- Department of Stellar Physics, Instituto de Astrofísica de Andalucía (IAA-CSIC), Consejo Superior de Investigaciones Científicas, Granada, Spain
Recent fully non-adiabatic theoretical studies of M dwarf models show that they have the potential to excite radial, and low-order, low-degree non-radial modes, as well as solar-like oscillations, due to models being completely convective or having large convective envelopes. The observational efforts aiming at discovering pulsating M dwarfs with photometric ground-based and space observations, and with high-precision spectroscopy are presented. With periods predicted in the 20 min to 3 h range and empirically estimated amplitudes of just a few μmag or a few tens of cm s−1, a clear detection of a pulsating M dwarf has not yet been achieved, and the race is still open. The precision attained by the latest-generation high-resolution spectrographs, of the order of cm s−1, may be the key to unveil the, until today, elusive pulsations in M dwarf stars.
1. Introduction
A quick look at the so-called pulsation Hertzsprung-Russell diagram (HRD) seems to show that all star classes, at a certain point in their evolution, sustain oscillations in their interior. However, a closer look, reveals certain areas which still lack the presence of intrinsic pulsators. One of them is the coolest edge of the main sequence, populated by low mass stars, and in particular, by M dwarfs (≃ 0.10–0.60 M⊙, Teff≃ 2,500–4,000 K).
M dwarfs are the most abundant class of stars, as it is estimated that they constitute more than 70% of the stars in our solar neighborhood (Henry et al., 2006). They are favorite targets of dedicated searches for small planets, both through transits or radial velocities, such as MEarth (Irwin et al., 2015) or CARMENES (Quirrenbach et al., 2018), due to favoring conditions for detection, thanks to their small sizes and low luminosities, compared to solar-like stars. The knowledge that our nearest M dwarf neighbors', Proxima and Barnard, also host planets (Anglada-Escudé et al., 2016; Ribas et al., 2018) has also contributed to the recent hype about this star class. More discoveries will surely be on the way, as statistical estimations derived from the planet yield of Kepler and K2 missions, point at 2.5 Earth to Neptune size planets per M dwarf in orbits closer than 200 d (Dressing and Charbonneau, 2015).
However, the physical parameters of M dwarfs are not easy to obtain and there are persistent discrepancies in theoretical estimations from stellar evolution models and observations regarding fundamental parameters, such as mass, radius and effective temperature in eclipsing binary systems, as well as in interferometry of single stars. The radius anomaly (see e.g., Tognelli et al., 2018) has been related to the effects of enhanced magnetic activity, a spotted stellar surface, or metallicity, which have been invoked to explain the observational-theoretical disagreement (see e.g., Higl and Weiss, 2017; Garrido et al., 2019; Kochukhov and Shulyak, 2019, and references therein).
The observational discovery of pulsating M dwarfs would make asteroseismology tools available to contribute to accomplish a better characterization of the fundamental parameters of M dwarf stars and of any orbiting planets, and would be the culmination of a search that started in the 1960's.
2. Asteroseismic Potential
The stability of low-mass stars has been theoretically explored initially by several authors, starting with Gabriel (1964), Gabriel (1967), Toma (1972), Boury and Noels (1973), Opoien and Grossman (1974), and Gabriel and Grossman (1977). They all reach the conclusion, through the evaluation of a vibrational stability coefficient in the adiabatic approximation, that the fundamental radial mode of low-mass stars may be excited by the nuclear burning of deuterium (D) or He3, either in the pre-main sequence or main sequence stages. The high dependence of the nuclear reactions on the temperature, which always has a destabilization effect on the modes, is known as the ϵ-mechanism. Besides, although neglecting the effects of convection, some of these studies also suggest that convection may also play a role in the driving of the modes. Noels et al. (1974) found that the ϵ-mechanism caused by He3 burning was at least partially responsible for the excitation of low-degree, low-order g-modes in 0.5 M⊙ models. However, none of these studies resolve the complete non-adiabatic pulsation equations, in which the energy equation is solved to evaluate the energy balance of the modes in each oscillation cycle.
More recently, the instability of M dwarfs was suggested by Baran et al. (2011b) based on unpublished theoretical analysis that found the excitation of the fundamental radial mode, in the 35–45 min period range, due to the ϵ-mechanism produced by He3 burning.
