- 1School of Agricultural Sciences, Makerere University, Kampala, Uganda
- 2International Institute of Tropical Agriculture, Yaoundé, Cameroon
Introduction: Farming systems influence composition and abundance of microbial communities.
Methodology: A study was conducted using morphotyping and enumeration methods to determine the composition and spore abundance of Arbuscular Mycorrhizal Fungi (AMF) in sweet potato producing regions in eastern Uganda. Sampling was done from fields with crop types (CTs) including legumes (groundnuts, common beans, cowpea, soybeans, green grams), sorghum, sweet potato, and fallowed fields which were used as a control. Three agro-ecological zones (AEZs) i.e., Mt. Elgon High Farmlands (MEHF), Lake Victoria Crescent (LVC), and Southern and Eastern Lake Kyoga Basin (SELKB) were considered.
Results and discussion: A total of 6 AMF genera comprising of Glomus, Acaulospora, Scutellospora, Entrophospora, Archaeospora, and Gigaspora were isolated from the study sites. Agro-ecological zones had a significant (p<0.05) effect on Entrophospora spp. while crop types had a significant (p<0.05) effect on Gigaspora spp. although all the AMF genera were present in all AEZs and CTs. Spore abundance was similar across the AEZs except for MEHF (177) which was lower while spore abundance lowest in sweet potato (177) and largest in fallow (224), attributed to soil properties and similar crops included in the crop rotation program. The AMF can be isolated, identified, and multiplied to produce bioinoculants for the regions.
1 Introduction
Arbuscular mycorrhizal fungi are obligate endosymbionts of up to 90% terrestrial plants (1) belonging to the phylum Glomeromycota (2) and sub-phylum Glomeromycotina (3). They are gaining prominence in agriculture for increasing crop production. Therefore, understanding their biogeographical patterns and drivers for maintaining ecosystem services amidst changes in farming systems aimed at agro-ecological adaptability is a prerequisite. Various biotic and abiotic factors and biological processes are reported to impact on microbial biogeographical patterns (4, 5). Several studies have identified different edaphic variables that influence soil bacterial and fungal community compositions (6). Abiotic factors include soil type (7), soil texture (8), soil acidity (9), soil temperature (10), soil moisture (11), soil available P (12) and cropping systems (tillage, crop rotation, fallowing) (13–16). Öpik et al. (17) reported that AMF communities vary in composition due to differences in ecosystems under different disturbance regimes. Other factors include organic matter (18), soil biota (19, 20), and plant communities (21, 22).
Some studies in sub-Saharan Africa have focused on the diversity and spore abundance of AMF (e.g., 23–26) but have not emphasized agricultural zoning and cropping systems moreover in Uganda. Cropping systems influence the biological, physical, and chemical properties of soils, as well as the geographical distribution of plants, which greatly impact on the diversity and spore abundance of AMF (23). Crops such as cereals, legumes, coffee, bananas, cassava, and other root and tuber crops that benefit from AMF associations dominate Africa’s landscape (27). Less frequently cultivated fallow fields always have higher spore abundance than fields under frequent conventional cultivation (23). In the drier areas of the Maasai-Mara Ecosystem in Kenya, maize and wheat monocrops recorded significantly lower AMF diversity, species richness, and spore density in the wet and dry season than the maize-bean intercrops dominated by Scutellospora and Acaulospora species (28). Similarly, the dominance of Scutellospora and Acaulospora had been reported earlier in Western Kenya (29). Sporulation of genus Acaulospora was high in acidic soils (26, 30), increasing their dominance in soils of a pH range of 5.51 to 6.77 (28). Castillo et al. (31) reported high sporulation of Acaulospora spp. Under conventional tillage than no-tillage. On the other hand, Jansa et al. (32) reported higher sporulation of Scutellospora spp. In undisturbed and moderately disturbed soils. The limited occurrence of Gigaspora spp. In various ecosystems compared to other AMF genera has been confirmed by their low density reported by Schalamuk et al. (33) Jefwa et al. (34), and Muchane et al. (28).
In the study of Belay et al. (24) a total of 15 AMF genera (Glomus, Acaulospora, Funneliformis, Gigaspora, Scutellospora, Septoglomus, Claroideoglomus, Entrophospora, Rhizophagus, Paraglomus, Diversispora, Pacispora, Racocetra, Sclerocytis, and Ambispora) were isolated from both field and trap culture soils. A total of 31 species was observed in the study in an irrigated mixed fruit cropping system that received manure followed by 23 species in a crop rotation field with teff, sesame, and sunflower that received 50 kg Urea ha-1 and 100 kg DAP ha-1 (24). In the same study, 15 AMF species were noted in a 30-year-old natural forest with acacia, fig, and stinkwood trees, 14 species in an acacia plantation, and up to 11 species in fields of sorghum or maize monocrops receiving 50 to 100 kg Urea ha-1 and 100 to 150 kg DAP ha-1 (24). Glomus and Acaulospora AMF were the most diverse groups represented by 9 species each, followed by Funneliformis and Gigaspora. Glomus and Acaulospora spp. Produce more spores in a shorter time than Scutellospora and Gigaspora in the same environment (23, 35, 36). Seven genera comprising Acaulospora, Ambispora, Glomus, Claroideoglomus, Pacispora, Gigaspora, and Scutellospora were isolated from cassava cropping fields in Abengourou, East Côte d’Ivoire of which the genus Glomus was dominant (37). Earlier on in South Africa, studies had shown that the rhizosphere of cassava in Limpopo contained Acaulospora scrobiculata, Glomus rubiforme, and Gigaspora sp. Whereas the Mpumulanga soils had Acaulospora scrobiculata, Acaulospora mellea, Acaulospora racticede, Glomus etunicatum, Glomus rubiforme, Gigaspora sp, and Scutellospora sp (38).
However, no studies have been carried out on the composition and spore abundance of AMF in fields under sweet potato production. Therefore, the objective of the study was to determine the composition and spore abundance of AMF as influenced by different crop types (CTs) in three sweet potato producing AEZs in eastern Uganda.
2 Materials and methods
2.1 Study sites description
The study was conducted in eastern Uganda covering three agro-ecological zones (AEZ) namely, Mt. Elgon High Farmlands (MEHF), Lake Victoria Crescent (LVC), and Southern and Eastern Lake Kyoga Basin (SELKB). The sites were distributed, in Magola and Rubongi sub-counties of Tororo district (MEHF); Busware and Banda sub-counties of Namayingo district (LVC) and, in Bukedea, Ongi’no, Malera, Kolir, and Kachumbala sub-counties of Bukedea district (SELKB). The landscape of MEHF has steep slopes and is divided by many valleys. The climate is cool and wet with the southern part being warmer with less rain in July than the northern part. Rainfall peaks in April and May but is generally more than 100 mm per month from March to November. In the LVC, the landscape of West of the Nile River in the LVC is an old land surface marked by ridges or laterite-capped hills, long slopes, and wide, often swampy valleys while on the East of the Nile, the landscape is rolling with wide valleys and relatively less rolling. Soils are often acidic and low in K, but with moderate levels of organic matter. Southern and eastern Lake Kyoga Basin (SELKB) has a gently rolling landscape with wide valleys draining to Lake Kyoga. The soils of the western part of this zone are generally loamy on the ridges and upper slopes and sandy loam on the lower slopes. This sub-humid AEZ has two cropping seasons with almost equal average rainfall intensity, 560 mm during March-June, and 540 mm during July-November (Figure 1).
