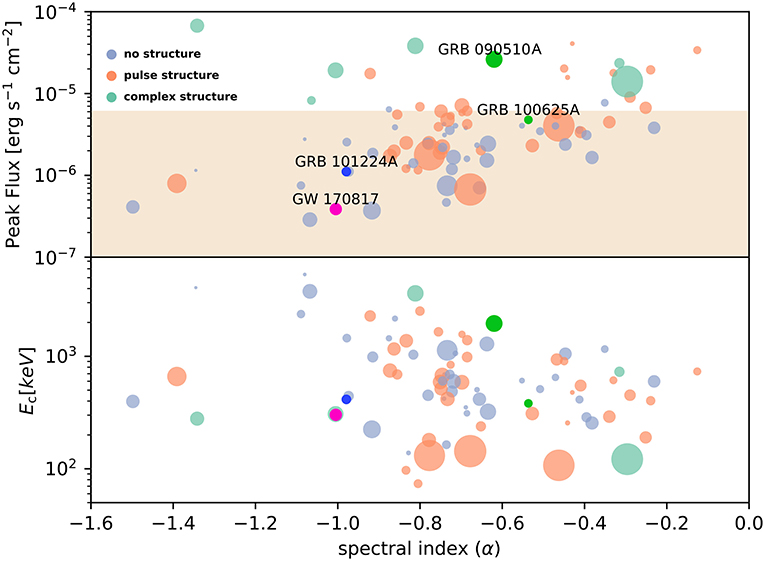
Figure 2. Comparison of the spectral properties of GRB 170817A and the spectrally and temporally similar GRB 101224A with respect to our sample of well-fit short GRBs. Colors indicate light curve structure (see labels in the top left) and size indicates the duration from our Bayesian block analysis. The shaded region corresponds to the flux level at which off-axis short GRBs become the dominant contribution to the total rate (see Figure 5).
2.2. Analysis of All Short GBM-Detected GRBs
All Fermi-GBM GRBs with a reported duration <2 s were reanalyzed with the method described in Greiner et al. (2016) and temporal bins were selected for spectral analysis if they exceeded a threshold of 3σ above background. To study the peak flux spectral properties of the population, we discarded events with errors >50% on the photon spectral index and 60% on the cutoff energy to eliminate poorly fit spectra while simultaneously retaining those with constraints as good as those for GRB 170817A. The durations of the events were calculated by taking the start of the first significant bin to the end of the last significant bin which could include bins in between that were not significant.
Fermi-GBM has observed 355 sGRBs until mid-2014 (Bhat et al., 2016). After removal of poor fits and sGRB with badly modeled background in the GBM catalogs, we were left with 248 GRBs. Our cut on parameter constraints reduced the sample to 68 sGRBs. The choice of a cutoff power law for the spectral function does introduce a bias for low flux GRBs that would only be fit by a power law. These would not have proper flux estimates due to a lack of a measurable energy cutoff and are the main contribution to sGRBs that were removed from the sample due to poor constraints on the spectral parameters. Since these GRBs have low flux, our rate estimates are conservative.
In order to assess temporal smoothness of the light curves, the pulse structure was determined by dividing the observed significant intervals into three categories (which are color-coded in Figure 2): (1) single bin, (2) contiguous bins with monotonic increase/decrease or rise and decay pulse shape and, (3) complex which including non-contiguous bins.