Rodríguez-López et al. (2012) (hereafter RL12) carried out the first non-radial, non-adiabatic pulsational study of M dwarf models in the 0.1–0.5 M⊙ mass range, solving the complete non-adiabatic pulsation equations, and finding the fundamental radial mode to be unstable in nearly the whole mass range. The ϵ-mechanism produced by D burning excited modes in the ~4–8 h period range, for young low-mass models of 0.1 and 0.2 M⊙. However, the probability of these modes to be observable is meager, as the time needed for their initial unknown amplitude to develop an e factor, known as the e-folding time τfold, is longer than the age of the model. Somehow in contradiction with this result, Palla and Baraffe (2005) in their non-adiabatic pulsating study of brown dwarfs found that the 0.1 M⊙ models with ages below 1.1 Myr had τfold shorter than the age of the model and hence being more favorable for detection. RL12 also found that older and higher mass models were excited in the ~25–40 min period range, with favorable τfold, being much more promising for detection. The ϵ-mechanism produced by He3 burning, and the so-called convective flux-blocking mechanism (Guzik et al., 2000), where the radiative flux coming from the interior is blocked at the tachocline in partially convective models, were found responsible for the driving of these modes.
In a subsequent paper, Rodríguez-López et al. (2014) (hereafter RL2014) repeated the instability analysis for an extended grid of models covering a larger and denser range of masses, 0.10–0.60 M⊙ with ΔM = 0.05 M⊙, using OPAL equation of state (Rogers and Nayfonov, 2002), solar and subsolar metallicities, different values of the mixing length parameter and models with boundary conditions given by PHOENIX NextGen atmospheres (Allard et al., 2000). The authors found two islands of instability (see Figure 1 and Table 1 for a recap):
• The first one, covering the whole range of masses, for models younger than 2 Myr, with the fundamental radial mode excited by the ϵ-mechanism fueled by D burning (periods ~4–11 h) and models younger than 50 Myr excited by the flux-blocking mechanism (periods ~1–2 h). The instability region is approximately enclosed by the ranges Teff = [2,800, 3,500] K, logg = [3.1, 3.6]. The unfavorable τfold, coupled to the high rotation rates expected for these young, pre-main-sequence models, still in their contraction phase, probably with a high flaring rate, make the detection of these oscillations very challenging.
• The second island of instability is defined by the physical parameters of models excited by the ϵ-mechanism produced by the He3 thermonuclear reactions, the flux-blocking mechanism or a combination of both. The instability region is coarsely limited by the ranges Teff = [3,300, 4,300] K, logg = [4.5, 5.1] (see Figure 1). The models cover the 0.20–0.60 M⊙ range in the main sequence, from completely to partially convective, with periods in the range 20 min to 1 h for the fundamental and low degree (ℓ = 0–3) low-order (k = 0–3) p- modes and 1–3 h for low-order low-degree g-modes.
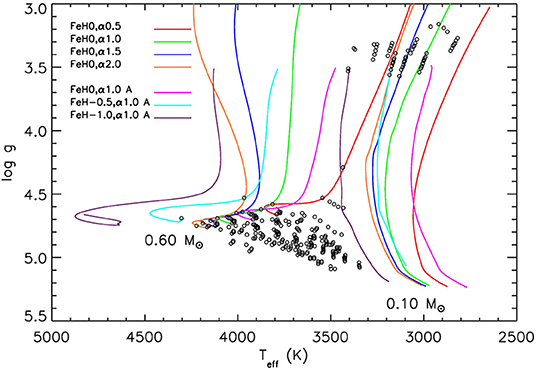
Figure 1. Evolutionary tracks of the 0.10 and 0.60M⊙ models of the different grids in RL14. Labels refer to FeH0 = solar metallicity; FeH-0.5 and FeH-1 subsolar metallicity, α = mixing length parameter, A = model with atmosphere. Open circles plot the location of the unstable models and define the instability strip in terms of Teff and logg. Figure from RL14.
Given that M dwarf models are completely convective for M ≲ 0.30–0.35M⊙ and for higher masses have convective envelopes that may reach about half of the star in radius, they have the potential to show solar-like oscillations, which are stochastically damped and excited by turbulent convective motions near the surface of the star. RL14 also presented a concise theoretical exercise to demonstrate the potential of M dwarfs as stochastic pulsators. Using a method involving the region of maximum available energy and location of the last antinode of a scaled radial eigenfunction, the authors found that solar metallicity models with a mixing length parameter α = 2, present stochastically excited modes with periods in the range of 2 min–1 h, for very young models with ages <50 Myr, while older models show oscillation periods below 2 min. In the light of these results, to accomplish an observational detection of solar-like oscillations in M dwarfs currently seems extremely challenging, due to the very short periods for M dwarfs in the main sequence, and the still short periods and complicated observational light-curves or radial velocity time-series, expected to be populated with activity effects for very young M dwarfs still in the contracting phase. However, this may be the perfect science case to gather new insights into the internal structure of M dwarfs with future instruments in extremely large telescopes, like HIRES (Marconi et al., 2018) at ELT.
