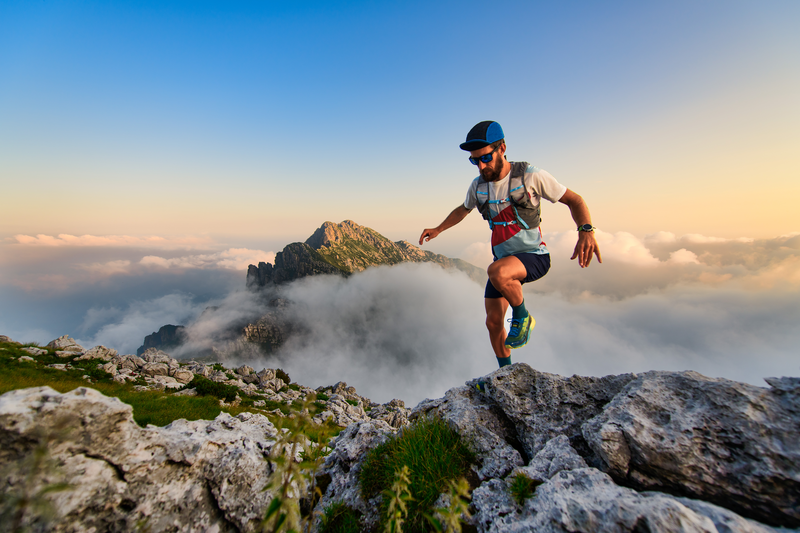
95% of researchers rate our articles as excellent or good
Learn more about the work of our research integrity team to safeguard the quality of each article we publish.
Find out more
PERSPECTIVE article
Front. Astron. Space Sci. , 26 June 2018
Sec. Stellar and Solar Physics
Volume 5 - 2018 | https://doi.org/10.3389/fspas.2018.00021
This article is part of the Research Topic Imagining the Future of Astronomy and Space Science View all 10 articles
To make transformational scientific progress with the space weather enterprise the Sun, Earth, and heliosphere must be studied as a coupled system, comprehensively. Rapid advances were made in the study, and forecasting, of terrestrial meteorology half a century ago that accompanied the dawn of earth observing satellites. Those assets provided a global perspective on the Earth's weather systems and the ability to look ahead of the observer's local time and to. From a heliospheric, or space, weather perspective we have the same fundamental limitation as the terrestrial meteorologists had—by far the majority of our observing assets are tied to the Sun-Earth line—our planet's “local time” with respect to the Sun. This perspective intrinsically limits our ability to “see what is coming around the solar limb” far less to gain any insight into the global patterns of solar weather and how they guide weather throughout the heliosphere. We propose a mission concept—the Heliospheric Meteorology Mission (HMM)—to sample the complete magnetic and thermodynamic state of the heliosphere inside 1AU using a distributed network of deep space hardened smallsats that encompass the Sun. The observations and in situ plasma measurements made by the fleet of HMM smallsats would be collected, and assimilated into current operational space weather models. Further, the HMM measurements would also being used in an nationally coordinated research effort—at the frontier of understanding the coupled heliospheric system—as a means to develop the next generation models required to provide seamless prediction for the geospace environment to protect vital infrastructure and human/robotic explorers throughout the solar system. The HMM mission concept naturally allows for research-motivated technology development that can improve forecast skill.
It is widely acknowledged that the discipline of space weather forecasting is five to six decades behind its terrestrial equivalent (e.g., Schrijver et al., 2015). Advances in the latter were precipitated by observational advances where data from beyond one local time frame (e.g., Holton, 1992) and, at the dawn of the satellite era, when global perspectives of tropospheric weather systems (e.g., Wexler, 1962) were brought to bear. One significant advance in the interpretation of meteorological data followed the identification of Rossby waves in the upper stratosphere (Rossby, 1939) and the recognition that local weather disturbances are intrinsically tied to these global-scale weather patterns (Gray, 1968). This determination led to significant advances in short-, mid-, and long-term forecasting skill (e.g., Thompson, 1983; Win-Nielsen, 1991) and a perception that terrestrial storms, once considered to be intrinsically unpredictable, conceptually transitioned to an intermittent part of a global system that was predictable to an acceptable degree (e.g., Lorenz, 1973). The challenge to the solar physics and space weather community is simple—can the methodology and heritage of terrestrial meteorology be utilized to advance forecasting of the solar end of the sun-earth system through complete observations of the Sun's magnetized atmosphere? What follows is just one possible approach to closing that “60 year” gap.
