- 1College of Land Resources and Environment, Jiangxi Agricultural University, Nanchang, China
- 2Key Innovation Center of Agricultural Waste Resource Utilization and Non-point Source Pollution Prevention and Control of Jiangxi Province, Nanchang, China
Recently, the excessive propagation of water hyacinth has led to serious ecological and environmental problems; thereby, its treatment and disposal are of great significance. Moreover, the remediation of heavy metals in soil is a hot topic at present. Thus, water hyacinth was adopted to prepare biochar to investigate its effect on Cd accumulation in lettuce by pot experiments in this study. The optimal application amount of water hyacinth biochar was 1% (30 t ha−1), considering the Cd absorption and yield of lettuce plants. Compared with those of control, the application of biochar prepared at 700°C for 2 h with an amount of 3% (90 t ha−1) resulted in a reduction in Cd by 73.6% and 38.1%, respectively, in the shoots and roots of lettuce. Within a certain carbonization time (0.5~2 h) and carbonization temperature (300°C~700°C), the content of available Cd in the soil decreases with the increase of the carbonization temperature and time, which might be the main reason for the lower Cd concentration in lettuce after applying the biochar. Furthermore, scanning electron microscopy (SEM) and energy-dispersive X-ray fluorescence spectroscopy (EDS) analyses showed that Cd was fixed on the biochar in a state of passivation, leading to a sharp decrease in the available Cd in the soil. Moreover, it was concluded that the application of biochar brings with it an obvious increase in the enzyme activity increment in the soil up to 2.3 times. Lastly, 16sRNA sequencing has shown that biochar addition leads to variations in microbial structure and abundance in soil. Accordingly, biochar prepared by water hyacinth can increase lettuce yield and reduce the concentration of heavy metals in lettuce.
Introduction
Biochar as an adsorbent has been proven to be an effective method to treat heavy metal-contaminated soils (1, 2). Compared with other remediation techniques for contaminated soil, biochar has the advantages of economy, efficiency, and ease of implementation. Biochar is a kind of aromatic carbon material with high carbon content, a complex pore structure, and a large specific surface area produced by pyrolysis of some biomass undergoing complete or partial anoxic conditions (3, 4). Previous studies have shown that biochar application on arable land can promote soil and water conservation, reduce soil nutrient loss and greenhouse gas emissions, and repair heavy metal polluted soil (5). Zhang etal. (6) proved a significant reduction at 39.3% of Cd content in the rice grains by sludge biochar application. Nie et al. (1) applied biochar prepared from waste bagasse to soil to significantly reduce the content of heavy metals (Cu, Pb, and Cd) in Brassica. Studies have shown that heavy metals in soil can combine with a large number of functional groups on the surface of biochar to form stable covalently bonded compounds. Tan etal. (7) proved that the heavy metals in the soil could be adsorbed through the complexation of metal ions with different functionalities on both the outer and inner surfaces of the biochar. In addition, heavy metals may exchange with some metal cations in biochar or react with oxygen-containing functional groups in biochar (8, 9). Whatever the case, the mobility of heavy metals can also be reduced by the use of biochar in the soil by altering the redox state. However, the effectiveness of soil remediation by biochar was associated with the specifications of biochar, such as its surface area, metal content, alkalinity, etc. These performances could vary significantly with the change in biomass type. Salam etal. (2) reported that the application of rice straw and rapeseed residue biochar substantially decreased the Pb concentration in roots and shoots of Chinese cabbage by 40.81% and 30.26%, respectively. Obviously, biochar prepared from different raw materials has great differences in its adsorption performance for heavy metals, mainly because the performance of biochar is closely related to the properties of raw materials, such as the influence of oxygen-containing functional groups, specific surface areas, and aromatic compounds. Therefore, it is of great significance to find suitable raw materials for biochar preparation to improve the heavy metal adsorption performance in heavy metal-contaminated soil.
Water hyacinth, a native species of the Amazon Basin, is now seen as the most important troublesome aquatic plant worldwide. Excessive reproduction and growth of water hyacinth, which caused eco-environmental damages, have brought on social attention (10). Therefore, the management of water hyacinths is very important to maintain ecological balance. Water hyacinth biochar has recently shown potential for metal adsorption from industrial, agricultural, and household wastewater. Zhang etal. (6) evaluated biochar derived from water hyacinths, generated at four different pyrolysis temperatures, as a method for adsorbing Cd from an aqueous solution. In their study, maximum Cd removal (70 mg g−1) was achieved at a pyrolytic temperature of 450°C in a nitrogen gas environment because the water hyacinth contains a large amount of cellulose. Thus, the biochar made from water hyacinth has a large specific surface area, containing a large number of active functional groups and binding sites, which is the fundamental reason for its stronger adsorption capacity for heavy metals (11, 12). Although there are many reports on the adsorption of heavy metal ions in water by water hyacinth biochar, there has been little study focusing on the restoration of heavy metals that contaminated farmland. It was unknown whether water hyacinth biochar could reduce the uptake of heavy metals by crops grown in contaminated soil or not.
Thus, this study was designed to explore the effects of water hyacinth biochar on lettuce growth in Cd-contaminated soil. The investigation is divided into three parts: (1) determine the effect of water hyacinth biochar on lettuce growth and heavy metal accumulation within the plant; (2) explore the process and mechanism of biochar passivation of heavy metals in soil; and (3) evaluate the changes in enzyme and microbial activity induced by biochar in the heavy metal-contaminated soil.
Materials and methods
Preparation of water hyacinth biochar
The water hyacinth was taken from the Science and Technology Park of Jiangxi Agricultural University. The water hyacinth was washed two to three times with deionized water to remove the dirt on the surface. The washed water hyacinth was dried naturally until it had a certain toughness. It was then dried at 80°C until constant weight after being cut to 3–4 cm in length. The dried water hyacinth would be crushed and screened (100 mesh) and then placed into the dryer. The crushed water hyacinth wrapped within two to three layers of tinfoil was placed into a ceramic crucible. The pyrolysis temperatures were 300°C, 500°C, and 700°C with a heating rate of 10°C/min to carbonize at 0.5, 1, and 2 h in a nitrogen atmosphere. The biochar was taken from the tinfoil cool to room temperature. The biochar itself contains heavy metals that were removed by immersing them in 0.1 mg L−1 HCl for 12 h and then washed with ionic water until neutral. Finally, the biochar was obtained by placing them in an oven at 80°C for 24 h. Preliminary experimental results showed that the specific surface area and pore size of water hyacinth biochar were 0.935 m2/g and 31.2 nm, respectively, which were better than biochar prepared from other raw materials under the same preparation conditions, such as rice straw and corn straw.
Experimental design
The soil samples were crushed and screened through 10 meshes that lay flat on plastic film. Later, they were removed after being sun dried. A certain concentration of Cd solution was prepared and sprayed into the soil to make the Cd concentration in the soil reach 5 mg kg−1. The soil was then kept stable for 8 weeks while being stirred to ensure that the Cd was evenly mixed in the soil. In total, 20, 40, and 60 g of water hyacinth biochar (1%, 30 t ha−1; 2%, 60 t ha−1; 3%, 90 t ha−1) were added and intensively mixed with the 2-kg of Cd-contaminated soil. The biochar amount was translated in terms of tons per hectare reported by Ebido etal. (13). Following this, the mixture was placed into the plastic flowerpot that kept the soil in the flooded state (about 3 cm water layer). Eventually, five lettuce seeds were sown in the pot and two plants were set in each container after maintaining the soil water content in the field capacity of 60%. Meanwhile, compound fertilizers equivalent to 60 kg ha−1 of N, 60 kg ha−1 of P2O5, and 60 kg ha−1 of K2O were also incorporated with topsoil.