Rodríguez et al. (2016) calculated, for representative M dwarf models in RL14, the periods at maximum power and amplitudes expected for the solar-like oscillations, from Corsaro et al. (2013) scaling relations, that had not been calibrated for M dwarfs. For models in the main sequence, periods at maximum power are in the 1–4 min range and corresponding tiny amplitudes in the 1–7 μmag range. Only for very young models, with ages below 2 Myr, they find periods at maximum power in the 30 min–1 h range and amplitudes in the 15–130 μmag range, more feasible from an observational point of view, but, as said above, with light curves expected to be plagued by other effects. It should be emphasized the good agreement of the periods with those derived by RL14 with a completely different method.
3. The Observational Quest
A specific photometric observational survey to look for M dwarf pulsations from the ground was carried out by Baran et al. (2011b), Krzesinski et al. (2012), and Baran et al. (2013) mainly at Mount Suhora Observatory. They surveyed a total of 120 M dwarfs, with spectral types M0V-M5V, typically for 1 night, that would cover several cycles of their expected pulsating periods between about 20 and 45 min. In most cases they reached a detection threshold of ~1 ppt, but no confirmed candidate was reported. Even if it is known from other pulsating stars that instability strips are not pure, and that rates of pulsating to no pulsating stars are low, from this ground-based study, should one pulsator be found, a ratio below 1% would be derived. This study made obvious that if M dwarf pulsations were to be detected, the photometric precision had to be increased.
The obvious next step was to take advantage of the extremely high precision provided by photometric space missions to search for these pulsations. Baran et al. (2011a) analyzed a sample of six M0V M dwarfs from Kepler short cadence database, with roughly 1 min sampling time, reaching detection thresholds of ~10 ppm and not detecting any pulsating M dwarf. Rodríguez-López et al. (2015) also analyzed four Kepler short cadence M dwarfs reaching detection thresholds in the range 2.5–16 ppm, again with non-positive detections.
Rodríguez et al. (2016) analyzed all 87 M dwarfs present in Kepler short cadence database, which covered all the cool and dwarf star list of Dressing and Charbonneau (2013). They analyzed the sample in search for thermodynamical and solar-like oscillations, reaching detection limits below 10 μmag for the brightest objects (~15%) and below 20 μmag for about 40% of the sample, while a threshold below 50 μmag was reached for an 80% of the sample. Again, no significant signal that could unequivocally be attributed to pulsations was found, and the yielded ratio of pulsators to non-pulsators, for a putative detection, still would be below 1%.
There are a plethora of other works in the literature not specifically searching for oscillations, but analyzing M dwarfs' photometric variability for the sake of finding planets, eclipsing binaries, or characterize the M dwarfs' variability in terms of rotation coupled to spots, flares or other photospheric or chromospheric activity. Among others, Hartman et al. (2011) analyzed the variability of K and M dwarfs from the HATNet transiting planet survey, Davenport et al. (2012) analyzed a sample of M dwarfs common to 2MASS and SDSS databases in search for flares in the NIR, Rappaport et al. (2014) analyzed high-precision Kepler photometry searching for M dwarfs' rapid rotators and just recently Zhan et al. (2019) analyzed TESS satellite (Ricker et al., 2015) 2-min cadence data to search for rapidly rotating M dwarfs; this only to name a very few studies on M dwarfs photometry. None of them attributed the encountered variability to pulsations.
Notwithstanding the null results of photometric searches, due to the low expected amplitudes, examples exists in the literature of pulsating stars which indicate that a signal of 10 μmag in photometry can have an equivalent amplitude of 1 m s−1 in radial velocities (see e.g., Zima et al., 2006), achievable nowadays by high-precision spectrographs. Despite the volume of radial velocity (RV) data of M dwarfs in the archives of these kind of instruments, such as HARPS (Bonfils et al., 2013), that do consistent searches for exoplanets around several types of stars, these archive RV time-series have long time baselines with a low time cadence, typically a few RV measurements per month, which are not useful to search for rapid pulsations in the time domain from around 20 min to 3 h, expected for main sequence M dwarfs.
