- 1Department of European and Mediterranean Cultures, University of Basilicata, Matera, Italy
- 2Mediterranean Agronomic Institute of Bari (CIHEAM Bari), Valenzano, Italy
- 3School of Agricultural, Forestry, Environmental and Food Sciences, University of Basilicata, Potenza, Italy
- 4Department of Soil, Plant, and Food Science, University of Bari, Bari, Italy
- 5Water Research Institute (IRSA), National Research Council (CNR), Bari, Italy
- 6Department of Agricultural Sciences, Food, Natural Resources and Engineering (DAFNE), University of Foggia, Foggia, Italy
Introduction: Emerging contaminants in irrigation wastewater can cause bioaccumulation in crops, posing health risks to humans and other living organisms. The extent to which different emerging contaminants (ECs) are taken up by plants varies depending on the physicochemical properties of the contaminants and the type of crops grown.
Methods: This study investigated the fate of emerging contaminants (ECs), particularly pharmaceuticals, in durum wheat grown on soils irrigated with treated wastewater in southern Italy. The experiment was conducted in lysimeters irrigated with wastewater during previous crop cycles, assessing the presence and distribution of ECs in both soil and plant tissues. Three different levels of exposure to ECs were compared: freshwater irrigation (FW), wastewater treated to European average contaminant levels (TWWx1), and a triple dose of contaminants (TWWx3).
Results: The behavior of 12 different ECs in the plant-soil complex was analyzed and found variable. Fluconazole and carbamazepine were found to have higher uptake concentrations in the plant, with accumulation observed in the plant and grain, especially in the TWWx3 treatment. However, some ECs (such as Sulfamethoxazole, Trimethoprim, Ketoprofen, Diclofenac, Metoprolol, and Naproxen) showed high uncertainties in their fate, probably due to degradation in soil and influential crop parameters.
Discussion: The results of this study contribute to the argument that the reuse of treated wastewater for irrigation, if properly monitored, can be a safe approach in agriculture and can support policymakers in developing future legislative frameworks for sustainable water management.
1 Introduction
The agricultural sector plays a strategic role in improving the availability of food and achieving food security (1). Rice, maize, and wheat are essential crops that contribute to nearly half of the world’s food calories, making them crucial for global food security (2). The productivity of agriculture is underpinned by healthy and productive soils in combination with the accessibility to freshwater resources (3). However, urbanization, industrialization, and intensive agriculture development have introduced pollutants and synthetic chemicals into the ecosystem, causing significant environmental issues (3, 4).
The Mediterranean, a major agricultural region, is grappling with environmental challenges and freshwater pollution caused by a range of newly identified chemical substances known as emerging contaminants (5). Emerging contaminants (ECs) in agriculture, including nano-plastics, per- and polyfluoroalkyl substances (PFAS), endocrine disrupting chemicals (EDCs), antibiotics, hormones, and pain relievers, are potential environmental pollutants with adverse effects on crops, soil, water, and the broader environment (6). ECs may occur naturally or be synthesized for various medical, industrial, and other practical applications (7).
Reuse of treated wastewater (TWW) for irrigation has become a widespread practice in the arid and semi-arid areas of the Mediterranean, Middle East, and Asia. However, this practice presents challenges, including soil salinity, potential health risks from pathogens and heavy metals, and complex environmental and socio-economic considerations (8). The use of treated wastewater for crop irrigation and pesticides in agriculture can significantly increase the prevalence of ECs in arid and semi-arid countries (9). A primary concern associated with reusing wastewater for agricultural irrigation is the introduction of pharmaceuticals and personal care products (10), as they can trigger diverse physiological effects in humans (11). PPCPs are considered ECs due to their persistence, potential toxicity, and bioaccumulation in the environment (12). Wastewater, compost, and manure applications release PPCPs into agricultural soil systems (13). These contaminants may then undergo various biotic and abiotic transformations within the soil environment.
The long-term use of contaminated irrigation water affects plant ecosystem health, aquatic ecosystems, soil microorganisms, normal plant growth and development, and the quantity and quality of agricultural produce (2). Therefore, understanding the concentration, behavior, and cycling of contaminants, along with their degradation pathways, is crucial for the remediation of these substances originating from various sources (7). The extent to which different ECs are taken up by plants varies depending on the physicochemical properties of the contaminants and the type of crops grown (14).
Scientific studies have attempted to characterize the uptake of ECs from reclaimed water into agricultural soils (15–17) and various crops, including fruits (12, 18–20), vegetables (16, 19, 21), and cereals (22, 23). These studies have employed different plant-growth methods (e.g., hydroponic cultivation, crops in pots under greenhouse, field cultivation conditions), different sets of contaminants, types of plants, and irrigation methods, resulting in some inconsistency in conclusions (24). Consequently, a gap remains in understanding the extent and specific conditions under which these contaminants appear in irrigated crop production systems. This is particularly relevant for cereal cultivation, where data on EC bioaccumulation and translocation under real-field growing conditions is limited.
Durum wheat is a staple crop in the Mediterranean region. Climate change is expected to increase irrigation water requirements for durum wheat cultivation in the Mediterranean region by up to 16% (25). As the reuse of treated wastewater for irrigation becomes more prevalent, further research is needed to quantify the occurrence of emerging contaminants (ECs) and identify the factors that influence their presence and mobility in agricultural environments utilizing treated wastewater. This will be crucial for ensuring food safety and quality as durum wheat production adapts to a changing climate in the Mediterranean region.
This research builds upon the previous work of Denora et al. (20) and investigates the fate of ECs, particularly pharmaceuticals, in durum wheat cultivation on soils irrigated with treated wastewater in southern Italy. By expanding our understanding of how ECs behave in agricultural systems using reclaimed water, this study can provide valuable insights to support the safe and sustainable use of treated wastewater for irrigation in the Mediterranean region.
2 Materials and methods
2.1 Experimental design
The trial was conducted at the Centro Ricerche Agrobiologiche ALSIA Metapontum, located in the province of Matera, Italy, at coordinates 40.4029 N, 16.7944 E. The Mediterranean climate is characterized by hot, dry summers with average temperatures ranging from 24°C to 28°C and moderate, wet winters, experiencing an annual rainfall of 600–700 millimeters.
The “Saragolla” variety (Triticum durum Desf.) was sown on January 13, 2022, in the same pots previously utilized for growing tomatoes (20). The cultivation concluded with the harvest on June 21, 2022. Seeds were planted in lysimeters of 0.8 m³ each, with 4 rows containing 90 seeds each and spaced 13 cm apart between rows. The distribution followed a randomized block experimental design (Figure 1) with four repetitions.
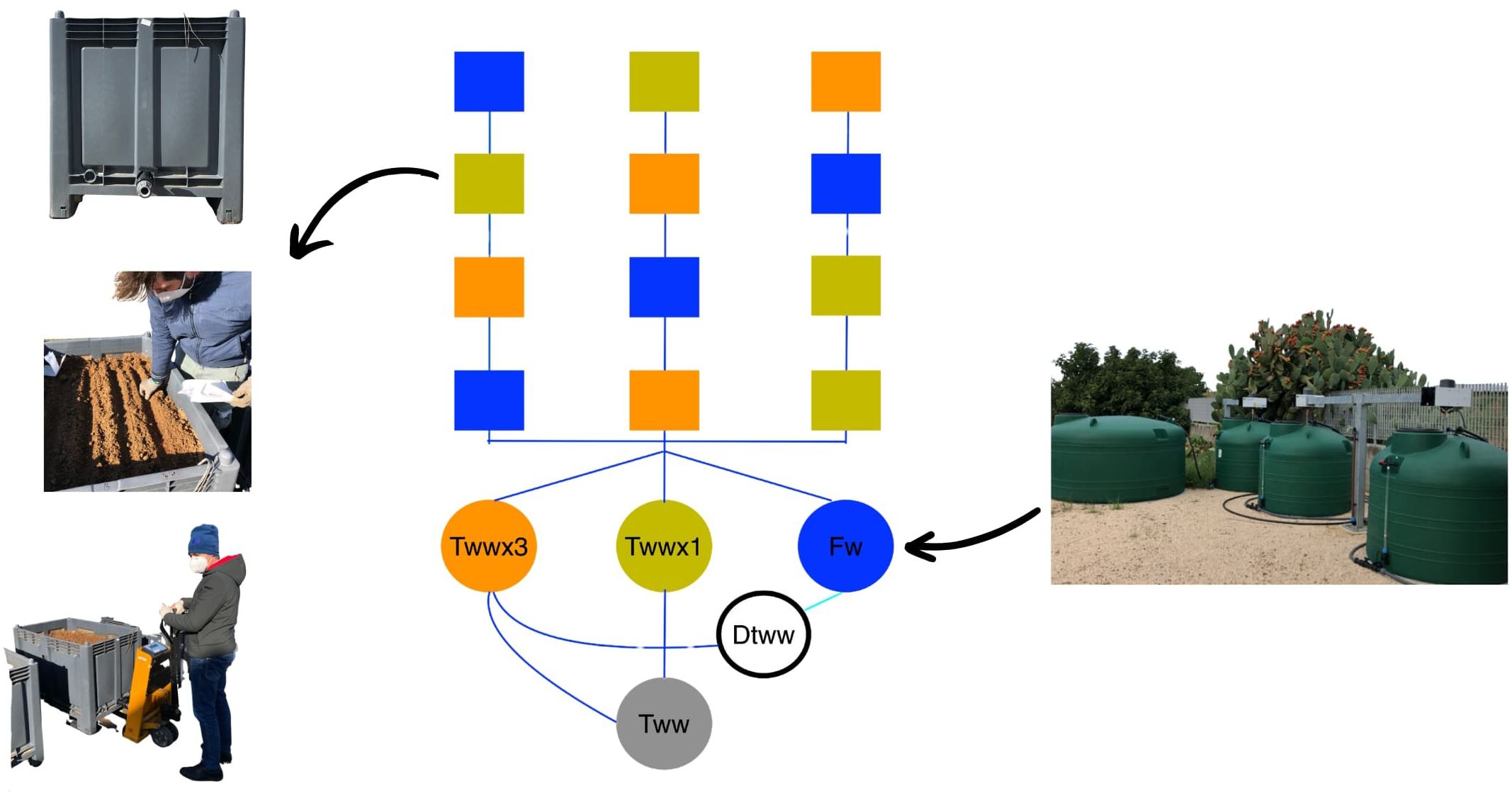
Figure 1. The experimental setup includes tanks for water treatments (TWWx1, TWWx3, FW), lysimeters for various scenarios, and tanks for storing treated wastewater (TWW) and its safe disposal (DTWW).
The experimental design included the comparison of three irrigation treatments (Figure 1):
i. Control (FW): Irrigation with surface freshwater (FW) from the irrigation network commonly used by farmers in the region for crop irrigation.
ii. TWWx1: Irrigation with tertiary (TWW) municipal wastewater that has been spiked with target contaminants at a dose corresponding to the European average concentration.
iii. TWWx3: Irrigation with tertiary (TWW) municipal wastewater spiked with ECs at a triple dose compared to the TWWx1 treatment.
This experimental setup allowed for a systematic evaluation of the fate and behavior of ECs in the soil-plant system when irrigated with reclaimed water containing varying levels of emerging pollutants. TWW was derived from the secondary sewage effluent of the municipal wastewater treatment plant (WWTP) in Ferrandina (Italy) using rapid sand filtration (rSF), followed by peracetic acid treatment (contact times greater than 60 minutes and doses of 2.5 mg/L).
Table 1 presents the average values with standard deviations for the primary conventional parameters of both surface freshwater (FW) and tertiary wastewater (TWW) throughout the study period.
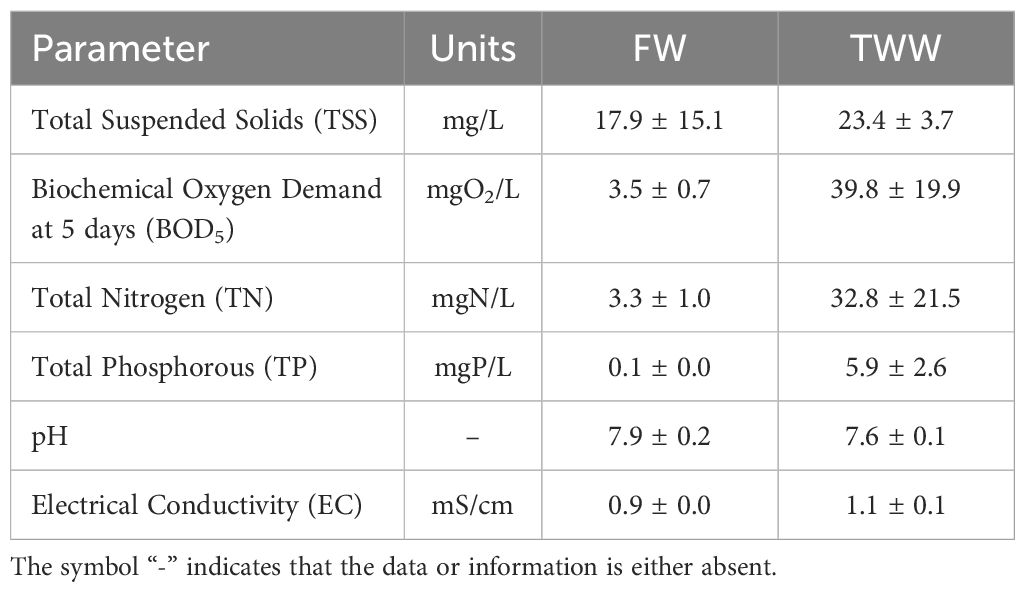
Table 1. Main conventional parameters of FW and TWW that were detected during the experimental period.
