- 1Horticultural Sciences Department, Institute of Food and Agricultural Sciences, Indian River Research and Education Center, University of Florida, Fort Pierce, FL, United States
- 2Plant Pathology Department, Institute of Food and Agricultural Sciences, Indian River Research and Education Center, University of Florida, Fort Pierce, FL, United States
- 3Horticultural Research Laboratory, U.S. Department of Agriculture, Agricultural Research Services, Ft. Pierce, FL, United States
Introduction: Improving soil fertility is a top priority in Florida’s citrus growing regions, especially in the age of Huanglongbing (HLB; also known as citrus greening). This disease severely reduces fine root mass, causes higher incidences of nutrient deficiencies, and eventually results in the death of affected trees. Additionally, the soils commonly found in Florida’s citrus growing regions are sandy (greater than 98%) and naturally low in fertility, making the nutrient management of HLB-affected trees even more challenging. As a result, interest in organic amendments to increase soil fertility are being tested. Although hardwood chip mulches are successfully used in other regions of the country, no studies exist observing their use on the soils in Florida’s citrus growing regions; therefore, the objectives of this study were to measure the impacts of hardwood oak mulch on (i) Florida Alfisols characteristics and (ii) HLB-affected citrus trees.
Methods: A two-treatment field study using 6-year-old ‘Valencia’ sweet orange trees (Citrus × sinensis) grafted on US-812 (C. reticulata × C. trifoliata) rootstock was conducted in Florida’s Indian River District (IRD). The experimental treatment consisted of 0.08 m of hardwood chip mulch sourced from oak trees applied every September for 3 years (2020, 2021, and 2022) while the control treatment had no mulch applied. Soil chemical and physical properties, leaf nutrient concentration, and leaf Candidatus Liberibacter asiaticus (CLas) titer was collected in the fall (October), winter (January), spring (April), and summer (July).
Results and discussion: Overall, after 3 years, oak mulch applications increased soil available phosphorus (32%), potassium (66%), magnesium (71%), organic matter (49%), and moisture (25-88%, depending on the season); however, oak mulch inconsistently impacted leaf nutrient concentrations and was not effective at suppressing HLB. The results show that annual applications of hardwood oak mulch can improve the chemical and physical properties of sandy soils within three years, however, these improvements did not reduce the severity of HLB.
1 Introduction
Soil is the foundation of agricultural productivity. Although it is widely accepted that soils with high fertility, organic matter, and water holding capacity can lead to increased plant growth and yield, many soils do not have these characteristics at levels high enough to support large scale commercial agriculture without substantial synthetic fertilizer inputs (1–3). While applications of synthetic fertilizers may improve agricultural yields in the short term by providing adequate nutrients to plants, improper application rates and methods can cause nutrient toxicity, reduce microbial activity, and acidify the soil, depleting soil fertility (4, 5). In the past 5 decades, there has been a growing interest in management practices that improve soil fertility. Ideally, these management practices improve soil physical, chemical, and/or biological properties, increasing soil fertility and thus reducing fertilizer and irrigation inputs. The use of cover crops, for example, has become widespread due to the reported increases in organic matter, water holding capacity, and beneficial effects on the microbiome (6–8). Similarly, the use of green manure, compost, biochar, and hardwood mulch have been reported to increase organic matter and soil fertility (9, 10).
Many of these methods are gaining interest in Florida, particularly in the citrus growing regions which have soils with low fertility. The soils found along Florida’s central east coast pose major challenges to the area’s citrus industry. Categorized as eastern flatwoods, the soils predominantly belong to the orders Alfisol and Spodosol (11). These soils generally have organic matter percentages less than 1%, poor cation exchange capacity (CEC), and low nutrient concentrations requiring considerable nutrient management to produce citrus (12). The challenging soil conditions are even more consequential in the age of the devastating citrus disease Huanglongbing (HLB; citrus greening). In the past decade Florida’s citrus production has declined by an estimated 75%, primarily due to HLB (13). The disease is caused by the bacteria Canditatus Liberibacter asiaticus (CLas) which enters the leaf phloem during the feeding activity of the Asian citrus psyllid (Diaphorina citri). During subsequent root and canopy flushes, CLas spreads systemically throughout the tree via the phloem. Symptoms include root and canopy dieback, poor fruit yield and quality, and the eventual death of the affected tree (14, 15). Huanglongbing is now considered endemic to Florida’s citrus growing regions and no cure is currently known (16). As a result, management of HLB-affected citrus focuses on increasing fruit quality and prolonging the producing life of diseased trees. Due to HLB’s deleterious effects on citrus roots, management strategies that improve rhizosphere conditions may improve HLB-affected tree health (17). Additionally, practices that reduce irrigation and/or fertilization costs may improve the economic sustainability of citrus operations in the HLB era (18).
The application of hardwood mulches has not been studied for use in Florida’s citrus growing regions but has been shown to be effective in improving soil fertility in other systems. A study conducted by Germer et al. (19) found that wood chips sourced from sweet cherry tree (Prunus avium) branches improved soil physical properties. In organic apple (Malus × domestica) production, 0.10 m of conifer wood mulch improved soil organic matter and tree growth after 4 years (20). Similarly, Sønsteby et al. (21) showed that spruce bark (Picea abies) led to increased soil moisture and leaf phosphorus (P) and potassium (K) concentrations in strawberry (Fragaria × ananassa) after 3 years.
Mulch sourced from the hardwood portions of oak trees may increase soil fertility and reduce HLB severity. In Florida, it has been reported that citrus trees with HLB had reduced symptoms when growing underneath the dripline of oak trees (22, 23). Research by Pitino et al. (22) showed that liquid extracts from the leaves of laurel oak (Quercus laurifolia) trees reduced CLas titer of HLB-affected sweet orange trees in a greenhouse study. This is consistent with Tanase et al. (24) and Paray et al. (25) which showed liquid oak extracts have antibacterial properties.
With literature supporting the use of hardwood mulches as an effective method to improve soil fertility and evidence indicating that oak trees may contain antibacterial compounds, the use of oak mulch may be an effective strategy for HLB-affected tree management. No studies have been conducted on the use of hardwood chip mulches in the age of HLB; therefore, the objectives of this study were to measure the impacts of hardwood oak mulch on (i) Florida Alfisols characteristics and (ii) HLB-affected citrus trees.
2 Materials and methods
2.1 Site description
This study was conducted at the United States Department of Agriculture, Agricultural Research Service (USD-ARS) Picos farm located in Fort Pierce, Florida, USA (27°26’01.2”N 80°25’51.0”W). Twenty-four ‘Valencia’ sweet orange (Citrus × sinensis) trees grafted on US-812 (C. reticulata × C. trifoliata) rootstock were organized into a completely randomized design with two treatments, oak mulch and control. Each treatment was replicated three times and each replicate (plot) included four trees (a total of 12 trees per treatment). All trees were planted in 2013 and were managed using industry standard practices. The grove soil was classified as Oldsmar fine sand; moreover, this series is a member of the sandy, siliceous, hyperthermic family of Alfic Arenic Haplaquads Glossaqualfs (26). Prior to the start of the experiment (September 2019), soil pH was 6.33 measured in a 1:1 soil to water ratio (27). Soil CEC was 3.71 cmolc kg-1 using Ammonium acetate (NH4CH3CO2) buffered to pH 7 (28). Lastly, percent organic matter was estimated to be < 1% using loss on ignition (29).
