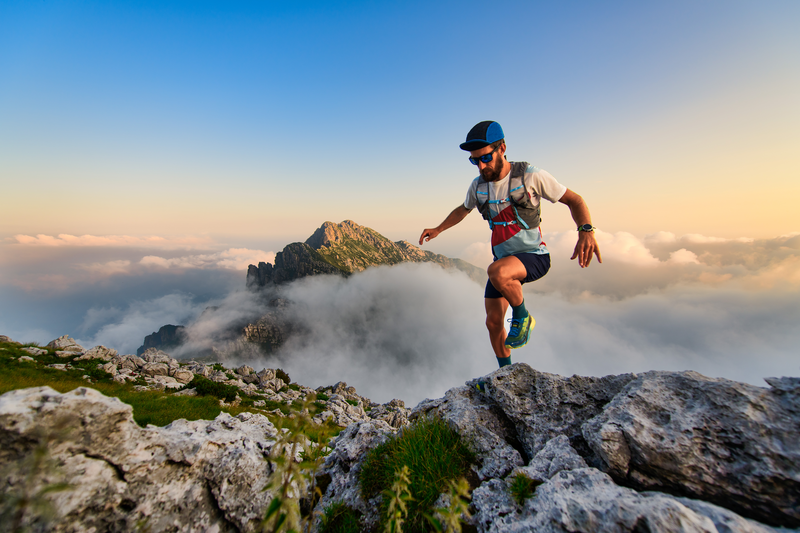
95% of researchers rate our articles as excellent or good
Learn more about the work of our research integrity team to safeguard the quality of each article we publish.
Find out more
REVIEW article
Front. Soil Sci. , 12 April 2024
Sec. Soil Management
Volume 4 - 2024 | https://doi.org/10.3389/fsoil.2024.1345895
Sandy soils are characterized by low soil moisture content and nutrient retention due to high permeability, limiting crop productivity and threatening food security in arid and semi-arid regions worldwide. Various reclamation technologies have been developed to address these challenges, but their effectiveness has not been comprehensively evaluated. This systematic review evaluated the performance of 42 sandy soil reclamation technologies reported in 144 studies from 27 countries that met specified selection criteria. Performance was evaluated based on response ratio (RR) of aboveground biomass and grain yield, as indicators of productivity, and soil moisture content and soil organic carbon (SOC), as indicators of soil health. The 42 technologies employed four main soil amendments: biochar, organic amendments, organic amendments combined with biochar, and soft rock. Overall, all technologies increased productivity and improved soil health. Biochar application was found to be the most effective technology, increasing grain yield by 51.6%, aboveground biomass by 67.4%, soil moisture content by 17.3%, and SOC by 74.2%. Soft rock application increased grain yield by 20.3%, aboveground biomass by 27.6%, soil moisture content by 54.5%, and SOC by 12.8%. Organic amendments increased grain yield by 48.7%, aboveground biomass by 45.6%, soil moisture content by 20.8%, and SOC by 36.7%. However, the combination of biochar and organic amendments showed lower improvements, with increases of 25.4%, 15.6%, 1.3%, and 25.4% for grain yield, aboveground biomass, soil moisture content, and SOC, respectively. Our conclusion is that the findings provide strong evidence that sandy soil reclamation technologies can significantly improve crop productivity and food security. Considering the variability in technologies responses across continents, there is need for further research to determine the optimal technology for specific locations, crops, and management practices.
The global population is predicted to grow from 7.8 billion in 2020 to 9 billion by 2050 (1), necessitating comprehensive measures to address food security and nutrition. These measures must encompass actions across the entire food value chain, including production (2). Sustainable agricultural intensification at production level, characterized by diversification, efficient production methods, and climate change mitigation strategies, has been suggested as a response to food insecurity (3). However, millions of hectares of global land mass are unsuitable for conventional agriculture due to low productivity and high water and nutrient permeability, necessitating technological intervention. Sandy soils, covering approximately 900 million hectares worldwide (4), are among these unsuitable lands. The high permeability of sandy soils to water and associated nutrients limits crop productivity (5) and increases groundwater contamination when fertilizers are applied (6). In arid and semi-arid regions, where sandy soils are prevalent, the predicted climate change and variability are expected to exacerbate food security and nutrition challenges for farmers who rely on these lands (7). Reclaiming sandy soils through technologies that mitigate water and nutrient percolation is essential for enhancing crop production and ensuring food security.
To enhance the productivity of sandy soils, various technologies for improving soil moisture and nutrient retention have been developed. These approaches involve using soil water retention technologies which optimize rainwater utilization and minimize irrigation needs around the plant roots (8, 9). For example, biochar application is reported to influence soil hydro-physical properties by improving the ability of sandy soil to conserve water in arid or semi-arid conditions (10, 11), use of asphalt interrupts water movement by forming a continuous barrier under sandy soil (12), and soil management practices, including mulching and cover cropping, assist in moisture retention (13, 14). Organic amendments such as use of compost, food waste, crop residues and application of manure have also been reported to enhance sandy soil`s productivity by increasing soil organic matter content, enhancing soil microbial diversity and improving soil moisture and nutrient retention capacity (15–17). Previous studies have often evaluated these technologies in isolation, making it difficult to determine the most effective approach. Moreover, the results are often context-specific and frequently influenced by the experimental design and the conditions under which the experiments were conducted. Studies evaluating biochar application, such as those by (18–20), have investigated different application rates. Similarly, studies assessing water retention membranes, such as those by (21–23), have examined their effectiveness under different climate conditions. However, variations in experimental design and conditions between these studies hinder identification of the most suitable technology for improving sandy soil productivity in a specific region and determination of the optimal conditions to maximize its effectiveness.
Achieving United Nations Sustainable Development Goals (SDG) on ending hunger and achieving food and nutrition security necessitates transformation of agricultural and food systems to encompass more sustainable and climate-resilient production methods that also preserve biodiversity (24). This can be partly accomplished through identification, implementation, and scaling of technologies with proven effectiveness or widespread recognition in enhancing sandy soil productivity. However, there remains a substantial knowledge gap regarding the overall effect of sandy soil reclamation technologies on grain yield, aboveground biomass, soil moisture retention, and SOC. A thorough systematic review is needed to identify, evaluate, and synthesize the findings of various studies on sandy soil reclamation technologies and draw comprehensive conclusions.
The overarching objective of this study was thus to evaluate the effectiveness of sandy soil reclamation technologies in enhancing crop yield, aboveground biomass, soil moisture retention, and SOC levels. We hypothesized that sandy soil reclamation technologies would improve crop productivity by enhancing soil health through increased soil moisture retention and improved soil organic carbon. Therefore, systematic review was conducted, addressing the following crucial questions: (1) Are there temporal and spatial trends in terms of sandy soil reclamation technologies? (2) What is the overall effect of sandy soil reclamation technologies on crop performance? and (3) Does the effect depend on the type of crop, climate, and farm management practices?
An extensive literature search was conducted using prominent academic databases (Web of Science, SCOPUS, ProQuest) to identify published studies providing quantitative or qualitative data on the effectiveness of sandy soil reclamation technologies employed globally. The review covered studies published between 1969 and December 2022. An initial scoping search was conducted to identify sandy soil reclamation technologies that have gained widespread recognition and promotion, and the key indicators of impact discussed in the identified literature. Crop productivity and soil health emerged as the two primary indicators of impact, where soil health serves as the indicator of sustainability in the environmental domain (25). A comprehensive search string was developed incorporating the term ‘sandy soil’ or its synonyms, various sandy soil reclamation technologies, the performance metrics of the measured outcome, and the scope of the technology application (Table 1).
Table 1 Comprehensive search terms employed to identify relevant publications in Web of Science, SCOPUS, and ProQuest.
Preliminary screening of potential papers was conducted based on evaluation of their titles and abstracts. Relevant papers were then subjected to a thorough review based on the following criteria: (1) Publications in peer-reviewed scientific journals, (2) original field studies conducted on farms or research stations, excluding laboratory experiments, modeling studies, and literature reviews, and (3) publications reporting appropriate quantitative data on means, sample size, and measures of variance (e.g., standard deviation, standard error of the mean) for both the intervention and control groups. Additional criteria were (4) publications in the English language and (5) papers published 1969-2022 evaluating the effectiveness of sandy soil reclamation technologies in enhancing crop productivity and soil health. Grey literature, including books, reports, and doctoral theses, was excluded due to concerns about authenticity, potential publication bias, and accessibility challenges. Studies on sandy soil reclamation for non-agricultural purposes, such as construction, were also excluded, to maintain a strict focus on agricultural applications. The inclusion-exclusion criteria were mutually agreed by all co-authors through a consensus process.