The tertiary wastewater (TWW) used in the experiment was spiked with a mixture of emerging contaminants (ECs) for two of the irrigation treatments (TWWx1 and TWWx3). These ECs included clarithromycin (CLR), sulfamethoxazole (SMX), trimethoprim (TMP), carbamazepine (CBZ), diclofenac (DCF), fluconazole (FLC), climbazole (CLB), ketoprofen (KTP), metoprolol (MTP), naproxen (NAP), triclosan (TCS), and gemfibrozil (GFB). These compounds were specifically chosen because they are commonly detected in wastewater and are often not completely removed by standard wastewater treatment processes. Their presence in treated wastewater used for irrigation raises concerns about potential accumulation in crops and transfer into the food chain.
Table 2 shows the concentration values of target ECs in TWW, which range from low ng/L to low μg/L (26).
Table 3 summarizes the physicochemical parameters of the selected ECs. The multi-component standard solution (1000 ppm) was prepared using standards with a purity of more than 98%. This solution was mixed with irrigation wastewater at concentrations of 200 and 600 (μg/L) for each component, resulting in TWWx1 and TWWx3, respectively. Before sowing durum wheat, the experimental soils were tested to determine the residual EC concentration from the previous season. These data represent the initial condition (T0) and were crucial for carrying out the mass balance assessment.
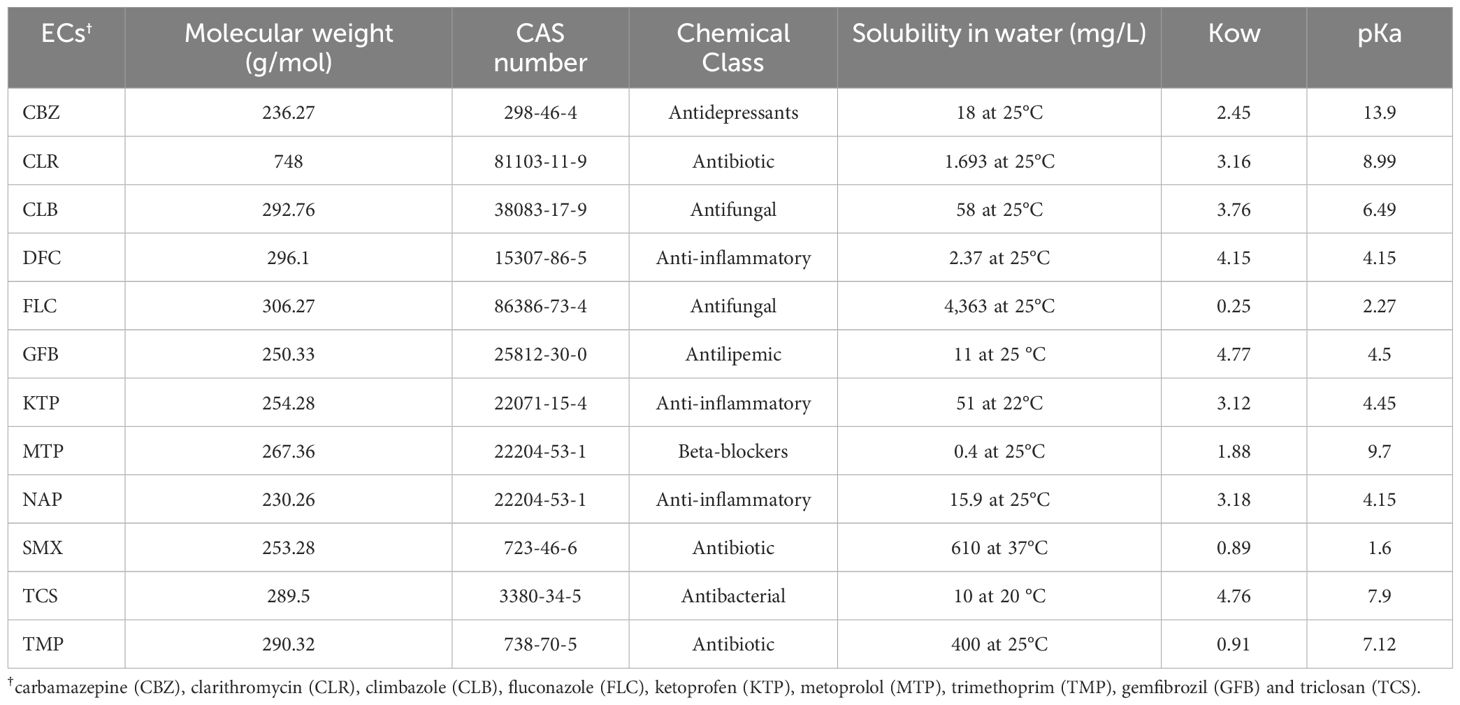
Table 3. Physicochemical Properties (Mw, Molecular Weight; Water Solubility; Kow, Octanol/Water Coefficient; pKa, Acid Ionization Constant) of the Selected ECs.
The experimental design includes four lysimetric devices (plots) for each irrigation treatment. The identical lysimeters used in the previous study (20) were employed to evaluate the effects of irrigation with wastewater on wheat (Figure 2). The soil studied is classified as sandy loam according to the United States Department of Agriculture, with the following physical and chemical properties: sand 84.7%; silt 3.3%; clay 12.0%; field capacity (measured at 0.03 MPa) of 13.2% dry weight (dw); wilting point (measured at -1.5 MPa) of 7.2% dw; bulk density of 1.45 Mg/m3; pH 8.3; electrical conductivity 0.10 dS m-1; organic matter 0.32% (Walkley and Black method); available phosphorus (Olsen method) 35.6 mg/kg; total potassium 0.92 g/kg (determined using inductively coupled plasma optical emission spectrometry, Agilent, ICP-OES 720); total nitrogen 0.51% (Kjeldahl method); mineral NO3-N 0.7 mg/kg; mineral NH4-N 2.7 mg/kg. This soil type is typical of the Ionian-Metapontine coast and is widely used for vegetable (27) and durum wheat farming. Furthermore, the soil’s favorable hydraulic conductivity made it easier to monitor the circulating solution movement with moisture sensors. There were no irrigation treatments planned, except after blooming, when the goal was to evaluate the influence and uptake of ECs on the lysimetric system. This choice was partly influenced by poor weather conditions such as high temperatures and the lack of precipitation in May and June (Figure 3).
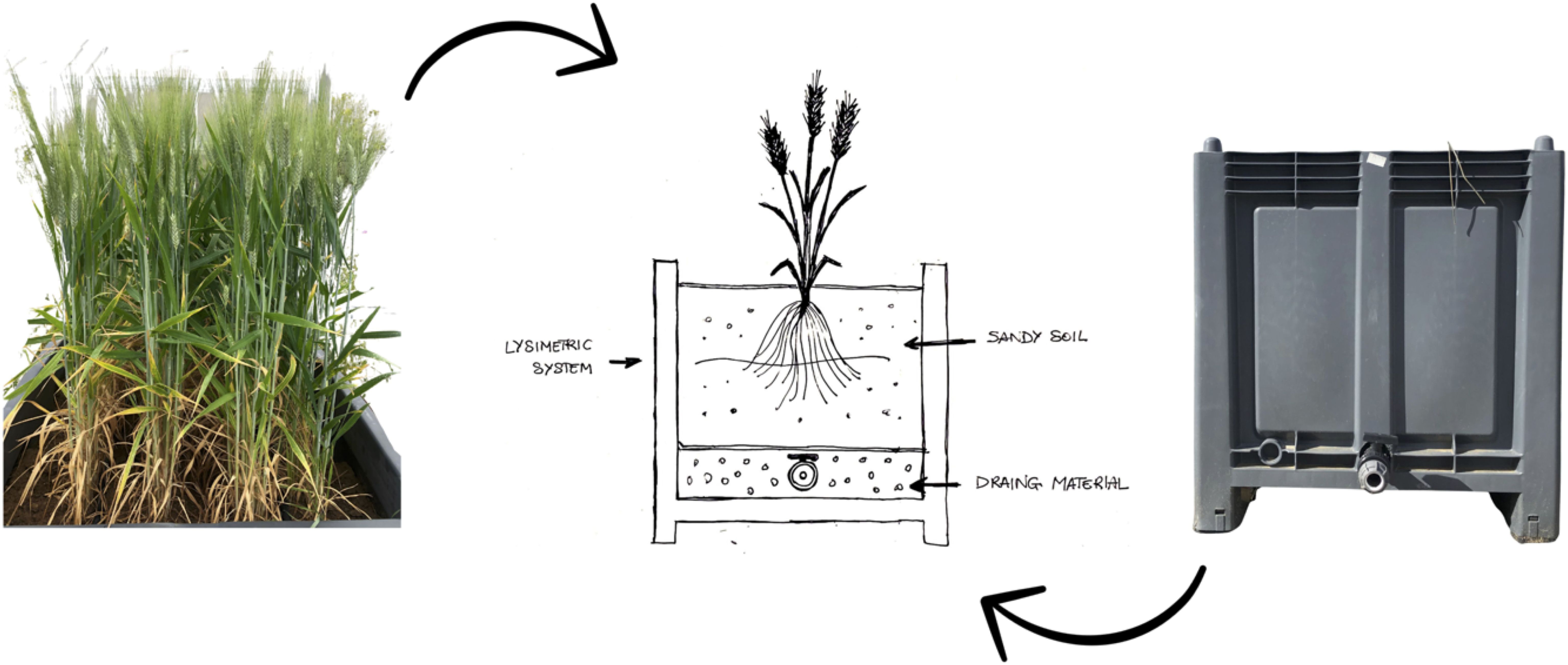
Figure 2. Schematic representation of the lysimetric weighing system, for determining water consumption, water flow, and mass balance of ECs.
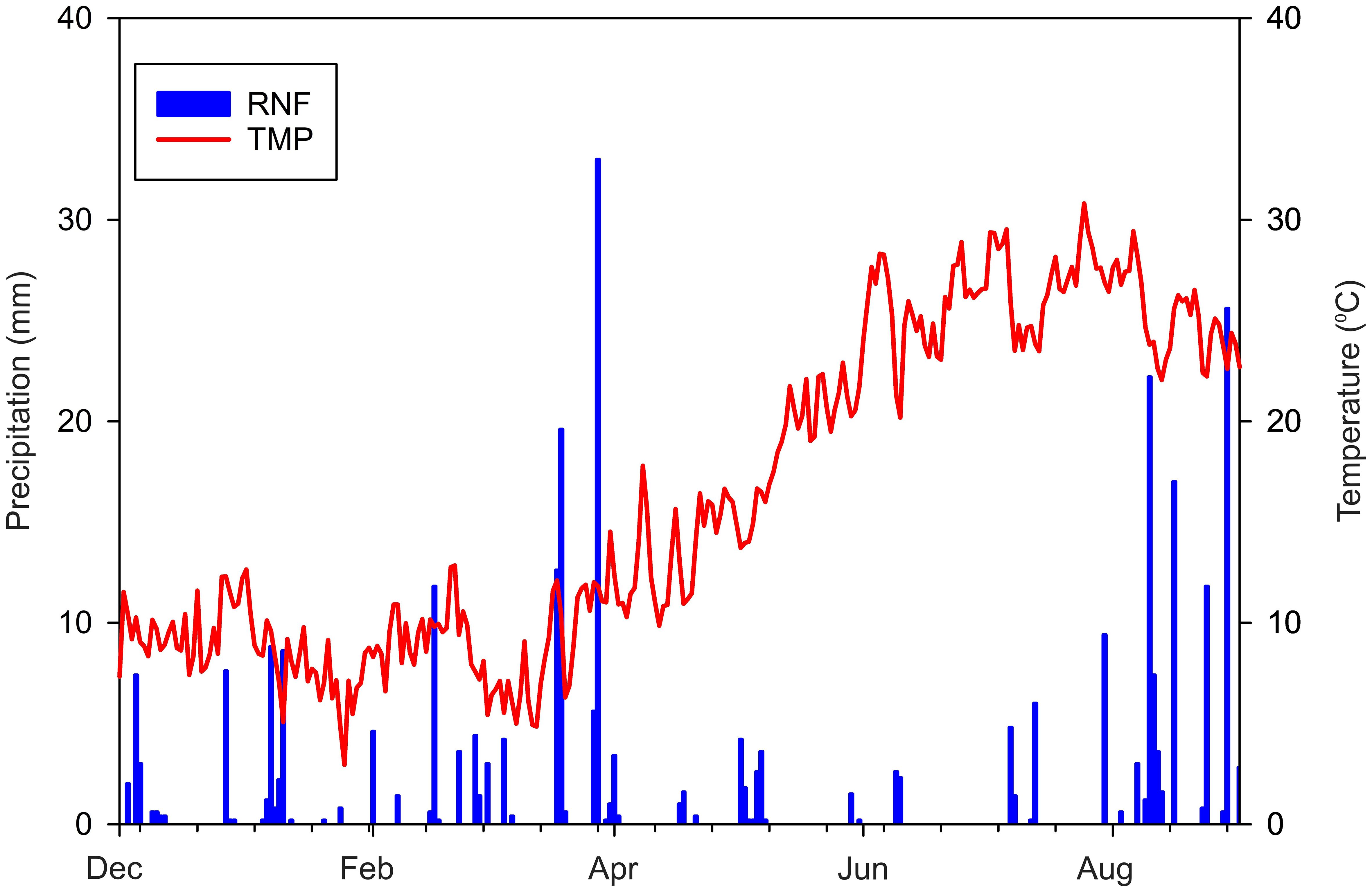
Figure 3. Climatic trends from December 2021 to August 2022: Precipitation (RNF) in millimeters (blue bars) and Temperature (TMP) in degrees Celsius (red line).