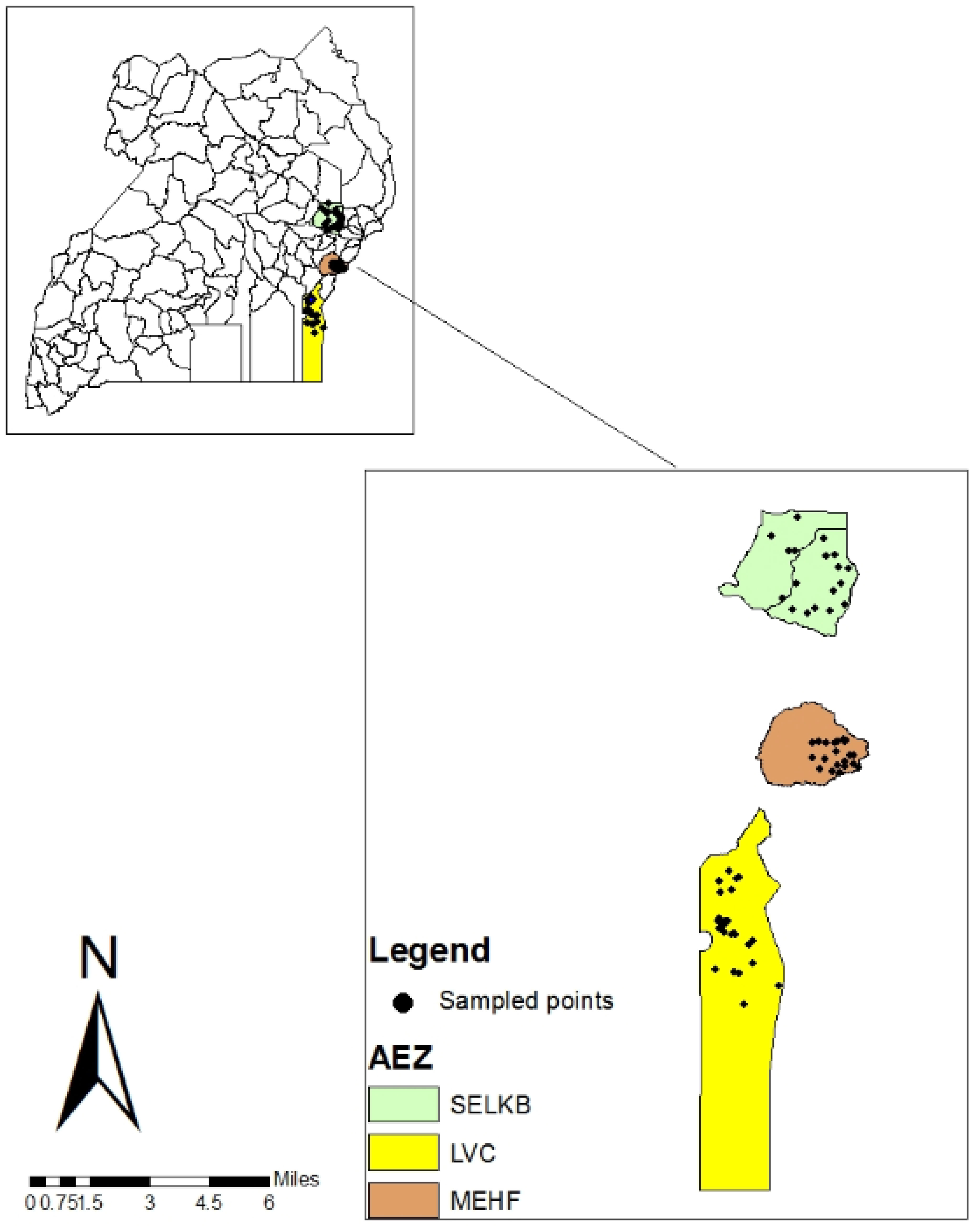
Figure 1 A map showing study areas in eastern Uganda. SELKB, Southern and Eastern Lake Kyoga Basin; MEHF, Mt. Elgon High Farmlands; LVC, Lake Victoria Crescent.
The area under sweet potato production in eastern Uganda is 159,948 hectares and the average yield is 5.3 t ha-1 while in central Uganda 98,054 hectares are under sweet potato production with an average yield of 3.2 t ha-1 (39). Further description of the AEZs is shown in Table 1.
2.2 Soil sampling procedure
A multistage sampling frame was used to select study sites. The study sites were selected considering the major sweet potato producing regions targeting different crop types and hence the selection of Mt. Elgon High Farmlands (MEHF), Lake Victoria Crescent (LVC), and Southern and Eastern Lake Kyoga Basin (SELKB). Districts that experience moisture stress conditions were considered and hence Tororo, Namayingo and Bukedea were selected. Occurrence of moisture stress conditions in these study sites is attributed to the high temperature recorded and rainfall that is unreliable and highly variable in terms of its onset, cessation, amount, and distribution (44). Sub counties i.e., Tororo (Magola and Rubongi sub-counties), Namayingo (Busware and Banda sub-counties) and Bukedea (Ongi’no, Malera, Kolir, and Kachumbala sub-counties) were randomly selected. In the selected sub-counties, a list of smallholder farmers (producing the targeted crops) was proposed by contact farmers in the respective sub-counties and farmers were randomly selected from the list. Farmers’ fields were used as replicates and the samples were obtained following the existing crop types and soil amendments on that field. The geographical location for each field was captured using a Geographical Positioning System (GPS). Farmers provided more details on the fields that were sampled from for the previous 5 years (Table 2). Nineteen (19) samples were obtained from SELKB, 20 from MEHF and 25 from LVC. It is worth noting that the farmers had racticed mono-cropping, inter-cropping, and crop rotation including cassava, groundnuts, sweet potato, maize, soybean, green grams, millet, sesame, oranges, eggplant, green pepper, cabbage, mangoes, onions, cowpea, upland rice, sunflower, and watermelon and, short-term fallows. A soil auger was used to obtain 12 samples from each site in a zigzag pattern at a depth of 0 – 20 cm, which were pooled together, and a 2 kg composite homogeneous sample obtained. The homogeneous sample was placed in a strong polythene bag and sealed securely to prevent further drying and labeled clearly for ease of identification. Samples were collected in a dry season, a period when sporulation increases (45). The samples were split for use in AMF identification and soil physical and chemical analysis.
2.3 Soil physical and chemical analysis
Each soil sample for physico-chemical analysis was air-dried, sieved through 2 mm sieve, homogenized, and analyzed for pH (1 soil:2.5 H2O ratio), soil organic carbon (SOC), total and available P, exchangeable cations (Ca2+, Mg2+, K+, and Na+) and texture at Soil, Water and Plant Analytical Laboratory of Makerere University following procedures outlined in Okalebo et al. (46). Soil pH was measured in a soil-water solution at a ratio of 1:2.5 (w/v) using a pH meter (Mettler-Toledo, AG 8603) after mixing on a rotary shaker for 30 minutes at 150 rpm (47). Total N was assessed after wet digestion of air-dried soil samples with a mixture of concentrated sulphuric acid (H2SO4) and selenium powder and salicylic acid and measured using a spectrophotometer (Jenway, 6405 UV/Vis) (48). Available P was extracted using Bray 1 method in a mixture of ammonium fluoride and hydrochloric acid, shaken for 1 minute at 150 rpm. The available P was complexed in a mixture of ascorbic acid and ammonium molybdate (49) and measured using a spectrophotometer (Jenway, 6405 UV/Vis). Exchangeable bases were extracted with ammonium acetate by shaking soil samples in ammonium solution for 20 minutes and measured using a flame-photometer (K+, Na+) (Jenway, Essex CM6 3LB) and atomic absorption spectrophotometer (Ca2+, Mg2+) (50) (Jenway, 6405 UV/Vis). Soil organic carbon was determined by wet oxidation with potassium dichromate under concentrated sulphuric acid at 150 °C for 30 minutes. The unreacted dichromate was titrated against standardized ferrous ammonium sulphate solution using ferroin indicator to determine the end point (51). Soil texture was determined using a Bouyoucos (Gallenkamp Bouyoucos) method (52).
2.4 Spore isolation and morphological identification of arbuscular mycorrhizal fungi genera
Arbuscular mycorrhizal fungi spores were isolated according to Jenkins (53) procedure with modifications by Ingleby (54) from 50 g of air-dried soil samples by wet sieving through 710 and 45 µm sieves, followed by sucrose gradient centrifugation. After centrifugation, spores and spore clusters were transferred into Petri dishes. The spores were distinguished into genera under the reflected light on stereomicroscope with the color of spore, spore size, hyphal attachments on spore and surface appearance of spore used as the diagnostic features and enumerated. Slide specimens were prepared for each AMF genus and further described under a compound microscope at magnification x40 with spore germination characteristics, spore wall characteristics, type of spore wall, number of layers and reaction to PVLG- Polyvinyl lacto glycerin (1.66g polyvinyl alcohol 20-25 cP, 10 ml lactic acid, glycerin 1 ml and 10 ml distilled H2O) and Melzer’s reagent (chloral hydrate, 1.5 g iodine, 5.0 g potassium iodide and 100 ml distilled H2O + PVLG). The spores were matched with genera described by International Culture Collection of VA Mycorrhizal Fungi (INVAM) West Virginia University Morgantown, WV, USA Website and Schenck and Perez (55, 56).
2.5 Data analysis
Analysis of Variance (ANOVA) using statistical analysis software (SAS), version 9.4 generalized linear model was used to determine the effects of AEZ and CT on AMF spore abundance. Spore abundance data were transformed in excel using square root pi (SQRTPI) the most preferred type of transformation for count data, to obtain symmetric distribution. Agro-ecological zone and CT were treated as fixed effects while fields were the random effects using the generalized linear model. Treatment means were separated using the least significant difference (LSD). Redundancy analysis (RDA) (57), the canonical version of principal component analysis (PCA), was used to examine the multiple correlations between soil properties (pH, OM and total N, available P, exchangeable Ca, K, Mg, and Na, sand, silt, and clay content) and genera of AMF in the sampling sites. The abundance values of AMF genera were centered and standardized in RDA using square root transformation. Soil properties were also standardized as explanatory variables using arcsine square root transformation before performing RDA. The AMF abundance data were pre-analyzed by Detrended Correspondence Analysis (DCA) using CANOCO software 4.5 (Micro-computer Power, Ithaca, NY) to choose a linear or unimodal ordination model for analysis. As the length of the gradient (first axis) was 0.668 below 3 by DCA, the RDA (canonical correlation analysis) was applied to the data obtained in this study.