Berdiñas et al. (2017) carried out the first search for M dwarfs pulsations using high-cadence, high-precision time-series spectroscopy obtained with HARPS, for which they derive an amplitude detection limit of ~0.5 m s−1 to achieve a confidence level of 90% for the detection of M dwarfs pulsations. They present results for the two most long-term stable stars in their sample, for which they found no signals with false alarm probability (FAP) below 1% in the expected range of pulsations. Nonetheless, they report some power excess for the two targets, which could be due to non-resolved oscillations, one of them with a 2 h period and amplitude as low as 0.36 m s−1, compatible with the theoretical prediction for low-radial, low-order g-modes, for a model with the same physical parameters as the target.
Berdiñas et al. (2019) reported preliminary results for the on-going Beating Red Dots (BRD) project, aimed at discovering pulsating M dwarfs with high-resolution spectrographs HARPS and HARPS-N (Cosentino et al., 2012). They acquired more observations for the pulsating candidate in Berdiñas et al. (2017), but were not able to confirm the oscillations, as the simulations predicted they would, with the acquisition of the new data in the carefully programmed observing sequence and cadence. This may be due to amplitude variability effects, as still some power remained at the same frequency, showing that the noise is not white. They reported no positive results for a second target and a signal at 4.7 h with FAP <1% which still requires further investigation by the BRD project, which is still on-going.
4. Discussion and Conclusions
Fully non-adiabatic theoretical studies predict the excitation of radial and non-radial modes in M dwarf models. All the non-adiabatic code of oscillations available in the community, such as GRACO (Moya and Garrido, 2008) or GYRE (Townsend and Teitler, 2013) are linear, i.e., the pulsation equations are linearized, so that the gain is that they are approachable to resolve, but the drawback is that the amplitude of the oscillations is unknown. The best we can do is to estimate how long it takes for an initial unknown mode amplitude to grow an e factor, which is known as the e-folding time, τfold. When this time is shorter than the age of the model, the mode has enough time to grow its initial amplitude and hence it is more favored to be excited.
The τfold for excited young pre-main sequence M dwarf models, either by the ϵ mechanism (ages <2 Myr, periods 4–11 h) fueled by D-burning or by the flux-blocking mechanism (ages <50 Myr, periods 1–2 h) is much longer than the age of the models. It seems natural, as the ϵ-mechanism operates in the center of the star, where the amplitudes of the modes are expected to be small, that modes have difficulties in overcoming all other damping regions throughout the star and show observable amplitudes on the surface. Up to now, only a few of objects seems to show oscillations attributed to the ϵ-mechanism (e.g., Moravveji et al., 2012; Battich et al., 2018), although some debate still exists about if this attribution is conclusive.
The best potential for M dwarfs to show observable oscillations, with τfold shorter than the age of the models, lie in the theoretical predictions for main sequence models with periods in the 20 min to 3 h range, due to either the ϵ-mechanism of He3 nuclear burning, the convective flux-blocking mechanism or a combination of both. Dedicated photometric searches with high-precision, high time-cadence Kepler space data, have put stringent limits to the amplitudes, which should be in the range of a few μmag (or ppm in flux), while the ratio of pulsators to non-pulsators would be below ~1%, should the first pulsator be found. This ratio might somewhat be improved if only targets within the predicted instability strip were considered.
However, low ratios have also been derived for other pulsating classes within the instability strip, such under 10% for fast sdBV (Østensen et al., 2011) or a 40% for δ Sct (Balona, 2018a). This co-existence of pulsators and non-pulsators within the theoretical and empirical instability strips is one of the open questions of asteroseismology. In particular, Balona (2018b) has recently proposed a yet unknown physical factor which would account for mode selection, and eventually suppression, in δ Sct and γ Dor stars, explaining the low pulsators-non pulsators ratio and coexistence.
The potential for solar-like excitation has also been noted due to M dwarfs being completely convective or having large convective envelopes, and it has been explored with Kepler short cadence data by Rodríguez et al. (2016) with no positive detection. The short predicted periods, of just a couple of minutes, and the tiny expected amplitudes, of a few μmag, render the observational detection of stochastically excited modes in M dwarfs, as of today, somewhat challenging.
The exploration of the possibility of finding pulsating M dwarfs through high-resolution spectroscopic time-series has been up to now, nearly as scarce as the scanty dedicated photometric surveys. One of the reasons is the extremely high time-cadence needed to sample the 20 min to 3 h pulsating range, coupled to the high competition and cost of observing nights at medium class telescopes. Berdiñas et al. (2017) derived that a pulsating signal with a 0.5 m/s RV amplitude could be detected with a 90% confidence with four continuous nights of observations. Unfortunately, these amount of nights are not easily awarded for exploratory programs.