The Heliospheric Meteorology Mission (hereafter, HMM) directly addresses the primary goal of the 2013 Heliophysics Decadal Survey (NRC, 2013) “Determine the origins of the Sun's activity and predict the variations of the space environment” while also offering considerable insight into how the geospace system responds through the second “Determine the dynamics and coupling of Earth's magnetosphere, ionosphere, and atmosphere and their response to solar and terrestrial inputs.” Fundamental knowledge of our heliospheric evolution developed through the hand-in-hand combination of observation and modeling with HMM will inform other community models and “Determine the interaction of the Sun with the solar system and the interstellar medium” and to look beyond for analogies with comparative heliospheres and their interactions with exoplanet atmospheres “Discover and characterize fundamental processes that occur both within the heliosphere and throughout the universe.” HMM implicitly diversifies observing capability and extends the current Sun-Earth line based ground and space-based assets. The integration of operations, data analysis, and advanced numerical modeling will realize the scientific potential of the mission. The integrated observations provided by HMM, the flow of technology, and provision of operationally vital data will strengthen ties between agencies and agency disciplines. Research and operational insights will direct instrument and technology developments built into new payloads that permit the enterprise to venture forward and remain vital in the operational and research domains. Finally, the continuous streams of data analysis and assimilation, advanced system modeling and hardware developments will foster and environment of education and inspiration for the next generation of engineers and scientists. As such, HMM realizes the vision of the DRIVE initiative of the Decadal Survey (See section 4 of NRC, 2013).
Since the 2006 launch of the twin Solar-Terrestrial RElations Observatory (STEREO; Howard et al., 2008) satellites and the Solar Dynamics Observatory (SDO; Lemen et al., 2012) in 2010, a picture of globally connected solar phenomenology has developed—the idea that emergence of magnetic flux in one location can trigger an event on the other side of the Sun. Furthermore, spatial and temporal relationships in the pattern of magnetic flux emergence have revealed that the Sun undergoes quasi-annual episodes of significantly enhanced activity of an amplitude equivalent to the more familiar solar magnetic cycle (McIntosh et al., 2014, 2015, 2017). These well-studied stronglylongitudnal flux emergence processes (e.g., Carrington, 1858; Castenmiller et al., 1986; Brouwer and Zwaan, 1990), appear to be driven by magneto-Rossby modes on the global scale (e.g., Zaqarashvili et al., 2015; Gurgenashvili et al., 2017; Dikpati et al., 2017, 2018; Löptien et al., 2018) which then force significant increases in the production of active regions (with the flares and Coronal Mass Ejections— CMEs— that result). Further, these flux emergence episodes also drive significant modulation in the short-wavelength radiative output and shape the magnetic skeleton of the heliosphere that also ducts the solar wind (e.g., McIntosh et al., 2015; Krista et al., 2018).
Comparing periods of enhanced solar activity with the occurrence of most destructive of solar storms (see e.g., Figure 1) we deduce that both are intrinsically tied to these active solar longitudes. Those active solar longitudes persist for many solar rotations (e.g., McIntosh et al., 2017) with periodic surges of the timescale of 11-ish months (e.g., McIntosh et al., 2015). Therefore, monitoring, understanding and forecasting the origin of these active longitudes is of vital importance in the context of short and long-term forecasting for the geospace and heliospheric environment, in addition to the protection of vital space and ground infrastructure. From our current, limited, vantage point we will not gain insight into these processes and, as a result, solar storms will remain high-frequency intrinsically unpredictable events, i.e., exactly where terrestrial meteorology was half a century ago with respect to the predictability of extreme weather events at the dawn of the space age and prior to global observations of our planet's atmosphere.