3 Results
3.1 Arbuscular mycorrhizal fungi genera and spore abundance
In this study, six AMF genera were distinguished based on morphological features. Spore features that were used to classify the AMF genera included color of spore, spore size, hyphal attachments on the spore, and surface appearance of spore (INVAM and 55). Gigaspora species were identified as large spores with a bulbous hyphal attachment. Scutellospora species were identified as large spores with bulbous hyphal attachment, germination shield, and flexible/separating walls. Acaulospora species were identified as spores having ornamented spore walls, presence of cicatrix/cicatrices, and spores forming on the side of the hypha. Entrophospora species had almost similar characteristics to those of Acaulospora spp. But their spores form into the neck of the hypha, and Glomus spp. Have either curved, straight, or gourd-like hyphal attachment (Figure 2).
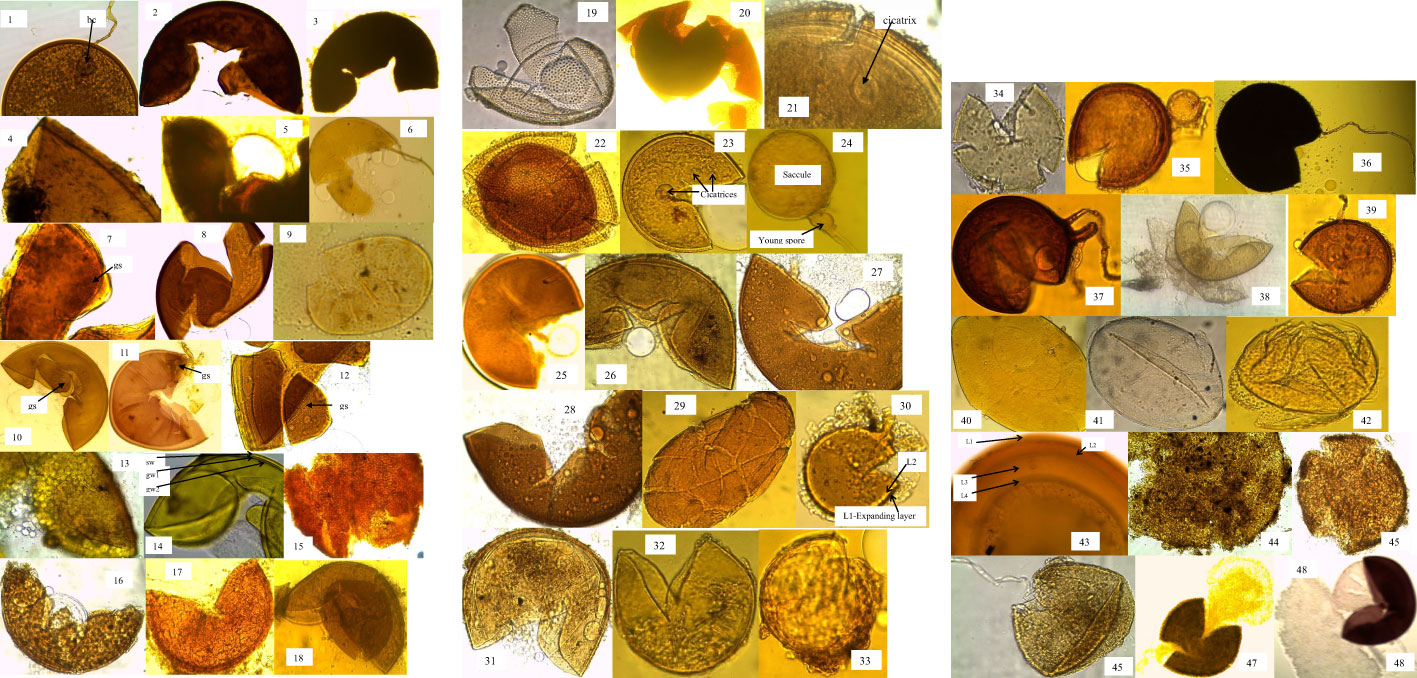
Figure 2 Some of the Glomeromycotan species identified from field soils across the AEZs and CTs. Magnification x40, bc, bulbous cell; gs, germination shield; sw, spore wall; gw, germination wall, l,layer, 1-6=Gigaspora spp., 7-12=Scutellospora spp., 13-20=Acaulospora spp., 21-25=Entrophospora spp., 26-39=Glomus spp., 40-48=Undefined spp.
Exploratory data analysis using box plots showed a variable range of distribution of spores within AEZs and CTs. The box represents the spore abundance range, the rhombus inside the box represents the mean of the spore abundance data, and the middle line represents the median, the lower and the upper bar mean the minimum and the maximum values of the data, respectively. Mean spore abundance across AEZs ranged between 48 and 58 spores while across CTs it ranged between 47 and 60 spores as shown by the medians of the box plots. Spore abundance was positively skewed across AEZs particularly in Mt. Elgon High Farmlands AEZ and in sweet potato fields because their medians are closer to the lower quartile, signifying non-normal distribution. The highest spore abundance was recorded in Southern and Eastern Lake Kyoga Basin AEZ, and in fallow fields since they had the highest mean values. The widest range of spore abundance was observed in Lake Victoria Crescent AEZ, and in legume fields since they had the longest whiskers (Figures 3A, B).
Using a generalized linear model for the presence and absence of AMF, the occurrence of AMF showed a slight variation in each AEZ and CT with two AMF genera significantly affected. The AEZs differently affected the mean spore abundance of Entrophospora spp. (p < 0.05) with SEKLB having a significantly (p < 0.05) higher number of spores than MEHF. The mean spore abundance of Gigaspora spp. (p < 0.05) was variable across the CTs with AMF spores dominating in fallow fields than the rest of the CTs (Table 3). T-test accepted the null hypothesis that total spore abundance between CTs in all AEZs was equal except for sorghum and sweet potato (t value=31.28; p=0.0010), sorghum and fallow (t value=0.00; p<.0001) and sweet potato and fallow (t value=9.28; p=0.0114) in MEHF and, sorghum and fallow (t value=0.00; p<.0001) in LVC.
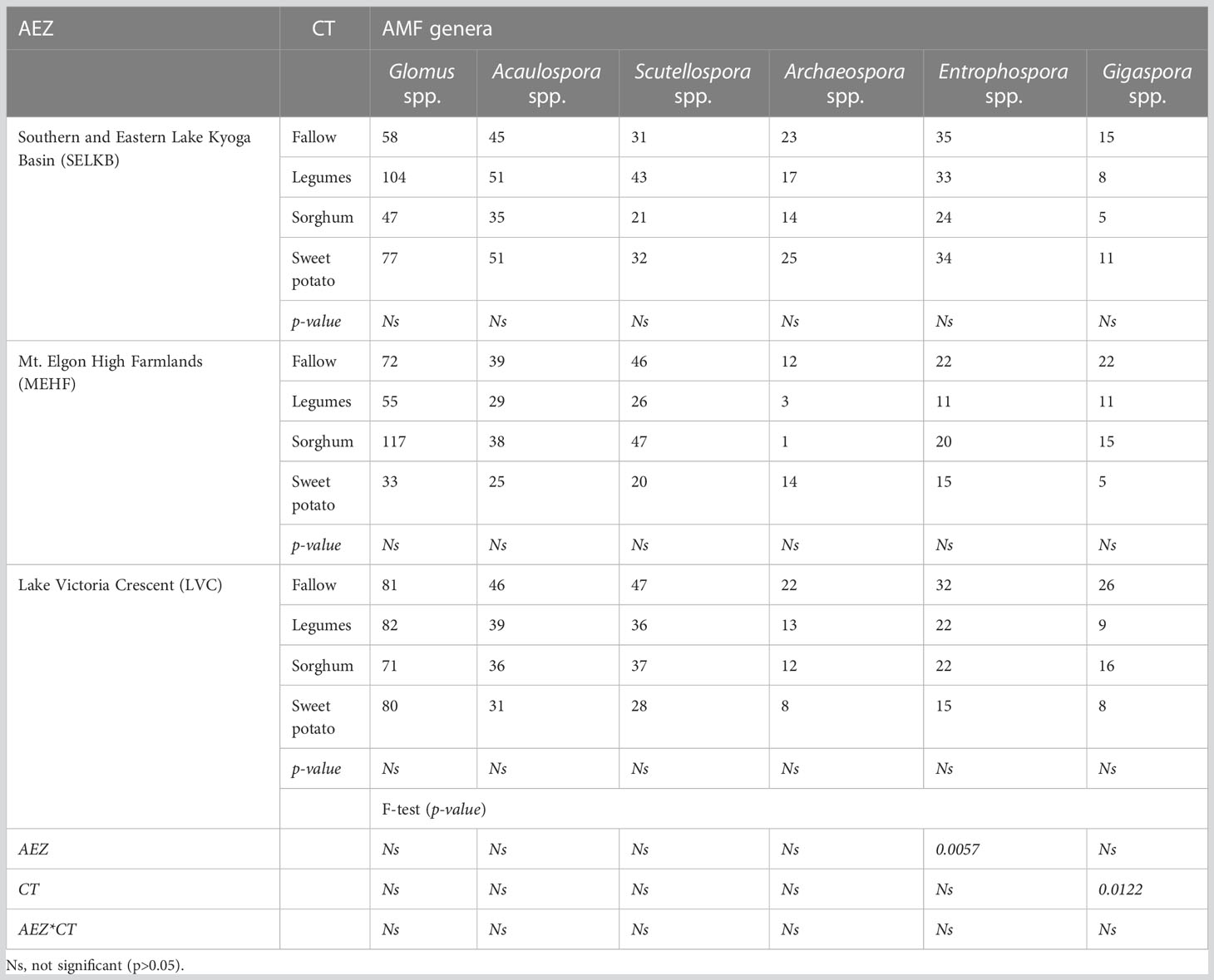
Table 3 Mean spore abundance of arbuscular mycorrhizal fungi in 50 g−1 air-dried soil across AEZs and CTs.