Publications screening adhered to the Preferred Reporting Items for Systematic Reviews and Meta-Analysis (PRISMA) statement (26). Figure 1 presents a PRISMA flow chart of the review process and the number of publications identified, screened, and included at each of the four phases of this study.
Figure 1 PRISMA flow chart illustrating the systematic steps employed in selection of relevant publications for this systematic review of sandy soil reclamation technologies. Number (N) of publications retained is shown in brackets.
A comprehensive literature review was conducted. Each selected publication was reviewed in detail to gather data on the effectiveness of sandy soil reclamation technologies in improving crop productivity and soil health. The data extracted were divided into the following categories: (1) bibliographic information: author, year of publication, title, and abstract; (2) locational information: continent, country, experimental site/s, global positioning systems (GPS) coordinates (both longitude and latitude) of experimental sites, and elevation; (3) farm management practices: technology used, application rates, fertilizer type and rates, irrigation type, and crop type; and (4) measures of outcome for crop productivity and soil health, units of measurement. Quantitative information, such as mean and standard deviation of measured parameters, was extracted when reported. For studies without quantitative data, the overall effect (neutral, positive, negative) or percentage change resulting from use of a given technology was recorded. To prevent inflated sample sizes and significance levels that could lead to type I errors, each outcome from a single study was considered as a separate data point, in accordance with the recommendation by (27). This approach ensured that each outcome was treated as an independent observation, avoiding overestimation of sample size and significance levels, as recommended by (28). For instance, if a single study reported outcomes for different crop species, seasons, locations, technology levels, or fertilizer application rates, each outcome was treated as an independent observation.
A total of 42 sandy soil reclamation technologies were identified and evaluated from the 144 publications reviewed (Appendix A). Technologies identified were categorized into broader classes of sandy soil reclamation technologies (Table 2), based on similarities or synonyms. Performance indicators were also identified and categorized (Table 3). The diverse range of sandy soil reclamation technologies resulted in identification of 470 metrics for assessing crop productivity and soil health, with 73% of these reported in only one study. To assess the effectiveness of the technologies, four key indicators were selected: grain yield and aboveground biomass as measures of crop productivity (38 and 18 studies, respectively), and soil moisture content and SOC as indicators of soil health (18 and 16 studies, respectively). Enhancing crop productivity (grain yield and aboveground biomass) while simultaneously improving soil health (SOC and soil moisture) is recognized as a hallmark of sustainable intensification (74). Low SOC levels, which negatively impact soil moisture retention, impose significant constraints on agricultural productivity worldwide. Grain yield directly represents the quantity of edible and marketable crop products, while aboveground biomass serves as a measure of crop growth. Grain yield and aboveground biomass are frequently used to compare the performance of different cropping systems, farm management practices, and crop varieties. Therefore, they are considered standard metrics for evaluating the effectiveness of sandy soil reclamation technologies in enhancing crop productivity. Soil moisture retention and SOC were analyzed as direct indicators of the ability of sandy soil reclamation technologies to improve soil water-holding capacity and carbon sequestration potential.
Table 2 Categories of sandy soil reclamation technologies identified in the 144 studies reviewed and respective references.
The spatial distribution of the reviewed publications was mapped in ArcGIS 10.3.1, utilizing the GPS coordinates of experimental sites from each study. Temporal characteristics were illustrated by charting the annual distribution of studies. For studies lacking GPS coordinates, site locations were extracted from Google Earth Pro (75), based on the study’s specified locations.
To enable meaningful comparisons of the effectiveness of the technologies across different regions and crop types, response ratio (RR) was employed as the effect size measure. It was selected because it allows comparison of outcomes across studies that use different measurement procedures (76) and was calculated as:
where ln is the natural logarithm, is the mean of the experimental group, and is the mean of the control group (76).
A negative RR value signifies that the test crop exhibits better performance without implementation of the technology. Percentage change was calculated to quantify the magnitude of change associated with each technology using Equation 2:
Owing to great heterogeneity in management practices and socio-economic conditions across the study sites, we categorized studies into subgroups with comparable conditions to elucidate the influence of moderating factors (such as fertilizer use, application rates, and continental variation) on the effectiveness of the technologies. Biochar was selected as a representative technology due to its prevalence across the reviewed studies and its diverse application in various regions. Response ratio, calculated using Equation 1, was employed to compare the impact of various moderating factors on performance indicators such as grain yield, aboveground biomass, soil moisture, and SOC.
Analysis of 144 publications spanning six continents (Africa, Asia, Europe, Oceania, North America, and South America) revealed that majority of studies were conducted in Africa (57), while the fewest were conducted in South America (5) (Figure 2). Overall, there was a consistent upward trend in the number of publications on sandy soil reclamation throughout the study period (Figure 3). Organic amendments were the primary technologies investigated for their potential to enhance productivity of sandy soils until 2007. Use of soft rock, biochar, and combined biochar and organic amendments emerged from 2007 onwards, with a gradual increase in the number of studies up to 2022.
Figure 2 Geographical distribution of sandy soil reclamation studies worldwide. The number of studies conducted in each continent is indicated within the legend.
Biochar application was reported to give the most substantial increase in grain yield, while soft rock application gave the least improvement (Figure 4). The next most effective technology for enhancing grain yield was application of organic amendments, with mean RR of 0.30 (95% confidence interval (CI) = 0.24, 0.37). Combining organic amendments and biochar resulted in RR of 0.22 (95% CI = 0.18, 0.26), indicating a lesser effect than when these technologies were applied individually. All four technologies gave an increase in aboveground biomass, but with varying effect size (Figure 4). Biochar application gave the most significant increase in aboveground biomass, with mean RR of 0.35 (95% CI = 0.25, 0.45), followed by organic amendment application, with mean RR of 0.32 (95% CI = 0.21, 0.44). Combined application of organic amendments and biochar gave the least substantial increase in aboveground biomass, with mean RR of 0.14 (95% CI = 0.12, 0.17), indicating a weaker effect than when these materials were applied individually. Soft rock application resulted in a moderate increase in aboveground biomass, with mean RR of 0.24 (95% CI = 0.13, 0.35).
Figure 4 Mean effect of the different sandy soil reclamation technologies on grain yield, aboveground biomass, soil moisture retention, and soil organic carbon. Number of publications (N) and number of independent observations (NO) are also shown.
Application of biochar, soft rock, organic amendments, and combined biochar and organic amendments to sandy soils led to increased soil moisture retention compared with the control group (Figure 4). Soft rock application gave the most significant enhancement in soil moisture retention, with mean RR of 0.40 (95% CI = 0.20, 0.60), followed by application of organic amendments, with mean RR of 0.15 (95% CI = 0.05, 0.24), and application of biochar, also with mean RR of 0.15 (95% CI = 0.13, 0.16), respectively. Combined application of organic amendments and biochar gave a non-significant increase in soil moisture content, with mean RR of 0.01 (95% CI = -0.03, 0.04).
All four technologies contributed to an increase in SOC content (Figure 4). However, biochar application gave the most substantial increase in SOC, with mean RR of 0.38 (95% CI = 0.31, 0.45), while soft rock application gave the least improvement, with mean RR of 0.10 (95% CI = -0.18, 0.38). Organic amendment application resulted in the second-highest increase in SOC, with mean RR of 0.26 (95% CI = 0.20, 0.32). Combined application of biochar and organic amendments also led to an increase in SOC, with mean RR of 0.24 (95% CI = 0.19, 0.28). However, this effect was less pronounced than when these materials were applied individually.
An extensive review of the selected literature revealed various factors that influence the effectiveness of sandy soil reclamation technologies, including application rate, fertilizer application, regional variations, application form, and biochar type, source, and processing methods. To illustrate the impact of moderating factors on biochar performance, we examined crop type, application rate, and regional distribution.
There was a clear impact of crop type and biochar application rate on grain yield and aboveground biomass. Biochar application enhanced grain yield and aboveground biomass of maize (Zea mays), millet (Pennisetum glaucum), chickpea (Cicer arietinum L.), and faba bean (Vicia faba L.) (Figure 5). Among the studied crops, millet exhibited the highest grain yield upon biochar application, with mean RR of 0.37 (95% CI = 0.32, 0.42), followed by maize, with mean RR of 0.32 (95% CI = 0.21, 0.43). Chickpeas and faba bean grain yield also increased upon biochar application, with mean RR of 0.20 (95% CI = -0.06, 0.47) and 0.14 (95% CI = 0.04, 0.25), respectively. The positive impact of biochar application was also evident in aboveground biomass. Maize exhibited the highest aboveground biomass increase, with mean RR of 0.43 (95% CI = 0.18, 0.67), followed by millet, with mean RR of 0.39 (95% CI = 0.26, 0.51). Chickpea and faba bean aboveground biomass also showed positive responses to biochar application, with mean RR of 0.22 (95% CI = 0.15, 0.31) and 0.19 (95% CI = 0.05, 0.32), respectively.