2.3. GBM Bursts Similar to GRB 170817A
We searched our GBM GRB database for events with similar spectral and temporal characteristics. On December 24, 2010, at 05:26:57.5 UTC, both Swift-BAT and Fermi-GBM triggered on the short GRB 101224A (McBreen, 2010), lasting 0.36 s. Our analysis of its temporal and spectral properties shows that the event is remarkably similar to GRB 170817A (Figures 2, 3), including its light curve structure. As noted earlier (Krimm et al., 2017), there is the galaxy pair MCG+08-34-033 nearby, at a distance of 75 Mpc. The offset of this GRB to the center of MCG+08-34-033 is <135 kpc, not unusual for sGRBs (Berger, 2010). Assuming this to be the distance of GRB 101224A, we calculate a fluence of 2.8 × 1047 erg in the 1 keV–10 MeV band. For comparison, assuming standard ΛCDM cosmological parameters (Planck Collaboration, 2015), the short GRB 090510A (Rau, 2009) at luminosity distance of 5,990 Mpc has an energy of 2.7 × 1053 erg and GRB 100625A (Bhat, 2010) at a luminosity distance of 2,590 Mpc has an energy of 2.3 × 1053 erg, making them markedly dissimilar from the two local events we identify (Figure 4). In Figure 2, the two candidate off-axis GRBs are spectrally weak and soft in comparison to the population, however, they do not deviate from the typical cutoff energy distribution. In contrast, GRB 0090510A and GRB 100625A are spectrally harder and brighter while being two orders of magnitude further away.
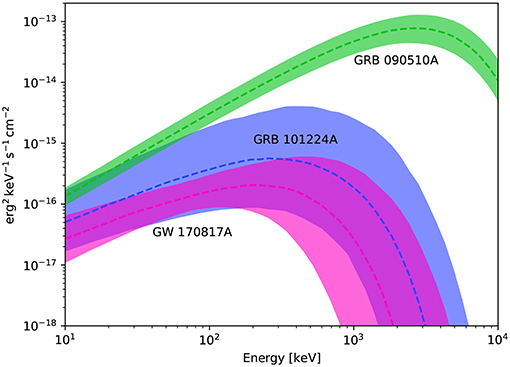
Figure 3. Comparison of the observer-frame νFν gamma-ray spectrum of GRB 170817A with that of GRB 101224A, and that of the distant and likely on-axis GRB 090510A. The shaded regions indicate the 1σ confidence interval for the flux.
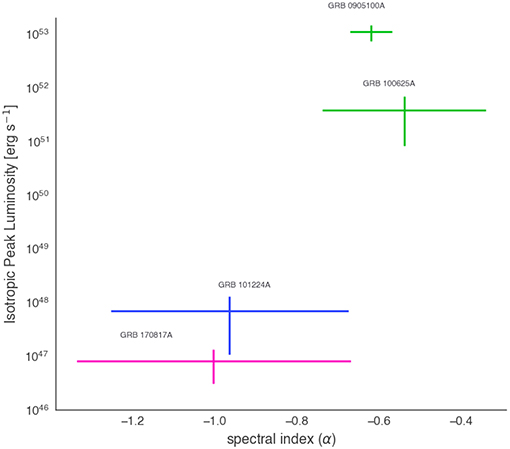
Figure 4. The isotropic luminosity of the peak flux bin for each GRB with redshift in our sample. Both local GRBs exhibit isotropic luminosities of about 1,000 times less and spectra markedly softer than those at larger distances. If we assume that high observed flux sGRBs are the result of on-axis emission, then we expect them to have harder observed spectra and high intrinsic luminosities.