The frequency of observation of the identified AMF genera was 100% except that of Archaeospora which was 86%. The dominant AMF genera across the different AEZs were Glomus spp., Acaulospora spp., Scutellospora spp., and Entrophospora spp., each constituting ≥ 10% of the spore abundance of the identified AMF. They accounted for 87% of the spores of the identified AMF in each AEZ while Gigaspora spp. And Archaeospora spp. Contributed only 13% of the spores. The total number of the AMF genera spores across AEZs was highest in Glomus followed by Acaulospora, Scutellospora, Entrophospora, Archaeospora, and Gigaspora except for MEHF AEZ where Gigaspora was more dominant than Archaeospora (Table 4).
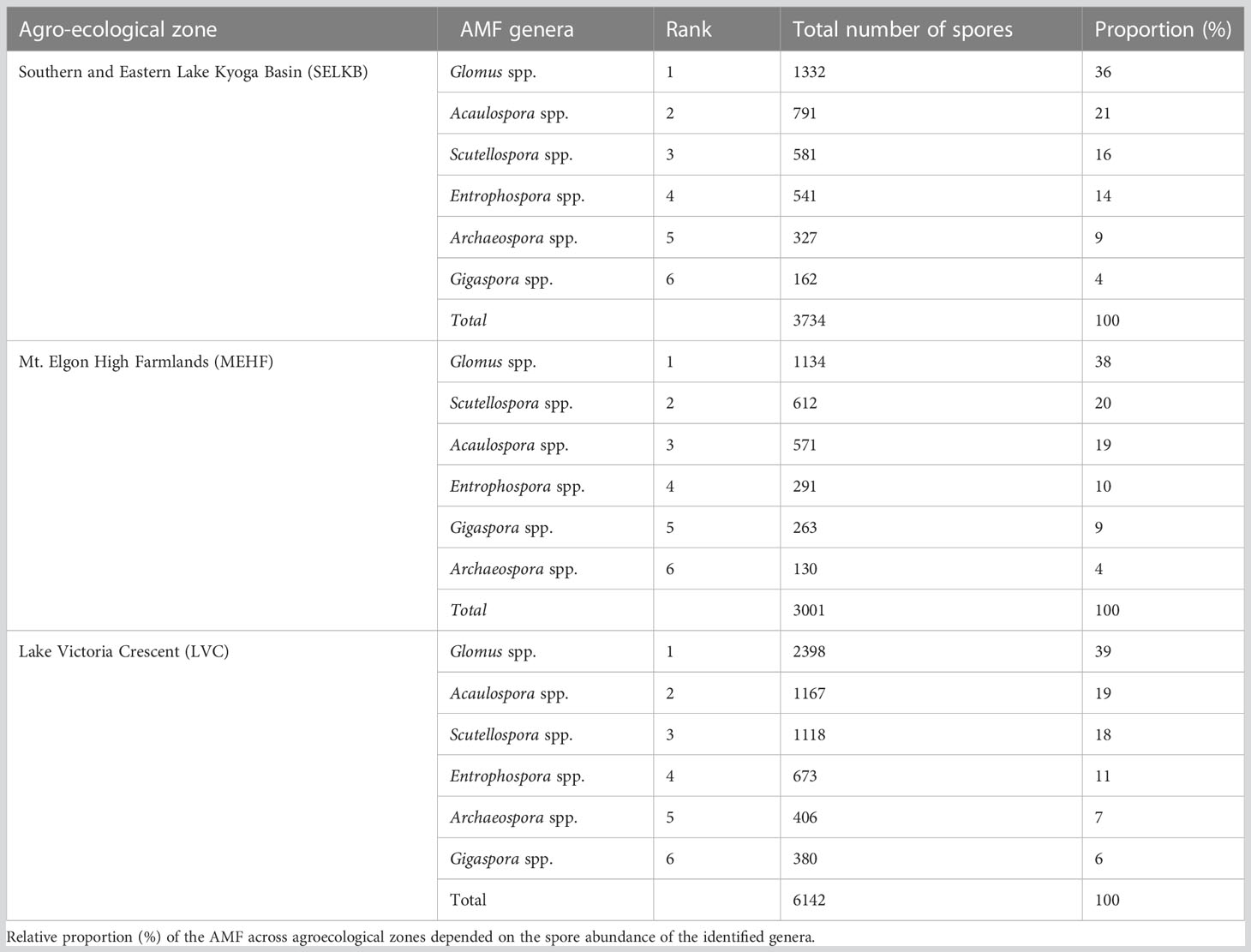
Table 4 Rank of total number of arbuscular mycorrhizal fungi spores per genera and their proportions in 50 g−1 air dried soil.
3.2 Correlation of soil properties and distribution of arbuscular mycorrhizal fungi
The soils in this study were weakly acidic but with low to moderate nutrient and OM levels. The mean values of measured soil properties were significantly (p ≤ 0.05) different across AEZs except for P and Na. Crop types significantly (p ≤ 0.05) influenced all soil properties except for pH, Ca, and Mg. The effect of the interaction of AEZs and CTs was only significant (p ≤ 0.05) on P, Na, Ca, and Mg (Table 5).
The first and the second RDA axis explained variance for 71 and 18%, respectively (Figure 4). According to the lengths of the arrows and the angles among them, OM, N, and pH had a strong positive correlation with the sporulation of Archaeospora, Entrophospora, Glomus and Scutellospora, and strong negative correlation with the sporulation of Acaulosopra and Gigaspora. Clay, K, Ca, and P had slight positive effects on the sporulation of Archaeospora, Entrophospora, Glomus and Scutellospora, and slight negative effects on the sporulation of Acaulospora and Gigaspora, because the arrows representing them are relatively short. Sand also had slight positive effects on the sporulation of Acaulospora, Archaeospora and Entrophospora, and slight negative effects on the sporulation of Glomus, Scutellospora and Gigaspora. Based upon the direction of the arrows, silt and Mg had a strong positive correlation with the sporulation of Acaulospora, Gigaspora, Scutellospora and Glomus, and a strong negative correlation with the sporulation of Archaeospora and Entrophospora. Sodium had a strong positive correlation with the sporulation of Glomus, Scutellospora and Gigaspora, and a strong negative correlation with the sporulation of Acaulospora, Archaeospora and Entrophospora.