Plant sample collection and fresh weight measurement
Lettuce plants were harvested after 40 days of growth, and the yield of lettuce in each flowerpot was measured by weighing all of the plant materials, including leaf blades and petioles (without roots). After transporting samples to the laboratory, plants were separated into roots and shoots and then rinsed with deionized water to remove surface soil. Thereafter, the samples were drained with filter paper before the plant and roots’ fresh weight measurements were carried out. Later, the samples were crushed and Cd detection pretreatment was conducted, in which process the shoot and roots were baked at 75°C to a constant weight within 30 min at 105°C.
Laboratory analyses
The available Cd in soil was extracted using the diethylenetriamine pentaacetic acid (DTPA) reagent. Atomic absorption spectrophotometry (ICE 3300, Perkin Elmer, USA) was used to detect Cd in the shoot, roots, and soil. Activities of soil urease and catalase were measured using the Solarbio activity detection kit. The enzyme activities were calculated in accordance with the instructions. Urease activity was defined as 1 µgNH3-N/g soil sample/day as an enzyme activity unit (U/g soil). Catalytic degradation of 1 µmol of H2O2/g of air-dried soil sample/day was defined as a catalase enzyme activity unit (U/g soil). The surface morphology of the corroded surface of each specimen was examined using the TESCAN VEGA3 scanning electron microscope (SEM). The chemical compositions were analyzed using AZtec X-MaxN80 energy-dispersive X-ray fluorescence spectroscopy (EDS).
Biochar characterization
The surface morphology of the samples was determined using SEM (JSM-6400, JEOL, Japan) equipped with an EDS (Link ISIS, Oxford Instruments) for analyzing surface elements. The functional groups on the surface of the adsorbent were analyzed by an IRPrestige-21 transform infrared spectrometer (Thermo Fisher Scientific, USA). The surface organic functional groups were determined by Fourier transform infrared (FTIR) spectroscopy (Nicolette is 50, Thermo Fourier, USA).
Analysis of soil microbial community abundance
The samples were sent to Novogene Company for 16sRNA sequencing. The final data were obtained from a series of processed and comparative analyses in line with the database. OTU clustering and species classification analyses were carried out to obtain the analysis results for each sample. The species composition was then analyzed based on the results of all the analyses to determine the differences between different samples.
Statistical analysis
Statistical analyses were performed through the SPSS 18.0 statistical package program (SPSS Institute, USA). One-way analysis of variance (ANOVA) and Turkey’s test were used to assess the statistical differences between the treatments. The level of significance was set at p< 0.05.
Results and discussion
Effect of water hyacinth biochar on the Cd contents of lettuce shoots
The effects of water hyacinth biochar application at different gradients on the Cd contents of lettuce shoots in Cd-contaminated soil were investigated. The application gradients of water hyacinth biochar were 0, 1%, 2%, and 3%, respectively. As shown in Figure 1A, when the application ratio of biochar prepared at 300°C for 0.5 h was 1%, the Cd content of the lettuce shoot was 0.72 mg kg−1 on day 40, which decreased by 10.0% compared to 0.80 mg kg−1 of the control. When the amount of water hyacinth biochar continued to increase to 2%, the Cd content of lettuce shoots decreased to 0.65 mg kg−1, 18.8%, and 9.7% lower than that of the control and 1% of biochar addition, respectively. The Cd content of lettuce shoots decreased significantly with the increase of the water hyacinth biochar application. The minimum Cd uptake of lettuce shoots appeared when the amount of water hyacinth biochar at 300°C for 0.5 h applied was 3%. The content was 0.43 mg kg−1, which was merely 53.8% of the Cd content of the control. Due to the passivation of biochar, most of the Cd in the soil was fixed on the biochar, reducing the uptake of Cd by lettuce shoots. The more biochar was applied, the less Cd the lettuce shoots absorb. However, the yield of lettuce was not positively correlated with the quantity of biochar applied. As shown in Supplementary Figure S1, when the biochar amount was 1%, the average fresh weight of each lettuce plant was 17.4 g after 40 days of cultivation, which increased by 34.9%, more than the 12.9 g of fresh weight of the control plant. The application of a certain amount of water hyacinth biochar was beneficial to the growth of lettuce plants. However, as the amount of water hyacinth biochar continued to increase, the yield of lettuce showed a trend of nonincreasing, even actually decreasing. While the biochar amount prepared at 300°C for 0.5 h increased to 2% and 3%, the average fresh weight of lettuce plants was only 17.1 and 15.0 g, respectively. Although the results were still higher than the control, they had decreased by 1.7% and 13.8% compared with the 1% biochar amount. In addition, if the preparation temperature and time of water hyacinth biochar were increased, the adsorption performance of biochar would be better. The application of 2% and 3% water hyacinth biochar with better adsorption performance leads to a greater yield reduction effect on lettuce. When the group with 3% biochar was prepared at 700°C for 2 h, the fresh weight of lettuce per plant was solely 4.4 g on day 40, which was 65.9% lower than that of the control. The possible reason for the yield reduction of lettuce caused by excessive application of biochar was that biochar retained soil nitrogen and reduced available nitrogen content in the soil. It indicated that the optimal application amount of water hyacinth biochar was 1%, considering the Cd absorption and yield of lettuce plants.
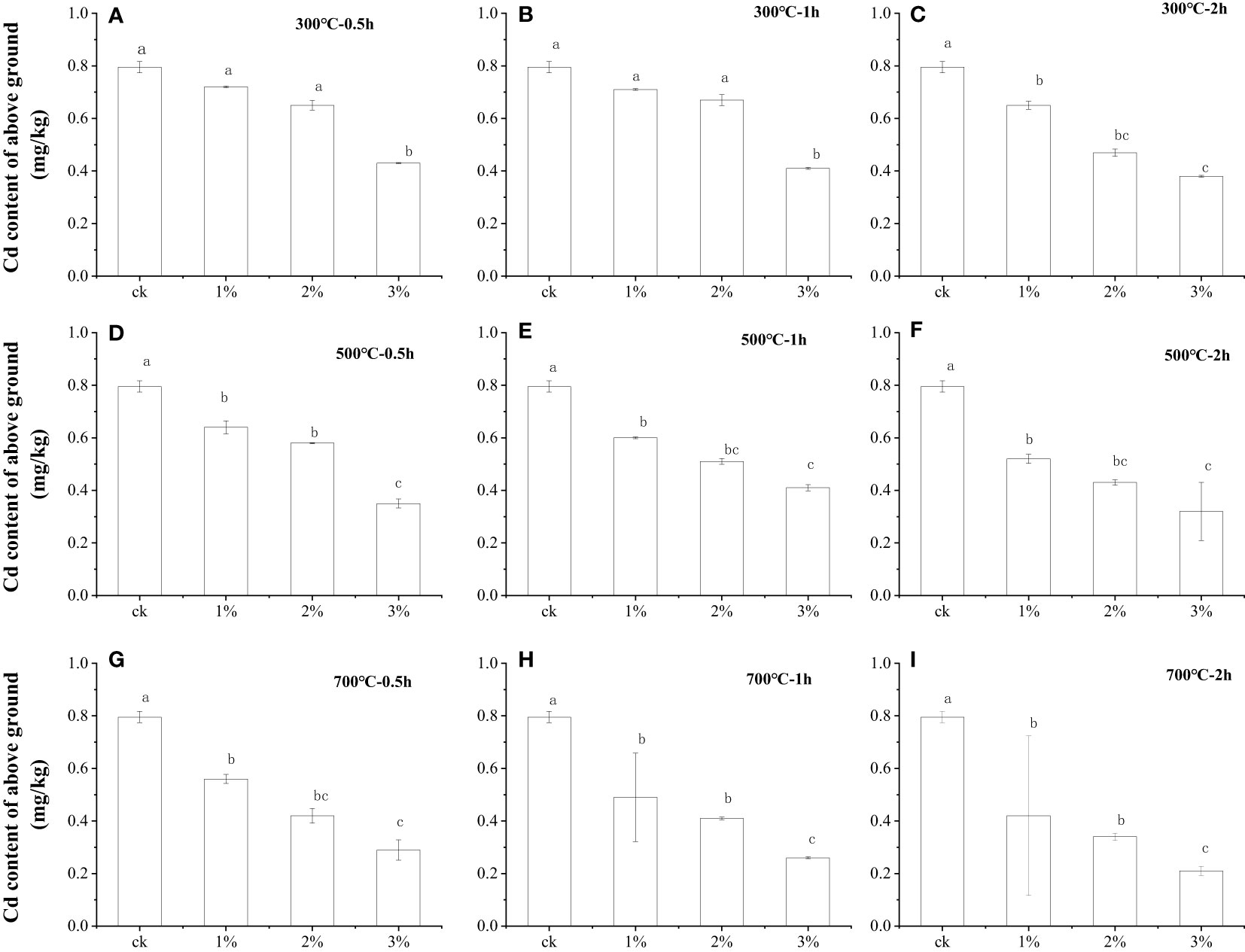
Figure 1 Comparison of the Cd content of lettuce shoot after water hyacinth biochar application with different preparation conditions. Small letters indicates significant differences.
