- Department of Geography, Soil and Water Ecosystems, Philipps-University Marburg, Marburg, Germany
A growing evidence base has shown that plastics are widely distributed in soils and could have negative effects on soil functions. However, within international standards for soil description, plastics are handled so far as one part of human-made artefacts. With the ongoing plastic crisis, such a simple classification may no longer be sufficient to provide a satisfactory description of plastics in soils. Based on the latest research on plastics in soils, these foreign components can no longer be understood as relevant only for soils in urban, industrial, traffic, mining and military areas. This perspective therefore aims to suggest a possible approach towards a future and more comprehensive description of plastics in soil characterization. Based on the existing definitions within the international soil description standards, a description concept and a corresponding field guide are proposed. The proposed approach comprises a recent definition of plastics and guidelines for the description of visible plastic residues in soils during field work. Classification approaches are developed for plastics abundance and distribution as well as plastic characteristics. Furthermore, pitfalls during the description, as well as during the extraction of plastics from soils in the field, and further limitations are discussed. Basic soil description during soil surveys or soil mapping, are a strong tool of soil science to derive environmental data sets. The perspective and the field guide presented in this paper are intended to change this circumstance and enable soil scientists to describe plastic residues in soils simple, comparable and adapted to existing standards in future.
Introduction
Plastics release into, and its preservation within the environment has become an emerging global crisis. In the meantime, the so-called global plastic crisis effects several ecosystems and poses major risks to the environment worldwide (1, 2). After decades of research on plastics within marine and freshwater ecosystems, it has become clear by now that also terrestrial systems are strongly affected by plastics inputs (3, 4). In the meantime, plastic residues have been detected worldwide in a wide variety of soil systems, including different land uses and soil conditions, but with a strong focus on agricultural used soils and therefore limited choice of soil systems (4).
Plastics within the soil environment can be defined as human-made, polymeric, solid and insoluble materials that are released within the environment during their production, each step of their product life-cycles or later deposition (e.g., landfills) (5–7). Most frequent produced and used plastic materials are thermoplastics including polymers like Polyethylene (PE), Polypropylene (PP) or Polyethylene tephraphalate (PET, e.g., PET-bottles) (8). Within the environment, research defines plastic as particles according different sizes (9). Even if this size classification is still under discussion, most scientists differentiate between macroplastics (>25 mm), mesoplastics (25–5 mm), microplastics (5–0.001 mm) in general or large microplastics (5–1 mm) and microplastics (1–0.01 mm) as well as nanoplastics (<0.001 mm/<1 µm) (6, 7). Those plastic particles can enter the environment directly as products within one of the above-mentioned size classes, like PET-bottles, single-use plastic bags or as smaller particles, named as primary microplastics (e.g., within personal care products) (7, 10). Furthermore, each plastic particle or item can be fragmented within the environment through physical, chemical and biological processes (11, 12). In general, plastic materials showing a strong resistance against different degradation processes, through their production and polymeric chemical properties as well as through additional additives (e.g., plasticizer, stabilizers) (11). Under preserved conditions, like buried within soil and therefore shielded against UV-light, plastics seem to be durable for very long time periods (11, 13).
Within soil science, research with a focus on plastics in soils has been taking place only since 2016. Over the last half-decade soil scientific research was able to lay down the evidence that plastics occur within industrial soils (Technosols) (14), garden soils (Hortisol) (15), floodplain soils (Fluvisols, Fluvic Gleysols) (16, 17) and seems to be a very common contamination within agricultural soils (e.g., Entisols, Vertisols, Haploxerols) (18–20) around the globe (4). The possible impacts on soil functions or soil organisms occurring from plastics and microplastics are a major subject of current studies (21, 22). Impacts proven so far, mostly under controlled conditions include impacts on soil physical properties, chemical processes as well as plant-soil relationships and soil organisms (23, 24). Furthermore, related risks include the uptake by plants, the transfer of small plastics to the human food chain and subsequent health risks (24).
The relatively novel finding, that plastics and micro- or nanoplastics are present within soils, may be surprising, since plastic has been considered before in soil description, but also in geoarchaeological research (25). Referring to the international standards for soil description during field surveys (FAO 2006) and the World Reference Base for Soil Resources (WRB 2015) plastics were already mentioned as part of the human-made materials as soil artefacts (26, 27). Additionally, archaeological or geoarchaeological research documents plastics in different soil archives and interpreted these findings as indicators of recent anthropogenic disturbances of the investigated soil archives (28).
Despite the comparatively late focus shift of soil science onto plastics, ongoing research on plastics in soils has to deal with a lack of standardized analytical methods for small plastic particles (micro- to nanoplastic size range) as well as a lack of standardized description concepts for plastic particles or preserved items, regardless of the particle size (29, 30). This lack of standardization or classification, also concerns the description of visible plastics during field work. Until know, visible plastic particles which are identified during soil surveys in the field, must be handled as a part of the soil artefacts according international classification standards (26, 27). However, this circumstance should already be viewed critically on its own, since in particular soils with a respective share of human-made artefacts are often classified as Technosols and seems to be related only to in urban, industrial, traffic, mining and military areas (SUITMA) soils (31).