However, although the photometric detection is still worth pursuing with the present and forthcoming wealth and quality of data from TESS space mission, the chase of pulsating M dwarfs seems to be more promising with the recently available and future high-resolution high-precision spectrographs, such as ESPRESSO (Pepe et al., 2014) and HIRES (Marconi et al., 2018). ESPRESSO promises an intrinsic RV precision of just 10 cm s−1, so the precision needed for a detection of a pulsating M dwarf will no longer be determined by the spectrograph, but by the intrinsic activity of the star, so a putative detection would benefit from a careful selection of the quietest targets.
The fact that non-unambiguous detection of pulsating M dwarfs has not yet been made, may be due to different reasons that have been discussed, e.g., in Rodríguez et al. (2016), namely: (i) the surveyed sample is not statistically significant, especially in the spectroscopic search, and considering that instability strips are not pure, (ii) the low amplitude of the oscillations have prevented a detection, and the pulsating signal may be embedded in the noise, (iii) we also should bear in mind the possibility that M dwarfs, after all, do not pulsate. However, despite limitations in the physics of the models and in the codes of oscillations, theoretical predictions made with the same codes and physics have proven correct for other stellar types.
A lot remains to be gained from the observational discovery of pulsations in M dwarfs: a precise an independent determination of their fundamental parameters and size of the convection zone will help to refine stellar evolution models and to solve radii and temperature discrepancies in theory and observations. Moreover, the refinement in stellar parameters will also increase the precision of fundamental parameters of accompanying exoplanets of M dwarf hosts. Other exciting possibilities if solar-like pulsations are detected is that they could help to constrain the nature of dark matter (Casanellas, 2015) or contribute to the detection of gravitational waves (Lopes and Silk, 2015) with future instrumentation.
Author Contributions
The author confirms being the sole contributor of this work and has approved it for publication.
Funding
CR-L acknowledges financial support from the State Agency for Research of the Spanish MCIU through the Center of Excellence Severo Ochoa award for the Instituto de Astrofísica de Andalucía (SEV-2017-0709) and project AYA2016-79425-C03-03P.
Conflict of Interest
The author declares that the research was conducted in the absence of any commercial or financial relationships that could be construed as a potential conflict of interest.
References
Allard, F., Hauschildt, P. H., and Schweitzer, A. (2000). Spherically symmetric model atmospheres for low-mass pre-main-sequence stars with effective temperatures between 2000 and 6800 K. Astrophys. J. 539, 366–371. doi: 10.1086/309218
Anglada-Escudé, G., Amado, P. J., Barnes, J., Berdiñas, Z. M., Butler, R. P., Coleman, G. A. L., et al. (2016). A terrestrial planet candidate in a temperate orbit around Proxima Centauri. Nature 536, 437–440. doi: 10.1038/nature19106
Balona, L. A. (2018a). Gaia luminosities of pulsating A-F stars in the Kepler field. Monthly Notices R. Astron. Soc. 479, 183–191. doi: 10.1093/mnras/sty1511
Balona, L. A. (2018b). Pulsation in intermediate-mass stars. Front. Astron. Space Sci. 5:43. doi: 10.3389/fspas.2018.00043
Baran, A. S., Fox-Machado, L., Lykke, J., Nielsen, M., and Telting, J. H. (2011a). Mt. Suhora survey—searching for pulsating M dwarfs in Kepler public dataset. Acta Astron. 61, 325–343. Available online at: https://ui.adsabs.harvard.edu/abs/2011AcA….61.325B/abstract
Baran, A. S., Winiarski, M., Krzesiński, J., Fox-Machado, L., Kawaler, S. D., Dróżdz, M., et al. (2011b). Mt. Suhora survey—searching for pulsating M dwarfs. I. Acta Astron. 61, 37–58. Available online at: https://ui.adsabs.harvard.edu/abs/2011AcA….61…37B/abstract
Baran, A. S., Winiarski, M., Siwak, M., Fox-Machado, L., Kozieł-Wierzbowska, D., Krzesinski, J., et al. (2013). Mt. Suhora survey—searching for pulsating M dwarfs. III. Acta Astron. 63, 41–52. Available online at: https://ui.adsabs.harvard.edu/abs/2013AcA….63…41B/abstract
Battich, T., Miller Bertolami, M. M., Córsico, A. H., and Althaus, L. G. (2018). Pulsational instabilities driven by the ε mechanism in hot pre-horizontal branch stars. I. The hot-flasher scenario. Astron. Astrophys. 614:A136. doi: 10.1051/0004-6361/201731463
Berdiñas, Z. M., Rodríguez, E., Amado, P. J., Rodríguez-López, C., Anglada-Escudé, G., and Jenkins, J. S. (2019). “Do M dwarfs pulsate? The search with the beating red dots project using HARPS,” in Highlights on Spanish Astrophysics X, Proceedings of the XIII Scientific Meeting of the Spanish Astronomical Society held on July 16–20, 2018, eds B. Montesinos, A. Asensio Ramos, F. Buitrago, R. Schödel, E. Villaver, S. Pérez-Hoyos, et al. (Salamanca), 266–271.