This enabled us to carry out irrigation treatments that restored the lysimetric system to field capacity. Between April and June 2022, a total of 200 liters per lysimeter was provided for the three comparable treatments, with an additional EC concentration of 40 mg L-1 for the TWWx1 treatment and 120 mg L-1 for the TWWx3 treatment.
During the late flowering phenological stage of durum wheat, each lysimeter was irrigated with a micro-flow system that included individual drippers. The initial irrigation was intended to restore the entire soil volume to its field water capacity (FWC). Following this, a weekly irrigation rotation was conducted, providing enough irrigation volume to re-establish soil moisture at the FWC level (28). Crop water consumption between irrigations was measured by weighing individual lysimeter tanks using a pallet jack fitted with load cells. The weight differential in the tank between the end of the previous irrigation and the start of the next one represented both water consumption during that time and the irrigation volume required to restore the soil’s Field Water Capacity to the target level. A probe was placed into each lysimeter in the experimental plot to confirm the correctness of the irrigation scheduling criteria and make any necessary adjustments to the irrigation volume. Soil moisture was measured using a probe equipped with Sentek Technologies’ Diviner 2000 sensors. All components of the water balance were carefully monitored, and drainage water samples were collected to follow any movement of ECs in the aquifer through the lysimeters (Figure 2). The choice to experiment with lysimeters in an open field rather than a greenhouse allowed for a more precise simulation of real agricultural conditions. Given that the durum wheat test occurred after the cultivation of tomatoes on the same lysimeters with the same experimental design, the irrigation volume was deliberately programmed for experimental purposes to optimally assess the absorption and fate of the selected ECs.
2.2 Extraction procedure
The modified QuEChERS approach developed by De Mastro et al. (29) was used to extract ECs from experimental soils. Since the QuEChERS extraction method was developed for materials with moisture content higher than 75%, such as plant matrices such as straw and grain, it was necessary to decrease the sample volume and increase the amount of injected to make the pores of the sample more accessible to the extraction solvent (30–32). The samples were pre-treated before the extraction process began. To remove soil, roots were washed with a light stream of tap water, rinsed with deionized water, and gently dried with absorbent paper, while straw and grains were finely chopped in a grinder. Samples were stored in a 50-mL centrifuge tube at -20°C until extraction. 2 g of roots, 1 g of straw, and 5 g of grains were placed in a 50 mL plastic centrifuge tube, with 10 mL of water added to all samples except the roots, which required 6 mL. After capping, the tubes were vortexed for one minute. Ten milliliters of acetonitrile (ACN) were added to the wet samples. The tubes holding straw and grain were shaken by hand for one minute, while the tubes containing roots were shaken for five minutes. In the case of straw, the sample rested for 15 minutes. This was followed by a salting phase with citrate buffer (4 g MgSO4 + 1 g NaCl + 0.50 g NaCitrate Dibasic Sesquihydrate + 1 g NaCitrate Tribasic Dihydrate). The tubes were shaken vigorously by hand for an additional 5 minutes. The straw and root samples were then centrifuged at 3700 rpm for 5 minutes. The times were doubled for grain samples, which were allowed to rest for two hours after this step. The phase separation between the aqueous and organic solvents after centrifugation enabled the extraction of 6 mL of the upper ACN layer with a pipette. For the purification step, the aliquot was added to 15 mL tubes containing 900 mg MgSO4 + 150 mg primary secondary amine (PSA) for roots or 900 mg MgSO4 + 150 mg PSA + 150 mg octadecyl (C18). After vortexing for 1 minute, the tubes were placed in a centrifuge for 5 minutes at 4000 rpm. The supernatant was filtered through a membrane filter (PVDF, 0.22 μm) and 1.5 mL was transferred to a screw-cap vial for LC-MS/MS analysis.
2.3 Bioconcentration and translocation factor evaluation
The bioconcentration factor (BCF) is an index related to the accumulation in plant tissues of a particular EC concerning its soil concentration in the soil. It was calculated according to (33), as defined in (Equation 1).
where Cplant and Csoil represent the concentrations (ng/g) of particular ECs in the wheat parts (root, straw and grain) and the soil, respectively, on a dry weight basis. The translocation factor (TF) represents the movement of ECs from the roots to various parts of the plant. It is calculated by comparing the concentration (ng/g) of ECs in the plant (straw and grain) to their concentrations (ng/g) in the roots, using Equation 2 (33, 34).
2.4 Statistical analysis
For experimental continuity, the same statistical analysis approach as the previous year was applied (20). We applied the ANOVA methodology to all datasets in a randomized block design with four replications. A one-way ANOVA was used, with irrigation treatments (FW, TWWx1, and TWWx3) as fixed variables and replication as a random variable. The complete dataset was examined using the assumptions of analysis of variance (ANOVA). The normal distribution of residuals from the model was evaluated both visually (by QQ-plot) and statistically (Shapiro-Wilk normality test). Additionally, Levene’s test was used to confirm homoscedasticity. The experimental design, together with random sampling for several matrices, met the ultimate ANOVA assumption. The ANOVA was performed on the model only when all three ANOVA assumptions were met. Following that, a post-hoc analysis of the calculated marginal averages was performed only in cases where the ANOVA revealed a statistically significant difference (p-value 0.05), using Tukey’s HSD (Honestly Significant Difference) using the agricolae package in RStudio (version 4.1.3), and results were plotted with SigmaPlot 15.0. The bioconcentration and translocation factors (BCF and TF) data did not meet ANOVA assumptions, so we presented them by mean data ± standard error. Furthermore, BCF data were jointly considered in a multivariate approach and processed statistically for principal component analysis (PCA). Before performing the PCA, the BCF values were standardized. The number of factors needed to adequately describe the data was determined based on eigenvalues and of percentage of the total variance accounted for by different factors. The results of PCA were graphically represented in two-dimensional plots. Principal component analysis (PCA) was performed on the correlation matrix of BCF values using the JMP software package, version 14.3 (SAS Institute Inc., Cary, NC, USA).
3 Results
3.1 Water balance components
The wheat growing cycle commenced with soil moisture levels near the field water capacity, resulting from previous irrigation and a rainy winter period. This led to the drainage of 204 mm of the total 275 mm of precipitation during the initial stages of the crop cycle. In contrast, the period between April and June, which is critical for durum wheat’s vegetative growth, was marked by a lack of precipitation and rising temperatures (Figure 3). During this period, 160 mm of irrigation water was applied to the lysimeters, offering a distinct opportunity to investigate the introduction and interaction of emerging contaminants in the soil-plant system. The study introduced two distinct cumulative concentrations of emerging contaminants (ECs) through treated wastewater irrigation in the TWWx1 and TWWx3 treatments, respectively. In the TWWx1 treatment, the cumulative EC concentration was 40 mg/L, while in the TWWx3 treatment, it was 120 mg/L, which was three times higher. These additions built upon the existing EC levels in the soil system, which had been established through previous experiments involving tomato crops under similar conditions.
3.2 Dynamics of the ECs soil-plant concentrations
The concentrations of emerging contaminants (ECs) in soil and plant tissues (roots, straw, and grain) at the end of the durum wheat cropping cycle are presented in Figure 4 for the TWWx1 and TWWx3 treatments. In contrast, the freshwater (FW) irrigation treatment did not result in significant levels of ECs in the soil-plant system, as it did not involve the application of spiked treated wastewater.
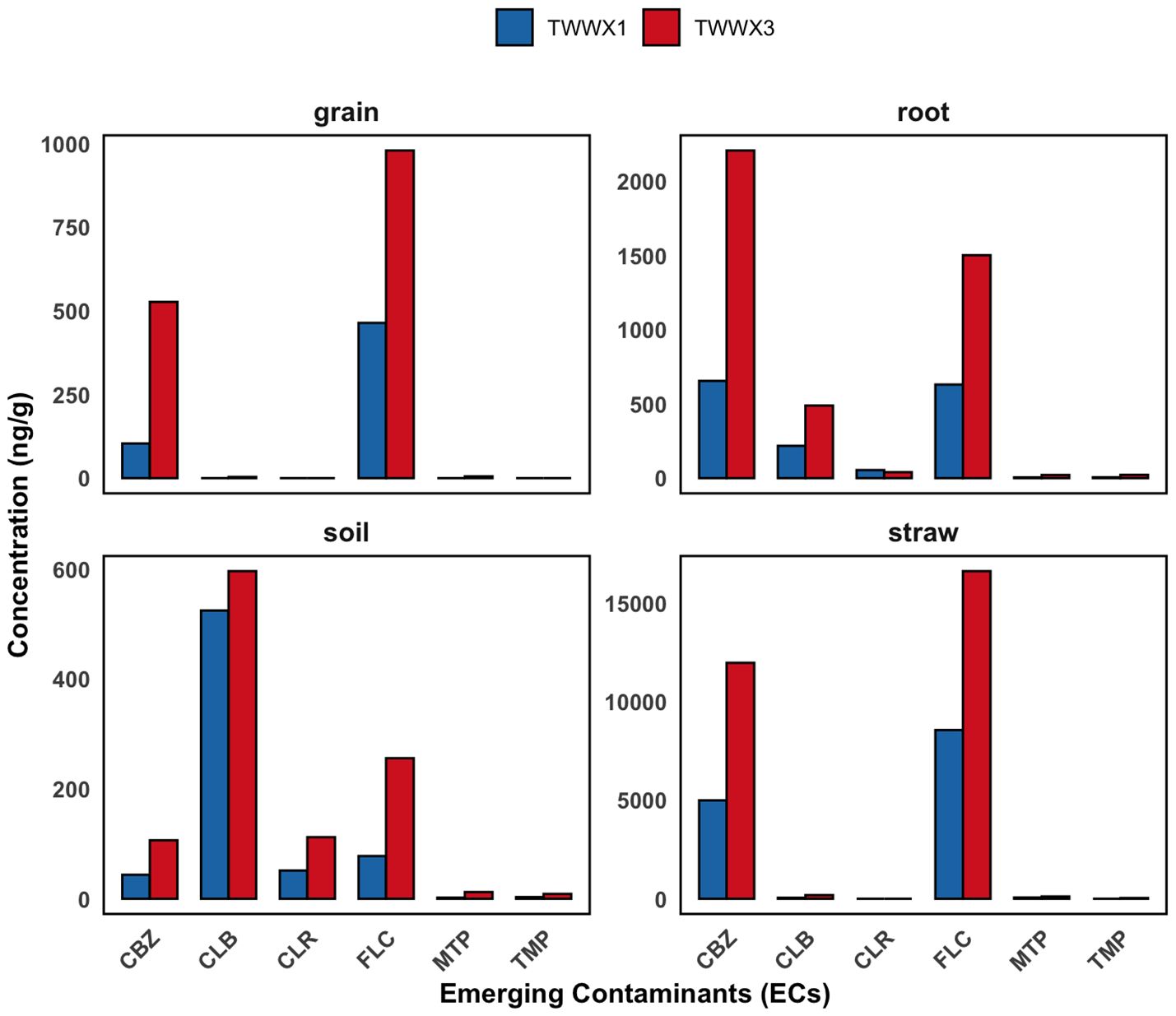
Figure 4. Concentrations of ECs (ng/g) found in soil and tissues of durum wheat cultivation, related to TWWx1 and TWWx3.