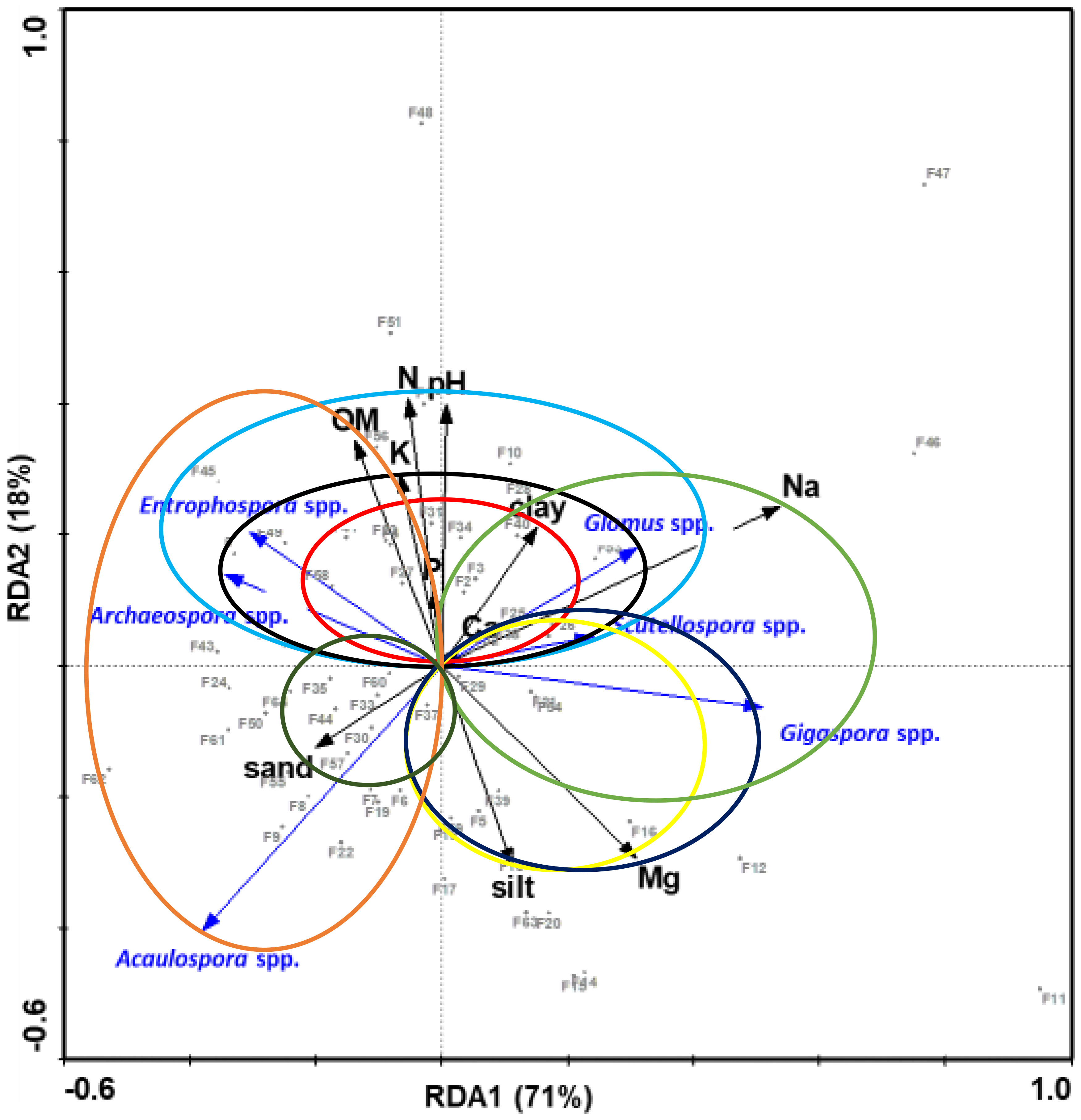
Figure 4 RDA (canonical correlation analysis) biplot of the 6 arbuscular mycorrhizal fungi genera (Glomus, Scutellospora, Gigaspora, Acaulospora, Archaeospora and Entrophospora) and soil properties from the sampled fields. The circle’s diameter is equal to the length of the soil property’s arrow. Genera lines that end in that circle have a positive regression for that soil property. The length of the arrow indicates increasing influence and the smaller the angle between the two arrows indicates closer relationships.
4 Discussion
The high spore abundance of Glomus spp. And Acaulospora spp. Across AEZs and CTs may be attributed to their high frequency of hyphal fusions that plug into compatible extraradical networks and hence immediate access to host plants and subsequent spore formation (58). Glomus and Acaulospora produce more spores in a shorter time than Scutellospora and Gigaspora in the same environment (23, 35, 36). Gigasporaceae species produce large spores (260 to 440 μm for Gigaspora margarita) that require a longer developmental period than small spores (59). Hence, their occurrence can be further ascertained through the study of AMF diversity in the root systems since spore extraction alone may miss species that may have not sporulated. The insignificant response of AMF genera except for Entrophospora to changes in AEZs could be due to quite similar soil properties attributed to similar agronomic practices carried out in the fields (Table 3). The total and mean spore abundance in this study (involving annual crops) were high compared to soils dominated by perennial crops and frequently supplied with inorganic fertilizers and pesticides (23, 34). The high spore abundance can be attributed to increased sporulation caused by frequent soil disturbance in annual crops fields. However, trap cultures were necessary to observe the composition of AMF as evidenced by several authors who reported fewer species from direct isolation from local soil and more species after trapping (26).
Crop type did not significantly affect AMF due to the similar conventional tillage carried out in the fields with the different crops (Table 3). The slight significant difference between fallow and sweet potato in MEHF and LVC was because fallows had been rested for an average of 6 months allowing for the recolonization and sporulation of AMF. Results showed that Gigaspora spp. Abundance was highest in the fallow fields (Table 3) which confirmed the results of Gai et al. (60) that Gigasporaceae spp. Are more often associated with wild plants than open fields. It also confirmed that the 6 months of fallowing was enough time for the development of their large spores (59).
The varying effects of sodium and magnesium on AMF genera in the present study (Figure 4) may be attributed to varying adaptability of AMF genera to varying levels of exchangeable bases (61). The positive effect of nitrogen on the spore abundance of Glomus, Scutellospora, Entrophospora, and Archaeospora might have been indirect through the increased supply of photosynthates from the host-crop to the fungi. Availability of soil N increases mycorrhizal activity (62) and hence its positive effect on the AMF genera.
It was expected that sporulation of all AMF genera is positively affected by organic matter since it is considered a source of energy for the growth and functioning of the fungi. However, the effect of organic matter on AMF growth and sporulation depends on the efficiency of the individual species in acquiring resources from the organic matter (63). Ng et al. (64) also reported that the chemical nature of soil carbon drives the structure and functioning of soil microbial communities which may vary from one AMF type to another. For example, pure cellulose obtained after proper decomposition increases asymbiotic AMF extraradical hyphae growth and root colonization (18, 65).
Soil texture has been reported to alter colonization of AMF (8, 66) depending on the sporulation patterns. The general soil textural classes in the present study were sandy clay loam and sandy loam which are reported to favor mycorrhizal development (67). Gigasporaceae (Gigaspora spp. And Scutellospora spp.) dominate in sandy soils (68) and they are also indicators of soils with lower clay content (69). Glomaraceae and other AMF families with small spores do not show any strong dependence on soil characteristics (70). However, Lekberg et al. (68) also reported that Scutellospora cerradensis (Sc. Rtl) was the only member of Gigasporaceae that occurred predominantly in clayey soil (containing 41:11:48 of clay, silt, and sand). From the same study they reported that Glomaraceae colonized roots well in sandy soil (containing 3:7:90 of clay, silt, and sand), clayey soil (containing 41:11:48 of clay, silt, and sand) and sand/clay mixture (containing 4:1 v/v). There’s a possibility that the difference in biomass allocation and the growth patterns of extraradical hyphae of Gigasporaceae and Glomaraceae are affected by soil texture (71, 72).
Most importantly, the soil pH was within a favorable range (5.85-6.64) for fungi and supported sporulation of especially, Glomus, Scutellospora, Entrophospora, and Archaeospora, and negatively affected the sporulation of Acaulospora and Gigaspora. Earlier, Muchane et al. (28) reported that AMF were favored by pH 5.51 to 6.67, while Dobo et al. (73) reported pH 6.18 to 6.28 to increase AMF sporulation in agricultural soils. However, it was expected that Acaulospora spp. Would be positively affected by the present pH since Acaulosporaceae are tolerant to acidic tropical soils (26, 74) and they highly sporulate under conventional tillage (a common practice in the sampled sites) as compared to other AMF families (31). For example, Acaulospora laevis is predominat in low pH soils and germinates well at pH 4-5 while Gigaspora heterogama isolated from warm climates and maintained in tropical areas showed varying germination rates (8 to 78%) in the same environmental conditions (75).
The six AMF genera identified in this study were present in all the AEZs and CTs but differences in their spore production were not significant. The AMF genera observed in this study were lower than 12 isolated in Sudan (76), 15 in Ethiopia (24), 15 in Southern China (77), 9 in Ethiopia (73) but higher than the 4 to 5 genera isolated in Kenya (23, 28, 34) and 4 in Rwanda (78). This may be due to variances in edaphic conditions and the cropping systems of the study sites. However, Glomus, Acaulospora, Scutellospora, and Gigaspora reported in this study were also observed in the studies carried out in the Sub-Saharan Africa (SSA) countries mentioned above. Incidentally, there were no new AMF genera identified, which alludes to low composition in these AEZs with the mean spore abundance of only Entrophospora spp. In SEKLB being significantly higher than in MEHF.