2.4. Expectations for Off-Axis Emission
While there is only one published prediction of the properties of off-axis emission (Janka et al., 2006), the generic expectations are a decreased luminosity and non-complex observed light curve structure due to the relativistic smoothing of the internal emission episodes (Salafia et al., 2016). Similarly, the stacking of spectra observed over the profile of the jet leads to an observed increase in low-energy photons and thus a softer spectrum below the νFν peak (Yamazaki et al., 2002). These are exactly the properties of GRB 170817A. Recently, studies have attempted to advance these predictions in light of more of these events (e.g., Eichler, 2018; Gottlieb et al., 2019; Kathirgamaraju et al., 2019). Moreover, many of these newer observations confirm that the jet is indeed beamed implying that the emission was observed off-axis. Conversely, observed on-axis emission probes the internal Lorentz profile for the jet allowing the observer to see multi-episodic emission as well as the direct spectral (harder) shape of the spectrum. Given this separation into two populations, with a coincidence of expected and observed properties, our working hypothesis below is that GRB 170817A and the likes are members of the same population but observed off-axis. This is observationally supported also by the measurement of superluminal motion of the radio jet of GRB 170817A from which an off-axis angle of 20 ± 5° is inferred (Mooley et al., 2018). The alternative, a low-luminosity population has the additional problem that a luminosity difference of a factor 105 would have to be accommodated with the same emission mechanism which is challenging (Begue et al., 2017). Instead, several other explanations have been proposed, the most popular being emission from mildly relativistic cocoon (Kasliwal et al., 2017; Lazzati et al., 2017; Kathirgamaraju et al., 2018) or emission from shock-breakout (Gottlieb et al., 2018).
2.5. Modeling of sGRB Rates
With another promising off-axis sGRB identified, and a third one suggested recently (Troja et al., 2018), we can ask the question: how many of these events we have detected with Fermi-GBM in the past without recognizing them as being nearby and off-axis?
We base our simple estimates of the rate of sGRBs on the method proposed previously (Guetta and Piran, 2005; Coward et al., 2012). We assume that the peak energy flux limit for detection by the GBM detector is 10−7 erg s−1 cm−2. Knowing the spectrum and the redshift, the maximum distance dmax at which a given GRB is visible can be estimated (after k-correction). This maximum distance corresponds to a maximum volume Vmax. Then the rate is estimated as
where Ω = 0.5 is the fraction of the sky seen by GBM and T = 9 years is the duration of the mission. This leads to a rate of GRB 170817A and GRB 101224A of ~170 and ~2 Gpc−3 yr−1. This is far more than the rate of individual other short GRBs, being around 0.03 Gpc−3 yr−1, with the exception of GRB 080905 which has high individually derived rate compared with other sGRBs (Coward et al., 2012). While the rates given above are strongly sensitive to the chosen value of the flux limit, the ratio between rates does not depend on this flux limit, provided that the k-correction can be neglected, as is the case for bursts at small redshift.
2.6. Estimate of the Ratio of On-Axis to Off-Axis Bursts
We estimate the ratio of sGRBs seen on- and off-axis as a function of redshift. The ratio of such off-axis bursts to on-axis bursts strongly depends on the structure of the jet and on the luminosity function; both poorly understood. We assume that the angular-dependent, emitted, co-moving luminosity of a burst can be expressed as a step function
where L0 is the luminosity emitted in the direction of the jet, θ0 is the opening angle, θobs is the angle between the jet's direction and the observer direction, and is a reduction factor, taken to be 100 (we note that this is conceptually different from off-axis afterglow emission, where due to sideways expansion θ0 is growing, from originally θ0 < θobs toward θ0>θobs at late stages). We further assume that if θobs is larger than 45°, the sGRB is not seen.
The distribution of emitted luminosity can be obtained by
where Ψ(L0) and ξ(θ0) are the distributions of the jet's luminosity, L0, and of the opening angle. We can take those distributions in the form
where Θ is the Heaviside function, Lb is the break luminosity which can be taken at few 1053 erg s−1, β = 0.5 describes the decay of the luminosity distribution (Ghirlanda et al., 2016) and erg s−1 is the minimum luminosity of an on-axis burst. The opening angle distribution function is taken to be a log normal distribution centered on θref = 7° with width σ = 0.1. These forms and values are taken to mimic values and general shapes derived from the simulations of Janka et al. (2006).
We further assume that the rate of sGRBs follows the retarded star formation rate (Li, 2008)
where SFR is the star formation rate at a given redshift and f is the distribution of delay τ, taken to be a log-normal distribution (Wanderman and Piran, 2015; Beniamini and Piran, 2019) centered on 3 Gyr with a deviation of 0.2 Gyr. The star formation rate is taken to be the form
via work of Hopkins and Beacom (2006), though it is possible to adapt the calculations to other forms since knowledge of the true SFR is uncertain.