However, latest research was able to indicate a alleged multiple and spatially widespread evidence of plastics in soils, especially in less anthropogenic affected and shaped soils, outside of SUITMA areas (e.g., 4, 15, 16, 20). Therefore it becomes clear that potentially each soil worldwide, but especially soils under agricultural usage, can contain significant amounts of plastics in different size ranges (4, 21, 23). Within this context and the assumed ongoing increase of global plastic production with a doubling of recent production rates by 2030 (5), it is likely that large quantities of plastics will be continuously released to soil environments despite extensive policy measures (5, 32). A first indication for this future phenomenon, was already investigated through the ongoing COVID-19 pandemic, leading to an increased input of plastics into the environment through the global increased demand of single-use plastics (SUPs, e.g., packaging plastics, face masks) and littering of those SUPs (33).
Global evidence of plastics in soils, an emerging intensification of the global plastic crisis and follow up environmental dissemination and accumulation of plastics in soils, illustrates the need to consider plastic in soils more comprehensively and to collect comparable environmental data. This applies both to focused micro- or nanoplastic studies as well as the documentation of larger meso- and macroplastic residues during field work. The identification of different input pathways of plastics into soils, like littering or agricultural practices (e.g., fertilization through compost or sewage sludge) as examples for primary sources (20, 34, 35), highlights the extent of global plastic inputs in soils and the question of how to deal with plastic detections during soil scientific field works and surveys.
For these reasons, the perspective paper presented here, aims to suggest a feasible way to consider plastics in the soil description more extensively in the future. Oriented to the existing international standards for basic soil description, a methodological approach and classification systems based on the combination of basic soil horizon information and plastic description, will be presented within this perspective. The developed field guide should allow an easy, time-saving and comparable description of plastics in soils, which can be included in soil surveys to enable an improved global database on plastic occurrences in soils. Thereby the perspective focusses on visible plastics within the size of macro- to mesoplastics, as well as coarse microplastics, related to the coarse soil fraction (36).
Theoretical background
Plastics as synthetic polymers and human-made materials, produced from petrochemical raw materials (e.g., natural gas, oil, coal) (7, 11), have become one of the most produced and used materials in the world. Within the post-World War II period, starting within the 1950s, plastics build the basis for the technological revolution in the second half of the 20th century (25). Different properties like easy and low-cost production, versatility, adaptability and resistance to degradation make plastic the most widely used every-day life material (13, 25).
Regarding plastics in soils, reaching soil systems via various possible input pathways, plastics can be considered as anthropogenic contaminants, based on their exclusively human-introduced presence on earth (25). Within this background, plastics are considered clearly as “human-made materials” and part of the anthropogenic “artefacts” class or if present as a membrane, defined as “geomembranes” the international standards of soil description (27). Following the FAO (2006) definitions plastics can be defined as artefacts which are “created by humans as part of an industrial manufacturing process” or as geomembranes “made of polyvinyl chloride (PVC) or high-density polyethylene (HD-PE)”. Within the FAO (2006) guidelines, artefacts can be descripted according the following characteristics: abundance, kind, size, hardness, weathering and colour. However, there is only one class of artefact kinds suitable for plastics, defined as “synthetic solids”. The description and classification of other characteristics follows the rules for rock fragments or mineral nodules.
If following this standardized description options for plastics found in soils, only limited information about plastic characteristics in comparison to current (micro-)plastic descriptions will be obtained (9). In general, the description of the abundance (e.g., particles per kg soil dry weight), size (e.g., given in µm of longest diagonal), surface weathering and colour is also common for the description (micro-)plastics in recent studies (6, 10, 23). However, their description is only difficult to compare to that of rock fragments, as proposed by FAO guidelines. While the abundance classification based on volume (%) can be transferred to plastics, the description of plastic particle types and shapes as an important feature of environmental plastics, must be described in more detail (37). For example, plastics can occur as complete pieces, fragments or films among others, with broken to rounded shapes within the soil environment (38). Even the size classification of plastic, with the usual categories such as macro-, meso- and microplastics, as well as the description of plastics surface degradation (weathering) differs significantly from features of natural rock fragments. These differences highlight the fact that the description of plastics in soils according to the recognized international standards can only be accomplished to a limited extent, with regard to current trends in soil related (micro-)plastic research.