The grain analysis of durum wheat grown under the TWWx1 and TWWx3 treatments, which received treated wastewater with different cumulative concentrations of ECs, revealed varying EC levels in the grain (Table 4). Specifically, in the TWWx1 treatment, the concentrations of fluconazole (FLC) and carbamazepine (CBZ) were 464.26 ng/g and 103.73 ng/g, respectively (Figure 4). However, these concentrations increased to 979.51 ng/g for FLC and 526.89 ng/g for CBZ in the TWWx3 treatment, which received a higher cumulative dose of ECs in the irrigation water (Figure 4). This observed increase in grain EC concentrations with higher EC levels in the irrigation water demonstrates a clear dose-response relationship. The higher the cumulative concentration of ECs applied through the treated wastewater irrigation, the greater the accumulation of these contaminants in the durum wheat grain.
The data suggest that higher concentrations of ECs in irrigation water and soil lead to significant accumulation in grain, posing concerns about food safety and human health. Statistical comparisons between plant sections revealed that carbamazepine (CBZ) concentrations were significantly higher in straw than in other components of the system, with values of 4999.10 ng/g in TWWx1 and 11985.81 ng/g in TWWx3. This aligns with the findings of Martínez-Piernas et al. (35), who reported higher CBZ concentrations in leaves than in fruits. Furthermore, the levels of CLR in roots and soil differed significantly between the TWWx1 and TWWx3 treatments, supporting the selective absorption dynamics proposed by Camacho-Arévalo et al. (33). The concentrations of CLB in soil and plant sections, indicating bioaccumulation. This is substantiated by the soil concentrations for the TWWx1 and TWWx3 treatments, which were 524.73 ng/g and 596.39 ng/g, respectively. In contrast, the absence of DCF in all matrices across all treatments suggests its probable degradation, which is consistent with the findings of Christou et al. (18). The lack of DCF detection in the soil-plant system indicates that this particular EC may be more readily degraded or transformed compared to the other contaminants studied.
The presence of FLC in straw, roots, and grain in both TWWx1 and TWWx3 treatments confirms its significant absorption and translocation within the plant system. This finding aligns with the results reported by other authors (20, 36–38). In contrast, the absence of KTP, NAP, and SMX in plant and soil samples suggests poor absorption or breakdown of these contaminants. The variations observed in the presence of metoprolol (MTP) between the TWWx1 and TWWx3 treatments demonstrate that environmental circumstances and agricultural methods have a significant impact on the behavior of emerging contaminants. The detection of MTP in roots and soil only in the TWWx3 treatment confirms its absorption, as noted by Pico et al. (39). Similarly, the low concentrations of GFB and TCS in roots and soil suggest limited absorption capacity or degradation propensity of these contaminants. Our analysis highlights that the absorption and retention of ECs are significantly affected by the irrigation regime with treated wastewater. The marked differences are a result of the EC concentration in the irrigation water, the physicochemical properties of the contaminants, and the plants’ intrinsic capacity for assimilation and translocation. These findings underscore the importance of considering the complex interactions between irrigation water quality, contaminant characteristics, and plant-specific factors when assessing the fate and behavior of emerging pollutants in agricultural systems.
3.3 Bioconcentration and translocation factors
The uptake of pollutants from soil into plants is a fundamental process through which humans are exposed to ECs via the food chain. To quantify the accumulation of ECs in different plant tissues, we calculated the bioconcentration factor (BCF), which compares the concentrations of individual contaminants measured in each part of the crop to the soil concentration (33, 40). Table 5 presents the BCF values for the detected ECs in plant tissues and how they are affected by the irrigation water types (TWWx1 and TWWx3). The BCF values indicate a significant accumulation of CBZ, FLC, MTP, and TMP in the straw (leaves and culm) of wheat compared to the root and grain. CLR, KTP, GFB, and TCS show a general tendency to accumulate only in the root, with their performance varying according to the irrigation treatment (TWWx1 versus TWWx3). Importantly, the BCF values calculated for each crop part either decrease or remain essentially unchanged in the TWWx3 treatment compared to the TWWx1 treatment. Studies have reported that a BCF ≤1 indicates that even if the plant uptakes the pollutants, there is no significant accumulation in the plant parts (41, 42) including ECs (12). In contrast, a BCF >1 suggests that the plant accumulates the ECs in the root, shoot, or edible fractions. Low BCF values indicate that the plant tends to exclude the chemical element from its tissues. While CBZ and FLC in the TWWx1 and TWWx3 irrigation treatments had the highest BCF values (2.4 ± 0.4 versus 4.4 ± 0.7 and 5.5 ± 0.6 versus 3.9 ± 0.9), suggesting a tendency to accumulate significantly in the grain, the BCF values for the other studied ECs indicate that they do not dangerously accumulate in the edible part of the wheat (grain), as shown by BCF values less than or close to 1.
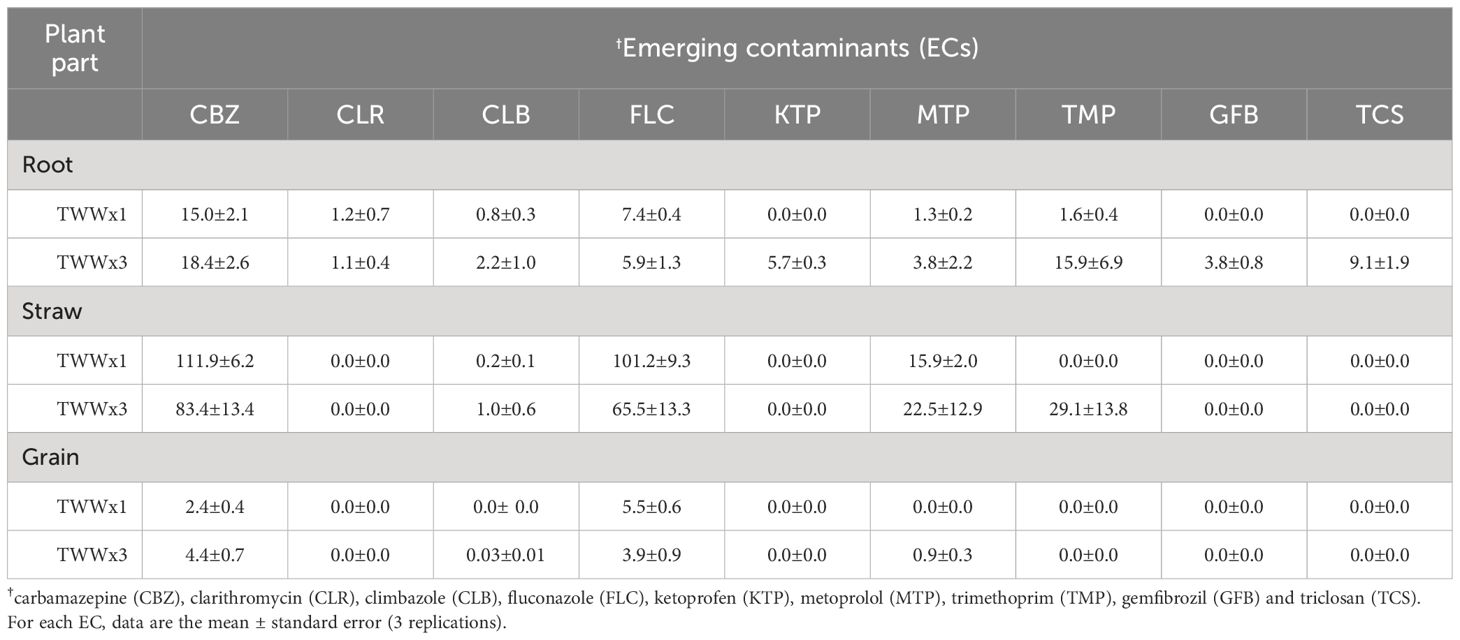
Table 5. Bioconcentration factor (BCF) values of ECs in different plant parts (root, straw, and grain) related to irrigation treatments (TWWx1 and TWWx3).
To evaluate the translocation of different ECs from the roots to the aerial parts of the durum wheat plant (straw and grain), the translocation factor (TF) was calculated as the ratio of the compound’s concentration in the aerial part to its concentration in the roots. Table 6 presents the TF values for the detected ECs in plant tissues based on the irrigation water types (TWWx1 and TWWx3). Diclofenac (DCF), naproxen (NAP), and sulfamethoxazole (SMX) were not quantified (below the limit of quantification) in the different plant parts (roots, straw, and grain); therefore, their TF values were not determined. TF values greater than 1 indicate that compounds are more likely to move from the roots to other parts of the plant rather than accumulate in the roots (40). The study revealed that carbamazepine (CBZ), fluconazole (FLC), and metoprolol (MTP) are the most effective in moving from the roots to the straw. These molecules show TF values between 5.43 and 15.42, which do not differ significantly between the two irrigation treatments (TWWx1 and TWWx3). Since the TF is expressed as the ratio of the EC concentration in the aerial part of the plant to the roots, CBZ, FLC, and MTP compounds showed higher concentrations in the straw (leaf and culm) compared to the roots, indicating an important root-to-straw translocation.
Table 5 presents the bioconcentration factor (BCF) values for the TWWx1 and TWWx3 irrigation treatments, considering different plant parts (root, straw, and grain) for each emerging contaminant (EC) separately. To integrate these data, a multivariate approach using principal component analysis (PCA) was employed (Table 7). The PCA allowed for the evaluation of which ECs, when considered simultaneously, contribute most to the differences between irrigation treatments and plant parts. The PCA results identified two factors that explain 45.7% and 28.8% of the total variance, respectively (Table 7). These factors represent the most significant contributors to the observed differences in the dataset. The PCA analysis provides a comprehensive overview of the relationships between the ECs, irrigation treatments, and plant parts, highlighting the key factors driving the observed variations.
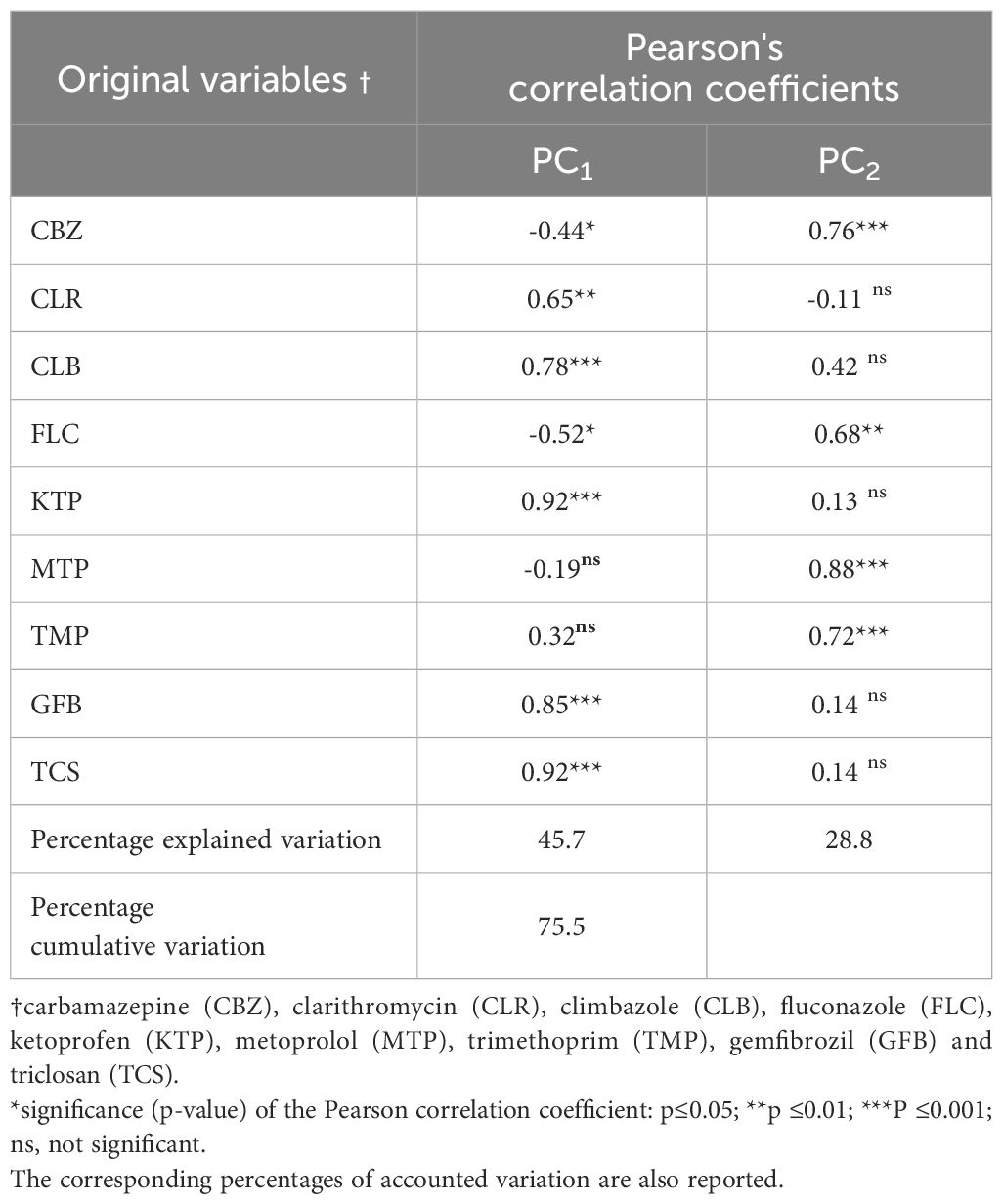
Table 7. Pearson's correlation coefficient values for the first two principal components (PC1-2), considering the original variables.