The rate of short GRBs between two given fluxes F1 and F2 up to a given redshift z can therefore be estimated as
where ρ0 is the local rate of short GRBs, dV/dz is the comoving volume and dL is the luminosity distance. Overall, the rate is a 5-fold integral computed numerically with parallel processing on a GPU using CUDA. The results are shown in Figure 5: on and off-axis emission rates are separated to show where each component of the total rate would dominate. For the local Universe at redshift smaller than 0.02, off-axis emission would dominate. Conversely, on-axis sGRBs dominate the observed rate at high redshift. Notably, even after integrating deep into the Universe, the low end of the observed flux distribution would be dominated by off-axis events. Even if this computation needs to be refined, it indicates that for the future LIGO/Virgo observing runs we can expect to detect faint short GRBs until their horizon exceeds 300 Mpc. While on-axis sGRBs, beamed at us, are visible to a redshift of about 1.5 (the highest redshift sGRBs are 1.4, 1.7, and 2.6, the last one being highly debated); off-axis GRBs are unbeamed, and with the same instrument sensitivity can only be seen to about 1/30 of those distances, i.e., about 300 Mpc. Our analysis of Fermi-GBM data suggests that at least a few percent (for an off-axis flux reduction factor = 1,000), if not up to 30% ( = 100) of sGRBs are local (Figure 5). We note that this fraction also depends on the parametrization of the jet profile, on the merger delay and on the luminosity distribution. This range of the local sGRB rate is consistent with the detection of one such event during LIGO run O2 with a distance threshold of 100 Mpc.
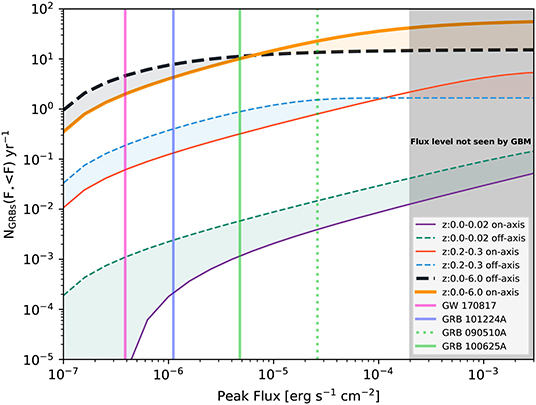
Figure 5. The cumulative short-GRB rate distribution is shown as a function of flux integrated over F* to F, calculated by assuming a jet structure and luminosity function and then convolving this with the binary neutron star merger rate for various redshift integration ranges. The GRBs with redshift in our sample are plotted for comparison. The shaded region on the right indicates the flux values for which GBM has not observed a GRB.
3. Implications and Conclusions
Given the assumption that the bulk of sGRBs are local off-axis events, it begs the question to why this is not observed in the Swift sGRB redshift distribution? This question can be split into two questions: (a) what is the detection rate of Swift-BAT for off-axis GRBs? and (b) why has Swift-XRT not detected the X-ray afterglows of off-axis GRBs?
As to the latter question, the noteworthy difference between on- and off-axis GRBs is that the afterglows of the latter are fainter and delayed by up to several days depending on the off-axis angle (Rhoads, 1997), as also observed for GRB 170817A (Troja et al., 2017). Thus, for off-axis angles larger than ~10°, no afterglow is visible during the first 2–3 days in which case further follow-up with Swift would have terminated. Out of the 104 short GRBs which Swift has detected until October 2017 (out of which 35 have a redshift, all being >0.1), no X-ray afterglow was found for 7, excluding 14 GRBs with no or late satellite slews—thus about 8% of short Swift-detected GRBs are candidates for a local, off-axis population.