Figure 1. A timeline representation of the major flare record of the past two solar cycles, with the daily hemispheric sunspot number. Without the effects of smoothing filters, the quasi-annual Rossby-mode-driven surges in solar magnetic activity are clear, as is the lack of hemispheric synchronization. (The largest surge without a large flare reported by GOES is that in mid 2012 in the southern hemisphere—which was responsible for the July 23 event). The inset shows the “Butterfly” (latitude vs. time) diagram of extreme-ultraviolet brightpoints (e.g., McIntosh et al., 2014) where several episodes of enhanced activity in each solar hemisphere are also clearly visible.
From the previous section, it would appear that in order to significantly advancing our knowledge of the Sun's atmosphere and its evolution on timescales of months, weeks, and years that we must invest in an observing strategy for the entire solar atmosphere. Such a “meteorological” observing strategy may rapidly close the many decades of lag in understanding— hence the “Heliospheric Meteorological Mission.”
In a proverbial nutshell, the HMM objective would be to fly a stream of fixed-design deep space hardened/validated smallsat spacecraft in orbits off the Sun-Earth line to monitor the 360 degree environment of the solar atmosphere and the heliosphere extending from it. These smallsats would have internal guidance, propulsion, and communications assets. Each spacecraft would be equipped with a number of well characterized, compact instruments belonging to a range of “plug-n-play” instrumentation and/or relay/transmitter equipment. These instruments would be supplied by the institutions in the community according to their particular technological heritage (see below). The data from the HMM fleet of spacecraft will provide information on solar magnetism, the outer solar atmosphere, and in situ magnetic, thermodynamic, particulate and radiative environment. The community would decide on the optimal flight arrangement, including the arrangement of necessary relay platforms required to collect the data. The HMM fleet data will be an invaluable resource for the research community, model developers, and operational agencies where they can serve a dual purpose of being assimilated into current space weather forecast models while motivating advances required for next-generation forecast capability. HMM provides an observing strategy that is crucially missing from our current capability in a low-risk, high-heritage, and replaceable manner that engages and enables the entire national (and international) heliophysics community.
The HMM concept engages and enriches the operational, research, and technology communities in one effort to understand the meteorology of the Sun and Heliosphere. The observations acquired by the HMM fleet would be designed for direct assimilation into next-generation space weather forecast models—providing vital information about the global magnetic environment necessary for research efforts to understand the solar dynamo and other basic processes, drive surface (magnetic) flux transport models, and the current operational forecast models in addition to particular insight into flux distributions around the East limb that will become geo-effective in short order—in short, eliminating several scientifically strategic shortcomings. The broad distribution of the HMM in situ measurements can be used as validation points for those models of the magneto-thermal environment of the sub-1AU heliosphere. The technology enrichment comes from the distribution of construction efforts and the ability to adapt transforming technologies as they feedback from modeling and operational requirements.
The HMM concept would directly benefit multiple federal agencies—DoD, FAA, NASA, NOAA, and NSF—with space weather requirements. NASA and NSF would play a vital role in the fundamental understanding of the observational data and technology transfer in these compact, hardened, payloads. Furthermore, the data provided will be invaluable in assessing risk and providing ongoing evaluation of that risk for upcoming human missions away from our near-Earth space. The operational data and forecast model improvements that are driven by HMM will be of direct benefit to Space Weather Forecast operations in the commercial, military, and civilian domains.
The HMM concept could, and maybe should, seek instrument contributions (like those below) from communities outside of the US where expertise exists. Again, the priority would go to high-heritage observations that are well-understood from the present perspective.