Furthermore and as mentioned above the presence of artefacts or geomembranes is strongly linked to the description and classification of Technosols, as an abundance of ≥ 20 percent (volume or weighted average) leads to the classification of the respective soil as Technosol or the allocation of corresponding qualifiers (26). The examples of soils given in Figure 1, illustrates the occurrence of plastic in Technosols (Figures 1A, B, D, E) as well as in soils cultivated for agricultural purposes (Figures 1C, F–H), underlining that plastics are no longer solely associated to strong anthropogenic shaped soils. Especially the application of plastics during land cultivation through fertilizers (e.g., sewage sludge, compost), intensive use of films (e.g., mulching films), greenhouse practice (e.g., scaffold nets, films) or abrasion from agricultural equipment (e.g., tires) contribute to the expansion of plastic in soils (34, 39), among other factors like general littering (40), flooding within floodplains (17) or deposition of construction materials (31). Furthermore, the examples of plastic bearing soils emphasize that a description of the plastic particles following the existing international standards for soil description remains incomprehensive.
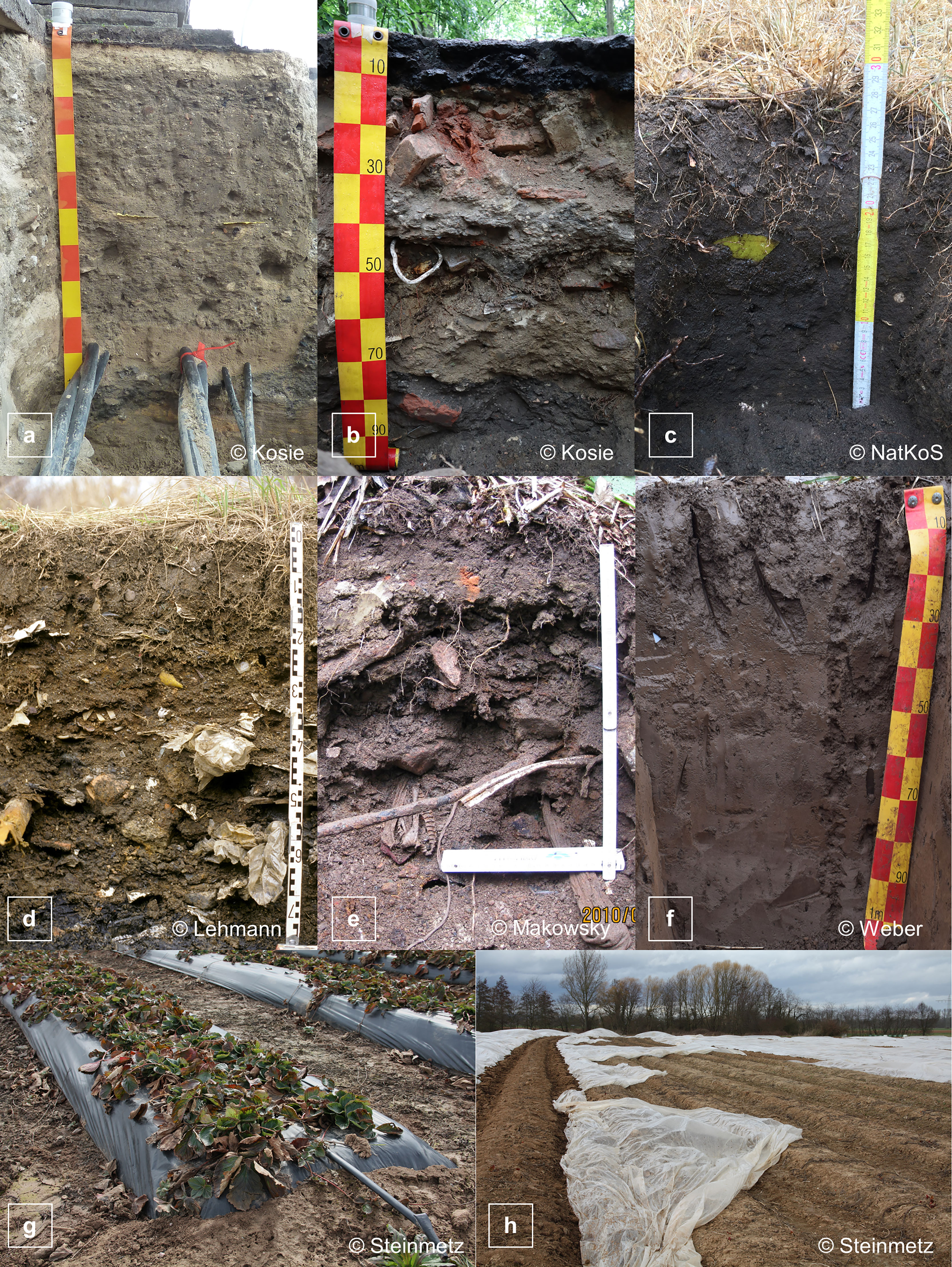
Figure 1 Examples of soils containing plastics. (A) Sealed Technosol including yellow membrane and cables, Berlin (Germany), © Kosie project group, https://hu.berlin/kosie (21.05.2021); (B) Sealed Technosol including debris and waste, Berlin (Germany), © Kosie project group, https://hu.berlin/kosie (24.06.2021); (C) Topsoil of urban green area including yellow plastic fragment, Berlin (Germany), © NatKoS project group, https://hu.berlin/natkos (07.09.2018) and Klingenfuß et al. (2019); (D) Technosol with gleyic properties including debris and waste, Rostock-Warnemünde (Germany), © A Lehmann (University Hohenheim, Germany) (17.05.2007); (E) Technosol including domestic waste, Bielefeld (Germany), © L. Makowsky (IFUA-Projekt-GmbH, Germany) (09.06.2010); (F) Haplic Fluvisol including white plastic fragment, Wetzlar (Germany), © C.J. Weber (07.04.2020); (G) Mulch film on agricultural field (Germany), © Z. Steinmetz (2022, 10.5194/soil-8-31-2022); (H) Plastic films on agricultural field (Germany), © Z. Steinmetz (2022).