Many studies have proved that the preparation temperature of biochar is an important factor that could affect the absorption of heavy metals (14, 15). The effect of the preparation temperature of water hyacinth biochar on lettuce Cd adsorption was also investigated. Figures 1A, D, G indicated that with 1% water hyacinth biochar application, prepared at 500°C within 0.5 h of carbonization, the heavy metal content of the lettuce shoots is 0.64 mg kg−1 on day 40. When compared to the control and biochar (300°C for 0.5 h) treatments, the heavy metal adsorption of lettuce shoots was reduced by 20.0% and 11.1%, respectively. The biochar prepared at a higher temperature has a better passivation effect in Cd-contaminated soil is shown in Figures 1B, C, E, F, H, I. The heavy metal content of lettuce shoot was only 0.56 mg kg−1 when 1% biochar with 700°C for 0.5 h preparation was applied, reduced by 44.0%, 22.2%, and 12.5% compared with the control and biochar addition application prepared at 300°C for 0.5 h and 500°C for 0.5 h, respectively. Moreover, the fresh weight of lettuce was reduced by 13.8% after applying a 1% ratio of water hyacinth biochar prepared at 700°C for 0.5 h compared with biochar prepared at 300°C for 0.5 h, but the yield of lettuce still increased by 16.6% compared to the control, as shown in Supplementary Figure S1G.
Effect of water hyacinth biochar on Cd contents of lettuce roots
The effects of application amount, preparation temperature, and carbonization time of water hyacinth biochar on the Cd uptake by lettuce roots were under investigation. Similar to the absorption of Cd in lettuce shoots, taking the application of 300°C for 0.5 h as an example, as the amount of biochar applied increases, the absorption of Cd in the roots of lettuce gradually decreases. As shown in Figure 2, when the amount of biochar prepared at 300°C for 0.5 h was 3%, the content of Cd in the roots of lettuce was 0.64 mg kg−1, which was 23.80% lower than the 0.84 mg kg−1 of the control. Compared to the application of 1% and 2%, it is also 21.0% and 20.0% higher. In particular, when comparing Figures 2A–C, it was obvious that the water hyacinth biochar with a longer carbonization time makes it more difficult for the roots of lettuce to absorb Cd from the soil. When all the test groups with a 3% applied amount of the water hyacinth biochar with a carbonization temperature and carbonization time of 300°C and 2 h, the content of Cd in the roots of lettuce was 0.53 mg kg−1. Compared with the application of the same amount of biochar prepared at 300°C for 0.5 h and 300°C for 1 h, the Cd concentration in the roots of lettuce decreased by 22.1% and 18.5%, respectively. The comparison of Figures 2A, D, G shows that water hyacinth biochar has different effects on root Cd absorption obtained at different carbonization temperatures. Also, taking the application amount of 3% biochar as an example, the application of water hyacinth biochar prepared at 700 for 2 h resulted in a Cd content of only 0.53 mg kg−1 in the roots of lettuce. The Cd content of the lettuce root system was reduced by 11.5% and 13.2%, relatively less accumulated than the data gained at a biochar preparation temperature of 300°C and 500°C. The experimental results show that increasing the amount of biochar applied or enhancing the adsorption performance of biochar by changing the carbonization time as well as the carbonization temperature are both effective methods to reduce the absorption of Cd in lettuce roots.
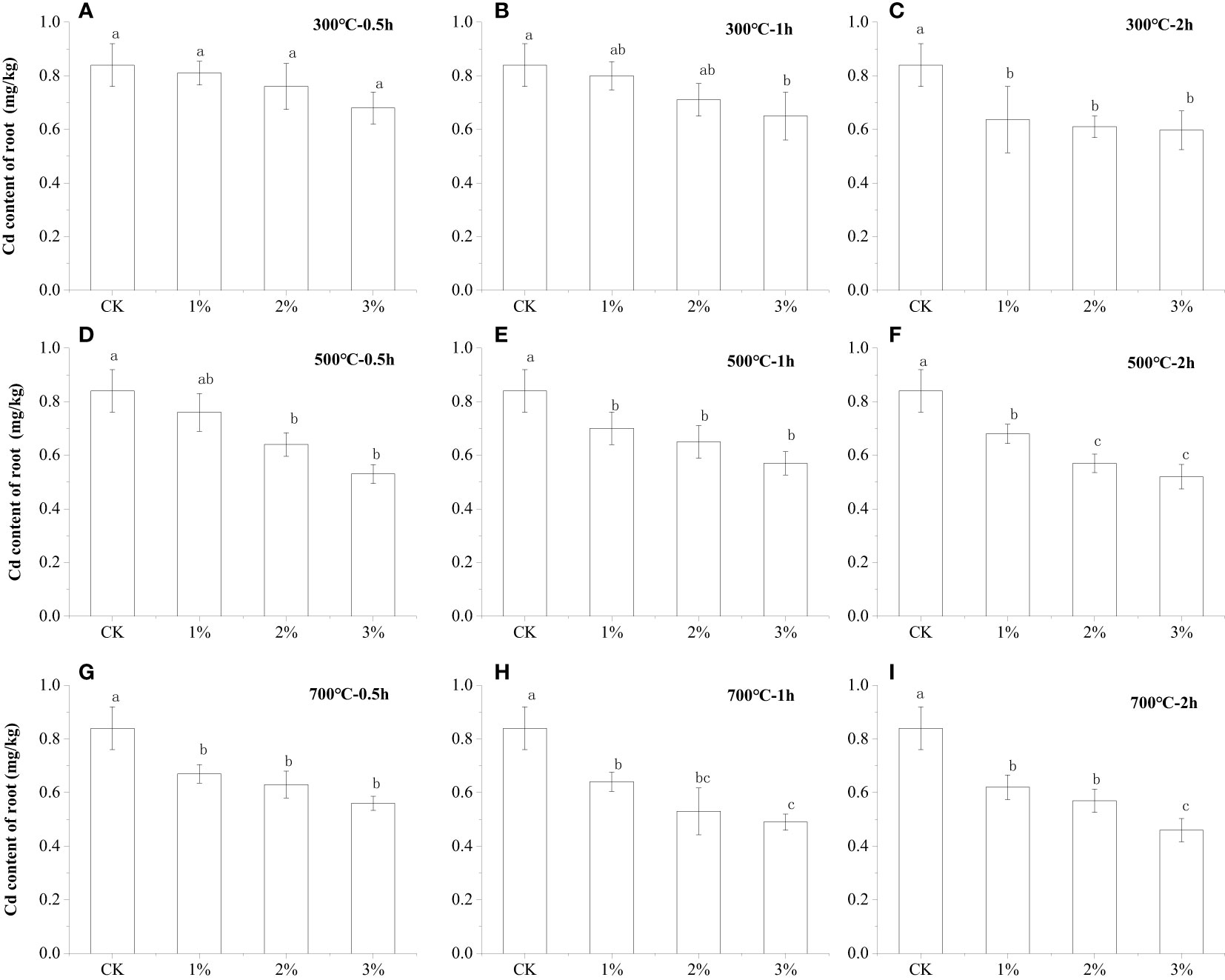
Figure 2 Comparison of the Cd content of lettuce root after water hyacinth biochar application with different preparation conditions. Small letters indicates significant differences.