HMM requires a smallsat platform, due to present restrictions on launch capability. Indeed, HMM can build off of the “Solar Weather Buoys” and “Telemachus” concept missions that were presented in the 2005 NASA Heliophysics Roadmap—slated for launch in 2022 (Hoeksema, 2005). The individual instrument engineering for HMM could, and maybe should, be distributed toward a common instrument interface on the spacecraft to significantly reduce cost. At time of writing several engineering and aerospace groups are exploring such spacecraft designs.
As stated above HMM must leverage high-heritage, compact instrument packages. In this way the vantage point is the main, or only, element changed—the measurements are (relatively) well-understood. The Telemachus and Solar Weather Buoys missions built off of this concept, except for the application of compact imagers. Over the intervening 15 years since the development of that roadmap we've made tremendous strides in instrument miniaturization. So, in terms of a baseline payload, each HMM spacecraft would have one or more of the following:
⋆ A compact Doppler magnetograph, more compact that SOHO/MDI and or SDO/HMI. For example, Magneto-Optical Filter based designs have been considered for mission concepts like than on INSPIRE (Klesh, 2018).
⋆ A compact white light coronagraph and/or heliospheric imager suite like those implemented on the Parker Solar Probe, or Solar Orbiter missions.
⋆ An in situ space plasma suite, such as the EPSS package of the Messenger mission (e.g., Andrews et al., 2007), or the SWEAP experiments on the Parker Solar Probe (e.g., Kasper et al., 2016).
⋆ A compact magnetometer to characterize the variability in the interplanetary vector field (e.g., Anderson et al., 2007; Bale et al., 2016).
⋆ A compact EUV Imager like that implemented in the SWAP payload on the ESA PROBA mission (Berghmans et al., 2006).
In terms of the primary mission, characterizing the global magnetic environment of the Sun, the Doppler magnetograph would be the minimum payload requirement of the mission fleet, likely leveraging the experioences of the Solar Orbiter PHI team to deliver a spectrally stable Doppler magnetograph for such orbits (e.g., Dominguez-Tagle et al., 2014). Ideally, should a space-hardened spacecraft bus with sufficient mass/energy configuration be found then this “minimum requirement” can, and should, be expanded to include in situ experiments and a coronagraph before any EUV imager.
A vital aspect of HMM, and especially when deployed as part of any operational framework, is how the data from the network of satellites can be rapidly relayed to Earth. While power will be an issue, it seems like laser telecom will be the preferred method of high data throughput at low mass. In recent times, such technology is growing in readiness (Tardivel et al., 2018).
The observations and measurements acquired from HMM will enable a new generation of heliophysicists with a view to understanding the coupled solar system as a whole. There will be undoubted advances in understanding the processes of magnetic flux emergence and those deeper-rooted processes that drive the solar cycle (see e.g., Jiang et al., 2014; Upton and Hathaway, 2014; Virtanen et al., 2017; Whitbread et al., 2017, as contemporary examples). There will be progress in understanding the evolution of global-scale magnetic topology that results which will lead to breakthroughs in understanding the global distribution of solar wind energies and compositional characteristics. All-in-all HMM will explore the origins of the Sun's activity and predict the variations of the space environment like no mission ever before by engaging vast tranches of the US and worldwide community in the effort through the novelty of the data and distribution of the technology effort.
In the HMM future, data assimilation-driven forecasts models using 360 degree information will be norm. The operational community at present requires knowledge that provides high skill predictions of space weather path, arrival time, and magnitude. At best these windows are broad, ranging from one to 3 days in scatter. In an environment of growing technological reliability it is too broad a window. Further, the critical failure of surface flux transport models to drive the current generation of forecast models mandates at least a mission to the“L5” Lagrange point to observe what may rotating onto the Sun-Earth line. As stated above, the data from HMM could be readily ingested into the current generation of models leading to significant improvement in skill just by providing a more comprehensive observational set. The research insight developed through the analysis of the global perspective provided by HMM will advance that further as we understand the evolution of the magnetically driven outer atmosphere. The data ingestion and assimilation effort that will be part of the mission will drive the creation of next-generation observationally-driven physics-based solar wind forecast models.