As to question (a), we start by re-capitulating that Swift-BAT only finds 10% of all GRBs being short, about a factor 2 smaller than the rates seen with CGRO-BATSE and Fermi-GBM. Furthermore, we note that the off-axis GRBs are soft (and faint), and that the Swift-BAT effective area peaks at ~80 keV (for a typical 30–45°off the telescope axis). This is on contrast to the peak efficiency of Fermi-GBM at 30 keV (for a typical angle of 30–45°off the NaI crystal axis. Thus, soft short GRBs suffer an additional bias in Swift-BAT detections, the accurate amount of which will have to be determined with a proper population simulation. However, in any case, it would increase the above value of 8%, making it not an upper limit anymore. It is therefore interesting to hypothesize that a large observational bias has occurred due to the combination of these two effects, as a result of which the observed Swift sGRB redshift distribution could have contributed in a beautiful way to fool we astronomers.
Past campaigns to follow-up sGRBs have focused their efforts on bright, energetic events. If we liberally extend our conclusions that dim and spectrally uninteresting events are in fact signatures of local neutron star mergers, then we must refocus our follow-up campaigns to examine and learn more about these faint events. With a sizable sample of confirmed local events, better estimates on rates will follow, and thus more accurate predictions for LIGO/Virgo detections. While the observed single NS-NS merger at 40 Mpc within the LIGO O2 run is grossly consistent with previous predictions of the merger rate in the local surrounding (Lipunov et al., 1987; Belczynski et al., 2002; Chruslinska et al., 2018), we conclude from Figure 5 that during O3 we can expect many common LIGO/Virgo/GBM detections.
On a more global aspect, it has already been estimated that GRBs, in general, leave only ~10% of galaxies hospitable for life, and only after z < 0.5 (Piran and Jimenez, 2014; Anchordoqui et al., 2017). A larger local population of sGRBs would substantially lower this rate and bring it closer to z = 0. Therefore, observations to measure the population size of local sGRBs and their luminosity function will aid in answering the question of “Are we really alone in the Universe”?
Data Availability Statement
The datasets generated for this study are available on request to the corresponding author.
Author Contributions
JB, JG, and DB developed the presented ideas and performed the analysis. DG, FB, and VL assisted in discussions and interpretation.
Funding
Support for the German contribution to Fermi-GBM was provided by the Bundesministerium für Bildung und Forschung (BMBF) via DLR under contract number 50 QV 0301. The MASTER project was supported in part by the Development Program of Lomonosov Moscow State University, Moscow Union OPTICA, and the Russian Science foundation grant 16-12-00085.
Conflict of Interest
The authors declare that the research was conducted in the absence of any commercial or financial relationships that could be construed as a potential conflict of interest.
Acknowledgments
JB and JG acknowledge support by the DFG cluster of excellence Origin and Structure of the Universe (www.universe-cluster.de). DB, FB, and JG acknowledge support by the DFG through SFB 1258. DG acknowledges support from NASA through the grants NNX16AB32G and NNX17AG21G issued through the Astrophysics Theory Program.