Berdiñas, Z. M., Rodríguez-López, C., Amado, P. J., Anglada-Escudé, G., Barnes, J. R., MacDonald, J., et al. (2017). High-cadence spectroscopy of M-dwarfs - II. Searching for stellar pulsations with HARPS. Monthly Notices R. Astron. Soc. 469, 4268–4282. doi: 10.1093/mnras/stx1140
Bonfils, X., Delfosse, X., Udry, S., Forveille, T., Mayor, M., Perrier, C., et al. (2013). The HARPS search for southern extra-solar planets. XXXI. The M-dwarf sample. Astron. Astrophys. 549:A109. doi: 10.1051/0004-6361/201014704
Boury, A., and Noels, A. (1973). Pulsational instability of a Star of 0.5 M0 during core hydrogen burning. Astron. Astrophys. 24:255.
Casanellas, J. (2015). “Dark matter searches with asteroseismology,” in European Physical Journal Web of Conferences, Volume 101 of European Physical Journal Web of Conferences (Toulouse), 01015.
Corsaro, E., Fröhlich, H.-E., Bonanno, A., Huber, D., Bedding, T. R., Benomar, O., et al. (2013). A Bayesian approach to scaling relations for amplitudes of solar-like oscillations in Kepler stars. Monthly Notices R. Astron. Soc. 430, 2313–2326. doi: 10.1093/mnras/stt059
Cosentino, R., Lovis, C., Pepe, F., Collier Cameron, A., Latham, D. W., Molinari, E., et al. (2012). “Harps-N: the new planet hunter at TNG,” in Ground-Based and Airborne Instrumentation for Astronomy IV, Volume 8446 of Proceedings of SPIE (Amsterdam), 84461V.
Davenport, J. R. A., Becker, A. C., Kowalski, A. F., Hawley, S. L., Schmidt, S. J., Hilton, E. J., et al. (2012). Multi-wavelength characterization of stellar flares on low-mass stars using SDSS and 2MASS time-domain Surveys. Astrophys. J. 748:58. doi: 10.1088/0004-637X/748/1/58
Dressing, C. D., and Charbonneau, D. (2013). The occurrence rate of small planets around small stars. Astrophys. J. 767:95. doi: 10.1088/0004-637X/767/1/95
Dressing, C. D., and Charbonneau, D. (2015). The occurrence of potentially habitable planets orbiting M dwarfs estimated from the full Kepler dataset and an empirical measurement of the detection sensitivity. Astrophys. J. 807:45. doi: 10.1088/0004-637X/807/1/45
Gabriel, M. (1964). La stabilité vibrationnelle de kruger 60 A et des naines rouges. Ann. Astrophys. 27:141.
Gabriel, M. (1967). Structure et stabilité vibrationnelle des naines rouges entièrement convectives. Ann. Astrophys. 30:745.
Gabriel, M., and Grossman, A. S. (1977). The pulsational stability of very low mass stars on the hydrogen and deuterium main sequences. Astron. Astrophys. 54, 283–290.