Finally, within environmental (micro-)plastic research, especially with a soil related focus, there is a strong need of action for i) data obtaining and ii) data harmonization, since there remains a lack of spatial plastic data in soils and only few comparable plastic descriptions are available (41). If a sufficient data acquisition about plastics in soils comes possible during field work and general soil surveys, following a structured classification scheme aligned with existing classifications, new harmonized data can be obtained and promote further research about plastics in soils. Nevertheless, such plastic identification and description approaches are clearly limited if conducted during field work and soil description. First, the description of plastics is limited to visible (naked-eye) plastics and therefore size dependent, focusing on macroplastics (>5 mm). Even if plastics occur often bright coloured and with eye-catching forms in contrast to the surrounding soil matrix, microplastic particles (<5 mm) will be detected only quite by chance. Second, plastics can be incorporated into the soil structure (e.g., macroaggregates) (42), which hinder a sufficient location, again size dependent. Third, the description of plastics is limited through the formation of descriptive classes, which allow a simplification and data harmonization on the one hand, but need classification and therefore loss of information as well as interpretation on the other hand.
Field guide for plastics detection within soil description
For the future improvement of plastic detection in soils and against the theoretical background presented above, a field guide was developed as part of this perspective to provide a first approach of a more in-depth detection of plastics during soil description. The field guide is available as supplementary information (SI) of this perspective and can be used as detail information or stand alone for field application. Oriented to the international standards of soil description in type and structure, the field guide provides a precise definition of “plastic artefacts”, guidelines for the description of plastics and a brief sampling recommendation as well as a documentation table. It can be applied directly during soil description in the field, follow up the description and documentation of pedogenic features. The proposed field guide contains the following parts: a) Abundance and distribution of plastics, classifying the number of plastic particles and their spatial distribution within each soil horizon; b) description of plastic characteristics including fixation, form and shape, size, degradation, colour and origin.
The application of the field guide can best be illustrated by a straightforward example in combination with the flow chart for plastic description given in Figure 2. Based on a soil profile classified as Halpic Fluvisol (location: N 50.554035, E 8.445300, Lahn River floodplain, Germany) according to WRB (2015), the necessary steps can be best understood. Within the topsoil section, a regular ploughed Ap horizon (0–40 cm, cropland), a single, white plastic fragment made from polyvinyl chloride (PVC) was found during regular soil survey and identified by ATR-FTIR analysis. This single PVC-fragment has a size of 3.5 cm (longest diagonal), broken edges and showing first cracks on the entire surface.