References
Abbott, B. P., Abbott, R., Abbott, T. D., Acernese, F., Ackley, K., Adams, C., et al. (2017a). GW 170817: Observation of gravitational waves from NS-NS binary merger. Phys. Rev. Lett. 119:161101. doi: 10.1103/PhysRevLett.119.161101
Abbott, B. P., Abbott, R., Abbott, T. D., Acernese, F., Ackley, K., Adams, C., et al. (2017b). Gravitational waves and gamma-rays from a binary neutron star merger: GW 170817 and GRB 170817A. Astrophys. J. 848:L13. doi: 10.3847/2041-8213/aa920c
Amati, L., Frontera, F., Tavani, M., in't Zand, J. J. M., Antonelli, A., Costa, E., et al. (2002). Intrinsic spectra and energetics of BeppoSAX gamma-ray bursts with known redshifts. Astron. Astrophys. 390, 81–89. doi: 10.1051/0004-6361:20020722
Anchordoqui, L. A., Weber, S. M., and Soriano, J. F. (2017). “Is there anybody out there?” in 35th International Cosmic Ray Conference, PoS(ICRC2017)254. Available online at: https://pos.sissa.it/301/254/pdf
Begue, D., Burgess, J. M., and Greiner, J. (2017). The peculiar physics of GRB 170817A and implications for short GRBs. Astrophys. J. 851:19. doi: 10.3847/2041-8213/aa9d85
Belczynski, K., Kalogera, V., and Bulik, T. (2002). A comprehensive study of binary compact objects as gravitational wave sources: evolutionary channels, rates and physical properties. Astrophys. J. 572, 407–431. doi: 10.1086/340304
Beniamini, P., and Piran, T. (2019). The gravitational waves merger time distribution of binary neutron star systems. Mon. Not. R. Astron. Soc. 487:4847. doi: 10.1093/mnras/stz1589
Berger, E. (2010). A short GRB “no-host” problem? Investigating large progenitor offsets for short GRBs with optical afterglows. New Astron. 55:1. doi: 10.1016/j.newar.2010.10.001
Bhat, N. (2010). GCN Circ., 10912. Available online at: https://gcn.gsfc.nasa.gov/other/100625A.gcn3
Bhat, N. P., Meegan, C. A., von Kienlin, A., Paciesas, W. S., Briggs, M. S., Burgess, J. M., et al. (2016). The third Fermi GBM gamma-ray burst catalog: the first six years. Astrophys. J. Suppl. 223:28. doi: 10.3847/0067-0049/223/2/28
Chruslinska, M., Belczynski, K., Klencki, J., and Benacquista, M. (2018). Double neutron stars: merger rates revisited. Mon. Not. R. Astron. Soc. 474, 2937–2958. doi: 10.1093/mnras/stx2923
Coward, D. M., Howell, E. J., Piran, T., Stratta, G., Branchesi, M., Bromberg, O., et al. (2012). The Swift short gamma-ray burst rate density: implications for binary neutron star merger rates. Mon. Not. R. Astron. Soc. 425, 2668–2673. doi: 10.1111/j.1365-2966.2012.21604.x
Eichler, D. (2018). Short gamma-ray bursts viewed from far off-axis. Astrophys. J. 869:L4. doi: 10.3847/2041-8213/aaec0d
Eichler, D., Livio, M., Piran, T., and Schramm, D. N. (1989). Nucleosynthesis, neutrino bursts and gamma-rays from coalescing neutron stars. Nature 340, 126–128. doi: 10.1038/340126a0
Feroz, F., Hobson, M. P., and Bridges, M. (2009). MULTINEST: an efficient and robust bayesian inference tool for cosmology and particle physics. Mon. Not. R. Astron. Soc. 398, 1601–1614. doi: 10.1111/j.1365-2966.2009.14548.x