The PCA revealed that the first factor (PC1) was strongly and positively correlated with the following emerging contaminants: clarithromycin (CLR), climbazole (CLB), ketoprofen (KTP), gemfibrozil (GFB), and triclosan (TCS) (p-value <0.01). This suggests that PC1 could represent a new factor linked to low root uptake and limited translocation of these ECs within the plant system. In contrast, the second factor (PC2) was positively associated with carbamazepine (CBZ), fluconazole (FLC), metoprolol (MTP), and trimethoprim (TPM) molecules (p-value < 0.01). PC2 can be considered a new factor resulting from the PCA, which is linked to high root uptake and the increased capacity of these ECs to move from the roots toward the aerial parts (straw and grain) of the wheat crop.
Figure 5 shows the biplot resulting from the principal component analysis. Based on this, the TWWx1 and TWWx3 irrigation treatments were separated along PC1, which is associated with low root uptake (particularly for the TWWx3 treatment) and reduced translocation to the aerial parts of the crop. Moreover, the plant parts (root, straw, and grain) were differentiated based on both PC1 and PC2. Specifically, PC2 was linked to high root uptake and increased translocation within the plant.
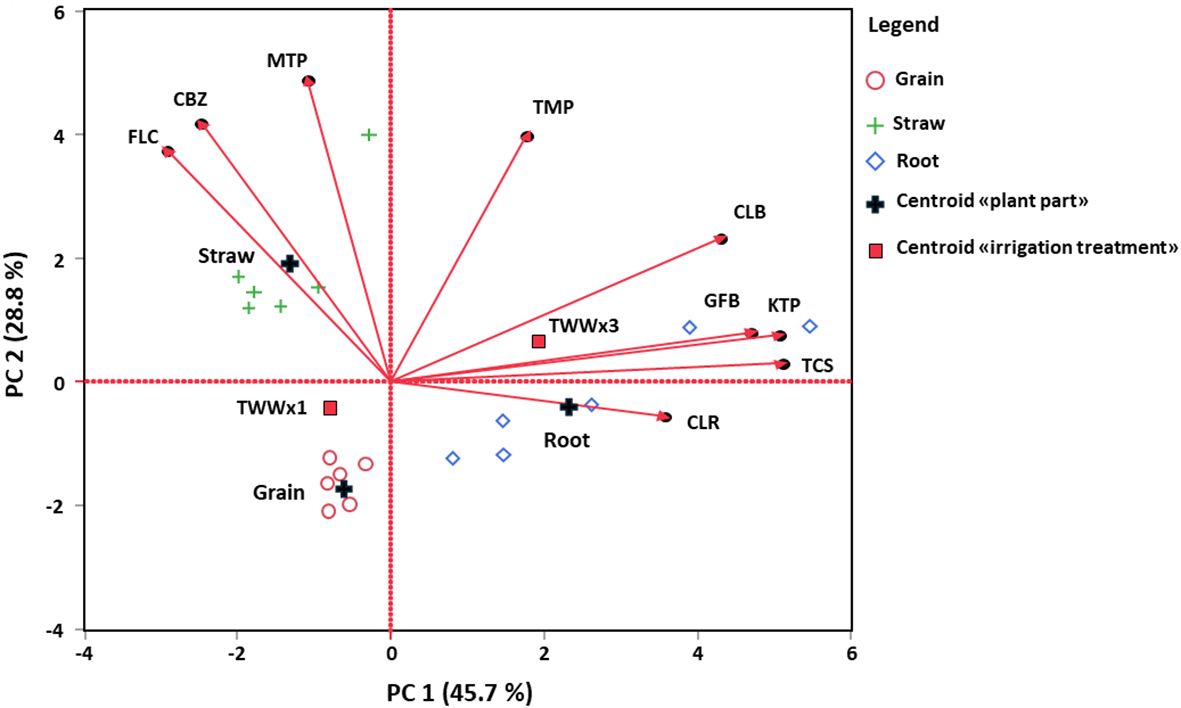
Figure 5. Biplot relative to the PCA analysis performed on BCF values related to different ECs: carbamazepine (CBZ), clarithromycin (CLR), climbazole (CLB), fluconazole (FLC), ketoprofen (KTP), metoprolol (MTP), trimethoprim (TMP), gemfibrozil (GFB) and triclosan (TCS).
The results from the principal component analysis are consistent with the bioconcentration factor (BCF) and translocation factor (TF) values reported in Tables 5, 6. Specifically, carbamazepine (CBZ), fluconazole (FLC), metoprolol (MTP), and trimethoprim (TPM) molecules exhibited high values (>1) for both BCF and TF, indicating their ability to accumulate and translocate effectively from the roots to the aerial parts of the plant. In contrast, clarithromycin (CLR), climbazole (CLB), ketoprofen (KTP), gemfibrozil (GFB), and triclosan (TCS) molecules showed values less than 1, particularly for TF, which suggests their limited ability to translocate from the roots toward the aerial parts of the plant. This difference in translocation efficiency is consistent with the observed differences in the bioaccumulation of these molecules in various plant parts.
3.4 Mass balance analysis of ECs using lysimeter technique
The lysimetric approach employed in this study was essential for conducting a detailed examination of how 12 ECs move through the soil, plant, and leachate compartments. The mass balance of ECs obtained using this technique offers a comprehensive analysis of the distribution, accumulation, and transformation of these pollutants within the soil-plant-water environment. The statistical analysis showed significant differences between each analyzed EC and the lysimetric system (Tables 8, 9). The lysimeter system encompasses all components of the mass balance, including grain, roots, plants, soil, drainage, and the unaccounted fraction, which is calculated as the residual of the mass balance for each EC. The percentage contribution of each component to the mass balance is computed relative to the total EC intake, considering their initial presence at the beginning of the study (T0).
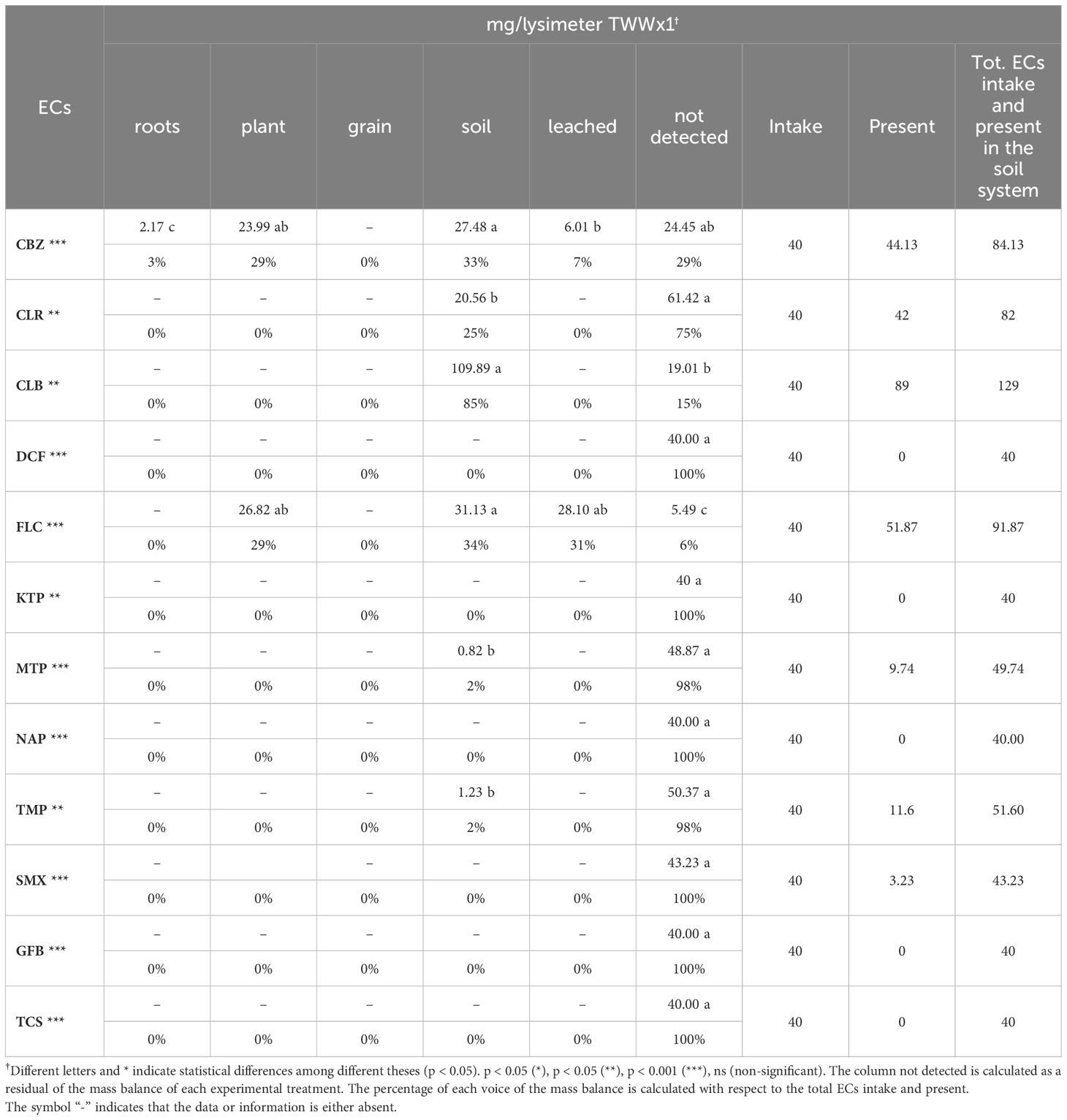
Table 8. Total intake and ECs accumulation in plants, leached water, and soil lysimeters in the TWWx1 treatment (mean values of three replicates are shown).
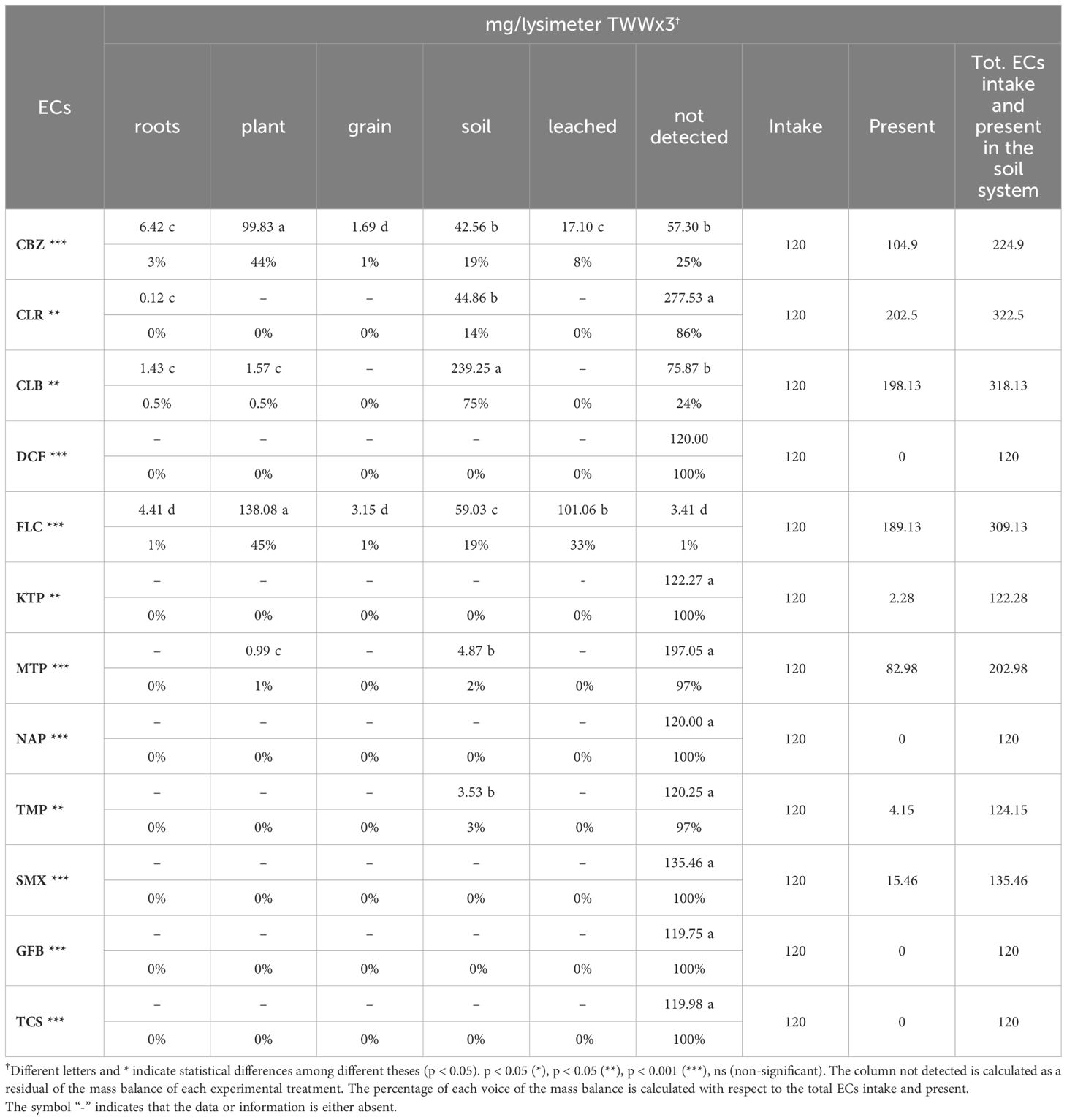
Table 9. Total intake and ECs accumulation in plants, leached water, and soil lysimeters in the TWWx3 treatment (mean values of three replicates are shown).