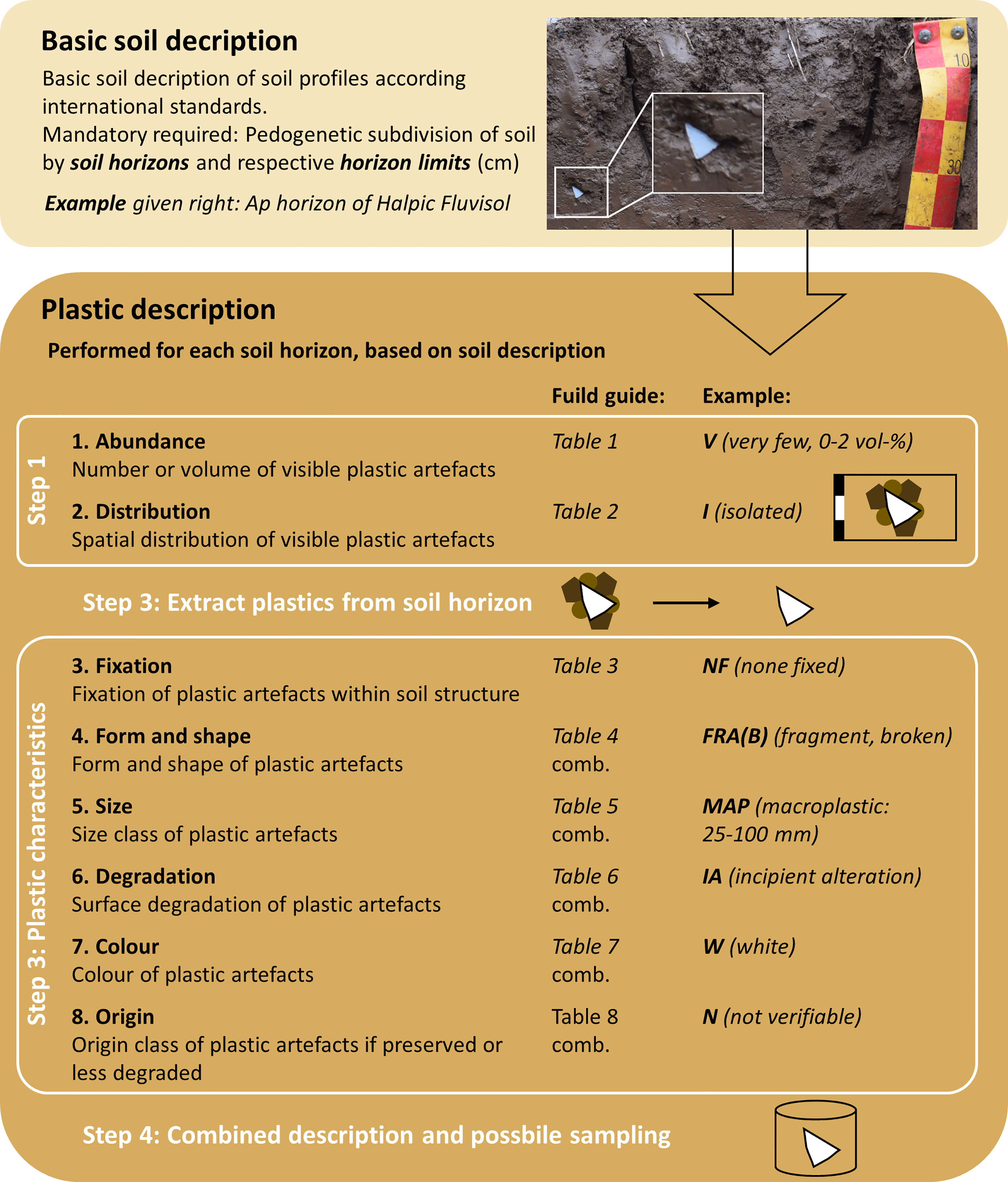
Figure 2 Schematic workflow for plastic detection and description based on the developed field guide (Supplementary Material).
Plastic detection and description can be conducted after basic soil description (required: soil horizons and horizon limits). First, the number of plastic particles and their distribution can be described. After this first step, plastic pieces had to be exposed from the soil profile, organized by depth and location for further detailed description. Afterwards the fixation of plastics within soil structure can be described. This classification was captured, as plastics can be incorporated into the soil structure (42), which makes it necessary to describe the strength of incorporation. Afterwards, plastics form and shape, following typical classes out of (micro-)plastic description and their size can be described and classified. The degradation of plastics, ranging from fresh to strongly degraded is described via the surface properties, as well as subsequently the colour. Finally, the origin of plastic pieces can be descripted, if partly preserved plastic pieces occur. According four classes including, consumer, agricultural, industrial or construction products, the origin can be assigned. Examples for plastic forms, shapes, sizes and degradation are given for macroplastics in Figure S1. Finally, some additional instructions for the sampling of the extracted plastic pieces within the field guide are provided.
Returning to the given example, the completed description of each soil horizon can be given as text, like “Ap horizon of Halpic Fluvisol containing very few [V], isolated [I] plastic artefacts including a single, none fixed [NF], broken fragment [FRA(B)], with incipient alteration [IA], white [W] surface and not verifiable origin [N]”. Furthermore, the description can be shown in abbreviated form like “V,I,NF,FRA(B),IA,W,N” or in table form (SI Table S9). Within datasets, a tabular form is preferable as a data frame based on the abbreviations.
Although the present approach allows for a comparatively simple classification, potential pitfalls exist during plastic description. Despite the general limitations introduced above, one pitfall occurs if several plastic pieces are present within a single soil horizon (e.g., Figure 1D) and quite complex due to the overall heterogeneous spatial distribution of plastic in soils (30, 31, 42). Until know and especially within the field of microplastic sampling and analysis, the study of plastics in soils is mostly related to fixed depths (30, 41). Nevertheless, a horizon-based description of soils, containing general pedogenic characteristics, is the fundamental basis of international soil description (27) and should therefore be retained when trying to develop this description towards a combined plastic description. Moreover, different pedogenic properties which lead to the differentiation of a soil into horizons (43), also have an influence on the potential mobility and relocation of plastics as well as the long-term preservation in soils, like already studied for early cultural or human-made artefacts (44).