Figure 5 Mean effect of sandy soil reclamation technologies on grain yield and aboveground biomass for different crop types. Number of publications (N) and number of independent observations (NO) are also shown.
Biochar also increased soil moisture and SOC content, but with effect size varying with crop type (Figure 6). Cocoyam showed the highest increase in SOC, with mean RR of 0.89 (95% CI = 0.77, 1.00). Soil organic carbon increases were also observed for maize and peanut, with mean RR of 0.21 (95%CI = 0.12, 0.29) and 0.10 (95% CI = -0.05, 0.25), respectively. In addition, biochar application increased soil moisture content, with the highest effect recorded in cocoyam (RR = 0.37; 95% CI = 0.31, 0.43). Mean RR for soil moisture content was 0.14 (95% CI = 0.13, 0.15) and 0.004 (95% CI = -0.04, 0.05) for peanut and maize, respectively.
Figure 6 Mean effect of sandy soil reclamation technologies on soil moisture content and soil organic carbon for different crops. Number of publications (N) and number of independent observations (NO) are also shown.
The impact of biochar application rate on grain yield, soil moisture, and SOC varied significantly (Figure 7). At application rates below 5 tons ha-1, biochar application resulted in the greatest increase in grain yield, with mean RR of 0.37 (95% CI = 0.23, 0.52). Similar increases in grain yield were observed at application rates between 5 and 15 tons ha-1 (RR = 0.24, 95% CI = 0.16, 0.32), at 16 and 26 tons ha-1 (RR = 0.03, 95% CI = -0.05, 0.11), and above 38tons ha-1 (RR = 0.09, 95% CI = 0.07, 0.11). However, a slight decrease in grain yield was recorded at application rates between 27 and 37 tons ha-1 (RR = -0.04, 95% CI = -0.13, 0.06). Soil moisture content also increased with biochar application, but the magnitude of the increase varied depending on the application rate. At application rates<5 tons ha-1, 5-15 tons ha-1, 16-26 tons ha-1, 27-37 tons ha-1, and above 38 tons ha-1, soil moisture retention increased, with mean RR of 0.15 (95% CI = 0.02, 0.27), 0.11 (95% CI = 0.08, 0.13), 0.19 (95% CI = 0.15, 0.22), 0.18 (95% CI = 0.14, 0.22), and 0.19 (95% CI = -0.12, 0.50), respectively. Soil organic carbon also increased with biochar application, with varying effect sizes at different application rates. At application rates<5 tons ha-1, 16-26 tons ha-1, 27-37 tons ha-1, and above 38 tons ha-1, SOC increases were observed, with mean RR of 0.33 (95% CI = 0.17, 0.50), 0.29 (95% CI = 0.18, 0.39), 0.62 (95% CI = 0.38, 0.86), 0.62 (95% CI = 0.20, 1.04), and 0.55 (95% CI = 0.35, 0.74), respectively.
Figure 7 Mean effect of sandy soil reclamation technologies on grain yield, soil moisture, and soil organic carbon in sandy soils across a range of biochar application rates (t/ha). Number of publications (N) and number of independent observations (NO) are also shown.
Application of biochar to sandy soil resulted in a significant increase in crop performance, as evidenced by enhanced aboveground biomass except in Europe (Table 4) and grain yield (Table 5). On average, biochar improved crop aboveground biomass with an effect size of 0.44 (95% CI = 0.13, 0.76) in Africa and 0.23 (95% CI = 0.10, 0.35) in Asia. However, in Europe, a slight decrease in aboveground biomass was observed, with mean RR of -0.01 (-0.05, 0.03). Within Africa, the most substantial increase in aboveground biomass was seen in studies conducted in Egypt (RR 0.56, 95% CI = -0.09, 1.22), followed by Tanzania (RR 0.54, 95% CI = 0.33, 0.75) and South Africa (RR 0.23, 95% CI = 0.15, 0.31). Similarly, biochar had a positive impact on aboveground biomass in all Asian countries. Among these, China exhibited the most notable effect, with mean RR of 0.38 (95% CI = 0.26, 0.51), while, Iran, India, and Indonesia displayed mean RR values of 0.23 (95% CI = 0.06, 0.40), 0.17 (95% CI = 0.03, 0.21), and 0.14 (95% CI = 0.03, 0.26), respectively. In Europe, a marginal decrease was observed in Poland, with mean RR of -0.01(95% CI = -0.05, 0.03).
Table 5 Estimated impact of biochar, quantified by mean response ratio (RR) and corresponding 95% confidence interval (CI), on crop grain yield on sandy soils in different continents and countries.
Application of biochar also led to a significant increase in grain yield, with African countries demonstrating the highest gains (mean RR 0.34, 95% CI = 0.09, 0.59), while Asian countries exhibited mean RR of 0.28 (95% CI = 0.21, 0.35) (Table 5). European countries again experienced a decline in grain yield following biochar application, with mean RR of -0.02 (95% CI = -0.08, 0.05). African countries, including Egypt, South Africa, and Zambia, displayed enhanced grain yield, with mean RR value of 0.44 (95% CI = 0.34, 0.55), 0.20 (95% CI = -0.06, 0.47), and 0.85 (95% CI = 0.50, 1.21), respectively, while Nigeria experienced a decline, with mean RR of -0.13 (95% CI = -0.40, 0.15). Asian countries, such as Indonesia, Iran, and China, reported increased grain yield following biochar application, with mean RR values of 0.36 (95% CI = 0.31, 0.40), 0.19 (95% CI = 0.10, 0.28), and 0.29 (95% CI = 0.21, 0.38), respectively. In Europe, Finland experienced an upward trend in grain yield, with mean RR of 0.08 (95% CI = 0.02, 0.15) while Germany witnessed a downward trend (RR -0.12, 95% CI = -0.19, -0.04).
In addition to boosting crop yields, biochar application rates influenced soil moisture retention and SOC content in sandy soils worldwide. Soil moisture content increased, with North America exhibiting the highest increase (mean RR 0.52, 95% CI = 0.42, 0.62), while Africa, Asia, Oceania and Europe showed mean RR values of 0.25 (95% CI = 0.22, 0.29), 0.18 (95% CI = 0.02, 0.34), 0.15 (95% CI = -0.05, 0.37), and 0.07 (95% CI = -0.04, 0.18), respectively (Table 6). Egypt and Nigeria exhibited mean RR values of 0.14 (95% CI = 0.13, 0.15) and 0.37 (95% CI = 0.31, 0.43), respectively. Bangladesh, Indonesia, and China also showed increased soil moisture retention, with mean RR values of 0.11 (95% CI = -0.02, 0.25), 0.005 (95% CI = -0.04, 0.05), and 0.43 (95% CI = 0.13, 0.72), respectively. Among European countries, Finland exhibited a decrease in moisture retention, with mean RR of -0.004 (95% CI = -0.04, 0.04), while Poland showed an increase, with mean RR of 0.15 (95% CI = -0.03, 0.32). The USA and Australia exhibited increased soil moisture retention, with mean RR values of 0.52 (95% CI = 0.42, 0.62) and 0.15 (95% CI = -0.05, 0.37), respectively.
Table 6 Estimated effect, based on mean response ratio (RR) and corresponding 95% confidence interval (CI), of biochar application on soil moisture content in sandy soils in different continents and countries.
Biochar application led to an increase in SOC worldwide, with Africa exhibiting the highest increase (mean RR 1.03, 95% CI = 0.70, 1.36). Asia, Europe, and Oceania showed mean RR values of 0.27 (95% CI = 0.19, 0.32), 0.78 (95% CI = 0.62, 0.95), and 0.40 (95% CI = 0.23, 0.57), respectively (Table 7). Zambia exhibited the highest increase among African countries, with mean RR of 1.97 (95% CI = 1.49, 2.44), while Ghana and Nigeria showed mean RR values of 0.57 (95% CI = 0.39, 0.72) and 0.56 (95% CI = 0.21, 0.93), respectively. Malaysia exhibited the highest increase in SOC among Asian countries, with mean RR of 0.55 (95% CI = 0.48, 0.62). Indonesia, India, and China also showed increases, with mean RR of 0.20 (95% CI = 0.17, 0.23), 0.21 (95% CI = 0.03, 0.25), and 0.12 (95% CI = 0.07, 0.17), respectively. In Europe, Finland exhibited mean RR of 2.31 (95% CI = 2.08, 2.53), while Germany, Poland, and Slovakia showed mean RR values of 0.44 (95% CI = 0.25, 0.63), 0.10 (95% CI = -0.06, 0.27), and 0.28 (95% CI = 0.21, 0.36), respectively. Oceania (Australia), similarly to other continents, showed increased SOC, with mean RR of 0.40 (95% CI = 0.23, 0.57).