Effect of water hyacinth biochar application on available Cd contents of soil
Many studies have revealed that the available Cd in the soil can explain its mobility and biological toxicity effects accurately (16, 17). In order to explore how water hyacinth biochar affects the Cd absorption of the lettuce in the soil, the available Cd concentration in the soil was determined at the end of the experiment. Turning to Figures 3A–I, with the amount of water hyacinth biochar beginning to rise, the available Cd in the soil showed a significant downward trend. When 1% biochar, which was prepared at 300°C for 0.5 h, was applied, the content of available Cd in the soil was 2.65 mg kg−1 at the end of this experiment, which was 6.4% lower than that of control at 2.83 mg kg−1. The available Cd in the soil decreased, and meanwhile, the amount of biochar increased. When the application dose of biochar (300°C for 0.5 h) was increased to 3%, the effective Cd in the soil decreased to 2.49 mg kg−1, which was 6.0% and 12.0% lower than that of the control and the treatments of 1% biochar under the same carbonization conditions. Similar to the previous lettuce shoot Cd tendency, the content of available Cd in the soil is also closely associated with the preparation conditions of biochar (carbonization temperature and carbonization time). When keeping the biochar input at 3%, the available Cd content was only 1.47 mg kg−1 in the soil after applying 700°C for 2 h biochar, which is 34.7% lower than that of preparation at 300°C for 2 h (2.25 mg kg−1), corresponding to only 51.6% of the control. Additionally, the soil available Cd content was 2.29 mg kg−1 after using biochar prepared at 700°C for 0.5 h, which was 55.8% higher than the soil available Cd in the soil with biochar application prepared at 700°C for 2 h. Evidently, within a certain carbonization time (0.5~2 h) and carbonization temperature (300°C~700°C), the amount of available Cd in the soil decreases with the increase of the carbonization temperature and the extension of the carbonization time. That might be the main reason for the lowest Cd concentration in lettuce shoots and roots after conducting the application of water hyacinth biochar at the carbonization condition of 700°C for 2 h.
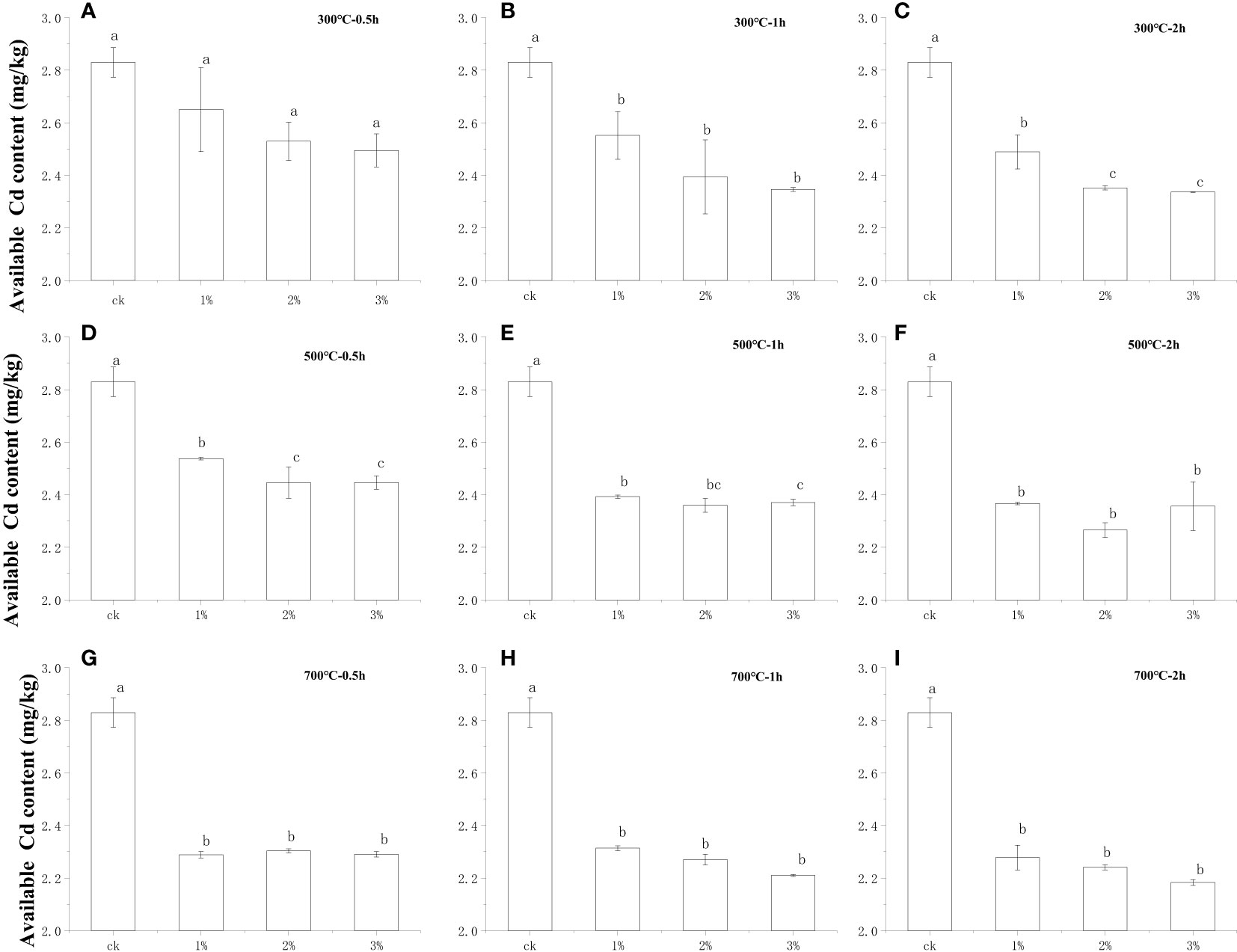
Figure 3 Comparison of available Cd concentration of soil by water hyacinth biochar application with different condition preparations. Small letters indicates significant differences.