This common circumstance in the case of strongly mixed soil substrates, can be overcome through the formation of combined classes (Figure 2, steps 3–8). Different classes like size classification can be combined through a plus symbol (e.g., MAP+CMP). However, this entails the risk of complex classes, hindering subsequent data handling. Finally, the description of single plastic pieces can be time-consuming depending on the number. Therefore, focused and question-oriented work is recommended, with a reduction of the description classes to the required minimum, if necessary.
Outlook
The presented perspective and the developed field guide, should offer an initial opportunity for a simple, straight-forward and harmonized description of plastics within soils. Even though research on plastics in terrestrial systems is still in its infancy, it is clear that more environmental data about plastics is needed to gain a further understanding of plastics occurrence, fate and behaviour in soils. Besides the increasing number of (micro-)plastic related studies, the group of public soil surveys, scientific surveys and special sampling campaigns, which all create soil profiles, could be a strong support to obtain a far-reaching data basis about plastics in soils. The perspective presented here should be used to discuss this topic more deeply in the future, to apply and improve the field guide and to make new recommendations. Therefore, it becomes important to proof the presented concepts in future and discuss changes and additions from practical application in the field. Also, the integration of the classification suggestions into simple online or app tools, and thus an extension to describe plastics also on soil surfaces in the context of citizen science would be an important future goal.
Data availability statement
The original contributions presented in the study are included in the article/Supplementary Material. Further inquiries can be directed to the corresponding author.
Author contributions
The author confirms sole responsibility for the following: study conception and design, data collection, analysis and interpretation of results, and manuscript preparation.
Funding
The author acknowledges facilitating this work through a PhD Scholarship from the Marburg University Research Academy (MARA).
Conflict of interest
The authors declares that the research was conducted in the absence of any commercial or financial relationships that could be construed as a potential conflict of interest.
Publisher’s note
All claims expressed in this article are solely those of the authors and do not necessarily represent those of their affiliated organizations, or those of the publisher, the editors and the reviewers. Any product that may be evaluated in this article, or claim that may be made by its manufacturer, is not guaranteed or endorsed by the publisher.
Acknowledgments
The author acknowledges facilitating this work through a PhD Scholarship from the Marburg University Research Academy (MARA). Furthermore, I would like to thank the Kosie (https://hu.berlin/kosie), and NatKoS (https://hu.berlin/natkos) project groups (Humboldt-Universität zu Berlin, Germany), Lutz Makowsky (IFUA-Projekt-GmbH, Bielefeld, Germany), Andreas Lehmann (University Hohenheim, Germany) and Zacharias Steinmetz (University Koblenz-Landau, Germany) for providing example pictures of plastic containing soil profiles or agricultural fields displayed in Figure 1.
Supplementary material
The Supplementary Material for this article can be found online at: https://www.frontiersin.org/articles/10.3389/fsoil.2022.917490/full#supplementary-material
References
1. Tulus V, Pérez-Ramírez J, Guillén-Gosálbez G. Planetary metrics for the absolute environmental sustainability assessment of chemicals. Green Chem (2021) 23:9881–93. doi: 10.1039/D1GC02623B
2. Shen M, Huang W, Chen M, Song B, Zeng G, Zhang Y. (Micro)plastic crisis: Un-ignorable contribution to global greenhouse gas emissions and climate change. J Cleaner Prod (2020) 254:120138. doi: 10.1016/j.jclepro.2020.120138
3. de Souza Machado AA, Kloas W, Zarfl C, Hempel S, Rillig MC. Microplastics as an emerging threat to terrestrial ecosystems. Global Change Biol (2018) 24:1405–16. doi: 10.1111/gcb.14020
4. Koutnik VS, Leonard J, Alkidim S, DePrima FJ, Ravi S, Hoek EM, et al. Distribution of microplastics in soil and freshwater environments: Global analysis and framework for transport modeling. Environ pollut (2021) 274:116552. doi: 10.1016/j.envpol.2021.116552
5. Syberg K, Nielsen MB, Oturai NB, Clausen LP, Ramos TM, Hansen SF. Circular economy and reduction of micro(nano)plastics contamination. J Hazard Mater Adv (2022) 5:100044. doi: 10.1016/j.hazadv.2022.100044
6. Hartmann NB, Hüffer T, Thompson RC, Hassellöv M, Verschoor A, Daugaard AE, et al. Are we speaking the same language? recommendations for a definition and categorization framework for plastic debris. Environ Sci Technol (2019) 53:1039–47. doi: 10.1021/acs.est.8b05297
7. Andrady AL. The plastic in microplastics: A review. Mar pollut Bull (2017) 119:12–22. doi: 10.1016/j.marpolbul.2017.01.082
8. PlasticsEurope. Plastics - the facts 2021: An analysis of European plastic production, demand and waste data (2021). Available at: https://plasticseurope.org/knowledge-hub/plastics-the-facts-2021/.