Table 7 Estimated effect, based on response ratio (RR) and corresponding 95% confidence interval (CI), of biochar application on soil organic carbon content in sandy soils in different continents and countries.
The higher number of publications on sandy soil reclamation in Asia and Africa compared with Europe, North America, Oceania, and South America can be attributed to the high population in Asia and Africa, which host 60% and 17% of the world`s population, respectively (98). This, coupled with rapid population growth rates in both continents, has put pressure on fertile land resources, leading to the expansion of agriculture into drylands dominated by sandy soils resulting in more efforts to reclaim sandy soils as evident by the high number of publications in the two continents. Furthermore, Africa and Asia have the most extensive sandy soil coverage globally, with 51% of Africa’s total land mass being sandy soils (99). The observed upward trend in publications on sandy soil reclamation technologies during the study period can be attributed to the global population increase and to decreasing availability of fertile land due to urbanization and settlement, necessitating expansion of crop production into sandy soils to meet the food and nutritional demands of the growing population. Prior to technological advances, organic amendments were among the earliest and most widely used methods for reclaiming sandy soils (100). This can be attributed to the abundance of readily available organic materials, such as crop residues and mulching organic materials, and the relatively low cost associated with their use compared with more expensive and time-consuming advanced technologies. Lack of organic residues due to low productivity or priority being given to the use of crop residues as livestock feed or fuel limit the application of organic amendments to soil.
All four sandy soil reclamation technologies included in this systematic review were found to increase crop productivity and improve soil health. The magnitude of these improvements was shown to vary depending on several factors, including application rate, type of amendment used, crop type, fertilizer application, irrigation practices, host continent, irrigation type, and cropping system. Biochar application had a more significant positive impact on grain yield and aboveground biomass compared with application of soft rock, organic amendments, or a combination of organic amendments and biochar (101, 102). The observed yield and biomass improvements associated with biochar application in the selected dataset are consistent with previous findings (103). Four key mechanisms contribute to the positive effect of biochar on soil and plant productivity (104). First, the porous structure of biochar enhances nutrient retention capacity, ensuring a steady supply of nutrients to plants (105). The spongy structure of biochar also increases water retention capacity of sandy soils ensuring a continuous supply for plant use (106). Second, biochar enhances CEC, enabling sandy soil to retain more positively charged ions, including essential nutrients. It also buffers soil pH, promoting nutrient availability (107). Third, biochar provides a habitat and carbon source for beneficial microbes involved in nutrient cycling, leading to enhanced microbial activity (108). Fourth, biochar can immobilize toxic substances such as heavy metals, reducing their uptake by crops and minimizing potential environmental harm (109). Additionally, our comprehensive analysis revealed that biochar application effectively enhances soil moisture retention. This can be attributed to its porous structure acting as sponge, absorbing water during rainfall or irrigation and gradually releasing it to plants during dry periods (110). Biochar also promotes SOC accumulation, directly by adding over 65% of the carbon in biomass to the soil (111) and indirectly by stimulating root exudation and increasing microbial activity (112). Biochar enhances soil aggregation, which protects organic matter from decomposition and promotes SOC accumulation over time (113).
Application of organic amendments, such as compost and other organic materials, has been shown to have positive effects on soil properties and crop productivity. These beneficial effects can be attributed to the porous structure of the organic matter added to the soil, which enhances water retention and nutrient availability. Formation of micro-aggregates, i.e., small clumps of soil particles that create pore spaces, further contributes to water retention. Improved soil structure, reduced soil compaction, and increased pore spaces, promoted by organic amendments, facilitates root penetration and moisture storage. Furthermore, organic amendments provide labile carbon sources that stimulate microbial growth and activity, leading to enhanced nutrient cycling and plant growth. Organic amendments have been demonstrated to have positive effects on maize grain yield when applied independently (114) or in combination with biochar (115).
Application of soft rock to sandy soil can increase soil moisture retention and SOC through various mechanisms, including buffering of soil pH. For instance, soft rock types such as gypsum can raise soil pH, thereby increasing the availability of essential nutrients for plant uptake, enhancing soil CEC, which promotes nutrient retention and availability to plants by increasing the soil’s ability to hold positively charged ions like potassium and calcium; enhancing soil moisture retention by improving soil structure, particularly by increasing water-holding capacity and reducing water loss through evaporation; and reducing the availability of toxic elements like aluminum by binding them to its mineral components, thereby making them less harmful to plants and promoting increased biomass and crop health. For instance, application of soft rock has been shown to increase millet grain yield by approximately 20%, millet aboveground biomass by over 25%, and soil moisture content by 2%, as demonstrated by (80).
The reported effect of sandy soil reclamation technologies on crop productivity and soil health varied depending on the type of the technology, crop type, management practices, and factors in the region where the technology was applied. Similar conclusions have been reached in meta-analyses evaluating the effects of other innovative technologies on crop productivity and soil health (116, 117). In the studies in our review, biochar demonstrated superior performance for cereals like millet and maize compared with legumes (e.g., chickpea and faba bean), as concluded previously by (105). These differences can be explained by variations in crop root systems and differences in nutrient uptake capabilities, and by differences in nutrient requirements between cereals and legumes (55). Additionally, reported crop performance exhibited a declining trend as biochar application increased, as found in previous studies (55, 105, 118). Our dataset indicated that at low to moderate application rates, biochar improves soil structure and enhances nutrient and moisture retention, thus increasing nutrient availability to plants. However higher or excess application of biochar may affect soil structure, impairing water drainage and potentially leading to waterlogging. Higher biochar application rates can also result in immobilization of nutrients reducing availability to plants. Furthermore, the effectiveness of biochar as a soil improver varies across different socio-economic contexts. Comparison of biochar application across continents revealed that biochar use resulted in the greatest increase in grain yield, aboveground biomass, soil moisture content, and SOC in Africa, supporting the conclusion by (119) that biochar boosts tropical, but not temperate, crop yields.
A few limitations in the data analysis process may have affected the study findings. First, there was great heterogeneity in the studies reviewed in terms of study design, conditions under which the studies were conducted (including variations in climate conditions and planting seasons), farm management practices (such as fertilizer application and irrigation), and reported statistical information (such as means and standard deviations). To overcome this challenge, we used RR to compare the effects of the technologies. Second, we limited the review to articles in the English language and excluded grey literature because of lack of authenticity. Finally, for comparative synthesis, use of two indicators of crop performance and two soil health characteristics might have potentially locked out other indicators that could be more sensitive to the use of a particular technology.
There is growing interest worldwide in sandy soil reclamation to improve crop productivity and soil health. Sandy soil reclamation technologies such as addition of biochar, organic amendments, soft rock, and combined organic amendments and biochar have good potential to enhance SOC content, soil moisture, and productivity of various crops. Among them, application of biochar showed the greatest potential for improving soil health and increasing crop productivity. High aboveground biomass is critical, since when returned to the soil it enhances SOC, which is commonly low in sandy soils. However, large geographical differences in terms of technology performance can arise, indicating that management practices need to be in line with local and regional specifics. Additionally, technology performance varies with application rates, field management practices and crop type. Therefore, future studies could review the effect specific sandy soil reclamation technologies on particular indicators in regions with similar ecological conditions for better generalization of the findings.
SM: Formal analysis, Methodology, Writing – original draft. SK: Writing – review & editing. SN: Writing – review & editing. SKN: Writing – review & editing. WK: Writing – review & editing. AS: Writing – review & editing. LN: Writing – review & editing.
The author(s) declare financial support was received for the research, authorship, and/or publication of this article. The study was conducted with co-funding from the Swedish University of Agricultural Sciences (SLU) for the project “Solution for increasing farm system resilience and carbon sinks on sandy soils, NCF 8 Project No NCF-C8-0507” funded by the Nordic Climate Facility (NCF), initiative of the Nordic Development Fund (NDF).
The authors appreciate the Swedish University of Agricultural Sciences (SLU) for the Ph. D scholarship awarded to SM.
The authors declare that the research was conducted in the absence of any commercial or financial relationships that could be construed as a potential conflict of interest.