Comparison of the surface structure of biochar before and after application
Figures 4A, B display the variation of the surface structure of the initial water hyacinth biochar and its application to soil on day 40 after the lettuce harvest. As shown in Figure 4A, the surface of water hyacinth biochar prepared is initially smooth and possesses a neat structure. It was indicated that the carbonization did not cause serious damage to the structure. After EDS analysis shown in Figure 4C, it was found that there were many kinds of elements such as Cl, Al, and Si on the surface of the water hyacinth biochar that was initially prepared, but the Cd element was not included. However, there were some holes that appeared in the surface structure due to the decomposition of the biochar after 40 days of stirring with the soil. Furthermore, many aggregates adhere to the surface of water hyacinth biochar after the application. EDS analysis of the aggregates suggested that there was not only a high abundance of Cd elements but also Fe, Mn, and other metal elements in the aggregates as well. FTIR analysis confirmed this speculation. Supplementary Figure S3 shows that the vibration of −OH at 3,440 cm−1 and the C=O functional groups at 1,708 cm−1 do not have an obvious displacement before and after biochar adsorption. However, after biochar was applied to soil, two distinct peaks appeared near wave numbers 490 and 550 cm−1, which might be the vibration of metal oxides, such as Mn–O, Fe–O, and Cd–O. Previous studies have shown that Mn, Fe, and other metal elements derived from soil could easily form some active functional groups such as Mn–O and Fe–O on the surface of biochar, which can easily combine with heavy metal Cd2+ to form stable complexes like Mn–O–Cd and Fe–O–Cd (18, 19). Owing to the strong effect of biochar adsorption and active functional groups after water hyacinth biochar was added to Cd-contaminated soil, Cd was fixed on the biochar, leading to a sharp decrease in its effective Cd in the soil. This is the underlying cause of lettuce roots and shoots reducing the absorption of Cd.
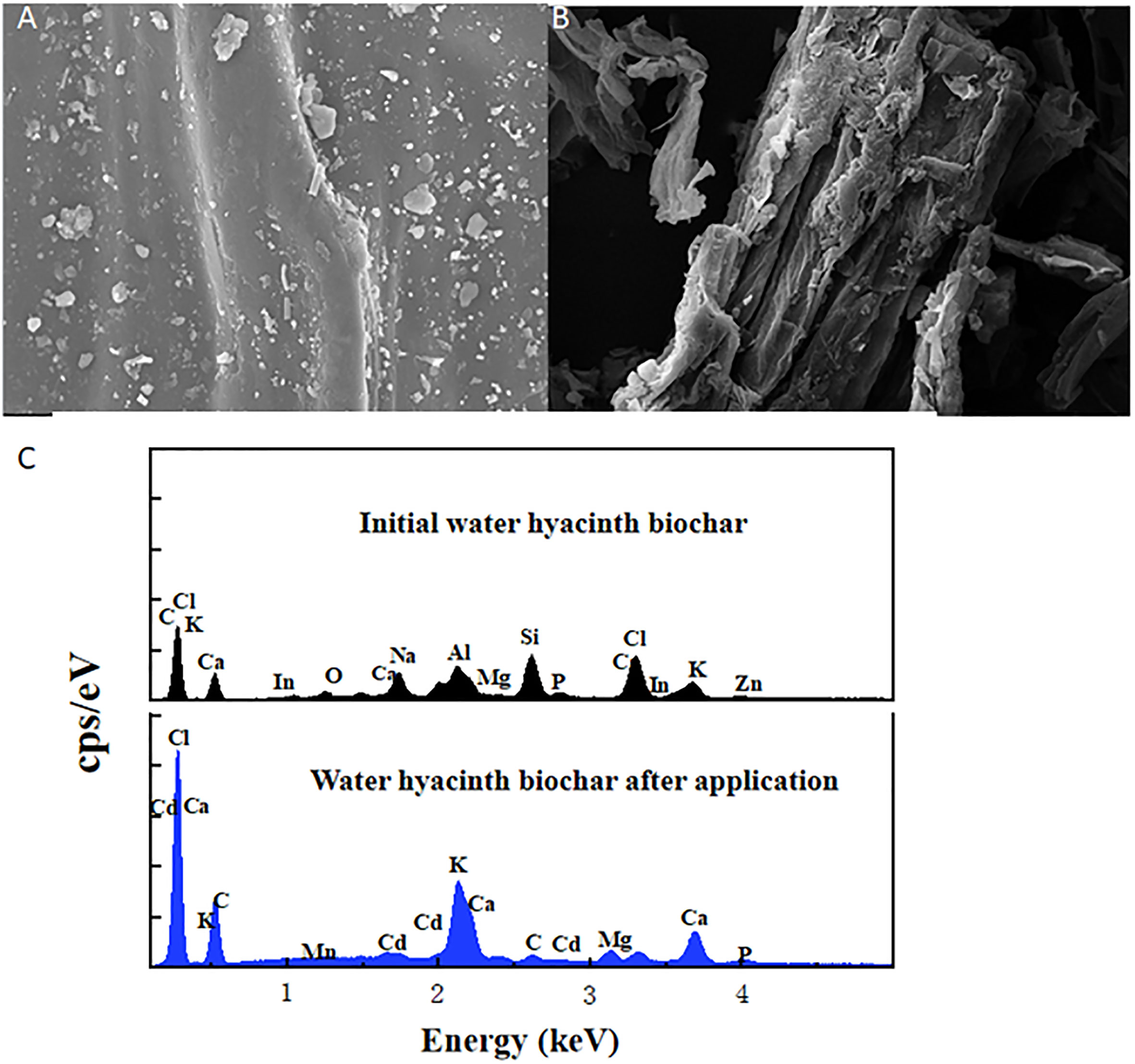
Figure 4 Comparison of the surface structure before and after application of water hyacinth biochar. (A) Initial water hyacinth biochar. (B) Biochar after being applied to soil on day 40. (C) EDS analysis of initial water hyacinth biochar and water hyacinth biochar after being applied to soil on day 40.
Effect of water hyacinth biochar application on soil enzyme activities
As an essential component of soil, soil enzymes acted as catalysts for various soil biochemical reactions and soil microbial activity, which were closely related to both the degradation and purification of soil pollutants. Its activities can reflect the intensity of soil biochemical reactions and their pollutant degradation ability (20). For this reason, the influences of water hyacinth biochar on urease and catalase activities in Cd-contaminated soil are presented in Figure 5. Soil enzyme activities significantly (p< 0.05) increased for all biochar treatments compared to the nonbiochar treatment. In the case of catalase, compared to the control with the activity of urease at 173.1 mg -N kg−1 h−1, soil amended with water hyacinth biochar prepared at 300°C for 0.5 h at application rates of 1%, increased the activity of urease by 42.3%. The activities of urease increased significantly with an increasing dose of biochar. Compared with the control, 2% and 3% biochar application resulted in urease enzyme activity in soil up to 291.6 and 301.0 mg -N kg−1 h−1, which increased by 68.5% and 73.9%, respectively. Additionally, the application of biochar with a better passivation effect of heavy metals could significantly promote the increase of soil enzyme activity. As mentioned above, with the increase in carbonization temperature and carbonization time, water hyacinth biochar has better performance in the passivation of heavy metals. In contrast, the amount of available heavy metals in soil showed an opposite trend to the activity of soil enzymes. When the application dose of water hyacinth biochar (700°C for 2 h) was 3%, the peak value of the urease enzyme activity in soil was up to 399.0 mg -N kg−1 h−1. It was 2.3 times that of the control group. As shown in Supplementary Figure S2, catalase activities in the soil showed the same tendency. Thus, it was concluded that the enzyme activities in the soil were enhanced significantly by the application of water hyacinth biochar.