The examination of the results reveals considerable differences in the behavior of these emerging contaminants (ECs), particularly when subjected to varying EC concentrations in treated wastewater (Figure 6). This comprehensive study provides insights into the fate and transit of ECs in agroecosystems, as well as their potential effects on soil health, plant absorption, and environmental contamination.
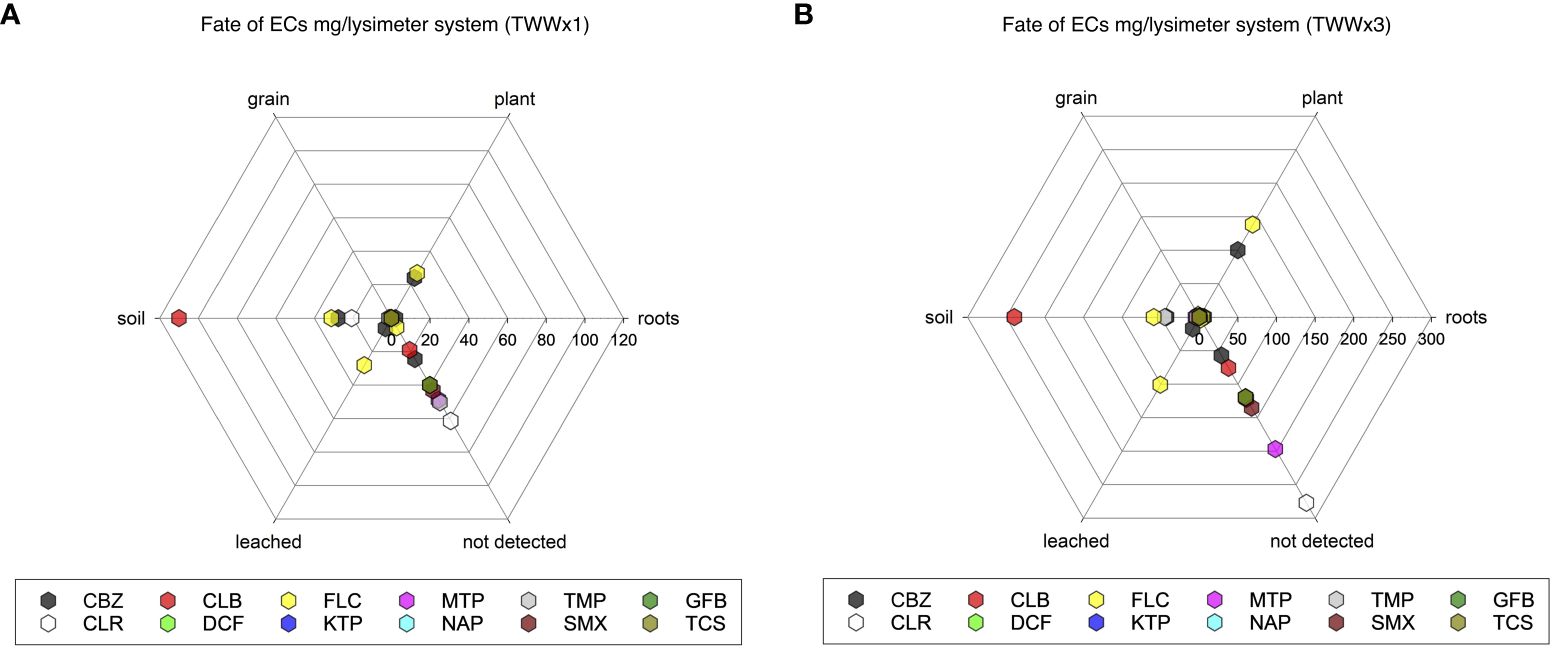
Figure 6. Mass balance analysis and the fate of ECs when subjected to the treatment TWWx1 (A) and TWWx3 treatment (B). The amount of ECs in soil-leached water and plant is in mg/lysimeter.
The distribution of CBZ in the TWWx1 system reveals extensive dispersion, with 3% of the total amount found in the roots (2.17 mg/lysimeter) and 33% in the soil (27.48 mg/lysimeter). In contrast, the TWWx3 treatment shows that the majority of CBZ (44%) is present in the aerial plant components (99.83 mg/lysimeter), consistent with the 29% measured in TWWx1. This suggests a substantial absorption capacity by plants, accompanied by lower soil build-up (19%) and increased leaching (17.10 mg/lysimeter, 8%). These findings are consistent with previous studies that have shown higher bioconcentration of CBZ in leaves compared to roots. However, it is important to note that the concentrations of CBZ in leaves can vary significantly between studies due to differences in exposure conditions such as medium concentration, exposure length, and plant species features.
The distribution of FLC under the TWWx1 treatment shows a considerable presence in both the plant and soil, with a higher proportion of leaching (28.10 mg/lysimeter, 31%) compared to CBZ. This indicates greater mobility in the soil for FLC.
The distribution of CBZ in the TWWx1 system shows extensive dispersion, with 3% of the total amount found in the roots (2.17 mg/lysimeter) and a significant portion (33%) in the soil (27.48 mg/lysimeter). However, under the TWWx3 treatment, the majority of CBZ (44%) is detected in the aerial plant components (99.83 mg/lysimeter), consistent with the 29% measured in TWWx1. This suggests that plants have a substantial capacity to absorb CBZ, which is accompanied by lower soil accumulation (19%) and a notable increase in CBZ leaching (17.10 mg/lysimeter, 8%). This pattern aligns with previous findings in plants, where CBZ bioconcentration is reported to be higher in leaves compared to roots (36, 38, 43, 44), supporting the observations in this study. It is important to note that the concentrations of CBZ in leaves can vary significantly between studies due to differences in exposure conditions, such as medium concentration, exposure duration, and individual plant species characteristics.
The examination of FLC distribution under the TWWx1 treatment reveals a considerable presence in both the plant and soil, with a higher proportion of leaching (28.10 mg/lysimeter, 31%) compared to CBZ. This suggests that FLC exhibits greater mobility in the soil than CBZ. Under the TWWx3 treatment, FLC concentrations in the aerial plant parts account for 45% (138.08 mg/lysimeter), and the leaching percentage is 33% (101.06 mg/lysimeter). These findings indicate an increased capacity for FLC to move through soil and water, consistent with earlier studies (20, 36).
In treatment TWWx1, CLR was not detected in roots, plants, grains, or leachate water. However, significant amounts were found in the soil, with 20.56 mg/lysimeter representing 25% of the total (Table 8). The majority of CLR, amounting to 61.42 mg/lysimeter (75%), was not detected, suggesting possible degradation or transport to unaccounted compartments. Similarly, in treatment TWWx1, CLB was not detected in roots, plants, grains, or leachate water. Significant presence was found in the soil, with 109.89 mg/lysimeter representing 85% of the total, while 19.01 mg/lysimeter (15%) went undetected (Table 8). This behavior was observed for CLR and CLB in treatment TWWx3, confirming the behavior of these ECs (Table 9). Other ECs, such as DCF, KTP, MTP, NAP, TMP, SMX, GFB, and TCS, were not found within the lysimetric system (Tables 8, 9). The lack of detection increases the likelihood of total degradation or concentrations below the detection limit. The absence of these chemicals in the leachate suggests that they were not transferred in considerable quantities through the soil or water. The undetected ECs raise concerns regarding the development of unrecognized metabolites, as well as their interaction and immobilization within soil or plant matrices, emphasizing the complexities of these pollutants’ destiny and behavior in agroecosystems.
4 Discussion
Reusing treated wastewater for irrigation introduces contaminants of ECs to the agricultural environment, potentially contaminating the environment and food chain. This study examined the presence of PPCP compounds in the soil-cereal system in Southern Italy. The findings of this study significantly enhance our understanding of the environmental fate of ECs in farm systems, particularly in the context of irrigation with treated wastewater (TWW) in Mediterranean environments. Our study reveals that the varied accumulation of ECs, such as carbamazepine (CBZ) and fluconazole (FLC), in plant tissues raises serious concerns regarding the safety of food grown under these conditions. These ECs have higher concentrations than the other pollutants. This conclusion may be attributed to (i) the intrinsic properties of each pollutant that influenced such behavior (45) and (ii) the variable degradability of numerous substances by the microbial community or by photodegradation/oxidation processes (46). Furthermore, CBZ and FLC were the only ECs detected in straw and grain (47), which found that their chemical characteristics and molecular structures influence plant absorption of chemicals. Plant roots readily absorb compounds with moderate lipophilicity (0 < Kow < 3) and molecular weights less than 500 (48). In this sense, CBZ and FLC were in the ideal range for plant translocation (49).
In line with the findings of the PCA, the effects of ECs on the soil-plant system, as determined by BCF, categorize ECs into two distinct groups. The first group comprises ECs with low root uptake and plant translocation, which, under our experimental conditions, primarily exist in anionic forms (such as KTP and GFB) or have high molecular weights (such as CLB). These properties lead to their sorption by the soil particles, thereby reducing their absorption and translocation in the aerial parts of the plant (34, 50). The second group of emerging contaminants (ECs) with high root uptake and translocation is characterized by cationic (MTP and TPM) or non-ionic compounds (CBZ and FLC). MTP is a positively charged compound that exhibits persistent behavior, leading to its accumulation in the soil (51) and prolonged availability for plants in the rhizosphere. TPM can exist in both neutral and cationic forms in the rhizosphere (52). Consequently, TPM can be absorbed by the negatively charged root surface and leaves, both as a cationic and neutral compound. This allows TPM to reach aboveground tissues through the transpiration flow, ultimately impacting leafy and marketable yields (34, 53). CBZ, being non-ionic and with a low molecular weight (54), can easily pass through root membranes and accumulate in leaves, as documented in numerous studies (55). Hydrophobicity is considered the most critical characteristic of neutral compounds in terms of plant uptake dynamics (43). Consequently, the hydrophilic nature of CBZ affects its presence in soil pore water and its ease of uptake by plants. CBZ’s low hydrophobicity may also explain its continuous translocation in plant aerial tissues (43). Other studies have found higher CBZ concentrations in the aerial sections of plants than in the roots (56), suggesting passive uptake unrestricted by the root membrane. García-Valcárcel et al. (57) observed that FLC is more prevalent in straw compared to other ECs, implying it can easily diffuse through the root membrane According to Herklotz et al. (58), FLC’s lowest pKa (2.27) compared to other studied ECs encourages its absorption and translocation in plants, with cabbage and Brassica rapa showing the highest concentrations of ECs with the lowest pKa. This variability underscores complex interactions within agroecosystems involving ECs, as noted in previous studies (20, 29, 59–61), which report diverse EC behaviors in different agricultural settings. The significant accumulation of CBZ in straw and substantial leaching of FLC necessitates a deeper understanding of the mobility and stability of these pollutants, as emphasized by Pérez et al. (38), who highlighted the varied mobility of different ECs in agricultural soils. The absence of specific compounds such as DCF, NAP, and SMX from plant and soil matrices, indicating either complete degradation or non-uptake by plants, aligns with findings from Ponce-Robles et al. (62) and Rout et al. (63), demonstrating uneven uptake of certain ECs by crops.