9. Kooi M, Koelmans AA. Simplifying microplastic via continuous probability distributions for size, shape, and density. Environ Sci Technol Lett (2019) 6:551–7. doi: 10.1021/acs.estlett.9b00379
10. Wang W, Ge J, Yu X, Li H. Environmental fate and impacts of microplastics in soil ecosystems: Progress and perspective. Sci Total Environ (2020) 708:134841. doi: 10.1016/j.scitotenv.2019.134841
11. Chamas A, Moon H, Zheng J, Qiu Y, Tabassum T, Jang JH, et al. Degradation rates of plastics in the environment. ACS Sustain Chem Eng (2020) 8:3494–511. doi: 10.1021/acssuschemeng.9b06635
12. Napper IE, Thompson RC. Environmental deterioration of biodegradable, oxo-biodegradable, compostable, and conventional plastic carrier bags in the Sea, soil, and open-air over a 3-year period. Environ Sci Technol (2019) 52(9):4775–83. doi: 10.1021/acs.est.8b06984
13. Bancone CE, Turner SD, Ivar do Sul JA, Rose NL. The paleoecology of microplastic contamination. Front Environ Sci (2020) 8:574008. doi: 10.3389/fenvs.2020.574008
14. Fuller S, Gautam A. A procedure for measuring microplastics using pressurized fluid extraction. Environ Sci Technol (2016) 50:5774–80. doi: 10.1021/acs.est.6b00816
15. Huerta Lwanga E, Mendoza Vega J, Ku Quej V, Jd C, Sanchez Del Cid L, Chi C, et al. Field evidence for transfer of plastic debris along a terrestrial food chain. Sci Rep (2017) 7:14071. doi: 10.1038/s41598-017-14588-2
16. Weber CJ, Opp C, Prume JA, Koch M, Andersen TJ, Chifflard P. Deposition and in-situ translocation of microplastics in floodplain soils. Sci Total Environ (2021) 819:152039. doi: 10.1016/j.scitotenv.2021.152039
17. Lechthaler S, Esser V, Schüttrumpf H, Stauch G. Why analysing microplastics in floodplains matters: application in a sedimentary context. Environ Sci: Processes Impacts (2021) 71:299. doi: 10.1039/D0EM00431F
18. van den Berg P, Huerta-Lwanga E, Corradini F, Geissen V. Sewage sludge application as a vehicle for microplastics in eastern Spanish agricultural soils. Environ pollut (2020) 261:114198. doi: 10.1016/j.envpol.2020.114198
19. Corradini F, Meza P, Eguiluz R, Casado F, Huerta-Lwanga E, Geissen V. Evidence of microplastic accumulation in agricultural soils from sewage sludge disposal. Sci Total Environ (2019) 671:411–20. doi: 10.1016/j.scitotenv.2019.03.368
20. Piehl S, Leibner A, Löder MG, Dris R, Bogner C, Laforsch C. Identification and quantification of macro- and microplastics on an agricultural farmland. Sci Rep (2018) 8:17950. doi: 10.1038/s41598-018-36172-y
21. Wang J, Liu X, Li Y, Powell T, Wang X, Wang G, et al. Microplastics as contaminants in the soil environment: A mini-review. Sci Total Environ (2019) 691:848–57. doi: 10.1016/j.scitotenv.2019.07.209
22. Selonen S, Dolar A, Jemec Kokalj A, Skalar T, Parramon Dolcet L, Hurley R, et al. Exploring the impacts of plastics in soil - the effects of polyester textile fibers on soil invertebrates. Sci Total Environ (2020) 700:134451. doi: 10.1016/j.scitotenv.2019.134451
23. He D, Luo Y, Lu S, Liu M, Song Y, Lei L. Microplastics in soils: Analytical methods, pollution characteristics and ecological risks. TrAC Trends Anal Chem (2018) 109:163–72. doi: 10.1016/j.trac.2018.10.006
24. He D, Zhang Y, Gao W. Micro(nano)plastic contaminations from soils to plants: human food risks. Curr Opin Food Sci (2021) 41:116–21. doi: 10.1016/j.cofs.2021.04.001
25. Zalasiewicz J, Waters CN, Ivar do Sul JA, Corcoran PL, Barnosky AD, Cearreta A, et al. The geological cycle of plastics and their use as a stratigraphic indicator of the anthropocene. Anthropocene (2016) 13:4–17. doi: 10.1016/j.ancene.2016.01.002
26. IUSS Working Group. World reference base for soil resources 2014, update 2015: International soil classification system for naming soils and creating legends for soil maps. World Soil Resour Reports FAO (2015).