All trees received the same irrigation and fertilizer management regardless of experimental treatment. Irrigation was supplied to the experiment using 39.7 L per hour microjet sprinklers (Maxijet, Dundee, FL, USA) placed on top of the mulch. A granular controlled release fertilizer consisting of macro and micro-nutrients was applied around the dripline of each tree in February, June, and September of each year. Nitrogen was applied at 168 kg ha-1, P at 0 kg ha-1, and K at 210 kg ha-1. Fertilizer application rates were calculated based on the soil and leaf nutrient concentrations as well as recommendations by the University of Florida, Institute of Food and Agricultural Sciences (UF/IFAS) (30).
2.2 Treatments
Two treatments were compared in this study. The control treatment received no mulch amendments, whereas the experimental treatment received mulch to a depth of 0.08-m evenly spread on the soil surface around the dripline of sweet orange trees. The mulch was applied every September for three years (2020, 2021, and 2022) on top of the subsequent years mulch and was not tilled or mixed into the soil. The mulch was sourced from the chipped hardwood branches of adult Laurel oak trees (Quercus laurifolia) and was 0.82% N, 0.05% P, 0.20% K, 0.19% Mg and had a C:N ratio of 37.54. Total nutrients added to the soil in each plot by the mulch was estimated to be 7.42 kg of N, 0.18 kg of P, 0.65 kg of K, and 0.68 kg of Mg per year. The mulch also contained 16 mg kg-1 of boron (B), 42 mg kg-1 zinc (Zn), and 48 mg kg-1 of manganese (Mn). Each of these micronutrients added less than 0.12 g per plot.
2.3 Soil analysis
Soil analysis was conducted seasonally (fall = October, winter = January, spring = April), and (summer = July) for three years. Four soil cores were taken around the dripline of each tree in each plot using a soil auger (One-Piece Auger model #400.48, AMS, Inc., American Falls, ID), 0.07 m in diameter and 0.10 m in depth, after removing the top layer of mulch. The cores were mixed to create one pooled sample per plot. Samples were then dried overnight and soil P, K, and magnesium (Mg) concentration was determined using Mehlich- III extraction (31). Briefly, 2.5 g of soil were sieved through a 0.5 mm screen (10 mesh) into an extraction tube (125 mL). A 20 mL of Mehlich III extractant solution (0.2 M CH3COOH + 0.015 M NH4F + 0.013 M HNO3 +0.001 M EDTA + 0.25 M H4NO3) was pipetted into the extraction tube containing the dry soil samples. The extraction tubes were placed on a mechanical shaker for 5 minutes. The soil suspension was filtered and placed into inductively coupled plasma (ICP) racks. Soil nutrients concentration was determined using ICP optical emission spectroscopy (ICP-OES, Spectro Ciros CCD, Fitzburg, MA, USA). The ICP machine was calibrated following manufacturer instruction. The calibration was verified using verification standards and quality controls were ran every 40 samples. Concentrations values from ICP read directly in lbs ac-1 and were later converted in kg ha-1.
Soil moisture was determined using a HH2 Moisture Meter coupled with a 0.06 m Delta-T soil moisture sensor (HH2 Moisture Meter, Delta-T Devices, Cambridge, UK). Four soil moisture readings were taken around the dripline of the 4 trees in each plot.
2.4 Leaf nutrient analysis
Leaf collection began in Summer 2020 and occurred seasonally (fall = October, winter = January, spring = April, and summer = July) for the duration of the experiment. Four mature, fully expanded leaves were collected from each tree in a plot and mixed to create one pooled sample per plot. Samples were dried at 80°C overnight then ground using a Thomas Wiley mill (Thomas Scientific, Swedesboro, NJ, USA) to pass through a 1.0 mm screen. The samples were then mixed with 5 mL of HNO3 in 50 mL vials to the 20 mL volume mark, covered with a watch glass, and placed on a digestor (DigiBlock 3000, SPC Science, USA) at 95°C for 90 minutes. After, 4 mL of 30% H2O2 was added to each tube and the tubes were placed back on the digestor for 20 minutes. The tubes were then cooled for 2 minutes, and distilled water (DI) H2O was added to each tube to reach the 50 mL volume mark. Samples were then examined using inductively coupled plasma mass spectroscopy (ICP-MS Spectro Ciros CCD, Fitzburg, MA, USA) (32). Leaf N, P, K, and Mg concentrations were expressed as a percentage of leaf dry mass.
2.5 Leaf CLas cycle threshold value
One leaf was collected from each quadrant of each tree per plot twice a year (winter and summer) for the entire duration of the study. Only leaves displaying HLB symptoms were sampled. Once collected, leaves were immediately placed on ice until analysis could be conducted. DNA was extracted from freshly ground midrib using the DNeasy Plant Mini kit ® (Qiagen, Hilden, Germany). Quantification of CLas DNA was done with Applied Biosystems 7500 Fast Real-Time PCR System (Thermo Fisher Scientific, Waltham, MS, USA). using the CQULA primers/probe set (33) with a standard curve of 101 to 106 copies of pLBA2 plasmid (34).
2.6 Statistical analysis
The trees were organized into a completely randomized design with two treatments, oak mulch and control. Each treatment was replicated three times and each replicant included four trees. For the statistical analysis, a linear mixed-effects model was fitted to examine the effects of time, treatments (control and mulched plot), and their interaction on soil and tree characteristics. Additionally, a two-sample t-test (p < 0.05) was used to determine significant differences between the control and mulch treatments at individual time points. The software R (https://www.r-project.org) was used to carry out statistical analysis and Microsoft Excel (https://office.microsoft.com/excel) was used to construct graphs.
3 Results
3.1 Soil nutrient and organic matter
Mulch applications impacted soil nutrient content throughout the year. Soil P content in mulched soils was, on average, 32% higher compared to non-mulched soils from Winter 2019 to Winter 2020, and 24% higher in Summer 2021 (Figure 1A). This increase was not observed from Fall 2021 to Summer 2022. Statistical analysis using a linear mixed-effects model further revealed that the treatment, time, and the interaction between treatment and time had significant p-values (p < 0.05) (Supplementary Table 1).