Most of the commercial mycorrhizal bioinoculants available in SSA are imported, expensive, and majorly contain Glomus species. During efficacy testing of these strains, the environmental conditions of the origin of the strains and where the bioinoculant is to be used may not be compared (79) yet it is important for the adaptability of AMF in the different local SSA edaphic and climatic conditions (80). The population of the introduced strains must build up for their increased competitiveness and effectiveness. In Tchabi et al. (81), AMF from Tropical Africa (Glomus hoi, Acaulospora spinosa, Glomus mosseae, Glomus etunicatum, and Acaulospora scrobiculata) averagely led to increased yam tuber growth by 51, 49, 38, 38 and 31%, respectively. Whereas exotic species from Europe were less efficient except for the three isolates of G. clarum isolates which increased tuber yield by 8.8, 15.6 and 55.6%, respectively compared to non-mycorrhizal control. Additionally, soil (from yam field) increased tuber yield by 40, 33, and 20% compared to exotic G. constrictum, the non-mycorrhizal control, and the exotic G. luteum confirming the existence of superior native AMF species in yam producing regions. Therefore, to overcome the problem of local adaptability of imported species and cost implications, mycorrhizal bioinoculants can be produced locally from the native species observed across different locations in SSA. The physiological characteristics of the species determine to a greater extent their survival and activity in the soil. Hence, different species will show varying responses, in terms of survival and activity. The ability of AMF to enhance root surface area by hyphal growth and provide an extra route for uptake as mycorrhizal pathway (82) depends on the AMF strains colonizing the plant roots. The efficiency of AMF strains is influenced differently by their development and activity of the external hyphae, hyphal transport rates, and solute interchange at the arbuscule-host root cell interface (83, 84). Therefore, the mixing of different compatible AMF strains during bioinoculant production will promote the complementary benefits of AMF strains in crop production.
Plant-host generalist AMF strains should be considered for bioinoculant production as compared to plant-host specialist AMF strains since during fallowing the latter can colonize weeds or new crops which eventually can serve as a source of additional spores (85). The compatibility of the sporogenous Glomus and Acaulospora species with different crops and environmental conditions require further testing for suitable local bioinoculant production. However, Acaulospora spp. Would greatly influence crop production in acidic tropical soils since the Acaulosporaceae species are tolerant to acidic soils (26, 74); highly effective in P-uptake and transfer to the host plant compared to Glomeraceae species (86); and they highly sporulate under conventional tillage (a common practice in sweet potato producing areas) as compared to other AMF families (31).
Glomus and Acaulospora species can be further identified using molecular techniques and their compatibility with different crops and environmental conditions in varying dosage tested for suitable local bioinoculant production. To reduce the cost of soil fertility amending inputs in crop production, bioinoculants containing the most effective species can be integrated with reduced rates of inorganic fertilizers.
5 Conclusion
A total of six AMF genera comprising of Glomus, Acaulospora, Scutellospora, Entrophospora, Archaeospora, and Gigaspora were isolated from the study sites. The composition and spore abundance of AMF recorded in the AEZs and CTs in the major sweet potato growing areas had limited significant differences due to the similar agricultural practices employed by farmers. The effect of soil parameters on AMF spore abundance varied from genera to genera, however, the strongest influence was by OC, N, pH, silt, Mg and Na. The most dominant AMF i.e., Glomus and Acaulospora species can be isolated for local bioinoculant production.
Data availability statement
The original contributions presented in the study are included in the article/supplementary materials. Further inquiries can be directed to the corresponding author.
Author contributions
RM conceived the research idea and conducted the research, collected, and analyzed the data, and co-wrote the manuscript; JT, PE, and CM assisted with conceiving the research and critically reviewed the manuscript. All authors contributed to the article and approved the submitter version.
Funding
This work was supported, in whole, by the Bill and Melinda Gates Foundation (Grant Number OPPGD1398). Under the grant conditions of the Foundation, a Creative Commons Attribution 4.0 Generic License has already been assigned to the Author Accepted Manuscript version that might arise from this submission. The work was done under the commercial products II (COMPRO II) project through a sub-agreement between the International Institute of Tropical Agriculture (IITA) and Makerere University. We are grateful to Makerere University for providing laboratory space and technical support.
Conflict of interest
The authors declare that the research was conducted in the absence of any commercial or financial relationships that could be construed as a potential competing interest.
Publisher’s note
All claims expressed in this article are solely those of the authors and do not necessarily represent those of their affiliated organizations, or those of the publisher, the editors and the reviewers. Any product that may be evaluated in this article, or claim that may be made by its manufacturer, is not guaranteed or endorsed by the publisher.
References
2. Schüβler A, Geherig H, Schwarzott D, Walker C. Analysis of partial glomales SSU rRNA gene sequences: Implications for primers design and phylogeny. Mycol. Res (2001) 105:5–15. doi: 10.1017/S0953756200003725
3. Spatafora JW, Chang Y, Benny GL, Lazarus K, Smith ME, Barbie ML, et al. A phylum-level phylogenetic classification of zygomycete fungi based on genome-scale data. Mycologia (2016) 108(5):1028–46. doi: 10.3852/16-042
4. Martiny JBH, Bohannan BJM, Brown JH, Colwell RK, Fuhrman JA, Green JL, et al. Microbial biogeography: putting microorganisms on the map. Nature (2006) 4:102–12. doi: 10.1038/nrmicro1341
5. Ramette A, Tiedje JM. Biogeography: an emerging cornerstone for understanding prokaryotic diversity, ecology, and evolution. Microb Ecol (2007) 53:197–207. doi: 10.1007/s00248-005-5010-2
6. Hazard C, Gosling P, van der Gast CJ, Mitchell DT, Doohan FM, Bending GD. The role of local environment and geographical distance in determining community composition of arbuscular mycorrhizal fungi at the landscape scale. ISMEJ (2013) 7:498–508. doi: 10.1038/ismej.2012.127
7. Kumar D, Kumar R, Anal AKD. Spore production, colonization, species diversity and factors influencing the association of arbuscular mycorrhizal fungi with litchi trees in India. J Environ Biol (2016) 37:91–100.
8. Zaller JG, Frank T, Drapela T. Soil sand content can alter effects of different taxa of mycorrhizal fungi on plant biomass production of grassland species. Eur J Soil Biol (2011) 47:175–81. doi: 10.1016/j.ejsobi.2011.03.001
9. Alguacil MDM, Torres MP, Montesinos-Navarro A, Roldán A. Soil characteristics driving arbuscular mycorrhizal fungal communities in semiarid Mediterranean soils. Appl Environ Microbiol (2016) 82:3348–56. doi: 10.1128/AEM.03982-15
10. Wilson H, Johnson BR, Bohannan B, Pfeifer-Meister L, Mueller R, Bridgham SD. Experimental warming decreases arbuscular mycorrhizal fungal colonization in prairie plants along a Mediterranean climate gradient. PeerJ (2016) 4:e2083. doi: 10.7717/peerj.2083
11. Zhang B, Chang SX, Anyia AO. Mycorrhizal inoculation and nitrogen fertilization affect the physiology and growth of spring wheat under two contrasting water regimes. Plant Soil (2016) 398(1-2):47–57. doi: 10.1007/s11104-015-2635-x
12. Gutjahr C. Phytohormone signaling in arbuscular mycorrhiza development. Curr Opin Plant Biol (2014) 20:26–34. doi: 10.1016/j.pbi.2014.04.003
13. Verbruggen E, Röling WFM, Gamper HA, Kowalchuk GA, Verhoef HA, van der Heijden MGA. Positive effects of organic farming on below-ground mutualists: large-scale comparison of mycorrhizal fungal communities in agricultural soils. New Phytol (2010) 186:968–79. doi: 10.1111/j.1469-8137.2010.03230.x
14. Karasawa T, Takebe M. Temporal or spatial arrangements of cover crops to promote arbuscular mycorrhizal colonization and p uptake of upland crops grown after non-mycorrhizal crops. Plant Soil (2011) 353:355–66. doi: 10.1007/s11104-011-1036-z
15. Schnoor TK, Lekberg Y, Rosendahl S, Olsson PA. Mechanical soil disturbance as a determinant of arbuscular mycorrhizal fungal communities in semi-natural grassland. Mycorrhiza (2011) 21:211–20. doi: 10.1007/s00572-010-0325-3
16. Zhang B, Li Y, Tusheng R, Tian Z, Wang G, He X, et al. Short-term effect of tillage and crop rotation on microbial community structure and enzyme activities of a clay loam soil. Biol Fertil. Soils (2014) 50(7):1077–85. doi: 10.1007/s00374-014-0929-4
17. Öpik M, Moora M, Liira J, Zobel M. Composition of root-colonizing arbuscular mycorrhizal fungal communities in different ecosystems around the globe. J Ecol (2006) 94:778–90. doi: 10.1111/j.1365-2745.2006.01136.x
18. Albertsen A, Ravnskov S, Green H, Jensen DF, Larsen J. Interactions between the external mycelium of the mycorrhizal fungus Glomus intraradices and other soil microorganisms as affected by organic matter. Soil Biol Biochem (2006) 38:1008–14. doi: 10.1016/j.soilbio.2005.08.015
19. Gamalero E, Martinnoti MG, Trotta A, Lemanceau P, Berta G. Morphogenetic modifications induced by pseudomonas fluorescens A6RI and glomus mosseae BEG12 in the root system of tomato differ according to plant growth conditions. New Phytol (2004) 155:293–300. doi: 10.1046/j.1469-8137.2002.00460.x
20. Artursson V, Finlay RD, Jansson JK. Interactions between arbuscular mycorrhizal fungi and bacteria and their potential for stimulating plant growth. Environ Microbiol (2006) 8:1–10. doi: 10.1111/j.1462-2920.2005.00942.x
21. Alguacil MM, Torres MP, Torrecillas E, Diaz G, Roldán A. Plant types differently promote the arbuscular mycorrhizal fungi biodiversity in the rhizosphere after revegetation of a degraded, semiarid land. Soil Biol Biochem (2011) 43:167–73. doi: 10.1016/j.soilbio.2010.09.029
22. Martínez-García L, Pugnaire FI. Arbuscular mycorrhizal fungi host preference and site effects in two plant species in a semiarid environment. Appl Soil Ecol (2011) 48:313–7. doi: 10.1016/j.apsoil.2011.04.003
23. Jefwa JM, Okoth S, Wachira P, Karanja N, Kahindi J, Njuguini S, et al. Impact of cropping systems and farming practices on occurrence of arbuscular mycorrhizal fungi (AMF) taita-taveta district in Kenya. Agricult. Ecosyst. Environ (2012) 157:32–9. doi: 10.1016/j.agee.2012.04.009
24. Belay Z, Vestberg M, Assefa F. Diversity and abundance of arbuscular mycorrhizal fungi across different cropping systems in a humid lowland of Ethiopia. Trop Subtropical Agroecosyst. (2015) 18:47–69.