The increasing reliance on treated wastewater (TWW), especially exacerbated by severe droughts in Europe during 2018-2020 (64), underscores the importance of understanding these dynamics. Hochstrat et al. (65) and Beard et al. (66) highlight the growing popularity of TWW use in both arid and temperate climates, emphasizing the need for effective management practices. Furthermore, the detection of emerging contaminants (ECs) such as FLC and CBZ in edible parts of crops necessitates immediate attention due to potential risks to consumer health. This concern is echoed by findings from Denora et al. (20), who also identified ECs in edible crop parts. The re-evaluation of wastewater treatment processes, as suggested by Verlicchi et al. (67), becomes imperative to mitigate these risks. The significant leaching of certain contaminants suggests potential broader environmental impacts extending beyond immediate agricultural contexts. This aligns with concerns raised by Khan and Barros (68) regarding the environmental implications of using TWW in agriculture. The presence of contaminants in the environment could have far-reaching effects on ecosystems and human health, necessitating the development of comprehensive guidelines and policies for the safe use of TWW, as recommended by Verlicchi et al. (67).
Our study contributes to a growing body of research that seeks to balance the benefits of TWW utilization in agriculture with the protection of ecosystems and human health. It highlights the need for an integrated approach considering both the agronomic and social implications of TWW use, as advocated by Hashem and Qi (69) and Shi et al. (61). Future research endeavors should focus on elucidating the mechanisms of contaminant uptake and translocation in different plant species under varying irrigation conditions. Long-term studies investigating the impact of emerging contaminants on soil microbial communities and ecosystem dynamics are essential for a holistic understanding of the ecological implications of contaminant exposure in agricultural settings.
5 Conclusions
The use of lysimeter techniques for mass balance analysis has confirmed the accuracy of measuring the behavior of emerging contaminants (ECs), thereby deepening our understanding and serving as a valuable benchmark for subsequent studies. The presence of ECs like fluconazole (FLC) and carbamazepine (CBZ) in the edible parts of crops demands immediate scientific and regulatory attention due to the potential health risks to consumers.
Considering that the concentrations of the ECs in the spiked treated wastewater (TWW) were higher than those in the original TWW, it is reasonable to expect their potential entry into the food chain. The absence of certain compounds, including diclofenac (DCF), naproxen (NAP), and sulfamethoxazole (SMX), in both plant and soil samples indicatesevi their complete degradation or non-uptake by the crops, aligning with research demonstrating selective EC uptake by different plant species.
Additionally, the substantial leaching observed for some contaminants points to potential extensive environmental repercussions, further emphasizing the urgency for comprehensive guidelines and policies governing the use of TWW in agriculture. This study aligns with the recommendations made by Verlicchi et al. (67), who advocate for a comprehensive strategy to safeguard environmental, animal, and human health. They call for a concise list of priority ECs amidst the increasing chemical diversity and recommend enhancing monitoring efforts, standardizing research methodologies, investigating EC persistence, bioaccumulation, toxicity, and fate in soil and crops, as well as developing predictive models. These recommendations aim to promote the sustainable and safe use of regenerated water in agriculture, with a keen focus on public health and environmental protection. Our research supports and extends these suggestions by demonstrating the effectiveness of lysimeter techniques in evaluating EC behavior, thereby contributing to the development of more informed and precise guidelines for the use of treated wastewater in agricultural settings.
Data availability statement
The original contributions presented in the study are included in the article/supplementary material. Further inquiries can be directed to the corresponding author.
Author contributions
MD: Conceptualization, Data curation, Formal analysis, Methodology, Resources, Writing – original draft. AM: Data curation, Formal analysis, Writing – original draft, Writing – review & editing. VC: Investigation, Methodology, Project administration, Resources, Writing – review & editing, Supervision. GB: Methodology, Project administration, Supervision, Writing – review & editing. FD: Formal analysis, Validation, Writing – review & editing, Resources. SM: Resources, Writing – review & editing. CD: Resources, Writing – review & editing. GG: Conceptualization, Formal analysis, Methodology, Project administration, Resources, Supervision, Writing – original draft, Writing – review & editing. MG: Conceptualization, Writing – review & editing. CF: Investigation, Writing – review & editing. MP: Conceptualization, Methodology, Project administration, Resources, Supervision, Writing – review & editing.
Funding
The author(s) declare financial support was received for the research, authorship, and/or publication of this article. This study was carried out within the Agritech National Research Center and received funding from the European Union Next-Generation EU (PIANO NAZIONALE DI RIPRESA E RESILIENZA (PNRR) – MISSIONE 4 COMPONENTE 2, INVESTIMENTO 1.4 – D.D. 1032 17/06/2022, CN00000022, CUP: C33C22000250001), as well as from the Ministero dell’Istruzione dell’Università e della Ricerca, Italy, grant number 2017C5CLFB.
Conflict of interest
The authors declare that the research was conducted in the absence of any commercial or financial relationships that could be construed as a potential conflict of interest.
Publisher’s note
All claims expressed in this article are solely those of the authors and do not necessarily represent those of their affiliated organizations, or those of the publisher, the editors and the reviewers. Any product that may be evaluated in this article, or claim that may be made by its manufacturer, is not guaranteed or endorsed by the publisher.
Author disclaimer
This manuscript solely reflects the views and opinions of the authors; neither the European Commission nor the Ministry can be held responsible for them.
References
1. Pawlak K, Kołodziejczak M. The role of agriculture in ensuring food security in developing countries: considerations in the context of the problem of sustainable food production. Sustainability. (2020) 12:5488. doi: 10.3390/su12135488
2. Naeem M, Gill R, Gill SS, Singh K, Sofo A, Tuteja N. Editorial: Emerging contaminants and their effect on agricultural crops. Front Plant Sci. (2023) 14:1296252. doi: 10.3389/fpls.2023.1296252
3. Bayabil HK, Teshome FT, Li YC. Emerging contaminants in soil and water. Front Environ Sci. (2022) 10:873499. doi: 10.3389/fenvs.2022.873499
4. Radwan EK, Abdel Ghafar HH, Ibrahim MBM, Moursy AS. Recent trends in treatment technologies of emerging contaminants. Environ Qual Manage. (2023) 32:7–25. doi: 10.1002/tqem.21877
5. Beltrán EM, Fernández-Torija C, Pablos MV, Porcel MÁ, García-Hortigüela P, González-Doncel M. The effect of PFOs on the uptake and translocation of emerging contaminants by crops cultivated under soil and soilless conditions. Ecotoxicol Environ Saf. (2021) 215:112103. doi: 10.1016/j.ecoenv.2021.112103
6. Sairam M, Maitra S, Praharaj S, Nath S, Shankar T, Sahoo U, et al. An insight into the consequences of emerging contaminants in soil and water and plant responses. Cham, Switzerland: Springer (2023). pp. 1–27. doi: 10.1007/978-3-031-22269-6_1
7. Pradhan B, Chand S, Chand S, Rout PR, Naik SK. Emerging groundwater contaminants: A comprehensive review on their health hazards and remediation technologies. Groundw Sustain Dev. (2023) 20:100868. doi: 10.1016/j.gsd.2022.100868
8. Canaj K, Mehmeti A, Morrone D, Toma P, Todorović M. Life cycle-based evaluation of environmental impacts and external costs of treated wastewater reuse for irrigation: A case study in southern Italy. J Clean Prod. (2021) 293:126142. doi: 10.1016/j.jclepro.2021.126142
9. Picó Y, Alvarez-Ruiz R, Alfarhan AH, El-Sheikh MA, Alobaid SM, Barceló D. Uptake and accumulation of emerging contaminants in soil and plant treated with wastewater under real-world environmental conditions in the Al Hayer area (Saudi Arabia). Sci Total Environ. (2019) 652:562–72. doi: 10.1016/j.scitotenv.2018.10.224
10. Shahriar A, Tan J, Sharma P, Hanigan D, Verburg P, Pagilla K, et al. Modeling the fate and human health impacts of pharmaceuticals and personal care products in reclaimed wastewater irrigation for agriculture. Environ Pollut. (2021) 276:116532. doi: 10.1016/j.envpol.2021.116532
11. Osuoha JO, Anyanwu BO, Ejileugha C. Pharmaceuticals and personal care products as emerging contaminants: Need for combined treatment strategy. J Hazard Mater Adv. (2023) 9:100206. doi: 10.1016/j.hazadv.2022.100206
12. Sunyer-Caldú A, Quintana G, Diaz-Cruz MS. Factors driving PPCPs uptake by crops after wastewater irrigation and human health implications. Environ Res. (2023) 237:116923. doi: 10.1016/j.envres.2023.116923
13. Keerthanan S, Jayasinghe C, Biswas JK, Vithanage M. Pharmaceutical and Personal Care Products (PPCPs) in the environment: Plant uptake, translocation, bioaccumulation, and human health risks. Crit Rev Environ Sci Technol. (2021) 51:1221–58. doi: 10.1080/10643389.2020.1753634
14. Colon B, Toor GS. A review of uptake and translocation of pharmaceuticals and personal care products by food crops irrigated with treated wastewater. Amsterdam, Netherlands: Elsevier (2016). pp. 75–100. doi: 10.1016/bs.agron.2016.07.001
15. Wu W, Ma M, Hu Y, Yu W, Liu H, Bao Z. The fate and impacts of pharmaceuticals and personal care products and microbes in agricultural soils with long term irrigation with reclaimed water. Agric Water Manag. (2021) 251:106862. doi: 10.1016/j.agwat.2021.106862
16. Li Y, Liu H, Xing W, Wang J, Fan H. Effects of irrigation water quality on the presence of pharmaceutical and personal care products in topsoil and vegetables in greenhouses. Environ Sci pollut Res. (2022) 30:13726–38. doi: 10.1007/s11356-022-22753-y
17. Li Y, Liu H, Wang J, Xing W, Fan H, Li B. Impacts of reclaimed water irrigation on the accumulation of pharmaceutical and personal care products in soil and cereals. Irrig Sci. (2024) 42:419–30. doi: 10.1007/s00271-023-00868-5
18. Christou A, Karaolia P, Hapeshi E, Michael C, Fatta-Kassinos D. Long-term wastewater irrigation of vegetables in real agricultural systems: Concentration of pharmaceuticals in soil, uptake and bioaccumulation in tomato fruits and human health risk assessment. Water Res. (2017) 109:24–34. doi: 10.1016/j.watres.2016.11.033
19. González García M, Fernández-López C, Polesel F, Trapp S. Predicting the uptake of emerging organic contaminants in vegetables irrigated with treated wastewater – Implications for food safety assessment. Environ Res. (2019) 172:175–81. doi: 10.1016/j.envres.2019.02.011
20. Denora M, Candido V, Brunetti G, De Mastro F, Murgolo S, De Ceglie C, et al. Uptake and accumulation of emerging contaminants in processing tomato irrigated with tertiary treated wastewater effluent: a pilot-scale study. Front Plant Sci. (2023) 14:1238163. doi: 10.3389/fpls.2023.1238163
21. Hussain A, Priyadarshi M, Dubey S. Experimental study on accumulation of heavy metals in vegetables irrigated with treated wastewater. Appl Water Sci. (2019) 9:122. doi: 10.1007/s13201-019-0999-4
22. Murrell KA, Teehan PD, Dorman FL. Determination of contaminants of emerging concern and their transformation products in treated-wastewater irrigated soil and corn. Chemosphere. (2021) 281:130735. doi: 10.1016/j.chemosphere.2021.130735
23. Liu X, Liu W, Tang Q, Liu B, Wada Y, Yang H. Global agricultural water scarcity assessment incorporating blue and green water availability under future climate change. Earths Future. (2022) 10. doi: 10.1029/2021EF002567
24. Manasfi R, Brienza M, Ait-Mouheb N, Montemurro N, Perez S, Chiron S. Impact of long-term irrigation with municipal reclaimed wastewater on the uptake and degradation of organic contaminants in lettuce and leek. Sci Total Environ. (2021) 765:142742. doi: 10.1016/j.scitotenv.2020.142742
25. Masia S, Trabucco A, Spano D, Snyder RL, Sušnik J, Marras S. A modelling platform for climate change impact on local and regional crop water requirements. Agric Water Manag. (2021) 255:107005. doi: 10.1016/j.agwat.2021.107005
26. Ben Mordechay E, Mordehay V, Tarchitzky J, Chefetz B. Pharmaceuticals in edible crops irrigated with reclaimed wastewater: Evidence from a large survey in Israel. J Hazard Mater. (2021) 416:126184. doi: 10.1016/j.jhazmat.2021.126184
27. Candido V, Campanelli G, D’Addabbo T, Castronuovo D, Renco M, Camele I. Growth and yield promoting effect of artificial mycorrhization combined with different fertiliser rates on field-grown tomato. Ital J Agron. (2013) 8:22. doi: 10.4081/ija.2013.e22
28. Allen R, Gr. GA. Crop EvapotranspirationGuidelines for Computing Crop Water Requirements. Food and Agriculture Organisation of the United Nations (FAO) Irrigation and Drainage Paper No 56 (1998). Rome. Available online at: https://www.fao.org/3/x0490e/x0490e00.htm (Accessed October 13, 2022).