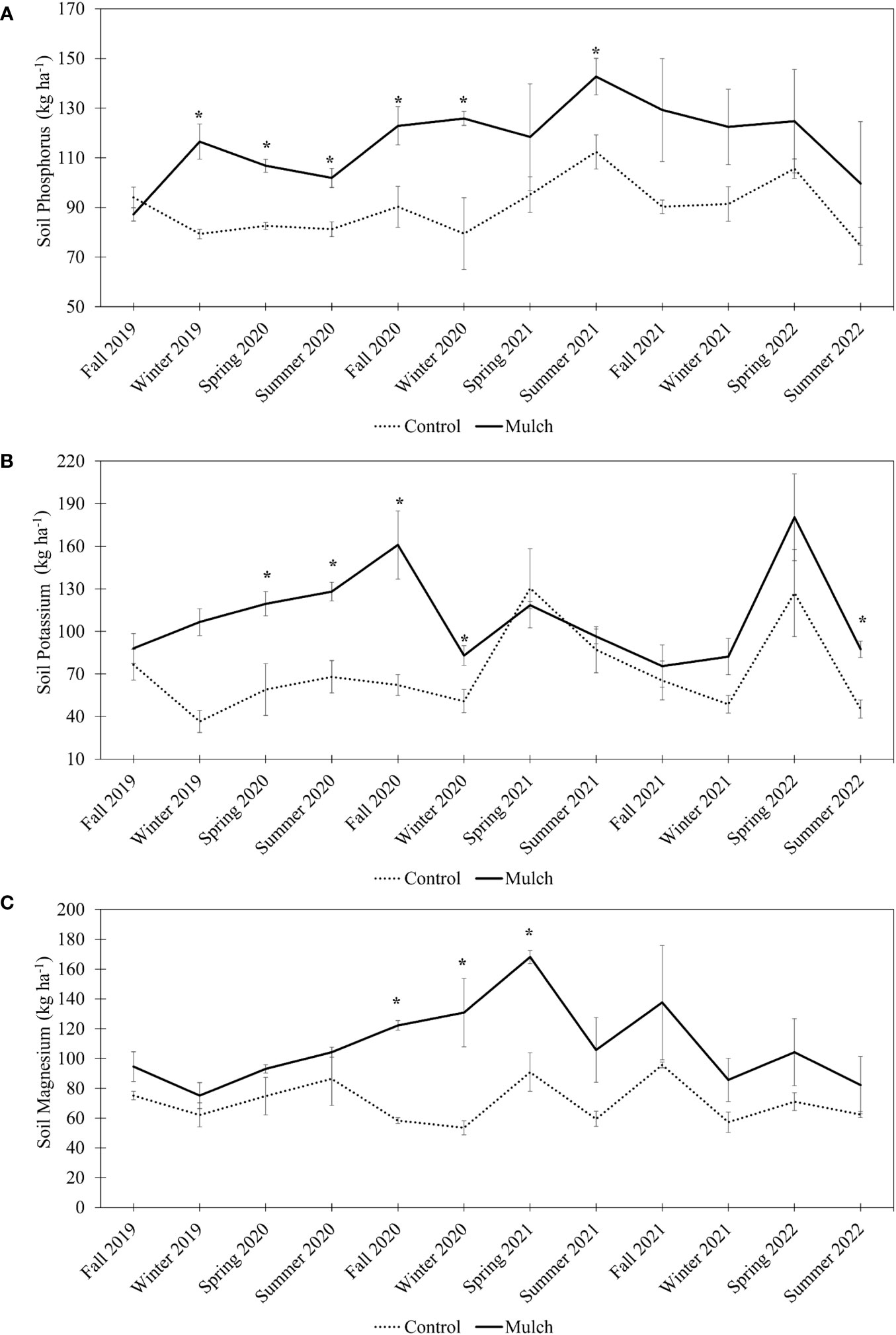
Figure 1 Soil phosphorus (A), soil potassium (B), and soil magnesium (C) in kg ha-1. The measurements were collected seasonally (from Fall 2019 to Summer 2022). Six-year-old HLB-affected ‘Valencia’ sweet orange (Citrus × sinensis) trees grafted on US-812 (C. reticulata × C trifoliata) rootstock were treated with 0.08 m of oak mulch (annually, for 3 years) or not treated with mulch (control). A two-sample t-test (p < 0.05) was used to determine significant differences between treatments. Bars represent standard error. Asterisks (*) indicate differences between means. A linear mixed-effects model was fitted to examine the effects of time, treatments (control and mulched plot), and their interaction on soil characteristics (reported in S1-3).
Similarly, soil K content was on average 66% greater in mulched soils compared to the control from Spring 2020 to Winter 2020 (Figure 1B). No differences were observed from Spring 2021 to Spring 2022, however, mulched soils contained 64% more K at the conclusion of the study. Additionally, significant p-values were observed for the treatment, time, and the interaction between treatment and time (Supplementary Table 2).
No differences in soil Mg were observed between mulched soils and non-mulched soils from Fall 2019 to Summer 2020 and from Summer 2021 to Summer 2022 (Figure 1C). Mulched plots did have an average of 71% more soil Mg compared to non-mulched plots from Fall 2020 to Spring 2021. Significant p-values were observed for the treatment, time, and the interaction between treatment and time (Supplementary Table 3).
No differences in organic matter were observed between the two treatments from Summer 2021 to Winter 2021 (Figure 2A). However, organic matter was 49% greater in mulched plots in Spring 2022. No differences in organic matter between treatments were observed at the conclusion of the study. Although, for the majority of the study, organic matter content was not statistically different between the control and the mulched plots, the average percent organic matter was always higher in mulched plots (between 24%-29%) compared to the control. Additionally, significant p-values were observed for the treatment, time, and the interaction between treatment and time (Supplementary Table 4).
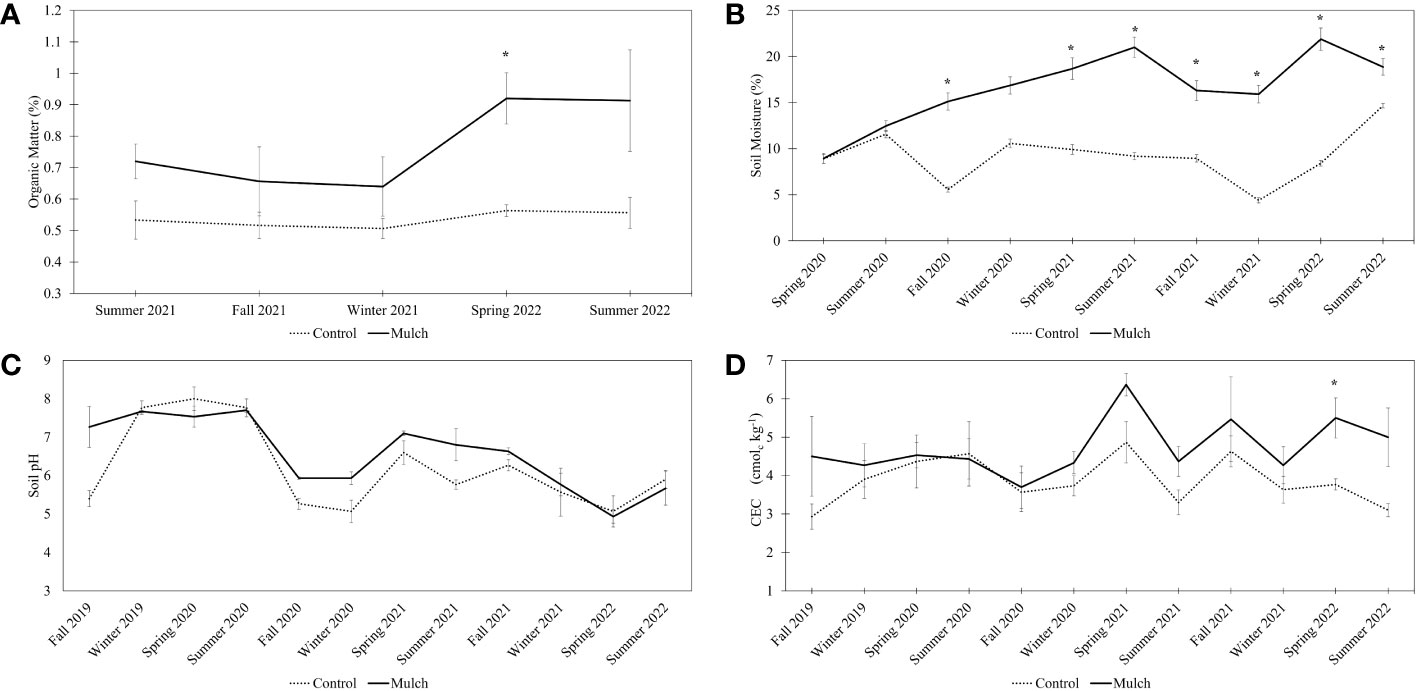
Figure 2 Soil organic matter (%) (A), moisture (%) (B), pH (measured in H2O) (C), and CEC (cmolc kg-1) (D). The measurements were collected seasonally (from Fall 2019 to Summer 2022). Six-year-old HLB-affected ‘Valencia’ sweet orange (Citrus × sinensis) trees grafted on US-812 (C. reticulata × C trifoliata) rootstock were treated with 0.08 m of oak mulch (annually, for 3 years) or not treated with mulch (control). A two-sample t-test (p < 0.05) was used to determine significant differences between treatments. Bars represent standard error. Asterisks (*) indicate differences between means. A linear mixed-effects model was fitted to examine the effects of time, treatments (control and mulched plot), and their interaction on soil characteristics (reported in S4-7).