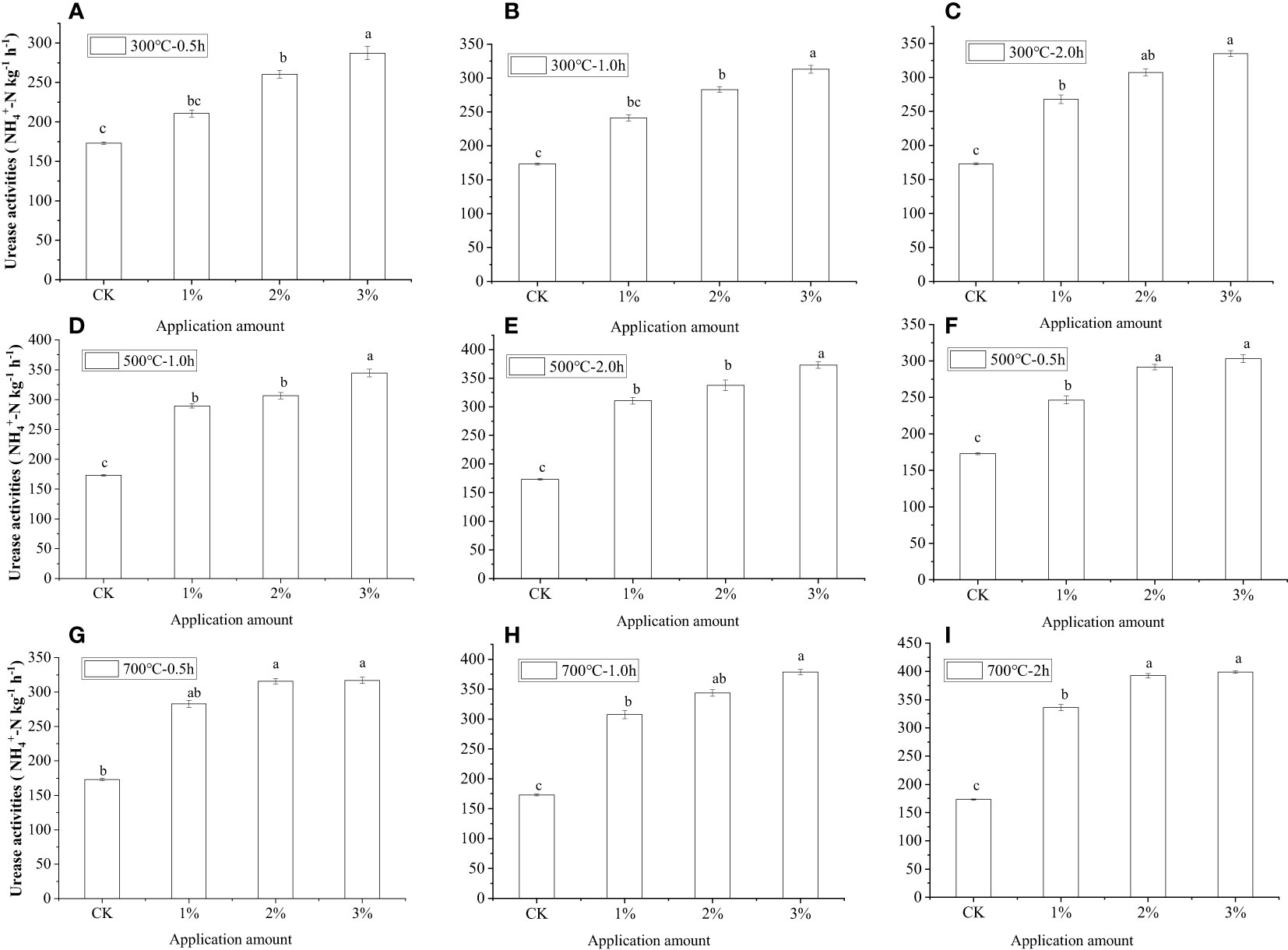
Figure 5 Comparison of urease activities before and after application of water hyacinth biochar. Small letters indicates significant differences.
Biochar application can heighten the activity of soil enzymes through many aspects of regulation. First, previous studies suggest that heavy metals can seriously impact enzyme activity in the soil, and enzyme activity would indirectly reflect the capacity of contaminated soil to self-purify (21–23). The application of biochar reduces the effective Cd concentration in the soil, bringing about the weakening of heavy metal toxicity, which is the main reason cause for the increase of enzyme activity in the soil. Secondly, previous studies suggest that the application of biochar itself can enhance the activity of soil enzymes. The findings were in keeping with Lee et al. (24) and Cui et al. (21), who found that changes in soil enzyme activity were related to an increase in soil pH. There was no doubt that the application of biochar can raise the pH of soil. Moreover, Oleszczuk et al. (25) reported that biochar application could promote water retention and increase the pore structure of the soil. An increase in the cation exchange capacity of soil by water hyacinth biochar had a positive effect on enzyme activity. Furthermore, a number of studies have also indicated that biochar application could result in an increase in soil organic matter and a decrease in soil nutrient loss. It is also an important reason for the increase in enzyme activity in the soil.
Effect of water hyacinth biochar application on soil microbial diversity
Meier et al. (26) showed that biochar application may lead to a variation in microbial structure and the abundance in soil, which is affected by biochar properties and application amount. Therefore, the soil samples were taken and sequenced to investigate the effect of biochar application on the microbial structure and abundance of Cd-contaminated soil on day 40. In Figure 6, different biochar application methods resulted in the different relative abundances of dominant bacteria in Cd-contaminated soil. To begin with, biochar application apparently reduced the relative abundance of proteobacteria. The abundance of proteobacteria with biochar application prepared at 700°C was lower than control. These results indicate that biochar passivation to heavy metals can reduce the number of proteobacteria in soil. The better the passivation performance of biochar applied, the lower the number of proteobacteria in soil. The main reason was that proteobacteria were sensitive to soil pH and negatively correlated with soil pH (27). Obviously, the pH of the soil will increase, resulting in the reduction of proteobacteria after water hyacinth biochar was applied to the soil, which was also the main reason for the evident reduction of acidobacteria in the soil. Secondly, bacteroidetes and firmicutes increased with biochar application significantly. These kinds of microorganisms were more suitable for growing in an environment with high organic content and alkaline pH (28). Adding water hyacinth biochar is an effective way to increase soil organic matter, and the sustained release of biochar can reverse the degradation rate of soil organic matter to a certain extent. Additionally, the application of water hyacinth biochar resulted in an unmistakable increase in actinomycete microorganisms. Kolton et al. (29) showed that an increase in actinomycetes, in general, could contribute to the resistance of crops to pests and diseases. Hence, applying water hyacinth biochar to contaminated soil might play a certain role in plant resistance to pests and diseases as well.