The preliminary HMM configuration would be a “string of pearls” around the Sun in the ecliptic plane. However, it is becoming more and more obvious that our inability to observe the Sun's polar regions and characterize the evolution of plasma flows and magnetism at high solar latitudes is also limiting our understanding. Indeed, papers such as that cited above (e.g., McIntosh et al., 2014) point to 55° latitude as the potential origin of the Sun's dynamo and, hence, as the source of all space weather. To address this issue continuous observations of the high latitude solar atmosphere are required. Taking the HMM concept, and sequentially launching four or six of the same smallsats into highly inclined orbits or solar polar orbits would provide 4-π observations of the Sun.
Naturally, deploying advanced coronagraphs as part of an extended HMM, like those capable of measuring magnetic fields in the corona (e.g., Tomczyk et al., 2008) may provide unique perspectives on (and solutions to) the “Bz” challenge of measuring and forecasting the magnetic orientation of solar disturbances before they reach the Earth. The present paradigm is a very short warning (~20 min) from upstream monitors at the lagrange “L1” point. Clearly, a matter requiring an observational solution.
HMM is doable with present technology. This mission, or one like it to observe the entire Sun all of the time, is critical in terms of scientific understanding of our star and for the operational need to protect our technologically advancing society. This short paper only scrapes the surface in terms of the numerous studies required to see something like HMM become reality.
All authors listed have made a substantial, direct and intellectual contribution to the work, and approved it for publication.
The National Center for Atmospheric Research is sponsored by the National Science Foundation.
The authors declare that the research was conducted in the absence of any commercial or financial relationships that could be construed as a potential conflict of interest.
We appreciate discussions with many colleagues in the construction of this concept, in no order: Alan Title, Karel Schrijver, Paulett C. Liewer, Neil Murphy, Justin Kasper, and Thomas Zurbuchen.
Anderson, B. J., Acuña, M. H., Lohr, D. A., Scheifele, J., Raval, A., Korth, H., et al. (2007). The magnetometer instrument on MESSENGER. Space Sci. Rev. 131, 417–450. doi: 10.1007/s11214-007-9246-7
Andrews, G. B., Zurbuchen, T. H., Mauk, B. H., Malcom, H., Fisk, L. A., Gloeckler, G., et al. (2007). The energetic particle and plasma spectrometer instrument on the MESSENGER spacecraft. Space Sci. Rev. 131, 523–556. doi: 10.1007/s11214-007-9272-5
Bale, S. D., Goetz, K., Harvey, P. R., Turin, P., Bonnell, J. W., Dudok de Wit, T., et al. (2016). The FIELDS instrument suite for solar probe plus. Measuring the coronal plasma and magnetic field, plasma waves and turbulence, and radio signatures of solar transients. Space Sci. Rev. 204, 49–82. doi: 10.1007/s11214-016-0244-5
Berghmans, D., Hochedez, J. F., Defise, J. M., Lecat, J. H., Nicula, B., Slemzin, V., et al. (2006). SWAP onboard PROBA 2, a new EUV imager for solar monitoring. Adv. Space Res. 38, 1807–1811. doi: 10.1016/j.asr.2005.03.070
Brouwer, M. P., and Zwaan, C. (1990). Sunspot nests as traced by a cluster analysis. Solar Phys. 129, 221–246.
Carrington, R. C. (1858). On the evidence which the observed motions of the solar spots offer for the existence of an atmosphere surrounding the Sun. Monthly Notices R. Astronom. Soc. 18, 169–177.
Castenmiller, M. J. M., Zwaan, C., and van der Zalm, E. B. J. (1986). Sunspot nests - Manifestations of sequences in magnetic activity. Solar Phys. 105, 237–255.