3.2 Soil moisture, pH, CEC, and visual characteristics
Significant differences in soil moisture were observed throughout most time points (Figure 2B). Mulched plots had increased moisture levels ranging from 25% to 88% more compared to control plots. Significant p-values were observed for the treatment, time, and the interaction between treatment and time were observed for soil moisture (Supplementary Table 5). No differences were observed in pH throughout the study (Figure 2C), however the linear mixed-effects model revealed significant p-values for time and the interaction between treatment and time (Supplementary Table 6). Soil CEC was significantly greater in mulched plots in Spring 2022 (Figure 2D). Additionally, time and the interaction between time and treatment had significant p-values (Supplementary Table 7).
At the conclusion of the study, a 0.06 m O horizon was observed in mulched plots (Figure 3A). This layer was noticeably absent in control plots (Figure 3B). The O horizon was much darker than the soil in control plots and consisted of broken-down mulch, citrus roots, earthworms, and fungi (Figure 3C). These organisms were not observed in control plots (Figure 3D).
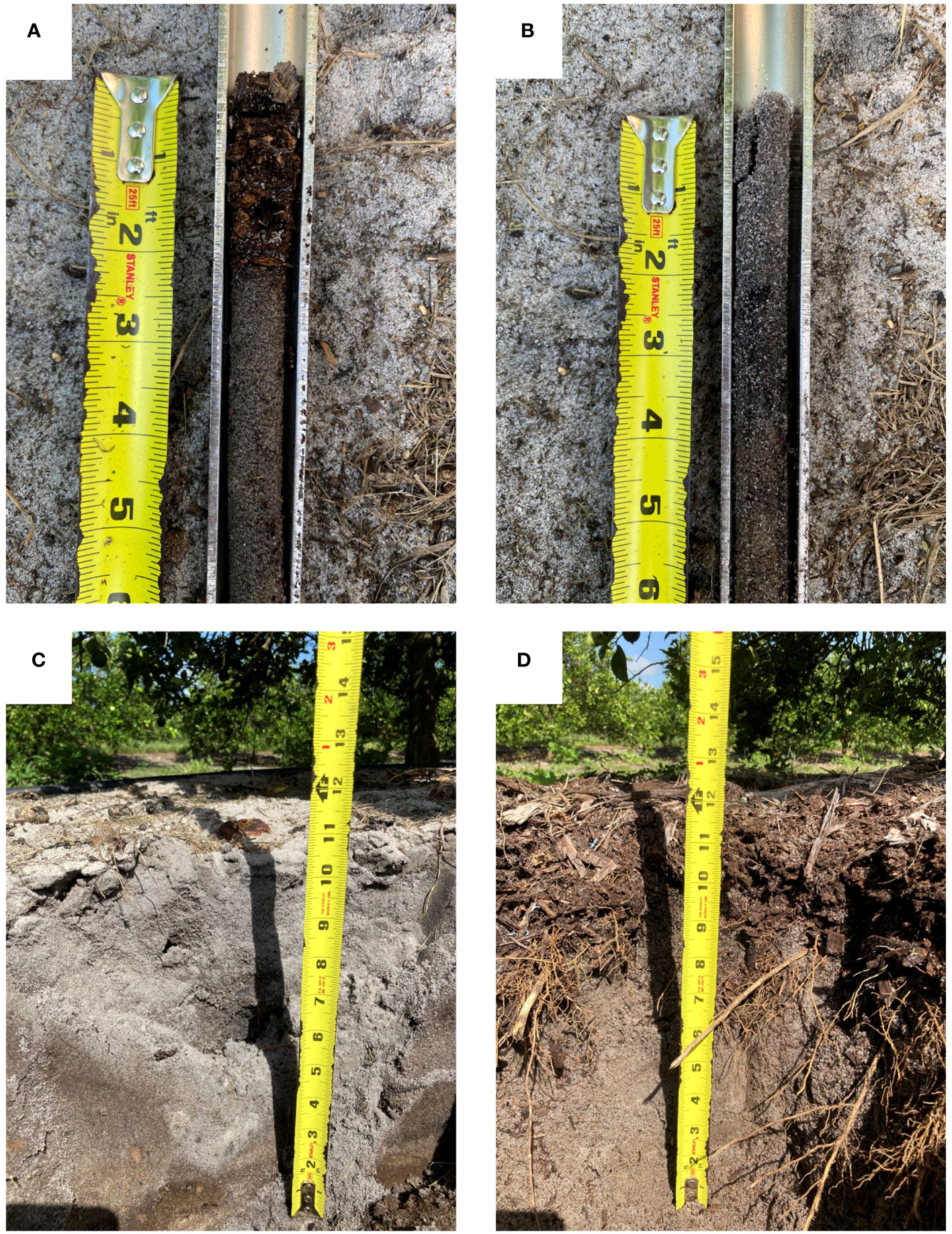
Figure 3 Pictures of soil cores (A, B) and soil horizons (C, D) from mulched plots and control plots. Six-year-old HLB-affected ‘Valencia’ sweet orange (Citrus × sinensis) trees grafted on US-812 (C. reticulata × C trifoliata) rootstock were treated with 0.08 m of oak mulch (annually, for 3 years, starting in Fall 2019) or not treated with mulch (control). Pictures were taken in Summer 2022, at the end of the study.
3.3 Leaf nutrient concentration
Differences were observed in leaf nitrogen (N) between Fall 2021 and Winter 2021 (Figure 4A). Trees in mulched plots contained roughly 10% more N compared to control plots during these two time points. Significant p-values were observed for treatment, time, and the interaction between treatment and time (Supplementary Table 8).