Ghirlanda, G., Salafia, O. S., Pescalli, A., Ghisellini, G., Salvaterra, R., Chassande-Mottin, E., et al. (2016). Short gamma-ray bursts at the dawn of the gravitational wave area. Astron. Astrophys. 594:A84. doi: 10.1051/0004-6361/201628993
Goldstein, A., Veres, P., Burns, E., Briggs, M. S., Hamburg, R., Kocevski, D., et al. (2017). An ordinary short gamma-ray burst with extraordinary implications: fermi-GBM detection of GRB 170817A. Astrophys. J. 848:L14. doi: 10.3847/2041-8213/aa8f41
Gottlieb, O., Nakar, E., and Piran, T. (2018). The cocoon emission–an electromagnetic counterpart to gravitational waves from neutron star mergers. Mon. Not. R. Astron. Soc. 473, 576–584. doi: 10.1093/mnras/stx2357
Gottlieb, O., Nakar, E., and Piran, T. (2019). Detectability of neutron star merger afterglows. Mon. Not. R. Astron. Soc. 488:2405. doi: 10.1093/mnras/stz1906
Greiner, J., Burgess, J. M., Savchenko, V., and Yu, H.-F. (2016). On the Fermi-GBM event 0.4s after GW150914. Astrophys. J. 827, L38–L47. doi: 10.3847/2041-8205/827/2/L38
Guetta, D., and Piran, T. (2005). The luminosity and redshift distribution of short-duration GRBs. Astron. Astrophys. 435, 421–426. doi: 10.1051/0004-6361:20041702
Hopkins, A. M., and Beacom, J. F. (2006). On the normalization of the cosmic star formation history. Astrophys. J. 651, 142–154. doi: 10.1086/506610
Janka, H.-Th., Aloy, M.-A., Mazzali, P. A., and Pian, E. (2006). Off-axis properties of short gamma-ray bursts. Astrophys. J. 645, 1305–1314. doi: 10.1086/504580
Kasliwal, M. M., Nakar, E., Singer, L., Kaplan, D. L., Cook, D. O., Van Sistine, A., et al. (2017). Illuminating gravitational waves: a concordant picture of photons from a neutron star merger. Science 358, 1559–1565. doi: 10.1126/science.aap9455
Kathirgamaraju, A., Barnio Duran, R., and Giannios, D. (2018). Off-axis short GRBs from structured jets as counterparts to GW events. Mon. Not. R. Astron. Soc. 473, L121–L125. doi: 10.1093/mnrasl/slx175
Kathirgamaraju, A., Tchekhovskoy, A., Giannios, D., and Duran, R. B. (2019). EM counterparts of structured jets from 3D GRMHD simulations. Mon. Not. R. Astron. Soc. Lett. 484, L98–L103. doi: 10.1093/mnrasl/slz012
Krimm, H. A., Baumgartner, W. H., Beardmore, A. P., Chester, M. M., Evans, P. A., Gehrels, N., et al. (2017). GRB 101224A: Swift Detection of a Short Burst. GCN #11484.
Lazzati, D., Lopez-Camara, D., Cantiello, M., Morsony, B. J., Perna, R., and Workman, J. C. (2017). Off-axis prompt X-ray transients from the cocoon of short gamma-ray bursts. Astrophys. J. 848:L6. doi: 10.3847/2041-8213/aa8f3d
Li, L. X. (2008). Star formation history up to z=7.4: implication for gamma-ray bursts and cosmic metallicity evolution. Mon. Not. R. Astron. Soc. 388:1487. doi: 10.1111/j.1365-2966.2008.13488.x
Lipunov, V. M., Postnov, K. A., and Prokhorov, M. E. (1987). The sources of gravitational waves with continuous and secrete spectra. Astron. Astrophys. 176, L1–L4.