Garrido, H. E., Cruz, P., Diaz, M. P., and Aguilar, J. F. (2019). The radius inflation problem in short-period low-mass binaries: a large sample analysis. Monthly Notices R. Astron. Soc. 482, 5379–5388. doi: 10.1093/mnras/sty3006
Guzik, J. A., Kaye, A. B., Bradley, P. A., Cox, A. N., and Neuforge, C. (2000). Driving the gravity-mode pulsations in γ Doradus variables. Astrophys. J. Lett. 542, L57–L60. doi: 10.1086/312908
Hartman, J. D., Bakos, G. Á., Noyes, R. W., Sipőcz, B., Kovács, G., Mazeh, T., et al. (2011). A photometric variability survey of field K and M dwarf stars with HATNet. Astron. J. 141:166. doi: 10.1088/0004-6256/141/5/166
Henry, T. J., Jao, W.-C., Subasavage, J. P., Beaulieu, T. D., Ianna, P. A., Costa, E., et al. (2006). The solar neighborhood. XVII. Parallax results from the CTIOPI 0.9 m program: 20 new members of the RECONS 10 parsec sample. Astron. J. 132, 2360–2371. doi: 10.1086/508233
Higl, J., and Weiss, A. (2017). Testing stellar evolution models with detached eclipsing binaries. Astron. Astrophys. 608:A62. doi: 10.1051/0004-6361/201731008
Irwin, J. M., Berta-Thompson, Z. K., Charbonneau, D., Dittmann, J., Falco, E. E., Newton, E. R., et al. (2015). “The MEarth-north and MEarth-south transit surveys: searching for habitable super-earth exoplanets around nearby M-dwarfs,” in 18th Cambridge Workshop on Cool Stars, Stellar Systems, and the Sun, Volume 18 of Cambridge Workshop on Cool Stars, Stellar Systems, and the Sun, eds G. T. van Belle and H. C. Harris (Flagstaff, AZ), 767–772.
Kochukhov, O., and Shulyak, D. (2019). Magnetic field of the eclipsing M-dwarf binary YY gem. Astrophys. J. 873:69. doi: 10.3847/1538-4357/ab06c5
Krzesinski, J., Baran, A. S., Winiarski, M., Fox-Machado, L., Dróżdż, M., Siwak, M., et al. (2012). Mt. Suhora survey—searching for pulsating M dwarfs. II. Acta Astron. 62, 201–212. Available online at: https://ui.adsabs.harvard.edu/abs/2012AcA….62.201K/abstract
Lopes, I., and Silk, J. (2015). Nearby stars as gravitational wave detectors. Astrophys. J. 807:135. doi: 10.1088/0004-637X/807/2/135
Marconi, A., Allende Prieto, C., Amado, P. J., Amate, M., Augusto, S. R., Becerril, S., et al. (2018). “ELT-HIRES, the high resolution spectrograph for the ELT: results from the Phase A study,” in Ground-based and Airborne Instrumentation for Astronomy VII, Volume 10702 of Society of Photo-Optical Instrumentation Engineers (SPIE) Conference Series (Austin, TX), 107021Y.
Moravveji, E., Moya, A., and Guinan, E. F. (2012). Asteroseismology of the nearby SN II progenitor rigel. II. epsilon-mechanism triggering gravity-mode pulsations? Astrophys. J. 749:74. doi: 10.1088/0004-637X/749/1/74
Moya, A., and Garrido, R. (2008). Granada oscillation code (GraCo). Astrophys. Space Sci. 316, 129–133. doi: 10.1007/s10509-007-9694-2
Noels, A., Boury, A., Scuflaire, R., and Gabriel, M. (1974). Pulsational instability towards non-radial oscillations in homogeneous stars of small mass. Astron. Astrophys. 31:185.
Opoien, J. W., and Grossman, A. S. (1974). An analysis of the pulsational stability of very low mass stars on the hydrogen and deuterium main sequence. Astron. Astrophys. 37, 335–338.
Østensen, R. H., Silvotti, R., Charpinet, S., Oreiro, R., Bloemen, S., Baran, A. S., et al. (2011). First Kepler results on compact pulsators—VI. Targets in the final half of the survey phase. Monthly Notices R. Astron. Soc. 414, 2860–2870. doi: 10.1111/j.1365-2966.2011.18405.x
Palla, F., and Baraffe, I. (2005). Pulsating young brown dwarfs. Astron. Astrophys. 432, L57–L60. doi: 10.1051/0004-6361:200500020
Pepe, F., Molaro, P., Cristiani, S., Rebolo, R., Santos, N. C., Dekker, H., et al. (2014). ESPRESSO: the next European exoplanet hunter. Astron. Nachr. 335:8. doi: 10.1002/asna.201312004
Quirrenbach, A., Amado, P. J., Ribas, I., Reiners, A., Caballero, J. A., Seifert, W., et al. (2018). “CARMENES: high-resolution spectra and precise radial velocities in the red and infrared,” in Ground-based and Airborne Instrumentation for Astronomy VII, Volume 10702 of Society of Photo-Optical Instrumentation Engineers (SPIE) Conference Series (Austin, TX), 107020W.