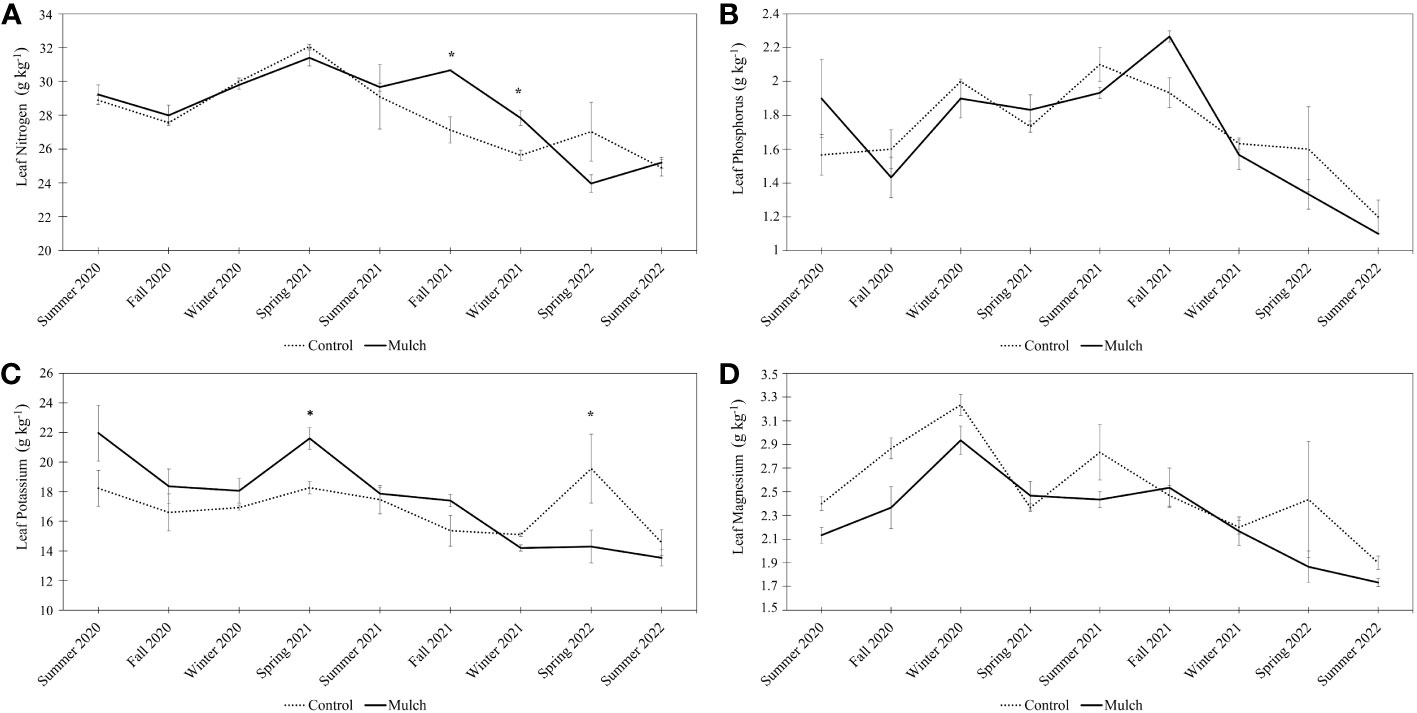
Figure 4 Leaf nitrogen (A), leaf phosphorus (B), leaf potassium (C), and leaf magnesium (D) in g kg-1. The measurements were collected seasonally (from Fall 2019 to Summer 2022). Six-year-old HLB-affected ‘Valencia’ sweet orange (Citrus × sinensis) trees grafted on US-812 (C. reticulata × C trifoliata) rootstock were treated with 0.08 m of oak mulch (annually, for 3 years) or not treated with mulch (control). A two-sample t-test (p < 0.05) was used to determine significant differences between treatments. Bars represent standard error. Asterisks (*) indicate differences between means. A linear mixed-effects model was fitted to examine the effects of time, treatments (control and mulched plot), and their interaction on soil characteristics (reported in S8-11).
No differences were observed in leaf P concentrations between the two treatments (Figure 4B), however, significant p-values were observed for time and the interaction between treatment and time (Supplementary Table 9). Leaf K concentrations only varied at the Spring 2021 and Spring 2022 time points (Figure 4C). During the Spring 2021 time point, trees in the mulched plots contained 17% more K compared to the control. The opposite occurred during the Spring 2022 time point. Trees in the control plots contained 31% more leaf K compared to trees in the mulched plots. Significant p-values were observed for treatment, time, and the interaction between treatment and time (Supplementary Table 10).
No differences were observed in leaf Mg concentrations between the two treatments (Figure 4D), however, significant p-values were observed for treatment and time (Supplementary Table 11).
3.4 Leaf CLas cycle threshold value
All trees at both the start and end of the experiment had cycle threshold (Ct) values less than 30 indicating HLB. During the spring of 2021 and summer of 2022, control trees had higher Ct values compared to mulched trees (Table 1). Additionally, the linear mixed-effects model revealed significant p-values for time treatment, however (Supplementary Table 12), no consistent pattern was established.
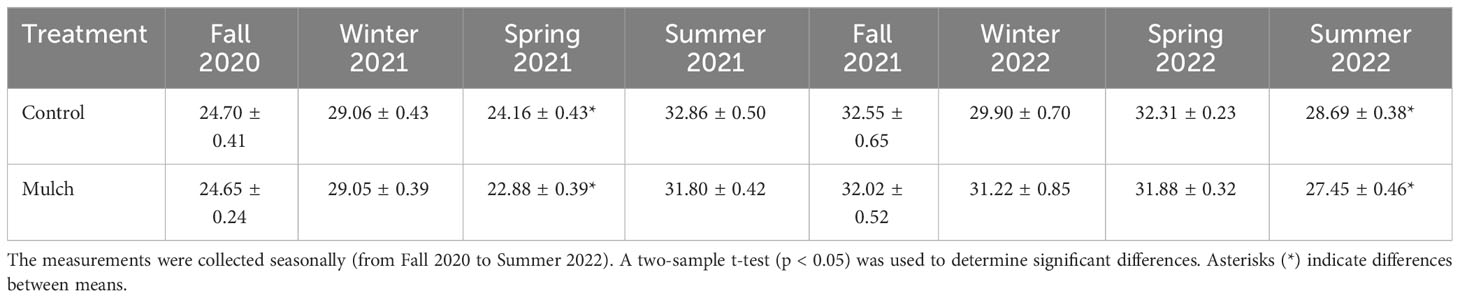
Table 1 Leaf Ct values from six-year-old HLB-affected ‘Valencia’ sweet orange (Citrus × sinensis) trees grafted on US-812 (C. reticulata × C. trifoliata) rootstock and treated with 0.08 m of oak mulch (annually for 3 years) or not treated with mulch (control).
4 Discussion
Soil fertility is a key factor which directly influences the productivity of agricultural land. In regions such as the central east coast of Florida, low fertility soils require extensive synthetic fertilizer inputs to remain productive. This study detected higher nutrient contents, organic matter, and moisture in mulched plots when compared to non-mulched plots. The increased soil nutrient concentrations found in mulched plots were likely a result of the breakdown of the mulch itself, as well as a higher retainment of already present nutrients. Nutrient analysis of the mulch showed 0.82% N, 0.05% P, 0.20% K, and 0.19% Mg, which corresponds to 7.42 kg of N, 0.18 kg of P, 0.65 kg of K, and 0.68 kg of Mg per plot per year. As the mulch decomposed, a portion of these nutrients were likely released into the rhizosphere. Additionally, organic matter content and CEC may have influenced the soil nutrient contents. While significant statistical differences in organic matter and CEC were only observed at one time point, mulched plots had higher averages throughout the study. It is widely accepted that organic matter is negatively charged and can hold cations essential for plant growth such as ammonium, potassium, and magnesium (35, 36).
The results of this study were consistent with research conducted on the impact of hardwood mulches in non-citrus cropping systems outside of Florida. In apple production for example, Yao et al. (37) found that a 0.15 m layer of hardwood chip mulch applied to the soil surface every 2 years increased soil nutrients, CEC, pH, organic matter, soil respiration, and soil bacteria populations during a 10-year study. Similarly, a 7-year study by Jones et al. (38) found that a 0.12 m layer of hardwood chips applied to the soil surface annually, led to higher soil moisture and increased organic matter contents compared to other orchard ground management techniques.