25. Mbogne JT, Temegne CN, Hougnandan P, Youmbi E, Tonfack LB, Ntsomboh-Ntsefong G. Biodiversity of arbuscular mycorrhizal fungi of pumpkins (Cucurbita spp.) under the influence of fertilizers in ferralitic soils of Cameroon and Benin. J Appl Biol Biotechnol (2015) 3(05):001–10. doi: 10.7324/jabb.2015.3501
26. Temegne NC, Wakem G-A, Taffouo DV, Mbogne TJ, Onguene AN, Youmbi E, et al. Effect of phosphorus fertilization on arbuscular mycorrhizal fungi in the bambara groundnut rhizosphere. Afr J Microbiol Res (2017) 11(37):1399–410. doi: 10.5897/ajmr2017.8680
27. Jefwa JM, Vanlauwe B, Coyne D, van Asten P, Gaidashova S, Rurangwa E, et al. Benefits and potential use of arbuscular mycorrhizal fungi (AMF). In: Dubois T, Hauser S, Staver C, Coyne D, editors. Proc. IC on banana and plantain in Africa. Acta Hort: International Society of Horticultural Sciences (2010). p. 479–86.
28. Muchane MN, Muchane M, Mugoya C, Masiga CW. Effect of land use system on arbuscular mycorrhiza fungi in maasai Mara ecosystem, Kenya. Afr J Microbiol Res (2012) 6:3904–16. doi: 10.5897/AJMR12.155
29. Mathimaran N, Ruh R, Jamab B, Verchot L, Frossard E, Jansa J. Impact of agricultural management on arbuscular mycorrhizal fungal communities in Kenyan ferralsol. Agricult. Ecosyst Environ (2007) 119(1–2):22–32. doi: 10.1016/j.agee.2006.06.004
30. Gai JP, Liu RJ. Effects of soil factors on AMF in the rhizosphere of wild plants. Chin J Appl Ecol (2003) 14:18–22.
31. Castillo CG, Borie F, Godoy R, Rubio R, Sieverding E. Diversity of mycorrhizal plant species and arbuscular mycorrhizal fungi in evergreen forest, deciduous forest and grassland ecosystems of southern Chile. J Appl Bot Food Qual (2006) 80:40–7.
32. Jansa J, Mozafar A, Anken T, Ruh R, Sanders IR, Frossard E. Diversity and structure of AMF communities as affected by tillage in a temperate soil. Mycorrhiza (2002) 12:225–34. doi: 10.1007/s00572-002-0163-z
33. Schalamuk S, Velázquez S, Chidichimo H, Cabello M. Fungal spore diversity of arbuscular mycorrhizal fungi associated with spring wheat: effects of tillage. Mycologia (2006) 98:22–8. doi: 10.1080/15572536.2006.11832708
34. Jefwa JM, Mung’atu J, Okoth P, Muya E, Roimen H, Njuguini S. Influence of cropping systems on occurrence of arbuscular mycorrhiza fungi in the high altitude regions of mt. Kenya. Trop Subtropical Agroecosyst. (2009) 11:277–90.
35. Bever JD, Morton JB, Antonovics J, Schultz PA. Host-dependent sporulation and species diversity of arbuscular mycorrhizal fungi in mown grassland. J Ecol (1996) 84:71–82. doi: 10.2307/2261701
36. Oehl F, Sieverding E, Ineichen K, Mäder P, Wiemken A, Boller T. Distinct sporulation dynamics of arbuscular mycorrhizal fungal communities from different agroecosystems in long term microcosms. Agricult. Ecosyst Environ (2009) 134:257–68. doi: 10.1016/j.agee.2009.07.008
37. Voko D-R R.B., Nandjui J. Sery J-MD, Fotso B, Amoa AJ, Kouadio M-SA, Coulibaly S, et al. Abundance and diversity of arbuscular mycorrhizal fungal (AMF) communities associated with cassava (Manihot esculenta crantz) rhizosphere in abengourou, East côte d’Ivoire. J Ecol Natural Environ (2013) 5(11):360–70. doi: 10.5897/jene2013.0407
38. Straker CJ, Hilditch AJ, Rey MEC. Arbuscular mycorrhizal fungi associated with cassava (Manihot esculenta crantz) in south Africa. South Afr. J Bot (2010) 76:102–11. doi: 10.1016/j.sajb.2009.09.005
39. Uganda Bureau of Statistics-Ministry of Agriculture, Animal Industry and Fisheries (UBOS-MAAIF). Uganda Census of agriculture 2008/2009. volume IV. Uganda: Crop Area and Production Report (2009).
40. Wortmann CS, Eledu CA. Uganda`s agroecological zones: a guide for planners and policy makers. Kampala, Uganda: Centro Internacional de Agricultura Tropical (1999).
41. Ebregt E, Struik PC, Abidin PE, Odongo B. Farmers’ information on sweet potato production and millipede infestation in north-eastern uganda. i. associations between spatial and temporal crop diversity and the level of pest infestation. NJAS-Wagen J Life Sc (2004) 52(1):47–68.
42. Kayuki CK, Mohammed BM, Maman N. Fertilizer use optimization: Principles and approach. In: Wortmann CS, Sones K, editors. Fertilizer use optimization in Sub-Saharan Africa. Nairobi, Kenya: CAB International (2017).
43. Sserumaga JP, Biruma M, Akwero A, Okori P, Edema R. Prevalence of sorghum anthracnose in different agroecologies of Uganda. Uganda J Agric Sci (2013) 14:125–35. doi: 10.4314/UJAS.V14I1
44. Chombo O, Lwasa S, Makooma TM. Spatial differentiation of small holder farmers’ vulnerability to climate change in the kyoga plains of Uganda. Am J Climate Change (2018) 7:624–48. doi: 10.4236/ajcc.2018.74039
45. Tchabi A, Coyne D, Hountondji F, Lawouin L, Wiemken A, Oehl F. Arbuscular mycorrhizal fungal communities in sub-Saharan savannas of Benin, West Africa, as affected by agricultural land use intensity and ecological zone. Mycorrhiza (2008) 18:181–95. doi: 10.1007/s00572-008-0171-8
46. Okalebo JR, Gathua KW, Woomer PL. Laboratory methods for soil and plant analysis. In: A working manual. second edition. tropical soil fertility and biology program, Nairobi Kenya. Nairobi Kenya: TSBF-CIAT and SACRED Africa (2002). p. 128.
47. Rhoades JD. In methods of soil analysis, part 2. 2nd ed. Page AL, Miller RH, Keeney DR, editors. Madison USA: American Society of Agronomy (1982).
48. Anderson JM, Ingram JSI. TSBF: A handbook of methods of analysis. Wallingford: CAB International (1989) p. 38–9.
49. Anderson JM, Ingram JSI. TSBF: A handbook of methods of analysis. Wallingford: CAB International (1989). p. 39.
50. Anderson JM, Ingram JSI. Tropical soil biology and fertility: A handbook of methods. Wallingford, UK: CAB International (1993). p35.
51. Anderson JM, Ingram JSI. Tropical soil biology and fertility: A handbook of methods. Wallingford, UK: CAB International (1993). p37.
52. Bouyoucos GJ. Hydrometer method improved for making particle size analyses of soils. Agron Journals (1962) 53:464–5. doi: 10.2134/agronj1962.00021962005400050028x
53. Jenkins WR. A rapid centrifugal flotation technique for separating nematodes from soil. Plant Dis Res (1964) 48:692. doi: 10.1007/s10333
54. Ingleby K. Assessment of mycorrhizal diversity in soils and roots, and nursery inoculation to improve the survival and growth of seedlings. Mycorrhizal Training Manual (2007) 1–43.
55. Schenck NC, Perez Y. Manual for the identification of VA mycorrhizal fungi. 3rd edn. Gainesville, Fla: Synergistic publications (1990).