All claims expressed in this article are solely those of the authors and do not necessarily represent those of their affiliated organizations, or those of the publisher, the editors and the reviewers. Any product that may be evaluated in this article, or claim that may be made by its manufacturer, is not guaranteed or endorsed by the publisher.
The Supplementary Material for this article can be found online at: https://www.frontiersin.org/articles/10.3389/fsoil.2024.1345895/full#supplementary-material
1. Brook BW, Buettel JC, Hong SH. Constrained scenarios for twenty-first century human population size based on the empirical coupling to economic growth. (2021), 1–11.
2. Lang T, Barling D. Food security and food sustainability: Reformulating the debate. Geogr J. (2012) 178:313–26. doi: 10.1111/j.1475-4959.2012.00480.x
3. Kirwan J, Maye D. Food security framings within the UK and the integration of local food systems. J Rural Stud. (2013) 29:91–100. doi: 10.1016/j.jrurstud.2012.03.002
4. Driessen PM, Deckers J, Spaargaren O. Lecture notes on the major soils of the world. Rome: Food and Agriculture Organization of the United Nations (FAO (2001). World Soil Resources Reports : FAO.
5. Pranagal J, Oleszczuk P, Tomaszewska-Krojańska D, Kraska P, Różyło K. Effect of biochar application on the physical properties of Haplic Podzol. Soil Tillage Res. (2017) 174:92–103. doi: 10.1016/j.still.2017.06.007
6. Shepherd MA, Bennett G. Nutrient leaching losses from a sandy soil in lysimeters. Commun Soil Sci Plant Anal. (1998) 29:931–46. doi: 10.1080/00103629809369997
7. Ren X, Weitzel M, O’Neill BC, Lawrence P, Meiyappan P, Levis S, et al. Avoided economic impacts of climate change on agriculture: integrating a land surface model (CLM) with a global economic model (iPETS). Clim Change. (2018) 146:517–31. doi: 10.1007/s10584-016-1791-1
8. Kavdir Y, Zhang W, Basso B, Smucker AJM. Development of a new long-term drought resilient soil water retention technology. J Soil Water Conserv. (2014) 69:154A–60A. doi: 10.2489/jswc.69.5.154A
9. Aoda MI, Smucker AJM, Majeed SS, Mohammed HA, Al-Sahaf FH, Robertson GP. Novel root zone soil water retention improves production with half the water in arid sands. Agron J. (2021) 113:2398–406. doi: 10.1002/agj2.20648
10. Alkhasha A, Al-Omran A, Aly A. Effects of biochar and synthetic polymer on the hydro-physical properties of sandy soils. Sustain. (2018) 10:1–18. doi: 10.3390/su10124642
11. Carter S, Shackley S, Sohi S, Suy T, Haefele S. The Impact of Biochar Application on Soil Properties and Plant Growth of Pot Grown Lettuce (Lactuca sativa) and Cabbage (Brassica chinensis). Agronomy. (2013) 3:404–18. doi: 10.3390/agronomy3020404
12. Gupta JP, Aggarwal RK. Use of an asphalt subsurface barrier for improving the productivity of desert sandy soils. J Arid Environ. (1980) 3:215–22. doi: 10.1016/S0140-1963(18)31649-5
13. Benigno SM, Cawthray GR, Dixon KW, Stevens JC. Soil physical strength rather than excess ethylene reduces root elongation of Eucalyptus seedlings in mechanically impeded sandy soils. Plant Growth Regul. (2012) 68:261–70. doi: 10.1007/s10725-012-9714-2
14. Zaidun SW, Jalloh MB, Awang A, Sam LM, Besar NA, Musta B, et al. Biochar and clinoptilolite zeolite on selected chemical properties of soil cultivated with maize (Zea mays L.). Eurasian J Soil Sci. (2019) 8:1–10. doi: 10.18393/ejss.468100
15. Larney FJ, Angers DA. The role of organic amendments in soil reclamation: A review. Can J Soil Sci. (2012) 92:19–38. doi: 10.4141/cjss2010-064
16. Garbowski M, Brown CS, Johnston DB. Soil amendment interacts with invasive grass and drought to uniquely influence aboveground versus belowground biomass in aridland restoration. Restor Ecol. (2020) 28:A13–23. doi: 10.1111/rec.13083
17. Mohd Dolit SA, Asli UA, Khamis AK, Baharulrazi N, Azman NR, Yunus NA. Improving the water retention characteristics of sandy soil using food waste compost amendment and indigenous microorganisms. Chem Eng Trans. (2022) 97:211–6.
18. Dong C, Chen Z, Xie Y, Zhang Y, Gou P, Yang J, et al. Effects of successive biochar addition to soil on nitrogen functional microorganisms and nitrous oxide emission. Sci Agric Sin. (2020) 53:4024–34.
19. Kalu S, Oyekoya GN, Ambus P, Tammeorg P, Simojoki A, Pihlatie M, et al. Effects of two wood-based biochars on the fate of added fertilizer nitrogen—a 15N tracing study. Biol Fertil Soils. (2021) 57:457–70. doi: 10.1007/s00374-020-01534-0
20. Oladele S, Adeyemo A, Awodun M, Ajayi A, Fasina A. Effects of biochar and nitrogen fertilizer on soil physicochemical properties, nitrogen use efficiency and upland rice (Oryza sativa) yield grown on an Alfisol in Southwestern Nigeria. Int J Recycl Org Waste Agric. (2019) 8:295–308. doi: 10.1007/s40093-019-0251-0
21. Demirel K, Kavdır Y. Effect of soil water retention barriers on turfgrass growth and soil water content. Irrig Sci. (2013) 31:689–700. doi: 10.1007/s00271-012-0345-1
22. Faisal AAH, Al-Ridah ZA, Naji LA, Naushad M, El-Serehy HA. Waste Foundry Sand as Permeable and Low Permeable Barrier for Restriction of the Propagation of Lead and Nickel Ions. Hindawi. Journal of Chemistry. (2020) 2020:1–3. Available at: https://www.proquest.com/scholarly-journals/waste-foundry-sand-as-permeable-low-barrier/docview/2431750899/se-2?accountid=14717.
23. Ityel E, Lazarovitch N, Silberbush M, Ben-gal A. An artificial capillary barrier to improve root-zone conditions for horticultural crops: response of pepper, lettuce, melon, and tomato. Irrig Sci. (2012) 30:293–301. doi: 10.1007/s00271-011-0281-5
24. Lal R. Advancing climate change mitigation in agriculture while meeting global sustainable development goals. Soil Water Conserv A Celebr 75 Years. (2020), 344.
25. Smith A, Snapp S, Chikowo R, Thorne P, Bekunda M, Glover J. Measuring sustainable intensi fi cation in smallholder agroecosystems : A review. Global Food Security. (2017) 12(July 2016):127–38. doi: 10.1016/j.gfs.2016.11.002
26. Moher D, Liberati A, Tetzlaff J, Altman DG. Academia and clinic annals of internal medicine preferred reporting items for systematic reviews and meta-analyses. Ann Intern Med. (2009) 151:264–9. doi: 10.7326/0003-4819-151-4-200908180-00135
27. Koricheva J, Gurevitch J. Uses and misuses of meta-analysis in plant ecology. J Ecol. (2014) 102:828–44. doi: 10.1111/1365-2745.12224
28. Higgins J, Green S. Chapter 22: Overview of reviews. Cochrane handbook for systematic reviews of interventions. Cochrane Database Syst Rev. (2008), 187–235. doi: 10.1002/9780470712184
29. Adekiya AO, Taiwo MA, Olayanju A, Ejue WS, Adekanye TA, Adenusi TT, et al. Effect of biochar on soil properties, soil loss, and cocoyam yield on a tropical sandy loam alfisol. Sci World J. (2020) 2020:9. doi: 10.1155/2020/9391630
30. Agbede TM, Adekiya AO, Odoja AS, Bayode LN, Omotehinse PO, Adepehin I. Effects of biochar and poultry manure on soil properties, growth, quality, and yield of cocoyam (Xanthosoma sagittifolium Schott) in degraded tropical sandy soil. Exp Agric. (2020) 56:528–43. doi: 10.1017/S0014479720000137
31. Amoakwah E, Frimpong KA, Okae-Anti D, Arthur E. Soil water retention, air flow and pore structure characteristics after corn cob biochar application to a tropical sandy loam. Geoderma. (2017) 307:189–97. doi: 10.1016/j.geoderma.2017.08.025
32. Are KS, Adelana AO, Fademi IO, Aina OA. Improving physical properties of degraded soil: Potential of poultry manure and biochar. Agric Nat Resour. (2017) 51:454–62. doi: 10.1016/j.anres.2018.03.009
33. Abd El-Mageed TA, Belal EE, Rady MOA, Abd El-Mageed SA, Mansour E, Awad MF, et al. Acidified biochar as a soil amendment to drought stressed (Vicia faba l.) plants: Influences on growth and productivity, nutrient status, and water use efficiency. Agronomy. (2021) 11:1290. doi: 10.3390/agronomy11071290
34. Agbede TM, Oyewumi A. Benefits of biochar, poultry manure and biochar–poultry manure for improvement of soil properties and sweet potato productivity in degraded tropical agricultural soils. Resour Environ Sustain. (2022) 7:100051. doi: 10.1016/j.resenv.2022.100051
35. Zhang H, Chen W, Zhao B, Phillips LA, Zhou Y, Lapen DR, et al. Sandy soils amended with bentonite induced changes in soil microbiota and fungistasis in maize fields. Appl Soil Ecol. (2020) 146:103378. doi: 10.1016/j.apsoil.2019.103378
36. Czaban J, Siebielec G. Effects of bentonite on sandy soil chemistry in a long-term plot experiment (II); effect on pH, CEC, and macro- and micronutrients. Polish J Environ Stud. (2013) 22:1669–76.