In mulched plots, the increase in soil moisture was observed in the decomposed mulch material and the adjacent soil directly below the recently applied mulch layer. This was expected as it is widely established that organic amendments increase soil moisture (39–41). The mulch is likely to have increased soil moisture by acting as a physical barrier, reducing evaporation (42). The large increases in soil moisture may not only improve soil conditions for microfauna and macrofauna but may reduce the amount of irrigation needed. Citrus roots were observed growing in the decomposed mulch material containing higher moisture, likely benefiting from the increased moisture. This may be of interest to growers looking to reduce the amount of water used in their irrigation practices.
Mulched plots contained a dark layer of decaying mulch whereas controlled plots had no such layer. After 3 years, approximately 0.23 m of mulch was applied. The resulting 0.065 m horizon at the conclusion of the study likely served as a pool for the increased soil nutrients and moisture previously mentioned. This observation is supported by our soil nutrient, moisture and organic matter data as well as literature which shows darker soils are significantly correlated with citrus grove production in Florida (43).
Although the mulch contributed an estimated 7.42 kg of N, 0.18 kg of P, 0.65 kg of K, and 0.68 kg of Mg per year to the soil and increased both organic matter and moisture, it did not, translate into consistent increases in leaf nutrient concentrations. This is similar to a study conducted by Foshee et al. (44), which found that pine bark nuggets applied to pecan trees (Carya illinoinensis) for 3 years led to no increases in leaf K or N; however, the opposite was observed by both Choi et al. (45), which found that apple trees treated with 0.12 m of wood chip mulch annually for 3 years had increased foliar nutrients, and Sønsteby et al. (21), which found that bark mulch applications resulted in increased leaf P and K, but decreased leaf N in a 3-year strawberry study.
The lack of differences in foliar nutrient concentrations between mulched plots and control plots in our study could be due to several factors including the impact of HLB on nutrient dynamics, a reduced root system in HLB-affected trees,. The studies described above were conducted on deciduous fruit trees and non-tree fruits, not citrus trees, likely leading to the differences between results. Additionally, the HLB-induced reduction of the fine root mass greatly impedes nutrient uptake capabilities in affected trees (46). This decrease in nutrient uptake in addition to the disruption and changes to nutrient dynamics within HLB-affected trees is well documented and may be to blame for the differences in results between this study and the current literature (47–51).
Mulch applications did not result in improved tree growth or reduced CLas Ct values. Laurel oak was specifically chosen as the mulch source due to its known antimicrobial properties. In a study by Pitino et al. (22), laurel oak leaf extracts made from 200 g of freshly ground leaves and 2 L of water was sprayed on HLB-affected citrus trees resulting in a decrease in HLB symptoms. The authors identified hydroxycinnamic acids as the likely antimicrobial compounds. In our study, hardwood portions of laurel oaks were used which likely do not contain high enough concentrations of these compounds to be effective against HLB in the field (52).
Although mulch applications did not improve the health of HLB-affected trees, the implications of improving soil fertility may be of interest to citrus growers. It is widely established that increased applications of macronutrients and micronutrients can improve the health, fruit quality, and yield of HLB-affected trees (53–56). The improved soil fertility obtained from mulch applications could logically supply the HLB-affected trees with more nutrients and thus over time improve tree health, yield, and fruit quality. Additionally, synthetic fertilizer applications make up a significant portion of citrus management costs (57–59). If soil fertility can be improved, synthetic fertilizer inputs could be reduced, possibly improving operational profitability. However, it is important to note that mulch material and application costs may outweigh the savings associated with reduced fertilizers.
The data presented above contribute to a clearer understanding regarding the impact of mulch amendments on sandy soils in Florida. Additionally, this research provides insights on the time it takes mulch amendments to improve soil fertility and the mulches lack of impact on tree characteristics in the 3-year timeframe of the study. While this study shows that mulch is not effective at improving HLB-affected tree health in 3-years, it can be used to improve soil fertility; therefore, using mulch amendments to improve soil characteristics may be a promising long-term method for citrus management in the age of HLB. This is also confirmed by the linear mixed-effects model which revealed that treatments, time, and the interaction between treatment and time were significant for most of the parameters observed over the course of the study. Further studies comparing hardwood mulches to other organic amendments are needed, and these studies should be conducted over longer periods of time to determine the impact of mulch on tree growth.
Data availability statement
The raw data supporting the conclusions of this article will be made available by the authors, without undue reservation.
Author contributions
LH contributed to the conceptualization, methodology, data analysis, and writing the original draft. JS contributed to the sample collection, methodology, and review and editing. RS and MP contributed to the conceptualization and review and editing. LR contributed to conceptualization, supervision, methodology, resources, writing-review and editing, and overall project administration. All authors contributed to the article and approved the submitted version.
Funding
This material is based upon work that is supported by the National Institute of Food and Agriculture, U.S. Department of Agriculture, under award #2019-38640-29878 through the Southern Sustainable Agriculture Research and Education program under subaward number GS20-225; and under National Institute of Food and Agriculture, U.S. Department of Agriculture award #2020-70029-33176. This research was also partially supported by the UF/IFAS A. H. Krezdorn Memorial Fund.
Acknowledgments
The authors are grateful to Randy Burton (UF/IFAS) and Steve Mayo (USDA-ARS) for their assistance in mulch acquisition and grove management.
Conflict of interest
The authors declare that the research was conducted in the absence of any commercial or financial relationships that could be construed as a potential conflict of interest.
The author LR declared that they were an editorial board member of Frontiers, at the time of submission. This had no impact on the peer review process and the final decision.
Publisher’s note
All claims expressed in this article are solely those of the authors and do not necessarily represent those of their affiliated organizations, or those of the publisher, the editors and the reviewers. Any product that may be evaluated in this article, or claim that may be made by its manufacturer, is not guaranteed or endorsed by the publisher.
Supplementary material
The Supplementary Material for this article can be found online at: https://www.frontiersin.org/articles/10.3389/fsoil.2023.1200847/full#supplementary-material
References
1. Lv F, Song J, Giltrap D, Feng Y, Yang X, Zhang S. Crop yield and N2O emission affected by long-term organic manure substitution fertilizer under winter wheat-summer maize cropping system. Sci Total Environ (2020) 732:139321. doi: 10.1016/j.scitotenv.2020.139321
2. Csikós N, Tóth G. Concepts of agricultural marginal lands and their utilisation: A review. Agric Syst (2023) 204:103560. doi: 10.1016/j.agsy.2022.103560
3. Guan X, Cheng Z, Li Y, Wang J, Zhao R, Guo Z, et al. Mixed organic and inorganic amendments enhance soil microbial interactions and environmental stress resistance of Tibetan barley on plateau farmland. J Environ Manage (2023) 330:117137. doi: 10.1016/j.jenvman.2022.117137
4. Foley JA, Defries R, Asner GP, Barford C, Bonan G, Carpenter SR, et al. Global consequences of land use. Science (2005) 309:570–4. doi: 10.1126/science.1111772
5. Scotti R, Pane C, Spaccini R, Palese AM, Piccolo A, Celano G, et al. On-farm compost: a useful tool to improve soil quality under intensive farming systems. Appl Soil Ecol (2016) 107:13–23. doi: 10.1016/j.apsoil.2016.05.004
6. Ding G, Liu X, Herbert S, Novak J, Amarasiriwardena D, Xing B. Effect of cover crop management on soil organic matter. Geoderma (2006) 130:229–39. doi: 10.1016/j.geoderma.2005.01.019
7. Ghimire R, Ghimire B, Mesbah AO, Idowu OJ, O’neill MK, Angadi SV, et al. Current status, opportunities, and challenges of cover cropping for sustainable dryland farming in the Southern Great Plains. J Crop Improv. (2018) 32:579–98. doi: 10.1080/15427528.2018.1471432
8. Castellano-Hinojosa A, Martens-Habbena W, Smyth AR, Kadyampakeni DM, Strauss SL. Short-term effects of cover crops on soil properties and the abundance of N-cycling genes in citrus agroecosystems. Appl Soil Ecol (2022) 172:104341. doi: 10.1016/j.apsoil.2021.104341
9. Schulz H, Dunst G, Glaser B. Positive effects of composted biochar on plant growth and soil fertility. Agron Sustain Dev (2013) 33:817–27. doi: 10.1007/s13593-013-0150-0
10. Zhang M, Zhang L, Riaz M, Xia H, Jiang C. Biochar amendment improved fruitquality and soil properties and microbial communities at different depths in citrusproduction. J Cleaner Prod (2021) 292:126062. doi: 10.1016/j.jclepro.2021.126062
11. Srivastava AK, Singh S. Citrus decline: soil fertility and plant nutrition. J Plant Nutr (2009) 32:197–245. doi: 10.1080/01904160802592706