Rappaport, S., Swift, J., Levine, A., Joss, M., Sanchis-Ojeda, R., Barclay, T., et al. (2014). M-dwarf rapid rotators and the detection of relatively young multiple M-star systems. Astrophys. J. 788:114. doi: 10.1088/0004-637X/788/2/114
Ribas, I., Tuomi, M., Reiners, A., Butler, R. P., Morales, J. C., Perger, M., et al. (2018). A candidate super-earth planet orbiting near the snow line of Barnard's star. Nature 563, 365–368. doi: 10.1038/s41586-018-0677-y
Ricker, G. R., Winn, J. N., Vanderspek, R., Latham, D. W., Bakos, G. Á., Bean, J. L., et al. (2015). Transiting exoplanet survey satellite (TESS). J. Astron. Telesc. Instr. Syst. 1:014003. doi: 10.1117/1.JATIS.1.1.014003
Rodríguez, E., Rodríguez-López, C., López-González, M. J., Amado, P. J., Ocando, S., and Berdiñas, Z. M. (2016). Search for pulsations in M dwarfs in the Kepler short-cadence data base. Monthly Notices R. Astron. Soc. 457, 1851–1863. doi: 10.1093/mnras/stw033
Rodríguez-López, C., Gizis, J. E., MacDonald, J., Amado, P. J., and Carosso, A. (2015). M dwarf search for pulsations within Kepler guest observer programme. Monthly Notices R. Astron. Soc. 446, 2613–2620. doi: 10.1093/mnras/stu2211
Rodríguez-López, C., MacDonald, J., Amado, P. J., Moya, A., and Mullan, D. (2014). The theoretical instability strip of M dwarf stars. Monthly Notices R. Astron. Soc. 438, 2371–2379. doi: 10.1093/mnras/stt2352
Rodríguez-López, C., MacDonald, J., and Moya, A. (2012). Pulsations in M dwarf stars. Monthly Notices R. Astron. Soc. 419, L44–L48. doi: 10.1111/j.1745-3933.2011.01174.x
Rogers, F. J. and Nayfonov, A. (2002). Updated and expanded OPAL equation-of-state tables: implications for helioseismology. Astrophys. J. 576, 1064–1074. doi: 10.1086/341894
Tognelli, E., Prada Moroni, P. G., and Degl'Innocenti, S. (2018). Theoretical uncertainties on the radius of low- and very-low-mass stars. Monthly Notices R. Astron. Soc. 476, 27–42. doi: 10.1093/mnras/sty195
Toma, E. (1972). Pulsational instability of stars during deuterium burning. Astron. Astrophys. 19:76.
Townsend, R. H. D., and Teitler, S. A. (2013). GYRE: an open-source stellar oscillation code based on a new Magnus multiple shooting scheme. Monthly Notices R. Astron. Soc. 435, 3406–3418. doi: 10.1093/mnras/stt1533
Zhan, Z., Günther, M. N., Rappaport, S., Oláh, K., Mann, A., Levine, A. M., et al. (2019). Complex rotational modulation of rapidly rotating M-stars observed with TESS. arXiv. doi: 10.3847/1538-4357/ab158c
Zima, W., Wright, D., Bentley, J., Cottrell, P. L., Heiter, U., Mathias, P., et al. (2006). A new method for the spectroscopic identification of stellar non-radial pulsation modes. II. Mode identification of the δ Scuti star FG Virginis. Astron. Astrophys. 455, 235–246. doi: 10.1051/0004-6361:20064877
Keywords: low-mass stars, M dwarf stars, stellar pulsations, stellar oscillations, asteroseismology
Citation: Rodríguez-López C (2019) The Quest for Pulsating M Dwarf Stars. Front. Astron. Space Sci. 6:76. doi: 10.3389/fspas.2019.00076
Received: 28 March 2019; Accepted: 02 December 2019;
Published: 17 December 2019.
Edited by:
Joyce Ann Guzik, Los Alamos National Laboratory (DOE), United StatesReviewed by:
Andrzej S. Baran, Pedagogical University of Kraków, PolandWolfgang Brandner, Max-Planck-Institut für Astronomie, Germany
Copyright © 2019 Rodríguez-López. This is an open-access article distributed under the terms of the Creative Commons Attribution License (CC BY). The use, distribution or reproduction in other forums is permitted, provided the original author(s) and the copyright owner(s) are credited and that the original publication in this journal is cited, in accordance with accepted academic practice. No use, distribution or reproduction is permitted which does not comply with these terms.
*Correspondence: Cristina Rodríguez-López, crl@iaa.es