Dikpati, M., Cally, P. S., McIntosh, S. W., and Heifetz, E. (2017). The origin of the “seasons” in space weather. Sci. Rep. 7:14750. doi: 10.1038/s41598-017-14957-x
Dikpati, M., McIntosh, S. W., Bothun, G., Cally, P. S., Ghosh, S. S., Gilman, P. A., et al. (2018). Role of interaction between magnetic rossby waves and tachocline differential rotation in producing solar seasons. Astrophys. J. 853:144. doi: 10.3847/1538-4357/aaa70d
Dominguez-Tagle, C., Appourchaux, T., Ruiz de Galarreta, C., Fourmond, J.-J., Philippon, A., Le Clec'h, J.-C., et al. (2014). “Optical characterization of the breadboard narrowband prefilters for Solar Orbiter PHI,” in Space Telescopes and Instrumentation 2014: Optical, Infrared, and Millimeter Wave,vol. 9143 of em Proc. Soc. Phot. Inst. Eng., 91435G (Montreal, QC).
Gray, W. M. (1968). Global view of the origin of tropical disturbances and storms. Monthly Weather Rev.96, 669–700.
Gurgenashvili, E., Zaqarashvili, T. V., Kukhianidze, V., Oliver, R., Ballester, J. L., Dikpati, M., et al. (2017). North-South asymmetry in rieger-type periodicity during solar cycles 19-23. Astrophys. J. 845:137. doi: 10.3847/1538-4357/aa830a
Hoeksema, T. (2005). Heliophysics: The New Science of the Sun-Solar System Connection, Recommended Roadmap for Science and Technology 2005-2035. Available online at: https://permanent.access.gpo.gov/LPS73216/Roadmap_FINALscr.pdf
Howard, R. A., Moses, J. D., Vourlidas, A., Newmark, J. S., Socker, D. G., Plunkett, S. P., et al. (2008). Sun Earth Connection Coronal and Heliospheric Investigation (SECCHI). Space Sci. Rev. 136, 67–115. doi: 10.1007/s11214-008-9341-4
Jiang, J., Hathaway, D. H., Cameron, R. H., Solanki, S. K., Gizon, L., and Upton, L. (2014). Magnetic flux transport at the solar surface. Space Sci. Rev. 186, 491–523. doi: 10.1007/s11214-014-0083-1
Kasper, J. C., Abiad, R., Austin, G., Balat-Pichelin, M., Bale, S. D., Belcher, J. W., et al. (2016). Solar Wind Electrons Alphas and Protons (SWEAP) investigation: design of the solar wind and coronal plasma instrument suite for solar probe plus. Space Sci. Rev. 204, 131–186. doi: 10.1007/s11214-015-0206-3
Klesh, A. (2018). Interplanetary Nano-spacecraft Pathfinder in Relevant Environment (inspire). Available online at: https://www.jpl.nasa.gov/cubesat/missions/inspire.php.
Krista, L. D., McIntosh, S. W., and Leamon, R. J. (2018). The longitudinal evolution of equatorial coronal holes. Astronom. J. 155, 153. doi: 10.3847/1538-3881/aaaebf
Lemen, J. R., Title, A. M., Akin, D. J., Boerner, P. F., Chou, C., Drake, J. F., et al. (2012). The Atmospheric Imaging Assembly (AIA) on the Solar Dynamics Observatory (SDO). Solar Phys. 275, 17–40. doi: 10.1007/s11207-011-9776-8
Löptien, B., Gizon, L., Birch, A. C., Schou, J., Proxauf, B., Duvall, T. L., et al. (2018). Global-scale equatorial Rossby waves as an essential component of solar internal dynamics. Nat. Astron. doi: 10.1038/s41550-018-0460-x. [Epub ahead of print].
Lorenz, E. N. (1973). On the existence of extended range predictability. J. Appl. Meterol. 12, 543–545.