37. Czaban J, Siebielec G, Czyz E, Niedźwiecki J. Effects of bentonite addition on sandy soil chemistry in a long-term plot experiment (I); effect on organic carbon and total nitrogen. Polish J Environ Stud. (2013) 22:1661–7.
38. Suzuki S, Noble AD, Ruaysoongnern S, Chinabut N. Improvement in water-holding capacity and structural stability of a sandy soil in northeast Thailand. Arid L Res Manage. (2007) 21:37–49. doi: 10.1080/15324980601087430
39. Agegnehu G, Bass AM, Nelson PN, Muirhead B, Wright G, Bird MI. Biochar and biochar-compost as soil amendments: Effects on peanut yield, soil properties and greenhouse gas emissions in tropical North Queensland, Australia. Agric Ecosyst Environ. (2015) 213:72–85. doi: 10.1016/j.agee.2015.07.027
40. McDonald MR, Bakker C, Motior MR. Evaluation of wood biochar and compost soil amendment on cabbage yield and quality. Can J Plant Sci. (2019) 99:624–38. doi: 10.1139/cjps-2018-0122
41. Novak JM, Watts DW, Sigua GC, Ducey TF. Corn grain and stover nutrient uptake responses from sandy soil treated with designer biochars and compost. Agronomy. (2021) 11(5):942. doi: 10.3390/agronomy11050942
42. Zahra MB, Aftab Z-E-H, Akhter A, Haider MS. Cumulative effect of biochar and compost on nutritional profile of soil and maize productivity. J Plant Nutr. (2021) 44(11):1664–76. doi: 10.1080/01904167.2021.1871743
43. Lipiec J, Usowicz B. Quantifying cereal productivity on sandy soil in response to some soil-improving cropping systems. Land. (2021) 10:1–16. doi: 10.3390/land10111199
44. Sahar EAM, Elsoofy DM, Abdou MAA, Abdelraouf RE. The role of organic fertilization in raising potato productive efficiency and water productivity under the conditions of organically poor sandy soil. Egypt J Chem. (2021) 64:2273–84. doi: 10.21608/ejchem.2021.61496.3323
45. Sulok KMT, Ahmed OH, Khew CY, Zehnder JAM, Lai PS, Jalloh MB, et al. Effects of organic amendments produced from agro-wastes on sandy soil properties and black pepper morpho-physiology and yield. Agronomy. (2021) 11(9):1738. doi: 10.3390/agronomy11091738
46. Zhao W, Zhou Q, Tian Z, Cui Y, Liang Y, Wang H. Apply biochar to ameliorate soda saline-alkali land, improve soil function and increase corn nutrient availability in the Songnen Plain. Sci Total Environ. (2020) 722:137428. doi: 10.1016/j.scitotenv.2020.137428
47. Li F, Liang X, Li H, Jin Y, Jin J, He M, et al. Enhanced soil aggregate stability limits colloidal phosphorus loss potentials in agricultural systems. Environ Sci Eur. (2020) 32:1–4. doi: 10.1186/s12302-020-0299-5
48. Yongkang S, Tingning Z, Zhang Y, Ma D, Yang J. Response of water-soluble salt accumulation in weathered gneiss spoil substrate to the addition of superabsorbent polymer under a semiarid climate. J Soils Sediments. (2020) 20:190–203. doi: 10.1007/s11368-019-02391-7
49. Sun Y, Zhang N, Yan J, Zhang S. Effects of soft rock and biochar applications on millet (Setaria italicaL.) crop performance in sandy soil. Agronomy-Basel. (2020) 10:669. doi: 10.3390/agronomy10050669
50. Zhang D, Zhang Y, Cao Z, Cheng T. Optimization of roots and copper slag to reinforce soft soil using response surface method. J Renew Mater. (2020) 8:1391–409. doi: 10.32604/jrm.2020.012695
51. Al-Shareef AR, Ismail SM, El-Nakhlawy FS. Effect of humic acid application rates on physicochemical and fertility properties of sandy loam soil grown with mung bean under different irrigation water regimes. Curr Sci. (2018) 115:1374–9. doi: 10.18520/cs/v115/i7/1374-1379
52. Dawood MG, Sadak MS, Bakry BA, El Karamany MF. Comparative studies on the role of benzoic, t-cinnamic, and salicylic acids on growth, some biochemical aspects, and yield of three flax cultivars grown under sandy soil conditions. Bull Natl Res Cent. (2019) 43:1–6. doi: 10.1186/s42269-019-0152-4
53. Selim E-M, Ali Mosa A. Fertigation of humic substances improves yield and quality of broccoli and nutrient retention in a sandy soil. J Plant Nutr Soil Sci. (2012) 175:273–81. doi: 10.1002/jpln.201100062
54. Abbas A, Yaseen M, Khalid M, Naveed M, Aziz M, Hamid Y, et al. Effect of biochar-amended urea on nitrogen economy of soil for improving the growth and yield of wheat (Triticum Aestivum L.) under field condition. J Plant Nutr. (2017) 40:2303–11. doi: 10.1080/01904167.2016.1267746
55. Agegnehu G, Bass AM, Nelson PN, Bird MI. Benefits of biochar, compost and biochar-compost for soil quality, maize yield and greenhouse gas emissions in a tropical agricultural soil. Sci Total Environ [Internet]. (2016) 543:295–306. doi: 10.1016/j.scitotenv.2015.11.054
56. Azeem M, Hale L, Montgomery J, Crowley D, McGiffen ME Jr. Biochar and compost effects on soil microbial communities and nitrogen induced respiration in turfgrass soils. PloS One. (2020) 15(11):e0242209. doi: 10.1371/journal.pone.0242209
57. Knoblauch C, Priyadarshani SHR, Haefele SM, Schröder N, Pfeiffer E-M. Impact of biochar on nutrient supply, crop yield and microbial respiration on sandy soils of northern Germany. Eur J Soil Sci. (2021) 72(4):1885–901. doi: 10.1111/ejss.13088
58. Niu J, Yang K, Tang Z, Wang Y. Relationships between soil crust development and soil properties in the desert region of north China. Sustainability. (2017) 9:725. doi: 10.3390/su9050725
59. Petkova G, Nedyalkova K, Mikova A, Atanassova I. Microbiological characteristics of biochar amended alluvial meadow soil. Bulg J Agric Sci. (2018) 24:81–4.