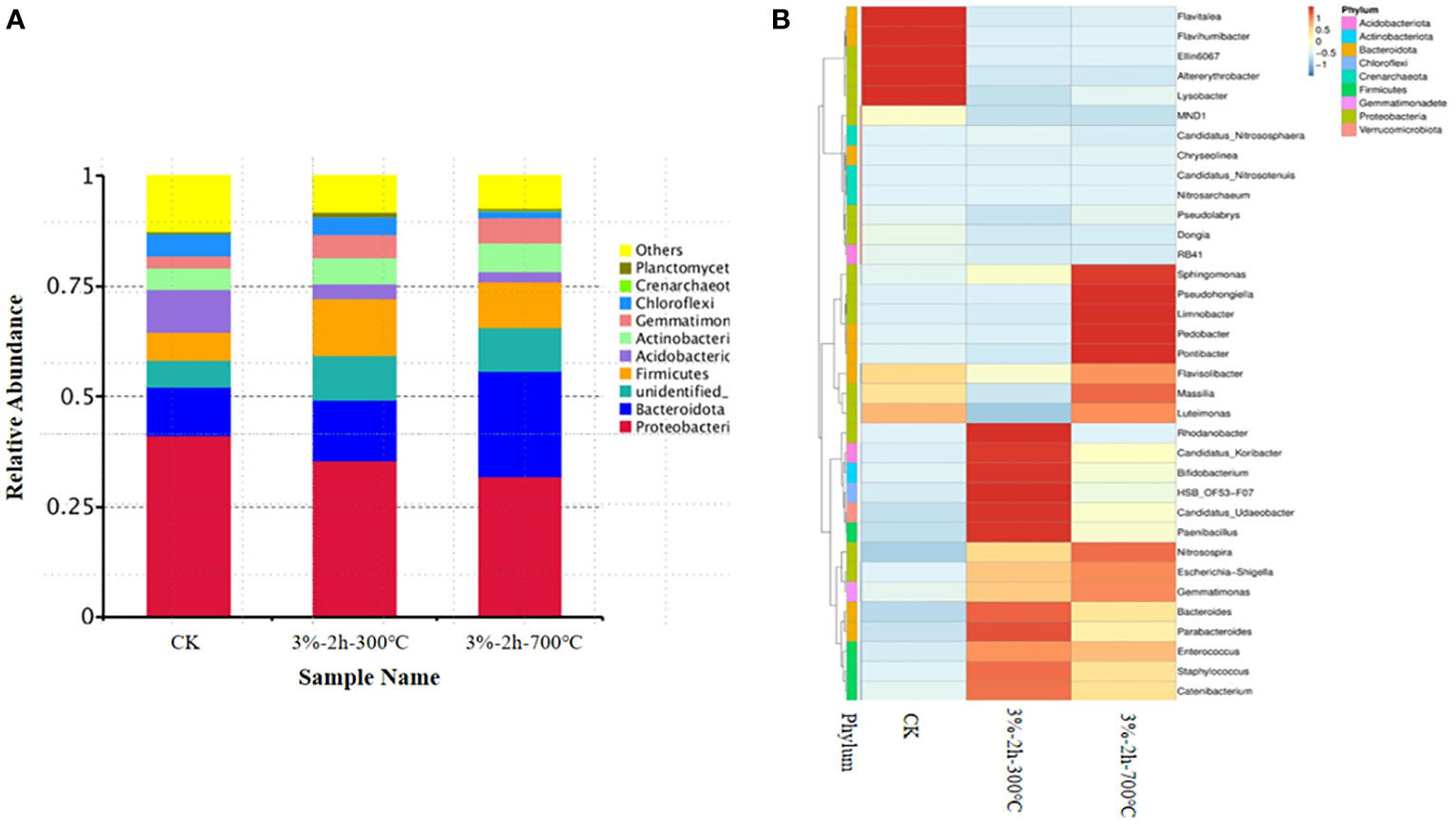
Figure 6 Comparison of soil microbial community structure before and after application of water hyacinth biochar. (A) Bacterial communities are formed at the phylum level. (B) Cluster analysis of species at the phylum level.
Conclusions
The optimal application amount of water hyacinth biochar was 1% (30 t.ha-1) considering the Cd absorption and yield of lettuce plants. The application of water hyacinth biochar prepared at 700℃-2h with the amount of 3% (90 t.ha-1)resulted in a reduction in Cd by 73.6% and 38.1% in the shoots and roots of lettuce. The major cause was that most of the Cd was fixed on the surface of the water hyacinth biochar, generating a 55.8% reduction in available Cd in the soil. Moreover, the biochar treatments could have a possible influence on enzyme and microbial diversity in the soil which was contaminated by heavy metals. In conclusion, biochar derived from water hyacinth, especially at the higher application rates, has the potential to reduce the bioavailability of heavy metals in soil, and consequently, improve the quality of soils and crops.
Data availability statement
The original contributions presented in the study are included in the article/Supplementary Material. Further inquiries can be directed to the corresponding author.
Author contributions
XY: conceptualization, methodology, validation, formal analysis, investigation, resources, data curation, writing—original draft, writing—reviewing and editing, visualization, supervision, project administration, and funding acquisition. CZ: conceptualization, methodology, validation, formal analysis, investigation, resources, data curation, writing—reviewing and editing, and visualization. YW: validation, formal analysis, investigation, and writing—original draft. LW: validation, formal analysis, and investigation. HH: validation, formal analysis, and investigation. CY: validation, formal analysis, and investigation. All authors contributed to the article and approved the submitted version.
Funding
This work was supported by the Natural Science Foundation of China (No. 52160002, 21707057) and the Natural Science Foundation of Jiangxi province (20192BAB213018).
Conflict of interest
The authors declare that the research was conducted in the absence of any commercial or financial relationships that could be construed as a potential conflict of interest.
Publisher’s note
All claims expressed in this article are solely those of the authors and do not necessarily represent those of their affiliated organizations, or those of the publisher, the editors and the reviewers. Any product that may be evaluated in this article, or claim that may be made by its manufacturer, is not guaranteed or endorsed by the publisher.