29. De Mastro F, Cocozza C, Traversa A, Cacace C, Mottola F, Mezzina A, et al. Validation of a modified QuEChERS method for the extraction of multiple classes of pharmaceuticals from soils. Chem Biol Technol Agric. (2022) 9:49. doi: 10.1186/s40538-022-00305-3
30. Díez C, Traag WA, Zommer P, Marinero P, Atienza J. Comparison of an acetonitrile extraction/partitioning and “dispersive solid-phase extraction” method with classical multi-residue methods for the extraction of herbicide residues in barley samples. J Chromatogr A. (2006) 1131:11–23. doi: 10.1016/j.chroma.2006.07.046
31. Pizzutti IR, de Kok A, Zanella R, Adaime MB, Hiemstra M, Wickert C, et al. Method validation for the analysis of 169 pesticides in soya grain, without clean up, by liquid chromatography–tandem mass spectrometry using positive and negative electrospray ionization. J Chromatogr A. (2007) 1142:123–36. doi: 10.1016/j.chroma.2006.12.030
32. Walorczyk S. Development of a multi-residue method for the determination of pesticides in cereals and dry animal feed using gas chromatography–tandem quadrupole mass spectrometry. J Chromatogr A. (2008) 1208:202–14. doi: 10.1016/j.chroma.2008.08.068
33. Camacho-Arévalo R, García-Delgado C, Mayans B, Antón-Herrero R, Cuevas J, Segura ML, et al. Sulfonamides in tomato from commercial greenhouses irrigated with reclaimed wastewater: uptake, translocation and food safety. Agronomy. (2021) 11:1016. doi: 10.3390/agronomy11051016
34. Mininni AN, Pietrafesa A, Calabritto M, Di Biase R, Brunetti G, De Mastro F, et al. Uptake and translocation of pharmaceutically active compounds by olive tree (Olea europaea L.) irrigated with treated municipal wastewater. Front Plant Sci. (2024) 15:1382595. doi: 10.3389/fpls.2024.1382595
35. Martínez-Piernas AB, Plaza-Bolaños P, Fernández-Ibáñez P, Agüera A. Organic microcontaminants in tomato crops irrigated with reclaimed water grown under field conditions: occurrence, uptake, and health risk assessment. J Agric Food Chem. (2019) 67:6930–9. doi: 10.1021/acs.jafc.9b01656
36. Sochacki A, Marsik P, Chen Z, Sisa M, Vymazal J. Fate of antifungal drugs climbazole and fluconazole in constructed wetlands - Diastereoselective transformation indicates process conditions. Chem Eng J. (2021) 421:127783. doi: 10.1016/j.cej.2020.127783
37. Ben Mordechay E, Mordehay V, Tarchitzky J, Chefetz B. Fate of contaminants of emerging concern in the reclaimed wastewater-soil-plant continuum. Sci Total Environ. (2022) 822:153574. doi: 10.1016/j.scitotenv.2022.153574
38. Pérez DJ, Doucette WJ, Moore MT. Contaminants of emerging concern (CECs) in Zea mays: Uptake, translocation and distribution tissue patterns over the time and its relation with physicochemical properties and plant transpiration rate. Chemosphere. (2022) 288:132480. doi: 10.1016/j.chemosphere.2021.132480
39. Pico Y, Belenguer V, Corcellas C, Diaz-Cruz MS, Eljarrat E, Farré M, et al. Contaminants of emerging concern in freshwater fish from four Spanish Rivers. Sci Total Environ. (2019) 659:1186–98. doi: 10.1016/j.scitotenv.2018.12.366
40. Fernandes AS, Bragança I, Homem V. Personal care products in soil-plant and hydroponic systems: Uptake, translocation, and accumulation. Sci Total Environ. (2024) 912:168894. doi: 10.1016/j.scitotenv.2023.168894
41. Ackah M, Anim AK, Gyamfi ET, Zakaria N, Hanson J, Tulasi D, et al. Uptake of heavy metals by some edible vegetables irrigated using wastewater: a preliminary study in Accra, Ghana. Environ Monit Assess. (2014) 186:621–34. doi: 10.1007/s10661-013-3403-0
42. Gatta G, Gagliardi A, Disciglio G, Lonigro A, Francavilla M, Tarantino E, et al. Irrigation with treated municipal wastewater on artichoke crop: assessment of soil and yield heavy metal content and human risk. Water (Basel). (2018) 10:255. doi: 10.3390/w10030255
43. Carter LJ, Harris E, Williams M, Ryan JJ, Kookana RS, Boxall ABA. Fate and uptake of pharmaceuticals in soil–plant systems. J Agric Food Chem. (2014) 62:816–25. doi: 10.1021/jf404282y
44. Miller EL, Nason SL, Karthikeyan KG, Pedersen JA. Root uptake of pharmaceuticals and personal care product ingredients. Environ Sci Technol. (2016) 50:525–41. doi: 10.1021/acs.est.5b01546
45. De Mastro F, Brunetti G, Traversa A, Cacace C, Cocozza C. Investigation of the effect of twelve pharmaceuticals on germination and growth parameters of basil (Ocimum basilicum L.). Appl Sci. (2023) 13:6759. doi: 10.3390/app13116759
46. Ascar L, Ahumada I, Morales N, Garrido T, Giordano A, Leiva K. Mobility of nonsteroidal anti-inflammatory drugs in soils with and without amendment of biosolid. J Chilean Chem Soc. (2017) 62:3593–6. doi: 10.4067/s0717-97072017000303593
47. Kodešová R, Klement A, Golovko O, Fér M, Nikodem A, Kočárek M, et al. Root uptake of atenolol, sulfamethoxazole and carbamazepine, and their transformation in three soils and four plants. Environ Sci pollut Res. (2019) 26:9876–91. doi: 10.1007/s11356-019-04333-9
48. Yan Q, Feng G, Gao X, Sun C, Guo J, Zhu Z. Removal of pharmaceutically active compounds (PhACs) and toxicological response of Cyperus alternifolius exposed to PhACs in microcosm constructed wetlands. J Hazard Mater. (2016) 301:566–75. doi: 10.1016/j.jhazmat.2015.08.057
49. Koba O, Golovko O, Kodešová R, Fér M, Grabic R. Antibiotics degradation in soil: A case of clindamycin, trimethoprim, sulfamethoxazole and their transformation products. Environ pollut. (2017) 220:1251–63. doi: 10.1016/j.envpol.2016.11.007
50. Kodešová R, Grabic R, Kočárek M, Klement A, Golovko O, Fér M, et al. Pharmaceuticals’ sorptions relative to properties of thirteen different soils. Sci Total Environ. (2015) 511:435–43. doi: 10.1016/j.scitotenv.2014.12.088
51. Grossberger A, Hadar Y, Borch T, Chefetz B. Biodegradability of pharmaceutical compounds in agricultural soils irrigated with treated wastewater. Environ pollut. (2014) 185:168–77. doi: 10.1016/j.envpol.2013.10.038
52. Christou A, Antoniou C, Christodoulou C, Hapeshi E, Stavrou I, Michael C, et al. Stress-related phenomena and detoxification mechanisms induced by common pharmaceuticals in alfalfa (Medicago sativa L.) plants. Sci Total Environ. (2016) 557–558:652–64. doi: 10.1016/j.scitotenv.2016.03.054
53. Tanoue R, Sato Y, Motoyama M, Nakagawa S, Shinohara R, Nomiyama K. Plant uptake of pharmaceutical chemicals detected in recycled organic manure and reclaimed wastewater. J Agric Food Chem. (2012) 60:10203–11. doi: 10.1021/jf303142t
54. Kumar K, Gupta SC. A framework to predict uptake of trace organic compounds by plants. J Environ Qual. (2016) 45:555–64. doi: 10.2134/jeq2015.06.0261
55. Montemurro N, Postigo C, Lonigro A, Perez S, Barceló D. Development and validation of an analytical method based on liquid chromatography–tandem mass spectrometry detection for the simultaneous determination of 13 relevant wastewater-derived contaminants in lettuce. Anal Bioanal Chem. (2017) 409:5375–87. doi: 10.1007/s00216-017-0363-1
56. Knight ER, Carter LJ, McLaughlin MJ. Bioaccumulation, uptake, and toxicity of carbamazepine in soil–plant systems. Environ Toxicol Chem. (2018) 37:1122–30. doi: 10.1002/etc.4053
57. García-Valcárcel AI, Loureiro I, Escorial C, Molero E, Tadeo JL. Uptake of azoles by lamb’s lettuce (Valerianella locusta L.) grown in hydroponic conditions. Ecotoxicol Environ Saf. (2016) 124:138–46. doi: 10.1016/j.ecoenv.2015.10.021
58. Herklotz PA, Gurung P, Vanden Heuvel B, Kinney CA. Uptake of human pharmaceuticals by plants grown under hydroponic conditions. Chemosphere. (2010) 78:1416–21. doi: 10.1016/j.chemosphere.2009.12.048
59. Jones ER, van Vliet MTH, Qadir M, Bierkens MFP. Country-level and gridded estimates of wastewater production, collection, treatment and reuse. Earth Syst Sci Data. (2021). 13:237–54. doi: 10.5194/essd-13-237-2021
60. Pullagurala VLR, Rawat S, Adisa IO, Hernandez-Viezcas JA, Peralta-Videa JR, Gardea-Torresdey JL. Plant uptake and translocation of contaminants of emerging concern in soil. Sci Total Environ. (2018) 636:1585–96. doi: 10.1016/j.scitotenv.2018.04.375
61. Shi Q, Xiong Y, Kaur P, Sy ND, Gan J. Contaminants of emerging concerns in recycled water: Fate and risks in agroecosystems. Sci Total Environ. (2022) 814:152527. doi: 10.1016/j.scitotenv.2021.152527
62. Ponce-Robles L, Benelhadj L, García-García AJ, Pedrero-Salcedo F, Nortes-Tortosa PA, Albacete J, et al. Risk assessment for uptake and accumulation of pharmaceuticals by baby leaf lettuce irrigated with reclaimed water under commercial agricultural activities. J Environ Manage. (2022) 324:116321. doi: 10.1016/j.jenvman.2022.116321
63. Rout PR, Zhang TC, Bhunia P, Surampalli RY. Treatment technologies for emerging contaminants in wastewater treatment plants: A review. Sci Total Environ. (2021) 753:141990. doi: 10.1016/j.scitotenv.2020.141990
64. Rakovec O, Samaniego L, Hari V, Markonis Y, Moravec V, Thober S, et al. The 2018–2020 multi-year drought sets a new benchmark in Europe. Earths Future. (2022) 10. doi: 10.1029/2021EF002394
65. Hochstrat R, Wintgens T, Melin T, Jeffrey P. Assessing the European wastewater reclamation and reuse potential — a scenario analysis. Desalination. (2006) 188:1–8. doi: 10.1016/j.desal.2005.04.096
66. Beard JE, Bierkens MFP, Bartholomeus RP. Following the Water: Characterising de facto Wastewater Reuse in Agriculture in the Netherlands. Sustainability. (2019) 11:5936. doi: 10.3390/su11215936
67. Verlicchi P, Lacasa E, Grillini V. Quantitative and qualitative approaches for CEC prioritization when reusing reclaimed water for irrigation needs – A critical review. Sci Total Environ. (2023) 900:165735. doi: 10.1016/j.scitotenv.2023.165735
68. Khan AHA, Barros R. Pharmaceuticals in water: risks to aquatic life and remediation strategies. Hydrobiology. (2023) 2:395–409. doi: 10.3390/hydrobiology2020026
Keywords: cereals, emerging contaminants, wastewater reuse, plant uptake, soil contamination, active compounds of pharmaceuticals, bioconcentration and translocation factors
Citation: Denora M, Mehmeti A, Candido V, Brunetti G, De Mastro F, Murgolo S, De Ceglie C, Gatta G, Giuliani MM, Fiorentino C and Perniola M (2024) Fate of emerging contaminants in the soil-plant system: a study on durum wheat irrigated with treated municipal wastewater. Front. Soil Sci. 4:1448016. doi: 10.3389/fsoil.2024.1448016
Received: 12 June 2024; Accepted: 23 July 2024;
Published: 12 August 2024.
Edited by:
Changyin Zhu, Nanjing Forestry University, ChinaReviewed by:
Tamer A. Elbana, National Research Centre, EgyptShixian Sun, Southwest Forestry University, China
Copyright © 2024 Denora, Mehmeti, Candido, Brunetti, De Mastro, Murgolo, De Ceglie, Gatta, Giuliani, Fiorentino and Perniola. This is an open-access article distributed under the terms of the Creative Commons Attribution License (CC BY). The use, distribution or reproduction in other forums is permitted, provided the original author(s) and the copyright owner(s) are credited and that the original publication in this journal is cited, in accordance with accepted academic practice. No use, distribution or reproduction is permitted which does not comply with these terms.
*Correspondence: Michele Perniola, bWljaGVsZS5wZXJuaW9sYUB1bmliYXMuaXQ=