12. Davies FS, Jackson LK. Citrus growing in Florida. In: Gainesville, FL: University of Florida Press (2009).
13. USDA. Florida Citrus Statistics 2020-2021. Maitland, FL: United States Department of Agriculture National Agriculture Statistics Service. (2022).
14. Bassanezi RB, Montesino LH, Stuchi ES. Effects of huanglongbing on fruit quality of sweet orange cultivars in Brazil. Eur J Plant Pathol (2009) 125:565. doi: 10.1007/s10658-009-9506-3
15. Graham JH, Johnson EG, Gottwald TR, Irey MS. Presymptomatic fibrous root decline in citrus trees caused by huanglongbing and potential interaction with phytophthora spp. Plant Dis (2013) 97:1195–9. doi: 10.1094/PDIS-01-13-0024-RE
16. Singerman A, Futch SH, Page B. Early performance of selected citrus rootstocks grafted with ‘Valencia’ Sweet orange in commercial settings within a citrus greening endemic environment. HortTechnol. hortte (2021) 31:417–27. doi: 10.21273/HORTTECH04784-20
17. Rossi L, Hallman LM, Santiago JM. Citrus root and rhizosphere dynamics in the age of HLB. Annu Plant Rev Onl. (2021) 4:605–24. doi: 10.1002/9781119312994.apr0769
18. Uthman QO, Atta AA, Kadyampakeni DM, Qureshi JA, Morgan KT, Nkedi-Kizza P. Integrated water, nutrient, and pesticide management of huanglongbing-affected sweet oranges on Florida sandy soils—A review. Plants (2022) 11:1850. doi: 10.3390/plants11141850
19. Germer S, Dongen RV, Kern J. Decomposition of cherry tree prunings and their short-term impact on soil quality. Appl Soil Ecol (2017) 117-118:156–64. doi: 10.1016/j.apsoil.2017.05.003
20. Neilsen G, Forge T, Angers D, Neilsen D, Hogue E. Suitable orchard floor management strategies in organic apple orchards that augment soil organic matter and maintain tree performance. Plant Soil (2014) 378:325–35. doi: 10.1007/s11104-014-2034-8
21. Sønsteby A, Nes A, Måge F. Effects of bark mulch and npk fertilizer on yield, leaf nutrient status and soil mineral nitrogen during three years of strawberry production. Acta Agricult. Scandinavica Sec. B — Soil Plant Sci (2004) 54:128–34. doi: 10.1080/09064710410030276
22. Pitino M, Sturgeon K, Dorado C, Cano LM, Manthey JA, Shatters RG, et al. Quercus leaf extracts display curative effects against Candidatus Liberibacter asiaticusthat restore leaf physiological parameters in HLB-affected citrus trees. P Phys Biochem (2020) 148:70–9. doi: 10.1016/j.plaphy.2020.01.013
23. Vincent C, Guha A, Killiny N, Diepenbrock L. Understory environment promotes photosynthetic efficiency and mitigates severity and function of an introduced, vectored pathosystem: a study of a feral citrus population in central Florida. Trees (2021) 35:1711–25. doi: 10.1007/s00468-021-02147-2
24. Tanase C, Nicolescu A, Nisca A, Ştefănescu R, Babotă M, Mare AD, et al. Biological activity of bark extracts from northern red oak (Quercus rubra L.): an antioxidant, antimicrobial and enzymatic inhibitory evaluation. Plants (2022) 11:2357. doi: 10.3390/plants11182357
25. Paray BA, Hoseini SM, Hoseinifar SH, Van Doan H. Effects of dietary oak (Quercus castaneifolia) leaf extract on growth, antioxidant, and immune characteristics and responses to crowding stress in common carp (Cyprinus carpio). Aquaculture (2020) 524:735276. doi: 10.1016/j.aquaculture.2020.735276
27. Miller RO, Kissel DE. Comparison of soil pH methods on soils of North America. Soil Sci Soc America J (2010) 74:310–6. doi: 10.2136/sssaj2008.0047
28. Schollenberger CJ, Simon RH. Determination of exchange capacity and exchangeable bases in soil—ammonium acetate method. Soil Sci (1945) 59:13-24.
29. Schulte EE, Hopkins BG. Estimation of Soil Organic Matter by Weight Loss-On-Ignition. In: Soil Organic Matter: Analysis and Interpretation. Madison, Wisconsin, USA: Soil Science Society of America (1996). p. 21–31.
30. Morgan KT, Kadyampakeni DM, Zekri M, Schumann AW, Vashisth T, Obreza TA. 2020-2021 Florida Citrus Production Guide: Nutrition Management for Citrus Trees. Gainesville, Florida, USA: UF IFAS Extension (2020).
31. Mylavarapu R, Obreza T, Morgan K, Hochmuth G, Nair V, Wright A. Extraction of Soil Nutrients Using Mehlich-3 Reagent for Acid-Mineral Soils of Florida. Gainesville, Florida, USA: University of Florida Institute of Food and Agriculture Science Electronic Data Information Source (UF/IFAS) (2014).
32. Isaac RA, Johnson WC. Elemental Analysis of Plant Tissue by Plasma Emission Spectroscopy: Collaborative Study. J Assoc Official Analytical Chemists (1995) 68:3. doi: 10.1093/jaoac/68.3.499
33. Wang Z, Yin Y, Hu H, Yuan Q, Peng G, Xia Y. Development and application of molecular-based diagnosis for ‘Candidatus Liberibacter asiaticus’, the causal pathogen of citrus huanglongbing. Plant Pathology (2006) 55:630–8. doi: 10.1111/j.1365-3059.2006.01438.x
34. Trivedi P, Sagaram US, Kim JS, Brlansky RH, Rogers ME, Stelinski LL, et al. Quantification of viable Candidatus Liberibacter asiaticus in hosts using quantitative PCR with the aid of ethidium monoazide (EMA). European J Plant Pathol (2009) 124:553–63. doi: 10.1007/s10658-009-9439-x
35. Helling CS, Chesters G, Corey RB. Contribution of organic matter and clay to soil cation-exchange capacity as affected by the pH of the saturating solution. Soil Sci Soc America J (1964) 28:517–20. doi: 10.2136/sssaj1964.03615995002800040020x
36. Christensen BT, Johnston AE. Chapter 18 Soil organic matter and soil quality—Lessons learned from long-term experiments at Askov and Rothamsted. In: Gregorich EG, Carter MR, editors. Developments in Soil Science. Amsterdam, NL: Elsevier. (1997). p. 399–430.