60. Puga AP, Grutzmacher P, Cerri CEP, Ribeirinho VS, Andrade CAD. Biochar-based nitrogen fertilizers: Greenhouse gas emissions, use efficiency, and maize yield in tropical soils. Sci Total Environ. (2020) 704:135375. doi: 10.1016/j.scitotenv.2019.135375
61. Ogola JBO, Macil PJ, Odhiambo JJO. Biochar application and rhizobium inoculation increased intercepted radiation and yield of chickpea in contrasting soil types. Int J Plant Prod. (2021) 15:219–29. doi: 10.1007/s42106-021-00141-9
62. Tammeorg P, Simojoki A, Mäkelä P, Stoddard FL, Alakukku L, Helenius J. Short-term effects of biochar on soil properties and wheat yield formation with meat bone meal and inorganic fertiliser on a boreal loamy sand. Agric Ecosyst Environ. (2014) 191:108–16. doi: 10.1016/j.agee.2014.01.007
63. Abd El-Mageed TA, Abdurrahman HA, Abd El-Mageed SA. Residual acidified biochar modulates growth, physiological responses, and water relations of maize (Zea mays) under heavy metal–contaminated irrigation water. Environ Sci pollut Res. (2020) 27:22956–66. doi: 10.1007/s11356-020-08847-5
64. Ch’ng HY, Ahmed OH, Majid NMA. Improving phosphorus availability, nutrient uptake and dry matter production of zea mays l. on a tropical acid soil using poultry manure biochar and pineapple leaves compost. Exp Agric. (2016) 52:447–65. doi: 10.1017/S0014479715000204
65. Demir Z, Gülser C. Effects of Rice Husk Compost on Some Soil Properties, Water Use Efficiency and Tomato (Solanum lycopersicum L.) Yield under Greenhouse and Field Conditions. Commun Soil Sci Plant Anal. (2021) 52(9):1051–68. doi: 10.1080/00103624.2021.1892731
66. Kiobia DO, Graef HA, Reuben P, Kahimba FC, Graef F, Eichler-Löbermann B, et al. Combining biochar with low rate of chemical fertiliser boosts maize biomass yield, regardless of tillage system, under humid conditions. J Agric Rural Dev Trop Subtrop. (2019) 120:55–62. doi: 10.17170/kobra-20190613557
67. Krismawati A, Arifin Z, Hermanto C, Tafakresnanto C. Application of biochar to improve maize performance on volcanic and sediment soil based parent materials in dry land. IOP Conf Ser Earth Environ Sci. (2021) 648(1):012177. doi: 10.1088/1755-1315/648/1/012177
68. Liao R, Yu H, Yang P, Lin H. Quantitative evaluation of pore characteristics of sodic soils reclaimed by flue gas desulphurization gypsum using X-ray computed tomography. L Degrad Dev. (2020) 31:545–56. doi: 10.1002/ldr.3446
69. Liu SY, Wang N, Xie JC, Jiang RG, Zhao ML. Study on the water retention effect of compound soil of arsenic sandstone and sand under the condition of typical crop planting. IOP Conf Ser Earth Environ Sci. (2017) 82(1):012089. doi: 10.1088/1755-1315/82/1/012089
70. Liu S, Guo ZC, Halder M, Zhang HX, Six J, Peng XH. Impacts of residue quality and soil texture on soil aggregation pathways by using rare earth oxides as tracers. Geoderma. (2021), 399. doi: 10.1016/j.geoderma.2021.115114
71. Masto RE, Ansari MA, George J, Selvi VA, Ram LC. Co-application of biochar and lignite fly ash on soil nutrients and biological parameters at different crop growth stages of Zea mays. Ecol Eng. (2013) 58:314–22. doi: 10.1016/j.ecoleng.2013.07.011
72. Awe GO, Reichert JM, Holthusen D, Ambus JV, de Faccio Carvalho PC. Characterization of microstructural stability of biochar-amended Planosol under conventional tillage for irrigated lowland rice ecosystem. Soil Tillage Res. (2021) 212:105051. doi: 10.1016/j.still.2021.105051
73. Hamza MA, Anderson WK. Responses of soil properties and grain yields to deep ripping and gypsum application in a compacted loamy sand soil contrasted with a sandy clay loam soil in Western Australia. Aust J Agric Res. (2003) 54:273–82. doi: 10.1071/AR02102
74. Yadav GS, Babu S, Das A, Datta M, Mohapatra KP, Singh R, et al. Productivity, soil health, and carbon management index of Indian Himalayan intensified maize-based cropping systems under live mulch based conservation tillage practices. F Crop Res. (2021) 264:108080. doi: 10.1016/j.fcr.2021.108080
75. Tech V, Britt K, McGee J. An introduction to google earth pro. Geospatial extension program; Virginia tech; Department of forest resources and environmental conservation: Blacksburg, VA, USA (2019).
76. Hedges LV, Gurevitch J, Curtis PS. The meta-analysis of response ratios in experimental ecology. Ecology. (1999) 80:1150–6. doi: 10.1890/0012-9658(1999)080[1150:TMAORR]2.0.CO;2
77. Nurida NL, Jubaedah. Biochar enriched with inorganic fertilizer for increasing fertilizer efficiency and soil improvement in acidic upland in East Lampung. IOP Conf Ser Earth Environ Sci. (2019) 393(1):012086. doi: 10.1088/1755-1315/393/1/012086
78. Partovi Z, Ramezani Etedali H, Kaviani A. Effects of applying biochar and straw on nitrate leaching and maize yield production. Water Environ J. (2021) 35:943–50. doi: 10.1111/wej.12684
79. Pandian K, Subramaniayan P, Gnasekaran P, Chitraputhirapillai S. Effect of biochar amendment on soil physical, chemical and biological properties and groundnut yield in rainfed Alfisol of semi-arid tropics. Arch Agron Soil Sci. (2016) 62:1293–310. doi: 10.1080/03650340.2016.1139086
80. Sun J, Cui L, Quan G, Yan J, Wang H, Wu L. Effects of biochar on heavy metals migration and fractions changes with different soil types in column experiments. BioResources. (2020) 15:4388–406. doi: 10.15376/biores
81. Sadowska U, Domagała-światkiewicz I, Żabiński A. Biochar and its effects on plant–soil macronutrient cycling during a three-year field trial on sandy soil with peppermint (Mentha piperita l.). part i: Yield and macro element content in soil and plant biomass. Agronomy. (2020) 10(12):1950. doi: 10.3390/agronomy10121950
82. Abiven S, Hund A, Martinsen V, Cornelissen G. Biochar amendment increases maize root surface areas and branching: a shovelomics study in Zambia. Plant Soil. (2015) 395:45–55. doi: 10.1007/s11104-015-2533-2
83. Cornelissen G, Martinsen V, Shitumbanuma V, Alling V, Breedveld GD, Rutherford DW, et al. Biochar effect on maize yield and soil characteristics in five conservation farming sites in Zambia. Agronomy. (2013) 3:256–74. doi: 10.3390/agronomy3020256
84. Wang X, Yang Y, Zhao J, Nie J, Zang H, Zeng Z, et al. Yield benefits from replacing chemical fertilizers with manure under water deficient conditions of the winter wheat – summer maize system in the North China Plain. Eur J Agron. (2020), 119. doi: 10.1016/j.eja.2020.126118
85. Zheng R, Sun G, Li C, Reid BJ, Xie Z, Zhang B, et al. Mitigating cadmium accumulation in greenhouse lettuce production using biochar. Environ Sci pollut Res Int. (2017) 24:6532–42. doi: 10.1007/s11356-016-8282-9
86. Tammeorg P, Simojoki A, Mäkelä P, Stoddard FL, Alakukku L, Helenius J. Biochar application to a fertile sandy clay loam in boreal conditions: effects on soil properties and yield formation of wheat, turnip rape and faba bean. Plant Soil. (2014) 374:89–107. doi: 10.1007/s11104-013-1851-5
87. Haider G, Steffens D, Moser G, Müller C, Kammann CI. Biochar reduced nitrate leaching and improved soil moisture content without yield improvements in a four-year field study. Agric Ecosyst Environ. (2017) 237:80–94. doi: 10.1016/j.agee.2016.12.019
88. Abdo AI. Changes in sandy soil hydro-physical properties as function of biochar and biogas slurry amendments. Soil Use Manag. (2020) 37(4):762–71. doi: 10.1111/sum.12650
89. Karim AA, Kumar M, Singh E, Kumar A, Kumar S, Ray A, et al. Enrichment of primary macronutrients in biochar for sustainable agriculture: A review. Crit Rev Environ Sci Technol. (2020) 52(9):1449–90. doi: 10.1080/10643389.2020.1859271
90. Sun L, Li J, Wang Q, Zhang Y, Xu Z, Wang R, et al. The effects of eight years of conservation tillage on the soil physicochemical properties and bacterial communities in a rain-fed agroecosystem of the loess plateau, China. L Degrad Dev. (2020) 31:2475–89. doi: 10.1002/ldr.3619
91. Fahreza A, Zaitun, Marliah A, Sabaruddin, Sufardi, Karmil TF, et al. Effect of sawdust biochar and cow manure application on soil fertility at peanut (Arachis hypogaea L.) land. IOP Conf Ser Earth Environ Sci. (2019) 334(1):012068. doi: 10.1088/1755-1315/334/1/012068