56. INVAM. International culture collection of (Vesicular) arbuscular mycorrhizal fungi. Morgantown, West Virginia: West Virginia University (2016). Available at: http://invam.wvu.edu/the-fungi/species-descriptions.
57. Lepš J, Šmilauer P. Multivariate analysis of ecological data using CANOCO. Cambridge, United Kingdom: Cambridge University Press (2003).
58. Sbrana C, Fortuna P, Giovannetti M. Plugging into the network: Belowground connections between germlings and extraradical mycelium of arbuscular mycorrhizal fungi. Mycologia (2011) 103:307–16. doi: 10.3852/10-125
59. Hepper CM. Isolation and culture of VA mycorrhizal (VAM) fungi. In: VA Mycorrhiza (Powell CL, Bagyaraj DJ, eds): (1984) 95–112. Boca Raton, FL: CRC Press.
60. Gai JP, Christie P, Feng G, Li XL. Twenty years of research on community composition and species distribution of arbuscular mycorrhizal fungi in china. a review. Mycorrhiza (2006) 16:229–39. doi: 10.1007/s00572-005-0023-8
61. Bothe H. Arbuscular mycorrhiza and salt tolerance of plants. Symbiosis (2012) 58:7–16. doi: 10.1007/s13199-012-0196-9
62. Borie F, Rubio R, Morales A. Arbuscular mycorrhizal fungi and soil aggregation. J Soil. Sci Plant Nutr (2008) 8:9–18. doi: 10.4067/S0718-27912008000200003
63. Zhu XC, Song FB, Liu SQ, Liu FL. Arbuscular mycorrhiza improve growth, nitrogen uptake, and nitrogen use efficiency in wheat grown under elevated CO2. Mycorrhiza (2016) 26:133–40. doi: 10.1007/s00572-015-0654-3
64. Ng EL, Patti AF, Rose MT, Schefe C, Wilkinson K, Smernik RJ, et al. Does the chemical nature of soil carbon drive the structure and functioning of soil microbial communities? Soil Biol Biochem (2014) 70:54–61. doi: 10.1016/j.soilbio.2013.12.004
65. Gryndler M, Vosátka M, Hrselová H, Chvátalová I, Jansa J. Interaction between arbuscular mycorrhizal fungi and cellulose in growth substrate. Appl Soil Ecol (2002) 19:279–88. doi: 10.1016/S0929-1393(02)00004-5
66. Nadian H, Smith SE, Alston AM, Murray RS, Siebert BD. Effects of soil compaction on phosphorus uptake and growth of Trifolium subterraneum colonized by four species of vesicular–arbuscular mycorrhizal fungi. New Phytol (1998) 140:155–65. doi: 10.1046/j.1469-8137.1998.00219.x
67. Carrenho R, Trufem SFB, Bononi VLR, Silva ES. The effect of different soil properties on arbuscular mycorrhizal colonisation of peanuts, sorghum and maize. Acta Botanica brasilica (2007) 21(3):723–30. doi: 10.1590/S0102-33062007000300018
68. Lekberg YRT, Koide R, Rohr JR, Aldrich-Wolfe L, Morton JB. Role of niche restrictions and dispersal in the composition of arbuscular mycorrhizal fungal communities. J Ecol (2007) 95(1):95–105. doi: 10.1111/j.1365-2745.2006.01193.x
69. Vieira LC, da Silva DKA, Escobar IEC, da Silva JM, de Moura IA, Oehl F, et al. Changes in an arbuscular mycorrhizal fungi community along an environmental gradient. Plants (2020) 9:52. doi: 10.3390/plants9010052
70. Duponnois R, Plenchette C, Thioulouse J, Cadet P. The mycorrhizal infectivity and arbuscular mycorrhizal fungal spore communities in soils of different aged fallows in Senegal. Appl Soil Ecol (2001) 17:239–51. doi: 10.1016/S0929-1393(01)00132-9
71. Boddington CL, Dodd JC. The effect of agricultural practices on the development of indigenous arbuscular mycorrhizal fungi. II. studies in experimental microcosms. Plant Soil (2000) 218:145–157.äs. doi: 10.1023/A:1014911318284
72. Hart MM, Reader RJ. Taxonomic basis for variation in the colonization strategy of arbuscular mycorrhizal fungi. New Phytol (2002) 153:335–44. doi: 10.1046/j.0028-646X.2001.00312.x
73. Dobo B, Asefa F, Asfaw Z. Diversity of arbuscular mycorrhizal fungi of different plant species grown in three cropping systems in wensho and shebidino districts of sidama in southern Ethiopia. Adv Biosci. Bioeng. (2016) 4(4):25–34. doi: 10.11648/j.abb.20160404.11
74. Bagyaraj DJ. Ecology of arbuscular mycorrhizal fungi. In: Kharwar RN, Upadhyay R, Dubey N, Raghuwanski R, editors. Microbial diversity and biotechnology in food security. India: SpringerLink (2014). p. 133–46.
75. de Novais CB, Sbrana C, Júnior OJS, Siqueira JO, Giovannetti M. Vegetative compatibility and anastomosis formation within and among individual germlings of tropical isolates of arbuscular mycorrhizal fungi (Glomeromycota). Mycorrhiza (2013) 23:325–31. doi: 10.1007/s00572-013-0478-y
76. Abdelhalim TS, Finckh MR, Babiker AG, Oehl F. Species composition and diversity of arbuscular mycorrhizal fungi in white Nile state, central Sudan. Arch Agron Soil Sci (2013) 60:377–91. doi: 10.1080/03650340.2013.793453
77. Wang C, Gu Z, Cui H, Zhu H, Fu S, Yao Q. Differences in arbuscular mycorrhizal fungal community composition in soils of three cropping systems in subtropical hilly area of southern China. PloS One (2015) 10(6):e0130983. doi: 10.1371/journal.pone.0130983
78. Gaidashova SV, Van Asten PJA, Jefwa JM, Delvaux B, Declerck S. Arbuscular mycorrhizal fungi in the East African highland banana cropping systems as related to edapho-climatic conditions and management practices: Case study of Rwanda. Fungal Ecol (2010) 3:225–33. doi: 10.1016/j.funeco.2009.09.002
79. Enkhtuya B, Raydlová J, Vostáka M. Effectiveness of indigenous and non-indigenous isolates of arbuscular mycorrhizal fungi from soils with degraded ecosystems and man-made habitats. Appl Soil Ecol (2000) 14:201–11. doi: 10.1016/S0929-1393(00)00057-3
80. Mukhongo RW, Tumuhairwe JB, Ebanyat P, Abdelgadir AH, Thuita M, Masso C. Production and use of arbuscular mycorrhizal fungi inoculum in Sub-Saharan Africa: Challenges and ways of improving. Int J Soil Sci (2016) 11(3):108–22. doi: 10.3923/ijss.2016.108.122
81. Tchabi A, Coyne D, Hountondji F, Lawouin L, Wiemken A, Oehl F. Efficacy of indigenous arbuscular mycorrhizal fungi for promoting white yam (Dioscorea rotundata) growth in West Africa. Appl Soil Ecol (2010) 45:92–100. doi: 10.1016/j.apsoil.2010.03.001
82. Smith FW, Mudge SR, Rae AL, Glassop D. Phosphate transport in plants. Plant Soil (2003) 248:71–83. doi: 10.1023/A:1022376332180
84. Hajiboland R, Aliasgharzad N, Barzeghar R. Influence of arbuscular mycorrhizal fungi on uptake of zn and p by two contrasting rice genotypes. Plant Soil Environ (2009) 55(3):93–100. doi: 10.17221/319-PSE
85. Öpik M, Moora M. Missing nodes and links in mycorrhizal networks. New Phytol (2012) 194:304–6. doi: 10.1111/j.1469-8137.2012.04121.x
Keywords: arbuscular mycorrhiza, composition, spore abundance, agroecological zone, cropping type
Citation: Mukhongo RW, Ebanyat P, Masso C and Tumuhairwe JB (2023) Composition and spore abundance of arbuscular mycorrhizal fungi in sweet potato producing areas in Uganda. Front. Soil Sci. 3:1152524. doi: 10.3389/fsoil.2023.1152524
Received: 27 January 2023; Accepted: 06 March 2023;
Published: 20 March 2023.
Edited by:
Marika Pellegrini, University of L’Aquila, ItalyReviewed by:
Mahaveer P. Sharma, ICAR Indian Institute of Soybean Research, IndiaVlad Stoian, University of Agricultural Sciences and Veterinary Medicine of Cluj-Napoca, Romania
Copyright © 2023 Mukhongo, Ebanyat, Masso and Tumuhairwe. This is an open-access article distributed under the terms of the Creative Commons Attribution License (CC BY). The use, distribution or reproduction in other forums is permitted, provided the original author(s) and the copyright owner(s) are credited and that the original publication in this journal is cited, in accordance with accepted academic practice. No use, distribution or reproduction is permitted which does not comply with these terms.
*Correspondence: Ruth Wilhem Mukhongo, ruthwilhemm@gmail.com