37. Yao S, Merwin IA, Bird GW, Abawi GS, Thies JE. Orchard floor management practices that maintain vegetative or biomass groundcover stimulate soil microbial activity and alter soil microbial community composition. Plant Soil (2005) 271:377–89. doi: 10.1007/s11104-004-3610-0
38. Jones J, Savin MC, Rom CR, Gbur E. Soil microbial and nutrient responses over seven years of organic apple orchard maturation. Nutrient Cycling Agroeco. (2020) 118:23–38. doi: 10.1007/s10705-020-10080-y
39. Thakur M, Kumar R. Mulching: Boosting crop productivity and improving soil environment in herbal plants. J Appl Res Med. Aromatic Plants (2021) 20:100287. doi: 10.1016/j.jarmap.2020.100287
40. Tuure J, Räsänen M, Hautala M, Pellikka P, Mäkelä PSA, Alakukku L. Plant residue mulch increases measured and modelled soil moisture content in the effective root zone of maize in semi-arid Kenya. Soil Tillage Res (2021) 209:104945. doi: 10.1016/j.still.2021.104945
41. El-Beltagi HS, Basit A, Mohamed HI, Ali I, Ullah S, Kamel E, et al. Mulching as a sustainable water and soil saving practice in agriculture: A review. Agronomy (2022) 12:1881. doi: 10.3390/agronomy12081881
42. Jiménez MN, Pinto JR, Ripoll MA, Sánchez-Miranda A, Navarro FB. Impact of straw and rock-fragment mulches on soil moisture and early growth of holm oaks in a semiarid area. CATENA (2017) 152:198–206. doi: 10.1016/j.catena.2017.01.021
43. Qamar Uz Z, Schumann AW. Nutrient management zones for citrus based on variation in soil properties and tree performance. Precis Agric (2006) 7:45–63. doi: 10.1007/s11119-005-6789-z
44. Foshee W, Goff WD, Patterson MG, Tilt KM, Dozier W, Tucker U, et al. Organic mulches affect soil and leaf nutrient levels of young pecan trees. J Arboricult (1999) 25:81–4. doi: 10.48044/jauf.1999.012
45. Choi H-S, Rom CR, Gu M. Effects of different organic apple production systems on seasonal nutrient variations of soil and leaf. Sci. Hortic (2011) 129:9–17. doi: 10.1016/j.scienta.2011.02.009
46. Mattos-Jr D, Kadyampakeni DM, Da Silva JR, Vashisth T, Boaretto RM. Reciprocal effects of huanglongbing infection and nutritional status of citrus trees: a review. Trop Plant Pathol (2020) 45:586–96. doi: 10.1007/s40858-020-00389-y
47. Pustika AB, Subandiyah S, Holford P, Beattie G, Iwanami T, Masaoka Y. Interactions between plant nutrition and symptom expression in mandarin trees infected with the disease huanglongbing. Austral Plant Disease Notes (2008) 3:112–5. doi: 10.1007/BF03211261
48. Shahzad F, Chun C, Schumann A, Vashisth T. Nutrient uptake in huanglongbing-affected sweet orange: transcriptomic and physiological analysis. J Am Soc Hortic Sci J Amer. Soc Hortic Sci (2020) 145:349–62. doi: 10.21273/JASHS04929-20
49. Hallman LM, Kadyampakeni DM, Ferrarezi RS, Wright AL, Ritenour MA, Johnson EG, et al. Impact of ground applied micronutrients on root growth and fruit yield of severely huanglongbing-affected grapefruit trees. Horticulturae (2022) 8:763. doi: 10.3390/horticulturae8090763
50. Hallman LM, Kadyampakeni DM, Fox J-P, Wright AL, Rossi L. Root-shoot nutrient dynamics of huanglongbing-affected grapefruit trees. Plants (2022) 11:3226. doi: 10.3390/plants11233226
51. Hallman LM, Kadyampakeni DM, Ferrarezi RS, Wright AL, Ritenour MA, Rossi L. Uptake of micronutrients in severely HLB-affected grapefruit trees grown on Florida Indian River flatwood soils. J Plant Nutr (2023) 1-15:4110–24. doi: 10.1080/01904167.2023.2221287
52. Morales D. Oak trees (Quercus spp.) as a source of extracts with biological activities: A narrative review. Trends Food Sci Technol (2021) 109:116–25. doi: 10.1016/j.tifs.2021.01.029
53. Atta AA, Morgan KT, Mahmoud KA. Split application of nutrients improve growth and yield of Huanglongbing-affected citrus trees. Soil Sci Soc America J (2021) 85:2040–53. doi: 10.1002/saj2.20310
54. Esteves E, Locatelli G, Bou NA, Ferrarezi RS. Sap analysis: A powerful tool for monitoring plant nutrition. Horticulturae (2021) 7:426. doi: 10.3390/horticulturae7110426
55. Kadyampakeni DM, Chinyukwi T. Are macronutrients and micronutrients therapeutic for restoring performance of trees affected by citrus greening? A discussion of current practices and future research opportunities. J Plant Nutr (2021) 44(19):2949–69. doi: 10.1080/01904167.2021.1927079
56. Hussain Z, Iftikhar Y, Mubeen M, Saleem MZ, Naseer MU, Luqman M, et al. Application of micronutrients enhances the quality of kinnow mandarin infected by citrus greening disease (Huanglongbing). Sarhad J Agric (2022) 38:360–71. doi: 10.17582/journal.sja/2022/38.1.360.371
57. Stansly PA, Arevalo HA, Qureshi JA, Jones MM, Hendricks K, Roberts PD, et al. Vector control and foliar nutrition to maintain economic sustainability of bearing citrus in Florida groves affected by huanglongbing. Pest Manage Sci (2014) 70:415–26. doi: 10.1002/ps.3577
58. Tansey JA, Vanaclocha P, Monzo C, Jones M, Stansly PA. Costs and benefits of insecticide and foliar nutrient applications to huanglongbing-infected citrus trees. Pest Manage Sci (2017) 73:904–16. doi: 10.1002/ps.4362
Keywords: citrus greening, soil fertility, organic matter, soil moisture, sandy soil
Citation: Hallman LM, Santiago JM, Fox J-P, Pitino M, Shatters RG Jr. and Rossi L (2023) Use of hardwood mulch applications to improve soil characteristics of Alfisols used in Florida citrus production. Front. Soil Sci. 3:1200847. doi: 10.3389/fsoil.2023.1200847
Received: 05 April 2023; Accepted: 25 September 2023;
Published: 09 October 2023.
Edited by:
Thiago Nogueira, São Paulo State University, BrazilReviewed by:
Haiying Tao, University of Connecticut, United StatesPaulo Pagliari, University of Minnesota Twin Cities, United States
Copyright © 2023 Hallman, Santiago, Fox, Pitino, Shatters and Rossi. This is an open-access article distributed under the terms of the Creative Commons Attribution License (CC BY). The use, distribution or reproduction in other forums is permitted, provided the original author(s) and the copyright owner(s) are credited and that the original publication in this journal is cited, in accordance with accepted academic practice. No use, distribution or reproduction is permitted which does not comply with these terms.
*Correspondence: Lorenzo Rossi, bC5yb3NzaUB1ZmwuZWR1
†Present address: Marco Pitino, AgroSource, Inc., Jupiter, FL, United States