92. Mastur S, Suswati D, Hatta M. The effect of ameliorants on improvement of soil fertility in post gold mining land at West Kalimantan. J Degrad Min Lands Manage. (2017) 04:873–80. doi: 10.15243/jdmlm
93. Ch’ng HY, Ahmed OH, Majid NMA, Jalloh MB. Reducing soil phosphorus fixation to improve yield of maize on a tropical acid soil using compost and biochar derived from agro-industrial wastes. Compost Sci Util. (2017) 25:82–94. doi: 10.1080/1065657X.2016.1202795
94. Greenberg I, Kaiser M, Polifka S, Wiedner K, Glaser B, Ludwig B. The effect of biochar with biogas digestate or mineral fertilizer on fertility, aggregation and organic carbon content of a sandy soil: Results of a temperate field experiment. J Plant Nutr Soil Sci. (2019) 182:824–35. doi: 10.1002/jpln.201800496
95. Gruss I, Twardowski JP, Latawiec A, Medyńska-Juraszek A, Królczyk J. Risk assessment of low-temperature biochar used as soil amendment on soil mesofauna. Environ Sci pollut Res Int. (2019) 26:18230–9. doi: 10.1007/s11356-019-05153-7
96. Srank D, Simansky V. Differences in soil organic matter and humus of sandy soil after application of biochar substrates and combination of biochar substrates with mineral fertilizers. Acta Fytotech Zootech. (2020) 23:117–24. doi: 10.15414/afz.2020.23.03.117-124
97. Solaiman ZM, Shafi MI, Beamont E, Anawar HM. Poultry litter biochar increases mycorrhizal colonisation, soil fertility and cucumber yield in a fertigation system on sandy soil. Agric. (2020) 10:1–14. doi: 10.3390/agriculture10100480
98. Sadigov R. Rapid growth of the world population and its socioeconomic results. Sci World J. (1930) 2022:2022. doi: 10.1155/2022/8110229
99. Hartemink AE, Huting J. Land cover, extent, and properties of Arenosols in Southern Africa. Arid L Res Manage. (2008) 22:134–47. doi: 10.1080/15324980801957689
100. Huang J, Hartemink AE. Soil and environmental issues in sandy soils. Earth-Sci Rev. (2020) 208:103295. doi: 10.1016/j.earscirev.2020.103295
101. Wu P, Singh BP, Wang H, Jia Z, Wang Y, Chen W. Bibliometric analysis of biochar research in 2021: a critical review for development, hotspots and trend directions. Biochar. (2023) 5(1):6. doi: 10.1007/s42773-023-00204-2
102. Rahim HU, Allevato E, Radicetti E, Carbone F, Stazi SR. Research trend of aging biochar for agro-environmental applications: a bibliometric data analysis and visualization of the last decade (2011–2023). J Soil Sci Plant Nutr. (2023) 23:4843–55. doi: 10.1007/s42729-023-01456-4
103. Essa RE, Afifi AA, Ahmed EA, El-Ashry SM. Effect of foliar application of yeast and algae on yield and quality of soybean in newly soils. Pakistan J Biol Sci. (2020) 23:1621–8. doi: 10.3923/pjbs.2020.1621.1628
104. Essa RE, Afifi AA, El-Ashry SM, Mohamed MF. Productivity of Some Winter Wheat (Triticum aestivum L.) Varieties through Integrated Application of Vermicompost and Biochar in Sandy Soil. Egypt J Agron. (2023) 45:201–12. doi: 10.21203/rs.3.rs-3026067/v1
105. Jeffery S, Verheijen FGA, van der Velde M, Bastos AC. A quantitative review of the effects of biochar application to soils on crop productivity using meta-analysis. Agric Ecosyst Environ. (2011) 144:175–87. doi: 10.1016/j.agee.2011.08.015
106. Ibrahim A, Horton R. Biochar and compost amendment impacts on soil water and pore size distribution of a loamy sand soil. Soil Sci Soc Am J. (2021) 85:1021–36. doi: 10.1002/saj2.20242
107. Lehmann J, Rillig MC, Thies J, Masiello CA, Hockaday WC, Crowley D. Biochar effects on soil biota - A review. Soil Biol Biochem. (2011) 43:1812–36. doi: 10.1016/j.soilbio.2011.04.022
108. Spokas KA, Novak JM, Stewart CE, Cantrell KB, Uchimiya M, DuSaire MG, et al. Qualitative analysis of volatile organic compounds on biochar. Chemosphere. (2011) 85:869–82. doi: 10.1016/j.chemosphere.2011.06.108
109. Ahmad M, Rajapaksha AU, Lim JE, Zhang M, Bolan N, Mohan D, et al. Biochar as a sorbent for contaminant management in soil and water: A review. Chemosphere. (2014) 99:19–33. doi: 10.1016/j.chemosphere.2013.10.071
110. Major J, Rondon M, Molina D, Riha SJ, Lehmann J. Maize yield and nutrition during 4 years after biochar application to a Colombian savanna oxisol. Plant Soil. (2010) 333:117–28. doi: 10.1007/s11104-010-0327-0
111. Laghari M, Naidu R, Xiao B, Hu Z, Mirjat MS, Hu M, et al. Recent developments in biochar as an effective tool for agricultural soil management: a review. J Sci Food Agric. (2016) 96:4840–9. doi: 10.1002/jsfa.7753
112. Pan F, Li Y, Chapman SJ, Khan S, Yao H. Microbial utilization of rice straw and its derived biochar in a paddy soil. Sci Total Environ. (2016) 559:15–23. doi: 10.1016/j.scitotenv.2016.03.122
113. Mao JD, Johnson RL, Lehmann J, Olk DC, Neves EG, Thompson ML, et al. Abundant and stable char residues in soils: Implications for soil fertility and carbon sequestration. Funct Nat Org Matter Chang Environ. (2013) 9789400756:479–84. doi: 10.1021/es301107c
114. Rahman MA, Jahiruddin M, Kader MA, Islam MR, Solaiman ZM. Sugarcane bagasse biochar increases soil carbon sequestration and yields of maize and groundnut in charland ecosystem. Arch Agron Soil Sci. (2022) 68:1338–51. doi: 10.1080/03650340.2021.1892651
115. Zahra MB, Aftab Z-E-H, Haider MS. Water productivity, yield and agronomic attributes of maize crop in response to varied irrigation levels and biochar–compost application. J Sci Food Agric. (2021) 101(11):4591–604. doi: 10.1002/jsfa.11102
116. Muchane MN, Sileshi GW, Jonsson M, Pumariño L, Barrios E. Agriculture, Ecosystems and Environment Agroforestry boosts soil health in the humid and sub-humid tropics : A meta- analysis. Agriculture, Ecosystems adn Environment. (2020) 15(295):106899. doi: 10.1016/j.agee.2020.106899
117. Kuyah S, Whitney CW, Jonsson M, Sileshi GW, Öborn I, Muthuri CW, et al. Agroforestry delivers a win-win solution for ecosystem services in sub-Saharan Africa. A meta-analysis. Agron Sustain Dev. (2019) 39:1–8. doi: 10.1007/s13593-019-0589-8
118. Lehman RM, Cambardella CA, Stott DE, Acosta-Martinez V, Manter DK, Buyer JS, et al. Understanding and enhancing soil biological health: The solution for reversing soil degradation. Sustain. (2015) 7:988–1027. doi: 10.3390/su7010988
Keywords: sandy soil reclamation, crop yield, biochar, soil health, soil organic carbon, organic amendments, soil moisture content
Citation: Musei SK, Kuyah S, Nyawira S, Ng’ang’a SK, Karugu WN, Smucker A and Nkurunziza L (2024) Sandy soil reclamation technologies to improve crop productivity and soil health: a review. Front. Soil Sci. 4:1345895. doi: 10.3389/fsoil.2024.1345895
Received: 28 November 2023; Accepted: 27 March 2024;
Published: 12 April 2024.
Edited by:
Bharat Prakash Meena, Indian Institute of Soil Science (ICAR), IndiaReviewed by:
Fathy Elbehiry, Kafrelsheikh University, EgyptCopyright © 2024 Musei, Kuyah, Nyawira, Ng’ang’a, Karugu, Smucker and Nkurunziza. This is an open-access article distributed under the terms of the Creative Commons Attribution License (CC BY). The use, distribution or reproduction in other forums is permitted, provided the original author(s) and the copyright owner(s) are credited and that the original publication in this journal is cited, in accordance with accepted academic practice. No use, distribution or reproduction is permitted which does not comply with these terms.
*Correspondence: Sylus Kipngeno Musei, c2lsYXNtdXNlaUBnbWFpbC5jb20=
Disclaimer: All claims expressed in this article are solely those of the authors and do not necessarily represent those of their affiliated organizations, or those of the publisher, the editors and the reviewers. Any product that may be evaluated in this article or claim that may be made by its manufacturer is not guaranteed or endorsed by the publisher.
Research integrity at Frontiers
Learn more about the work of our research integrity team to safeguard the quality of each article we publish.