McBreen, S. (2010). GCN Circ., 11489. Available online at: https://gcn.gsfc.nasa.gov/other/101224.gcn3
Meegan, C., Lichti, G., Bhat, P. N., Bissaldi, E., Briggs, M. S., Connaughton, V., et al. (2009). The Fermi gamma-ray burst monitor. Astrophys. J. 709, 791–804. doi: 10.1088/0004-637X/702/1/791
Mooley, K. P., Deller, A. T., Gottlieb, O., Nakar, E., Hallinan, G., Bourke, S., et al. (2018). Superluminal motion of a relativistic jet in the neutron star merger GW 170817. Nature 561, 355–359. doi: 10.1038/s41586-018-0486-3
Piran, T., and Jimenez, R. (2014). Possible role of gamma ray bursts on life extinction in the universe. Phys. Rev. Lett. 113:231102. doi: 10.1103/PhysRevLett.113.231102
Planck Collaboration (2015). Planck 2015 results. XIII. Cosmological parameters. Astron. Astrophys. 594:A13. doi: 10.1051/0004-6361/201525830
Rau, A. (2009). GCN Circ., 9347. Available online at: https://gcn.gsfc.nasa.gov/other/090510.gcn3
Rhoads, J. E. (1997). How to tell a jet from a balloon: a proposed test for beaming in gamma-ray bursts. Astrophys. J. 487, L1–L4. doi: 10.1086/310876
Salafia, O. S., Ghisellini, G., Pescalli, A., Ghirlanda, G., and Nappo, F. (2016). Light curves and spectra from off-axis gamma-ray bursts. Mon. Not. R. Astron. Soc. 461, 3607–3619. doi: 10.1093/mnras/stw1549
Scargle, J., Norris, J. P., Jackson, B., and Chiang, J. (2013). Studies in astronomical time series analysis. VI. Bayesian block representations. Astrophys. J. 764, 167–193. doi: 10.1088/0004-637X/764/2/167
The LIGO Scientific Collaboration and Virgo Collaboration (2017a). Fermi GBM Trigger 524666471/170817529: LIGO/Virgo Identification of a Possible Gravitational-Wave Counterpart. GCN #21505.
The LIGO Scientific Collaboration and Virgo Collaboration (2017b). LIGO/Virgo Identification of a Binary Neutron Star Candidate. GCN #21509.
The LIGO Scientific Collaboration and Virgo Collaboration (2017c). LIGO/Virgo G298048: Further Analysis of a Binary Neutron Star Candidate With Updated Sky Localization. GCN #21513.
Troja, E., Piro, L., van Eerten, H., Wollaeger, R. T., Im, M., Fox, O. D., et al. (2017). The X-ray counterpart tot he gravitational-wave event GW 170817. Nature 551, 71–74. doi: 10.1038/nature24290
Troja, E., Ryan, G., Piro, L., van Eerten, H., Cenko, S. B., Yoon, Y., et al. (2018). A luminous blue kilonova and an off-axis jet from a compact binary merger at z = 0.1341. Nat. Commun. 9:4089. doi: 10.1038/s41467-018-06558-7
Vianello, G., Lauer, R. J., Younk, P., Tibaldo, L., Burgess, J. M., Ayala, H., et al. (2015). “The multi-mission maximum likelihood framework (3ML),” in PoS(ICRC2015)1042 (The Hague). doi: 10.22323/1.236.1042
Wanderman, D., and Piran, T. (2015). The rate, luminosity function and time delay of non-Collapsar short GRBs. Mon. Not. R. Astron. Soc. 448:3026. doi: 10.1093/mnras/stv123
Keywords: GRBs, GWs, Fermi, cosmology, data analysis
Citation: Burgess JM, Greiner J, Bégué D, Giannios D, Berlato F and Lipunov VM (2020) Viewing Short Gamma-Ray Bursts From a Different Angle. Front. Astron. Space Sci. 7:40. doi: 10.3389/fspas.2020.00040
Received: 16 October 2018; Accepted: 05 June 2020;
Published: 20 August 2020.
Edited by:
Miguel Alejandro Mostafa, Pennsylvania State University (PSU), United StatesReviewed by:
Stefano Covino, Brera Astronomical Observatory, ItalyRosa Poggiani, University of Pisa, Italy
Copyright © 2020 Burgess, Greiner, Bégué, Giannios, Berlato and Lipunov. This is an open-access article distributed under the terms of the Creative Commons Attribution License (CC BY). The use, distribution or reproduction in other forums is permitted, provided the original author(s) and the copyright owner(s) are credited and that the original publication in this journal is cited, in accordance with accepted academic practice. No use, distribution or reproduction is permitted which does not comply with these terms.
*Correspondence: J. Michael Burgess, amJ1cmdlc3NAbXBlLm1wZy5kZQ==; Jochen Greiner, amNnQG1wZS5tcGcuZGU=