Supplementary material
The Supplementary Material for this article can be found online at: https://www.frontiersin.org/articles/10.3389/fsoil.2022.998654/full#supplementary-material
References
1. Nie CR, Yang X, Niazi NK, Xu XY, Wen YH, Rinklebe J, et al. Impact of sugarcane bagasse-derived biochar on heavy metal availability and microbial activity: A field study. Chemosphere (2018) 200:274–81. doi: 10.1016/j.chemosphere.2018.02.134
2. Salam A, Bashir S, Khan I. Two years impacts of rapeseed residue and rice straw biochar on Pb and Cu immobilization and revegetation of naturally co-contaminated soil. Appl.Geochem (2019) 105:97–104. doi: 10.1016/j.apgeochem.2019.04.011
3. El-Naggar A, Shaheen SM, Ok YS, Rinklebe J. Biochar affects the dissolved and colloidal concentrations of cd, Cu, Ni, and zn and their phytoavailability and potential mobility in a mining soil under dynamic redox-conditions. Sci Total Environ (2018) 624:1059–71. doi: 10.1016/j.scitotenv.2017.12.190
4. Ahmad M, Rajapaksha AU, Lim JE, Zhang M, Bolan N, Mohan D, et al. Biochar as a sorbent for contaminant management in soil and water: a review. Chemosphere (2014) 99:19–33. doi: 10.1016/j.chemosphere.2013.10.071
5. Beiyuan J, Awad YM, Beckers F, Wang J, Tsang DC, Ok YS, et al. (Im) mobilization and speciation of lead under dynamic redox conditions in a contaminated soil amended with pine sawdust biochar. Environ Int (2020) 135:105376. doi: 10.1016/j.envint.2019.105376
6. Zhang F, Wang X, Ji X, Ma L. Efficient arsenate removal by magnetitemodified water hyacinth biochar. Environ pollut (2016) 216:575–83. doi: 10.1016/j.envpol.2016.06.013
7. Tan Z, Wang Y, Zhang L, Huang Q. Study of the mechanism of remediation of cd-contaminated soil by novel biochars. Environ Sci pollut Res (2017) 24:24844–55. doi: 10.1007/s11356-017-0109-9
8. Zhu X, Chen B, Zhu L, Xing B. Effects and mechanisms of biochar-microbe interactions in soil improvement and pollution remediation: A review. Environ pollut (2017) 227:98–115. doi: 10.1016/j.envpol.2017.04.032
9. Gholizadeh M, Hu X. Removal of heavy metals from soil with biochar composite: A critical review of the mechanism. J Environ Chem Eng. (2021) 9:105830. doi: 10.1016/j.jece.2021.105830
10. Lu J, Wu J, Fu Z. Zhu L. Water hyacinth in China: A sustainability science-based management framework. Environ.l Manage (2007) 40:823–30. doi: 10.1007/s00267-007-9003-4
11. Liu C, Ye J, Lin Y. Removal of cadmium (II) using water hyacinth (Eichhornia crassipes) biochar alginate beads in aqueous solutions. Environ Pollu. (2020) 264:114785. doi: 10.1016/j.envpol.2020.114785
12. Yan SH, Song W, Guo JY. Advances in management and utilization of invasive water hyacinth (Eichhornia crassipes) in a quatic ecosystems-a review. Criti. Rev Biotechnol (2017) 37(2):218–28. doi: 10.3109/07388551.2015.1132406
13. Ebido NE, Edeh IG, Unagwu BO, Nnadi AL, Ozongwu OV, Obalum SE, et al. Ricehusk biochar effects on organic carbon, aggregate stability and nitrogen-fertility of coarse-textured ultisols evaluated using celosia argentea growth. Sains Tanah J Soil Sci Agroclimatology (2021) 18(2):177–87. doi: 10.20961/stjssa.v18i2.56330
14. Yang X, Wang H, Strong P, Xu S, Liu S, Lu K, et al. Thermal properties of biochars derived from waste biomass generated by agricultural and forestry sectors. Energies (2017) 10:469. doi: 10.3390/en10040469
15. Zhang J, Liu J, Liu R. Effects of pyrolysis temperature and heating time on biochar obtained fromthe pyrolysis of straw and lignosulfonate. Bioresour. Technol (2015) 176:288. doi: 10.1016/j.biortech.2014.11.011
16. Ayoub AS, Mcgaw BA, Shand CA. Phytoavailability of cd and zn in soil estimated by stable isotope exchange and chemical extraction. Plant Soil. (2003) 252:291–300. doi: 10.1023/A:1024785201942
17. Kulikowska D, Gusiatin ZM, Bukowska K, Katarzyna K. Humic substances from sewage sludge compost as washing agent effectively remove Cu and cd from soil. Chemosphere (2015) 136:42–9. doi: 10.1016/j.chemosphere.2015.03.083
18. Wang H, Xu X, Ren Z. Removal of phosphate and chromium(VI) from liquids by an amine-crosslinked nano-Fe3O4 biosorbent derived from corn straw. RSC Adv (2016) 6:47237–48. doi: 10.1039/C6RA06801D
19. Xue Y, Wang C, Hu Z, Zhou Y, Xiao Y, Wang T. Pyrolysis of sewage sludge by electromagnetic induction: Biochar properties and application in adsorption removal of Pb(II), Cd(II) from aqueous solution. Waste Manage (2019) 89:48–56. doi: 10.1016/j.wasman.2019.03.047
20. Subrahumnyam G, Shen JP, Liu YR. Effect of long term industrial waste effluent pollution on soil enzyme activities and bacterial community composition. Environ Monit Assess. (2016) 188:112. doi: 10.1007/s10661-016-5099-4
21. Cui H, Zhou J, Zhao Q, Si Y, Mao J, Fang G, et al. Fractions of Cu, cd, and enzyme activities in a contaminated soil as affected by applications of micro- and nanohydroxyapatite. J Soils Sediments. (2013) 13:742–52. doi: 10.1007/s11368-013-0654-x
22. Jia W, Wang B, Wang C, Sun H. Tourmaline and biochar for the remediation of acid soil polluted with heavy metals. J Environ Chem Eng. (2017) 5:2107–14. doi: 10.1016/j.jece.2017.04.015
23. Yang X, Liu J, Mcgrouther K, Huang H, Lu K, Guo X, et al. Effect of biochar on the extractability of heavy metals (Cd, Cu, Pb, and zn) and enzyme activity in soil. Environ Sci pollut Res (2016) 23:974–84. doi: 10.1007/s11356-015-4233-0
24. Lee SE, Ahmad M, Usman AA, Awad YM, Min SH, Yang JE, et al. Effects of biochar on soil quality and heavy metal availability in a military shooting range soil in Korea. Korean J Soil Sci Fert. (2011) 44:67–77. doi: 10.7745/KJSSF.2011.44.1.067
25. Oleszczuk P, Jośko I, Futa B, Pasieczna-Patkowska S, Pałys E, Kraska P. Effect of pesticides on microorganisms, enzymatic activity and plant in biochar-amended soil. Geoderma (2014) 214-215:10–8. doi: 10.1016/j.geoderma.2013.10.010
26. Meier S, Curaqueo G, Khan N, Bolan N, Rilling J, Vidal C, et al. Effects of biochar on copper immobilization and soil microbial communities in a metalcontaminated soil. J Soils Sediments. (2015) 17:1237–50. doi: 10.1007/s11368-015-1224-1
27. Nielsen S, MINCHIN T, KIMBER S. Comparative analysis of the microbial communities in agricultural soil amended with enhanced biochars or traditional fertilisers. Agr. Ecosyst Environ (2014) 191:73–82. doi: 10.1016/j.agee.2014.04.006
28. Pan F, Li Y, Chapman SJ, Khan S, Yao H. Microbial utilization of rice straw and its derived biochar 503 in a paddy soil. Sci Total Environ (2016) 559:15–23. doi: 10.1016/j.scitotenv.2016.03.122
Keywords: water hyacinth biochar, passivation, available Cd, enzyme activities, microbial structure and abundance
Citation: Zhou C, Wang Y, Wei L'e, Huang H, Yu C and Yin X (2022) Effects of water hyacinth biochar on lettuce growth in cadmium-contaminated soil. Front. Soil Sci. 2:998654. doi: 10.3389/fsoil.2022.998654
Received: 20 July 2022; Accepted: 22 September 2022;
Published: 08 November 2022.
Edited by:
Jiefei Mao, Xinjiang Institute of Ecology and Geography (CAS), ChinaReviewed by:
Sunday Ewele Obalum, University of Nigeria, NigeriaJianteng Sun, Guangdong University of Petrochemical Technology, China
Copyright © 2022 Zhou, Wang, Wei, Huang, Yu and Yin. This is an open-access article distributed under the terms of the Creative Commons Attribution License (CC BY). The use, distribution or reproduction in other forums is permitted, provided the original author(s) and the copyright owner(s) are credited and that the original publication in this journal is cited, in accordance with accepted academic practice. No use, distribution or reproduction is permitted which does not comply with these terms.
*Correspondence: Xin Yin, eWlueGluMTA4MUAxNjMuY29t