27. FAO. Guidelines for soil description. Rome: Food and Agriculture Organization of the United Nations (2006). 97.
28. Mytum H, Meek J. The iron age in the plastic age: Anthropocene signatures at castell henllys. Antiquity (2021) 95:198–214. doi: 10.15184/aqy.2020.237
29. Thomas D, Schütze B, Heinze WM, Steinmetz Z. Sample preparation techniques for the analysis of microplastics in soil–a review. Sustainability (2020) 12:9074. doi: 10.3390/su12219074
30. Möller JN, Löder MG, Laforsch C. Finding microplastics in soils: A review of analytical methods. Environ Sci Technol (2020) 54:2078–90. doi: 10.1021/acs.est.9b04618
31. Burghardt W, Morel JL, Zhang G-L. Development of the soil research about urban, industrial, traffic, mining and military areas (SUITMA). Soil Sci Plant Nutr (2015) 61:3–21. doi: 10.1080/00380768.2015.1046136
32. Chen Y, Awasthi AK, Wei F, Tan Q, Li J. Single-use plastics: Production, usage, disposal, and adverse impacts. Sci Total Environ (2021) 752:141772. doi: 10.1016/j.scitotenv.2020.141772
33. Dharmaraj S, Ashokkumar V, Hariharan S, Manibharathi A, Show PL, Chong CT, et al. The COVID-19 pandemic face mask waste: A blooming threat to the marine environment. Chemosphere (2021) 272:129601. doi: 10.1016/j.chemosphere.2021.129601
34. Braun M, Mail M, Heyse R, Amelung W. Plastic in compost: Prevalence and potential input into agricultural and horticultural soils. Sci Total Environ (2021) 760:143335. doi: 10.1016/j.scitotenv.2020.143335
35. Brandes E, Henseler M, Kreins P. Identifying hot-spots for microplastic contamination in agricultural soils–a spatial modelling approach for Germany. Environ Res Lett (2021) 16:104041. doi: 10.1088/1748-9326/ac21e6
36. Weber CJ, Opp C. Spatial patterns of mesoplastics and coarse microplastics in floodplain soils as resulting from land use and fluvial processes. Environ pollut (2020) 267:115390. doi: 10.1016/j.envpol.2020.115390
37. Hidalgo-Ruz V, Gutow L, Thompson RC, Thiel M. Microplastics in the marine environment: a review of the methods used for identification and quantification. Environ Sci Technol (2012) 46:3060–75. doi: 10.1021/es2031505
38. Cao L, Wu Di, Liu P, Hu W, Xu L, Sun Y, et al. Occurrence, distribution and affecting factors of microplastics in agricultural soils along the lower reaches of Yangtze river, China. Sci Total Environ (2021) 794:148694. doi: 10.1016/j.scitotenv.2021.148694
39. Tagg AS, Brandes E, Fischer F, Fischer D, Brandt J, Labrenz M. Agricultural application of microplastic-rich sewage sludge leads to further uncontrolled contamination. Sci Total Environ (2022) 806:150611. doi: 10.1016/j.scitotenv.2021.150611
40. Cowger WC, Gray A, Hapich H, Osei-Enin J, Olguin S,JR, Huynh B, et al. Litter origins, accumulation rates, and hierarchical composition on urban roadsides of the inland empire, California. Environ Res Lett (2021) 17:015007. doi: 10.1088/1748-9326/ac3c6a
41. Weber CJ, Weihrauch C, Opp C, Chifflard P. Investigating microplastic dynamics in soils: Orientation for sampling strategies and sample pre-procession. Land Degrad Dev (2020) 31(1):270–84. doi: 10.1002/ldr.3676
42. Zhang GS, Liu YF. The distribution of microplastics in soil aggregate fractions in southwestern China. Sci Total Environ (2018) 642:12–20. doi: 10.1016/j.scitotenv.2018.06.004
43. Hartemink AE, Zhang Y, Bockheim JG, Curi N, Silva SHG, Grauer-Gray J, et al. Soil horizon variation: a review. Adv Agron (2020) 160:125–85. doi: 10.1016/bs.agron.2019.10.003
Keywords: macroplastic, mesoplastic, sampling, soil mapping, field work, anthropogenic
Citation: Weber CJ (2022) Plastics in soil description and surveys – practical considerations and field guide. Front. Soil Sci. 2:917490. doi: 10.3389/fsoil.2022.917490
Received: 11 April 2022; Accepted: 29 June 2022;
Published: 18 July 2022.
Edited by:
Shan-Li Wang, National Taiwan University, TaiwanReviewed by:
Tariq Mehmood, Hainan University, ChinaCopyright © 2022 Weber. This is an open-access article distributed under the terms of the Creative Commons Attribution License (CC BY). The use, distribution or reproduction in other forums is permitted, provided the original author(s) and the copyright owner(s) are credited and that the original publication in this journal is cited, in accordance with accepted academic practice. No use, distribution or reproduction is permitted which does not comply with these terms.
*Correspondence: Collin J. Weber, Y29sbGluLndlYmVyQGdlby51bmktbWFyYnVyZy5kZQ==