McIntosh, S. W., Cramer, W. J., Pichardo Marcano, M., and Leamon, R. J. (2017). The detection of Rossby-like waves on the Sun. Nat. Astron. 1:0086. doi: 10.1038/s41550-017-0086
McIntosh, S. W., Leamon, R. J., Krista, L. D., Title, A. M., Hudson, H. S., Riley, P., et al. (2015). The solar magnetic activity band interaction and instabilities that shape quasi-periodic variability. Nat. Commun. 6, 6491. doi: 10.1038/ncomms7491
McIntosh, S. W., Wang, X., Leamon, R. J., and Scherrer, P. H. (2014). Identifying potential markers of the Sun's giant convective scale. Astrophys. J. Lett. 784:L32. doi: 10.1088/2041-8205/784/2/L32
NRC (2013). Solar and Space Physics: A Science for a Technological Society. Washington, DC: The National Academies Press.
Rossby, C. G. (1939). Relation between variations in the intensity of the zonal circulation of the atmosphere and the semi-permanent centers of action. J. Mar. Res. 2, 38–55.
Schrijver, C. J., Kauristie, K., Aylward, A. D., Denardini, C. M., Gibson, S. E., Glover, A., et al. (2015). Understanding space weather to shield society: A global road map for 2015-2025 commissioned by COSPAR and ILWS. Adv. Space Res. 55, 2745–2807. doi: 10.1016/j.asr.2015.03.023
Tardivel, S., Klesh, A. T., and Campagnola, S. (2018). Technology enabling interplanetary trajectories for nanospacecraft. J. Spacecraft Rockets 55, 95–105. doi: 10.2514/1.A33789
Thompson, P. D. (1983). History of numerical weather prediction in the united states. Bull. Am. Meterol. Soc. 64, 755–769.
Tomczyk, S., Card, G. L., Darnell, T., Elmore, D. F., Lull, R., Nelson, P. G., et al. (2008). An Instrument to Measure Coronal Emission Line Polarization. Solar Phys. 247:411–428. doi: 10.1007/s11207-007-9103-6
Upton, L., and Hathaway, D. H. (2014). Predicting the Sun's polar magnetic fields with a surface flux transport model. Astrophys. J. 780:5. doi: 10.1088/0004-637X/780/1/5
Virtanen, I. O. I., Virtanen, I. I., Pevtsov, A. A., Yeates, A., and Mursula, K. (2017). Reconstructing solar magnetic fields from historical observations. II. Testing the surface flux transport model. Astron. Astrophys. 604:A8. doi: 10.1051/0004-6361/201730415
Whitbread, T., Yeates, A. R., Muñoz-Jaramillo, A., and Petrie, G. J. D. (2017). Parameter optimization for surface flux transport models. Astron. Astrophys. 607:A76. doi: 10.1051/0004-6361/201730689
Keywords: dynamo theory, magnetic fields, sunspots, solar activity, space weather, space weather forecasting
Citation: McIntosh SW and Leamon RJ (2018) The Heliospheric Meteorology Mission: A Mission to DRIVE our Understanding of Heliospheric Variability. Front. Astron. Space Sci. 5:21. doi: 10.3389/fspas.2018.00021
Received: 13 April 2018; Accepted: 04 June 2018;
Published: 26 June 2018.
Edited by:
Lee Samuel Finn, Pennsylvania State University, United StatesReviewed by:
Ilpo Virtanen, University of Oulu, FinlandCopyright © 2018 McIntosh and Leamon. This is an open-access article distributed under the terms of the Creative Commons Attribution License (CC BY). The use, distribution or reproduction in other forums is permitted, provided the original author(s) and the copyright owner are credited and that the original publication in this journal is cited, in accordance with accepted academic practice. No use, distribution or reproduction is permitted which does not comply with these terms.
*Correspondence: Scott W. McIntosh, bXNjb3R0QHVjYXIuZWR1
Disclaimer: All claims expressed in this article are solely those of the authors and do not necessarily represent those of their affiliated organizations, or those of the publisher, the editors and the reviewers. Any product that may be evaluated in this article or claim that may be made by its manufacturer is not guaranteed or endorsed by the publisher.
Research integrity at Frontiers
Learn more about the work of our research integrity team to safeguard the quality of each article we publish.